DOI:
10.1039/C0SC00176G
(Edge Article)
Chem. Sci., 2010,
1, 119-125
Enabling tetracationic cyclophane production by trading templates†
Received
15th February 2010
, Accepted 1st April 2010
First published on
12th May 2010
Abstract
The time taken to produce the ubiquitous and promiscuous receptor, which has become known as the little “blue box”, has been halved as a result of using a pH-responsive derivative of 1,5-diaminonaphthalene to displace the template employed during its synthesis. The fact that this surrogate trades places within the cavity of the “blue box” is the key to the time-saving for the simple reason that it leaves the lock, so-to-speak, as soon as it becomes protonated by a strong acid. During the subsequent full characterisation of the “blue box”, it was discovered that the tetracationic cyclophane exists at least in two different polymorphs in the solid state.
Introduction
Cyclobis(paraquat-p-phenylene)1,2 (CBPQT4+) has played a key role in the development of both supramolecular chemistry3 and mechanostereochemistry4 for over two decades now. In tune with the gradual appearance of colour in the literature for the representation of the structural formulae of chemical compounds, this tetracationic cyclophane has earned for itself the nickname of the little “blue box” in the wider community of macrocyclic chemists.5 It is a rather unique compound for a variety of reasons—they include its dimensions, its shape, its rigidity, its strain, its electronic and redox properties and, not least of all, its charge. When the tetracation is accompanied by soft counterions (e.g., PF6−, BF4−), the salt is easily soluble in polar organic solvents (e.g., MeCN, MeNO2), yet when the counterions are hard (e.g., Cl−, Br−), it can be readily dissolved in aqueous solutions. Moreover, in polar organic solvents, the tetracationic cyclophane plays host in a promiscuous manner, forming 1
:
1 complexes with a wide range of π-electron rich guests, including hydroquinone,6 1,5-dioxynaphthalene derivatives,7 tetrathiafulvalene8 and aromatic amino acids.9 Also, it has been reported10 that the CBPQT4+ tetracation can assume the role of a π-electron deficient guest trapped inside the cavities of much larger π-electron rich, macrocyclic hosts. In the immediate past, we have demonstrated11 additionally a very strong noncovalent bonding interaction between CBPQT4+ and an included viologen species (V2+) when all three bipyridinium units are reduced to radical cations forming the [V˙+⊂CBPQT2(˙+)] complex: the strong radical pair interactions—also known as pimerization12—between the V˙+ guest and the CBPQT2(˙+) host represent a new binding motif in host–guest chemistry.13 The special characteristics and unique properties, associated with CBPQT4+, have led logically to the template-directed syntheses14 of mechanically interlocked molecules4,15 (MIMs), such as catenanes15,16 and rotaxanes15,17 which have spawned applications in fields as disparate as those involving molecular switches and machines,18 molecular electronic devices19 (MEDs), nanoelectromechanical systems20 (NEMS), and self-assembling nanosystems21 and nanoparticles.22 All these multifarious applications of CBPQT4+ require that the synthesis of this tetracationic cyclophane be straightforward, as well as efficient.
Since the birth1 of the “blue box” in 1988, the application of template-directed protocols14 to the production of the tetracationic cyclophane has led to dramatically increased yields, using as templates, for example, 1,5-bis[2-(2-hydroxyethoxy)ethoxy]naphthalene2c (BHEEN) or 1,5-bis-[2-(2-methoxyethoxy)ethoxy]naphthalene2d (BMEEN). Furthermore, the use of an ultra-high pressure (12–15 kbar) reactor, in conjunction with an adherence to template-directed protocols,14 led to a significant curtailing of reaction times while simultaneously improving the yields. Nonetheless, it is the purification of the tetracationic cyclophane, involving the removal of the template, by means of a time-consuming and energy-wasteful liquid–liquid extraction, where the efficiency of preparing quantities of CBPQT·4PF6 falters. During the liquid–liquid extraction procedure, the crude reaction mixture containing [template⊂CBPQT]·4Cl is solubilised in water, while the template—i.e. BHEEN or BMEEN—is extracted from the 1
:
1 complex by passing drops of chloroform through the aqueous layer during a period ranging from two days to more than a week. Following counterion exchange of the chloride ion with ammonium hexafluorophosphate, the crude product is then subjected to column chromatography on silica gel to afford pure CBPQT·4PF6. In this edge article, we report on a much improved isolation and purification procedure (Scheme 1) for obtaining rather pure CBPQT·4PF6. It involves the use of 1,5-bis[2-(2-hydroxyethoxy)ethylamino]naphthalene (BHEAN) (see ESI† for its preparation from 1,5-diaminonaphthalene) as a pH-responsive “template-exchanging” reagent which is capable of displacing the template from the [BHEEN⊂CBPQT]·4Cl complex, on account of the higher binding affinity of BHEAN—compared with BHEEN—and forming the [BHEAN⊂CBPQT]·4Cl complex by employing Le Chatelier's principle through addition of excess of BHEAN. The fact that this new complex can be disrupted very easily on addition of acid, and hence protonation of the amino functional groups in BHEAN to give BHEAN-H22+, eliminates the need for the lengthy liquid–liquid extraction step, thus reducing the work-up of CBPQT·4PF6 from more than a week to 30 min.
![Schematic representation of the template-directed synthesis and the subsequent purification steps in order to obtain CBPQT·4PF6 using BHEAN as a template-exchanging reagent. The CBPQT4+ ring was first of all constructed using BHEEN as the template under 15 kbar pressure in DMF. The purification steps involved (A) addition of BHEAN to an aqueous solution of [BHEEN⊂CBPQT]·4Cl, resulting in the substitution of BHEEN by BHEAN to form [BHEAN⊂CBPQT]·4Cl. (B) The subsequent protonation of the aromatic amino functionalities in BHEAN (by addition of conc. HCl) (C) effected the decomplexation of BHEAN from the cavity of the CBPQT4+ ring as a result of Coulombic repulsion. Note: The hypothetical [BHEAN-2H⊂CBPQT]·6Cl species is included for conceptual purposes only. Indeed, the decomplexation may occur after the mono-protonation of BHEAN. (D) A counterion exchange with NH4PF6 produced a white precipitate of CBPQT·4PF6, which was filtered and washed several times with H2O in order to isolate a high quality product.](/image/article/2010/SC/c0sc00176g/c0sc00176g-s1.gif) |
| Scheme 1 Schematic representation of the template-directed synthesis and the subsequent purification steps in order to obtain CBPQT·4PF6 using BHEAN as a template-exchanging reagent. The CBPQT4+ ring was first of all constructed using BHEEN as the template under 15 kbar pressure in DMF. The purification steps involved (A) addition of BHEAN to an aqueous solution of [BHEEN⊂CBPQT]·4Cl, resulting in the substitution of BHEEN by BHEAN to form [BHEAN⊂CBPQT]·4Cl. (B) The subsequent protonation of the aromatic amino functionalities in BHEAN (by addition of conc. HCl) (C) effected the decomplexation of BHEAN from the cavity of the CBPQT4+ ring as a result of Coulombic repulsion. Note: The hypothetical [BHEAN-2H⊂CBPQT]·6Cl species is included for conceptual purposes only. Indeed, the decomplexation may occur after the mono-protonation of BHEAN. (D) A counterion exchange with NH4PF6 produced a white precipitate of CBPQT·4PF6, which was filtered and washed several times with H2O in order to isolate a high quality product. | |
Experimental section
The production of the tetracationic cyclophane in a time-efficient manner is based initially on procedures2 reported in the literature.
CBPQT·4PF6: 1,1′-[1,4-phenylenebis(methylene)]bis(4,4′-bipyridinium) bis(hexafluorophosphate)1,2 (250 mg, 0.35 mmol), 1,4-bis(bromomethyl)benzene (93 mg, 0.35 mmol) and BHEEN (353 mg, 1.05 mmol) were dissolved in anhydrous DMF (10 mL). The reaction mixture was subjected to a pressure of 15 kbar in a Teflon high-pressure reaction vessel for 3 days at room temperature. The solvent was then removed under vacuum, and the resulting solid was partitioned between a saturated aqueous NH4Cl solution (200 mL) and CH2Cl2 (2 × 100 mL) to generate the [BHEEN⊂CBPQT]4+ complex as the tetrachloride salt, while transferring the free BHEEN into the organic layer. An excess of BHEAN (588 mg, 1.75 mmol) in MeCN (20 mL) was added to the aqueous solution, whereupon it changed colour from purple to green (see UV-Vis spectrophotometric titration, Fig. S5, ESI†) indicating the displacement of the BHEEN template from the 1
:
1 complex and the formation of the [BHEAN⊂CBPQT]4+ complex. The solution was washed with CH2Cl2 (2 × 100 mL) in order to remove the free BHEEN. A second batch of BHEAN (234 mg, 0.7 mmol) in MeCN (10 mL) was added to the aqueous solution, followed by a second washing of the aqueous solution with CH2Cl2 (2 × 100 mL) to ensure the near-complete displacement of the BHEEN template. Upon addition of an excess of HCl (12 M), the colour of the aqueous solution changed from intense green to light yellow, indicating the decomplexation of the [BHEAN⊂CBPQT]4+ complex to BHEAN-H22+ and CBPQT4+. Excess NH4PF6 was added to the aqueous solution in order to convert the counterions from Cl− to PF6−, resulting in the formation of a white precipitate of CBPQT·4PF6 which was isolated in close-to-pure form (163 mg, 42%)23 as indicated by 1H NMR spectroscopy (Fig. 1b).
![1H NMR spectra of (a) BHEAN, (b) CBPQT·4PF6 and (c) [BHEAN⊂CBPQT]·4PF6 in CD3CN at 233 K. Note: the CBPQT·4PF6 employed to run the spectrum recorded in (b) was purified by the method described herein without any chromatography. The CBPQT·4PF6 employed to run the spectrum in (c) was purified further in order to remove oligomeric by-products (cyclic and acyclic), denoted by * in (b).](/image/article/2010/SC/c0sc00176g/c0sc00176g-f1.gif) |
| Fig. 1
1H NMR spectra of (a) BHEAN, (b) CBPQT·4PF6 and (c) [BHEAN⊂CBPQT]·4PF6 in CD3CN at 233 K. Note: the CBPQT·4PF6 employed to run the spectrum recorded in (b) was purified by the method described herein without any chromatography. The CBPQT·4PF6 employed to run the spectrum in (c) was purified further in order to remove oligomeric by-products (cyclic and acyclic), denoted by * in (b). | |
Results and discussion
While the 1,5-dioxynaphthalene (DNP) system has been used widely as a recognition unit for CBPQT4+ in a host of MIMs, the constitutionally similar 1,5-diaminonaphthalene (DAN) has remained unexplored so far as a substrate for CBPQT4+. A preliminary experiment involving simply mixing DAN with CBPQT·4PF6 resulted in a deep blue-coloured solution, indicating the immediate formation of a complex. This observation prompted us to synthesise BHEAN (See ESI†). Before exploring the potential of BHEAN as a template trading partner with BHEEN, we evaluated the host–guest characteristics of the [BHEAN⊂CBPQT]·4PF6 complex by UV-Vis, 1D and 2D 1H NMR spectroscopy, isothermal titration microcalorimetry (ITC) and X-ray crystallography.
When BHEAN and CBPQT·4PF6 were mixed in a 1
:
1 molar ratio in CD3CN, the mixing at concentrations of 2.7 mM in substrate and receptor resulted in a green-coloured (λmax = 703 nm, Fig. S3, ESI†) solution, indicative of a charge-transfer interaction between the π-electron rich naphthalene ring system and the π-electron deficient bipyridinium units present in CBPQT4+, as the immediate outcome. The 1H NMR spectrum exhibited broad peaks at room temperature, a phenomenon which can be attributed to slow complexation–decomposition on the 1H NMR time-scale. On cooling the solution down to 233 K, however, the resonances for the [BHEAN⊂CBPQT]4+ complex became much better resolved as a consequence of slowing down the dynamic process. Fig. 1a records the 1H NMR spectrum of the free BHEAN. Fig. 1b shows the 1H NMR spectrum of the free CBPQT·4PF6 prepared here by the “template-exchanging” protocol. On complexation of this host with BHEAN, the resonances (Fig. 1c) arising from both the host and the guest undergo considerable shifts in keeping with the formation of a [BHEAN⊂CBPQT]·4PF6 complex. The changes in chemical shifts are listed as Δδ values in Table 1. These 1H NMR spectroscopic data support the hypothesis that the host–guest geometry in the case of the [BHEAN⊂CBPQT]4+ complex is very similar to that of the [BHEEN⊂CBPQT]4+ complex.
Table 1 The chemical shift changes (Δδ)a observed in 1H NMR spectra for an equimolar mixture (2.7 mM) of CBPQT·4PF6 with (1) BHEEN and (2) BHEAN relative to the free components—i.e., CBPQT·4PF6, BHEEN and BHEAN—recorded in CD3CN at 233 K
1 : 1 Stoichiometry |
Δδ for naphthalene protons |
Δδ for CBPQT4+ protonsb |
2/6 |
3/7 |
4/8 |
α |
β |
CH2N+ |
C6H4 |
Δδ is defined as: Δδ = δcomplex − δfree BHEAN/BHEEN/CBPQT4+. δcomplex is the chemical shift of a particular component, namely BHEAN, BHEEN or CBPQT·4PF6, in the complex in CD3CN at 233 K. δfree BHEAN/BHEEN/CBPQT4+ is defined as the chemical shift of free BHEAN, BHEEN or CBPQT·4PF6 in CD3CN at 233 K.
The 1H NMR signals for the constitutionally homotopic CBPQT4+ protons all separate into two sets of peaks as a consequence of the local C2 symmetry imparted on the host by the guests (BHEEN or BHEAN) upon complexation to give 1 : 1 inclusion complexes.
|
BHEEN : CBPQT·4PF6 |
−0.73 |
−1.48 |
−5.44 |
+0.21 |
−0.99 |
−0.09 |
+0.55 |
−0.27 |
−0.77 |
+0.08 |
+0.36 |
BHEAN : CBPQT·4PF6 |
−0.72 |
−1.34 |
−5.97 |
+0.24 |
−1.02 |
−0.16 |
+0.57 |
−0.28 |
−0.91 |
+0.05 |
+0.41 |
While a solid-state superstructure of the [BHEAN⊂CBPQT]4+ complex could add some additional authority (vide infra) to the claim that the host–guest geometry of the [BHEAN⊂CBPQT]4+ species is very similar to that of the [BHEEN⊂CBPQT]4+ complex in solution, we needed to know the relative strengths of the two complexes in solution as well as confirm the stoichiometry of the [BHEAN⊂CBPQT]4+ species in order to evaluate its potential as an effective template-exchanging reagent. In search of an answer to this question, we decided to carry out ITC experiments on both complexes in MeCN solution (Table 2). They revealed that the association constant (Ka = 58
000 M−1) for 1
:
1 complexation (confirmed also by means of a Job plot, Fig. S4, ESI†) between CBPQT·4PF6 and BHEAN is marginally higher than the Ka value (36
400 M−1)24 for the 1
:
1 complex formed between CBPQT·4PF6 and BHEEN. We reasoned that, if we were to use an excess of BHEAN to carry out the “template exchange”, then it would displace BHEEN from [BHEEN⊂CBPQT]4+ and form [BHEAN⊂CBPQT]4+ as the most thermodynamically stable product. Indeed, it can be done. Employing the two Ka values, we reached the conclusion (see ESI†) that adding a five-fold excess of BHEAN to a solution of the [BHEEN⊂CBPQT]4+ complex would result in an approximately 90% displacement of BHEEN—by BHEAN—from the cavity of the CBPQT4+ ring. Moreover, repeating this procedure twice was predicted to effect almost complete exchange. Since BHEAN can be easily protonated to form BHEAN-H22+, the template can be readily expelled from inside the tetracationic cyclophane in seconds.
Table 2 The thermodynamic binding data corresponding to the complexation between CBPQT·4PF6 host with (1) BHEENa and (2) BHEANb as guests in MeCN, as determined by ITC at 298 K
Guest |
ΔH°/kcal mol−1 |
TΔS°/kcal mol−1 |
ΔG°298 K/kcal mol−1 |
K
a/× 103 M−1 |
ref. 24.
0.39 mM standard solution of CBPQT·4PF6 was used for the titration into which a solution of 3.9 mM conc. of the BHEAN was added in 5 μL aliquots. Fits were performed using Microcal LLC software. The stoichiometries of the complexes were around 0.9, indicating that a 1 : 1 complex was formed.
|
(1) BHEEN |
−15.4 ± 0.1 |
−9.1 ± 0.1 |
−6.26 ± 0.04 |
36.4 ± 0.3 |
(2) BHEAN |
−7.2 ± 0.2 |
−0.7 ± 0.01 |
−6.47 ± 0.06 |
58.0 ± 0.2 |
Crystals of the 1
:
1 complex were grown by slow vapor diffusion of iPr2O into an MeCN solution containing equimolar amounts of CBPQT·4PF6 and BHEAN. The green single crystals were suitable for X-ray crystallographic analysis. The solid-state superstructure,25 which is illustrated in Fig. 2, represents a familiar geometrical relationship between the guest and the host, wherein [π⋯π] stacking26 is aided and abetted by [C–H⋯O]27 and [C–H⋯π]28 interactions. This result lends yet more credence to the choice of BHEAN as a “template-exchanging” reagent. The packing of the 1
:
1 complexes is discussed in the ESI†. The template-directed synthesis and subsequent purification of CBPQT4+ is summarised in Scheme 1. The 1H NMR spectrum (Fig. 1b) of the tetracationic cyclophane, obtained after removal of the BHEAN by protonation, reveals the presence of trace amounts of side products. These impurities can be removed, either by flash chromatography or by crystallisation, following vapour diffusion of Et2O into an MeCN solution of the crude CBPQT·4PF6.
![The wireframe representation of [BHEAN⊂CBPQT]·4PF6 solid-state superstructure. The PF6− counterions, solvent molecules, and H atoms have all been removed for clarity. Color code: CBPQT4+, blue; BHEAN, pink.](/image/article/2010/SC/c0sc00176g/c0sc00176g-f2.gif) |
| Fig. 2 The wireframe representation of [BHEAN⊂CBPQT]·4PF6 solid-state superstructure. The PF6− counterions, solvent molecules, and H atoms have all been removed for clarity. Color code: CBPQT4+, blue; BHEAN, pink. | |
In our pursuit of employing crystallisation methods to affect the purification of the tetracationic cyclophane, we found that slow vapour diffusion of iPr2O into a solution of CBPQT·4PF6 in MeCN leads to the formation of two different crystalline forms—one block-like and the other needle-like—which can be hand-picked from the mother liquor. The first one was shown to correspond with the chimney-like superstructure (Fig. 3) reported1 back in 1988. X-Ray crystallography revealed that the needle-like crystals have a different monoclinic unit cell,29 and a superstructure (Fig. 4), reminiscent of a “chessboard.” In the second polymorph of CBPQT·4PF6, there is a 40° twist angle relating the mean planes of the two pyridinium rings in the bipyridinium units. The two para-phenylene rings interact with the pyridinium rings in adjacent cyclophane molecules through π–π stacking.30 In common with the chimney-like superstructure, the CBPQT4+ rings are stacked directly on top of each other in adjacent unit cells. The absence of solvent molecules and the difference in the alignment of the CBPQT4+ rings and the companion PF6− counterions result in a more densely packed crystal.
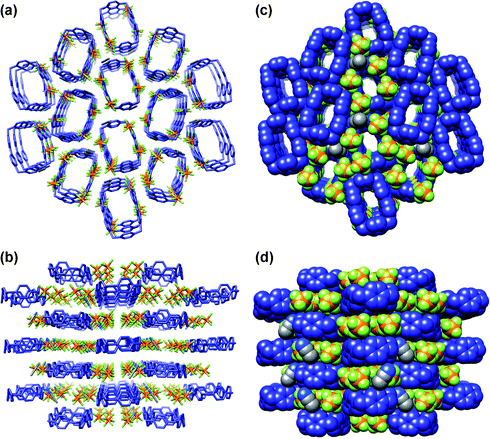 |
| Fig. 3 The “chimney” CBPQT·4PF6 solid-state gross structure. (a) Wireframe representation, viewed down the crystallographic a axis, (b) wireframe representation, viewed down the crystallographic c axis, (c) space-filling representation, viewed down the crystallographic a axis, (d) space-filling representation, viewed down the crystallographic c axis. Colour code: CBPQT4+, blue; MeCN and PF6− are coloured by element. C, gray; N, blue; P, orange, F, yellow. All H atoms have been omitted for clarity. | |
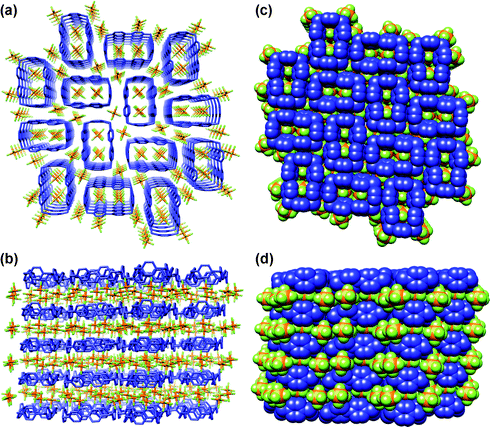 |
| Fig. 4 The “chessboard” CBPQT·4PF6 solid-state gross structure. (a) Wireframe representation, viewed down the crystallographic a axis, (b) wireframe representation, viewed down the crystallographic b + c axis, (c) space-filling representation, viewed down the crystallographic a axis, (d) space-filling representation, viewed down the crystallographic b + c axis. Color code: CBPQT4+, blue; PF6− are coloured by element. P, orange; F, yellow. All H atoms have been omitted for clarity. | |
Conclusion
We have watched the rise in the popularity of cyclobis(paraquat-p-phenylene) to the point where it is now commercially available, but at an exorbitant price. The time is therefore ripe to introduce a much more efficient means of extracting the 1,5-dioxynaphthalene template from its cavity. The use of a 1,5-diaminonaphthalene derivative as a surrogate achieves this objective in a simple and straightforward manner that is commensurate with a process that can be performed on a bulk scale. Furthermore, we have followed the evolution of the cyclophane from its early days till now, with respect to its reaction times and yields that have accompanied its synthesis. This information is presented in Table 3 along with the particular reaction conditions employed. We are confident that the latest procedure described herein that works for one tetracationic cyclophane would no doubt work for others.31
Table 3 The evolution of CBPQT·4PF6 production over a quarter of a century
Year |
Protocola |
Templateb |
Solventc |
Yieldd (%) |
Timee/h |
TDS = template-directed synthesis; LLE = liquid–liquid extraction; UHP = ultra-high pressure (15 kbar); “one step” implies the use of 4,4′-bipyridine as a starting material instead of 1,1′-[1,4-phenylenebis(methylene)]bis(4,4′-bipyridinium) bis(hexafluorophosphate).
BHEEB = 1,4-bis[2-(2-hydroxyethoxy)ethoxy]benzene; BHEEEB = 1,4-bis[2-[2-(2-hydroxyethoxy)ethoxy]ethoxy]benzene; BHEEN = 1,5-bis[2-(2-hydroxyethoxy)ethoxy]naphthalene; BMEEN = 1,5-bis[2-(2-methoxyethoxy)ethoxy]naphthalene.
Under the same reaction conditions, the use of DMF instead of MeCN, results in higher yields.
The yields were obtained under different reaction conditions, which are not strictly comparable. In general, the use of TDS and UHP results in higher yields.
The reaction times reported in the literature range from 2–9 days (under atmospheric pressure) or 1–3 days under UHP. LLE times range from 2–5 days, but, in practice, the procedure can take more than one week.
ref. 1.
ref. 2a.
ref. 2b.
ref. 2c.
ref. 2d.
Template-trading is a purification method we have developed in order to remove the template employed in the reaction. The procedure does not affect the yield, which is determined by the initial reaction conditions. The reason for obtaining a 42% yield—lower than the 81% reported (ref. 2d) in 1996—is because we employed a much more (3.5×) concentrated reaction mixture. Yields comparable to the method reported in 1996 can be obtained by employing more dilute reaction conditions.
|
1988f |
No TDS |
N/A |
MeCN |
12 |
48 |
1991g,h |
TDS/LLE |
BHEEB |
MeCN |
36 |
336 |
1991g,h |
TDS/LLE |
BHEEB |
DMF |
45 |
240 |
1991g,h |
TDS/UHP/LLE |
BHEEB |
DMF |
62 |
144 |
1992h |
TDS/LLE |
BHEEEB |
MeCN |
23 |
336 |
1992i |
One step/TDS/LLE |
BHEEN |
DMF |
23 |
288 |
1992i |
One step/TDS/UHP/LLE |
BHEEN |
DMF |
5 |
144 |
1996j |
TDS/LLE |
BMEEN |
DMF |
62 |
168 |
1996j |
TDS/UHP/LLE |
BMEEN |
DMF |
81 |
120 |
2010 |
TDS/UHP/Template-tradingk |
BHEEN |
DMF |
42 |
72 |
Acknowledgements
The research described in this edge article was sponsored by the National Center for Nano Technology Research at the King Abdulaziz City for Science and Technology (KACST) in Saudi Arabia. The authors thank Dr Turki M. Al-Saud and Dr Soliman H. Alkhowaiter at KACST for their generous support of this programme of research at Northwestern University. We also thank Dr Amy Sarjeant, Ms Charlotte C. Stern, and the personnel in the Integrated Molecular Structure Education and Research Center (IMSERC) at Northwestern University for their assistance in the collection of X-ray crystallographic data.
Notes and references
- B. Odell, M. V. Reddington, A. M. Z. Slawin, N. Spencer, J. F. Stoddart and D. J. Williams, Angew. Chem., Int. Ed. Engl., 1988, 27, 1547–1550 CrossRef.
-
(a) C. L. Brown, D. Philp and J. F. Stoddart, Synlett, 1991, 462–464 CrossRef CAS;
(b) P.-L. Anelli, P. R. Ashton, R. Ballardini, V. Balzani, M. Delgado, M. T. Gandolfi, T. T. Goodnow, A. E. Kaifer, D. Philp, M. Pietraszkiewicz, L. Prodi, M. V. Reddington, A. M. Z. Slawin, N. Spencer, J. F. Stoddart, C. Vicent and D. J. Williams, J. Am. Chem. Soc., 1992, 114, 193–218 CrossRef CAS;
(c) C. L. Brown, D. Philp, N. Spencer and J. F. Stoddart, Isr. J. Chem., 1992, 32, 61–67 CAS;
(d) M. Asakawa, W. Dehaen, G. L'abbé, S. Menzer, J. Nouwen, F. M. Raymo, J. F. Stoddart and D. J. Williams, J. Org. Chem., 1996, 61, 9591–9595 CrossRef CAS.
-
(a)
J.-M. Lehn, Supramolecular Chemistry, VCH, Weinheim, 1995 Search PubMed;
(b) J. S. Bradshaw and R. M. Izatt, Acc. Chem. Res., 1997, 30, 338–345 CrossRef CAS;
(c) J. F. Stoddart, Nat. Chem., 2009, 1, 14–15 Search PubMed.
- J. F. Stoddart and H. M. Colquhoun, Tetrahedron, 2008, 64, 8231–8263 CrossRef CAS.
- Reference has been made to the “blue box”, not only in the primary chemical literature ( M. B. Nielsen, Z.-T. Li and J. Becher, J. Mater. Chem., 1997, 7, 1175–1187 Search PubMed ), but also in a textbook. See:
J. W. Steed and J. L. Atwood, Supramolecular Chemistry, John Wiley and Sons, New York, 2009, p. 357 RSC.
-
(a) P. R. Ashton, B. Odell, M. V. Reddington, A. M. Z. Slawin, J. F. Stoddart and D. J. Williams, Angew. Chem., Int. Ed. Engl., 1988, 27, 1550–1553 CrossRef;
(b) P. R. Ashton, D. Philp, N. Spencer and J. F. Stoddart, J. Chem. Soc., Chem. Commun., 1991, 1677–1679 RSC;
(c) M. Asakawa, S. Iqbal, J. F. Stoddart and N. D. Tinker, Angew. Chem., Int. Ed. Engl., 1996, 35, 976–978 CrossRef CAS.
-
(a) M. V. Reddington, A. M. Z. Slawin, N. Spencer, J. F. Stoddart, C. Vicent and D. J. Williams, J. Chem. Soc., Chem. Commun., 1991, 630–634 RSC;
(b) R. Ballardini, V. Balzani, M. T. Gandolfi, L. Prodi, M. Venturi, D. Philp, H. G. Ricketts and J. F. Stoddart, Angew. Chem., Int. Ed. Engl., 1993, 32, 1301–1303 CrossRef;
(c) P. R. Ashton, D. Philp, N. Spencer, J. F. Stoddart and D. J. Williams, J. Chem. Soc., Chem. Commun., 1994, 181–184 RSC;
(d) M. Asakawa, P. R. Ashton, S. E. Boyd, C. L. Brown, R. E. Gillard, O. Kocian, F. M. Raymo, J. F. Stoddart, M. S. Tolley, A. J. P. White and D. J. Williams, J. Org. Chem., 1997, 62, 26–37 CrossRef CAS;
(e) S. Kang, S. A. Vignon, H.-R. Tseng and J. F. Stoddart, Chem.–Eur. J., 2004, 10, 2555–2564 CrossRef CAS.
-
(a) D. Philp, A. M. Z. Slawin, N. Spencer, J. F. Stoddart and D. J. Williams, J. Chem. Soc., Chem. Commun., 1991, 1584–1586 RSC;
(b) P.-L. Anelli, M. Asakawa, P. R. Ashton, R. A. Bissell, G. Clavier, R. Górski, A. E. Kaifer, S. J. Langford, G. Mattersteig, S. Menzer, D. Philp, A. M. Z. Slawin, N. Spencer, J. F. Stoddart, M. S. Tolley and D. J. Williams, Chem.–Eur. J., 1997, 3, 1113–1135 CrossRef CAS;
(c) M. Asakawa, P. R. Ashton, V. Balzani, A. Credi, C. Hamers, G. Mattersteig, M. Montalti, A. N. Shipway, N. Spencer, J. F. Stoddart, M. S. Tolley, M. Venturi, A. J. P. White and D. J. Williams, Angew. Chem., Int. Ed., 1998, 37, 333–337 CrossRef CAS;
(d) M. B. Nielsen, J. O. Jeppesen, J. Lau, C. Lomholt, D. Damgaard, J. P. Jacobsen, J. Becher and J. F. Stoddart, J. Org. Chem., 2001, 66, 3559–3563 CrossRef CAS;
(e) I. Aprahamian, J.-C. Olsen, A. Trabolsi and J. F. Stoddart, Chem.–Eur. J., 2008, 14, 3889–3895 CrossRef CAS.
- T. T. Goodnow, M. V. Reddington, J. F. Stoddart and A. E. Kaifer, J. Am. Chem. Soc., 1991, 113, 4335–4337 CrossRef CAS.
-
(a) D. J. McCord, J. H. Small, J. Greaves, Q. N. Van, A. J. Shaka, E. B. Fleischer and K. J. Shea, J. Am. Chem. Soc., 1998, 120, 9763–9770 CrossRef CAS;
(b) M. Alvaro, B. Ferrer, V. Fornés, H. Garcia and J. C. Scaiano, J. Phys. Chem. B, 2002, 106, 6815–6820 CrossRef CAS;
(c) W.-H. Huang, S. Liu, P. Y. Zavalij and L. Isaacs, J. Am. Chem. Soc., 2006, 128, 14744–14745 CrossRef CAS;
(d) J. Jiang and M. J. MacLachlan, Chem. Commun., 2009, 5695–5697 RSC.
-
(a) A. Trabolsi, N. Khashab, A. C. Fahrenbach, D. C. Friedman, M. T. Colvin, K. K. Cotí, D. Benítez, E. Tkatchouk, J.-C. Olsen, M. E. Belowich, R. Carmielli, H. A. Khatib, W. A. Goddard III, M. R. Wasielewski and J. F. Stoddart, Nat. Chem., 2010, 2, 42–49 Search PubMed;
(b) A. Trabolsi, A. C. Fahrenbach, S. K. Dey, A. I. Share, D. C. Friedman, S. Basu, T. B. Gasa, N. M. Khashab, S. Saha, I. Aprahamian, H. A. Khatib, A. H. Flood and J. F. Stoddart, Chem. Commun., 2010, 46, 871–873 RSC.
-
(a) E. M. Kosower and J. Hajdu, J. Am. Chem. Soc., 1971, 93, 2534–2535 CrossRef CAS;
(b) W. Geuder, S. Hünig and A. Suchy, Tetrahedron, 1986, 42, 1665–1677 CrossRef.
-
(a) D. J. Cram, Science, 1983, 219, 1177–1183 CrossRef CAS;
(b) D. J. Cram, Angew. Chem., Int. Ed. Engl., 1988, 27, 1009–1020 CrossRef.
-
(a) D. H. Busch and N. A. Stephenson, Coord. Chem. Rev., 1990, 100, 119–154 CrossRef CAS;
(b) S. Anderson, H. L. Anderson and J. K. M. Sanders, Acc. Chem. Res., 1993, 26, 469–475 CrossRef CAS;
(c)
Templated Organic Synthesis, ed. F. Diederich and P. J. Stang, Wiley-VCH, Weinheim, 1999 Search PubMed;
(d) J. F. Stoddart and H.-R. Tseng, Proc. Natl. Acad. Sci. U. S. A., 2002, 99, 4797–4800 CrossRef CAS;
(e) M. J. Blanco, J. C. Chambron, M. C. Jiménez and J.-P. Sauvage, Top. Stereochem., 2003, 23, 125–173 Search PubMed;
(f) D. H. Busch, Top. Curr. Chem., 2005, 249, 1–65 CAS;
(g) K. E. Griffiths and J. F. Stoddart, Pure Appl. Chem., 2008, 80, 485–506 CrossRef CAS.
-
(a) D. B. Amabilino and J. F. Stoddart, Chem. Rev., 1995, 95, 2725–2828 CrossRef CAS;
(b) M. C. T. Fyfe and J. F. Stoddart, Acc. Chem. Res., 1997, 30, 393–401 CrossRef CAS;
(c)
J.-P. Sauvage and C. Dietrich-Buchecker, Molecular Catenanes, Rotaxanes and Knots: A Journey Through the World of Molecular Topology, John Wiley and Sons, New York, 1999 Search PubMed;
(d) J. F. Stoddart, Chem. Soc. Rev., 2009, 38, 1802–1820 RSC.
-
(a)
G. Schill, Catenanes, Rotaxanes and Knots, Academic Press, New York, 1971 Search PubMed;
(b) P. R. Ashton, T. T. Goodnow, A. E. Kaifer, M. V. Reddington, A. M. Z. Slawin, N. Spencer, J. F. Stoddart, C. Vicent and D. J. Williams, Angew. Chem., Int. Ed. Engl., 1989, 28, 1396–1399 CrossRef;
(c) C. A. Hunter, J. Am. Chem. Soc., 1992, 114, 5303–5311 CrossRef CAS;
(d) C. O. Dietrich-Buchecker, B. Frommberger, I. Lüer, J.-P. Sauvage and F. Vögtle, Angew. Chem., Int. Ed. Engl., 1993, 32, 1434–1437 CrossRef;
(e) D. B. Amabilino, P. R. Ashton, A. S. Reder, N. Spencer and J. F. Stoddart, Angew. Chem., Int. Ed. Engl., 1994, 33, 433–437 CrossRef;
(f) T. J. Kidd, D. A. Leigh and A. J. Wilson, J. Am. Chem. Soc., 1999, 121, 1599–1600 CrossRef CAS;
(g) D. L. Simone and T. M. Swager, J. Am. Chem. Soc., 2000, 122, 9300–9301 CrossRef CAS;
(h) W.-Q. Deng, A. H. Flood, J. F. Stoddart and W. A. Goddard III, J. Am. Chem. Soc., 2005, 127, 15994–15995 CrossRef CAS;
(i) O. Š. Miljanić and J. F. Stoddart, Proc. Natl. Acad. Sci. U. S. A., 2007, 104, 12966–12970 CrossRef CAS;
(j) K. Patel, O. Š, Miljanić and J. F. Stoddart, Chem. Commun., 2008, 1853–1855 RSC;
(k) M. A. Olson, A. B. Braunschweig, L. Fang, T. Ikeda, R. Klajn, A. Trabolsi, C. A. Mirkin, B. A. Grzybowski and J. F. Stoddart, Angew. Chem., Int. Ed., 2009, 48, 1792–1797 CrossRef CAS;
(l) Y.-L. Zhao, L. Liu, W. Zhang, C.-H. Sue, Q. Li, O. Š. Miljanić, O. M. Yaghi and J. F. Stoddart, Chem.–Eur. J., 2009, 13, 13356–13380;
(m) Q. Li, W. Zhang, O. Š. Miljanić, C. B. Knobler, J. F. Stoddart and O. M. Yaghi, Chem. Commun., 2010, 46, 380–382 RSC.
-
(a) P.-L. Anelli, N. Spencer and J. F. Stoddart, J. Am. Chem. Soc., 1991, 113, 5131–5133 CrossRef;
(b) R. A. Bissell, E. Cordova, A. E. Kaifer and J. F. Stoddart, Nature, 1994, 369, 133–137 CrossRef;
(c) P. R. Ashton, R. Ballardini, V. Balzani, M. Bělohradský, M. T. Gandolfi, D. Philp, L. Prodi, F. M. Raymo, M. V. Reddington, N. Spencer, J. F. Stoddart, M. Venturi and D. J. Williams, J. Am. Chem. Soc., 1996, 118, 4931–4951 CrossRef CAS;
(d) N. Solladie, J.-C. Chambron, C. O. Dietrich-Buchecker and J.-P. Sauvage, Angew. Chem., Int. Ed. Engl., 1996, 35, 906–909 CrossRef CAS;
(e) D. B. Amabilino, M. Asakawa, P. R. Ashton, R. Ballardini, V. Balzani, M. Bělohradský, A. Credi, M. Higuchi, F. M. Raymo, T. Shimizu, J. F. Stoddart, M. Venturi and K. Yase, New J. Chem., 1998, 22, 959–972 RSC;
(f) F. Aricó, T. Chang, S. J. Cantrill, S. I. Khan and J. F. Stoddart, Chem.–Eur. J., 2005, 11, 4655–4666 CrossRef;
(g) W. R. Dichtel, O. Š. Miljanić, J. M. Spruell, J. R. Heath and J. F. Stoddart, J. Am. Chem. Soc., 2006, 128, 10388–10390 CrossRef CAS;
(h) J. M. Spruell, W. R. Dichtel, J. R. Heath and J. F. Stoddart, Chem.–Eur. J., 2008, 14, 4168–4177 CrossRef CAS;
(i) I. Yoon, O. Š. Miljanić, D. Benítez, S. I. Khan and J. F. Stoddart, Chem. Commun., 2008, 4561–563 RSC;
(j) H. Kim, W. A. Goddard III, S. S. Jang, W. R. Dichtel, J. R. Heath and J. F. Stoddart, J. Phys. Chem. A, 2009, 113, 2136–2143 CrossRef CAS;
(k) T. Ikeda, M. Higuchi and D. G. Kurth, J. Am. Chem. Soc., 2009, 131, 9158–9159 CrossRef CAS.
-
(a) R. A. van Delden, M. K. J. ter Wiel, M. M. Pollard, J. Vivario, N. Koumura and B. L. Feringa, Nature, 2005, 437, 1337–1340 CrossRef CAS;
(b) V. Serreli, C. F. Lee, E. R. Kay and D. A. Leigh, Nature, 2007, 445, 523–527 CrossRef CAS;
(c)
B. H. Northrop, A. B. Braunschweig, P. M. Mendes, W. R. Dichtel and J. F. Stoddart, Handbook of Nanoscience Engineering and Technology, ed. W. A. Goddard III, D. W. Brenner, S. E. Lyshevski and G. J. Iafrate, CRC Press, Boca Raton, 2007, 2nd edn, vol. 11, pp. 1–48 Search PubMed;
(d) E. R. Kay, D. A. Leigh and F. Zerbetto, Angew. Chem., Int. Ed., 2007, 46, 72–191 CrossRef CAS;
(e) J. M. Spruell, W. F. Paxton, J.-C. Olsen, D. Benítez, E. Tkatchouk, C. L. Stern, A. Trabolsi, D. C. Friedman, W. A. Goddard III and J. F. Stoddart, J. Am. Chem. Soc., 2009, 131, 11571–11580 CrossRef CAS;
(f) Y. B. Zheng, Y.-W. Yang, L. Jensen, L. Fang, B. K. Juluri, A. H. Flood, P. S. Weiss, J. F. Stoddart and T. J. Huang, Nano Lett., 2009, 9, 819–825 CrossRef CAS;
(g) R. Klajn, L. Fang, A. Coskun, M. A. Olson, P. J. Wesson, J. F. Stoddart and B. A. Grzybowski, J. Am. Chem. Soc., 2009, 131, 4233–4235 CrossRef CAS.
-
(a) C. P. Collier, G. Mattersteig, E. W. Wong, Y. Luo, K. Beverly, J. Sampaio, F. M. Raymo, J. F. Stoddart and J. R. Heath, Science, 2000, 289, 1172–1175 CrossRef CAS;
(b) M. Feng, L. Gao, Z. Deng, W. Ji, X. Guo, S. Du, D. Shi, D. Zhang, D. Zhu and H. Gao, J. Am. Chem. Soc., 2007, 129, 2204–2205 CrossRef CAS;
(c) J. E. Green, J. W. Choi, A. Boukai, Y. Bunimovich, E. Johnston-Halprin, E. DeIonno, Y. Luo, B. A. Sheriff, K. Xu, Y. S. Shin, H.-R. Tseng, J. F. Stoddart and J. R. Heath, Nature, 2007, 445, 414–417 CrossRef CAS;
(d) W. R. Dichtel, J. R. Heath and J. F. Stoddart, Philos. Trans. R. Soc. London, Ser. A, 2007, 365, 1607–1625 CrossRef CAS.
-
(a) Y. Liu, A. H. Flood, P. A. Bonvallet, S. A. Vignon, B. Northrop, H.-R. Tseng, J. O. Jeppesen, T. J. Huang, B. Brough, M. Baller, S. Magonov, S. Solares, W. A. Goddard III, C.-M. Ho and J. F. Stoddart, J. Am. Chem. Soc., 2005, 127, 9745–9759 CrossRef CAS;
(b) B. Brough, B. H. Northrop, J. J. Schmidt, H.-R. Tseng, K. N. Houk, J. F. Stoddart and C.-M. Ho, Proc. Natl. Acad. Sci. U. S. A., 2006, 103, 8583–8588 CrossRef CAS.
-
(a) A. N. Shipway, M. Lahav, R. Blonder and I. Willner, Chem. Mater., 1999, 11, 13–15 CrossRef CAS;
(b) A. B. Kharitonov, A. N. Shipway and I. Willner, Anal. Chem., 1999, 71, 5441–5443 CrossRef CAS;
(c) L. Sheeney-Haj-Ichia and I. Willner, J. Phys. Chem. B, 2002, 106, 13094–13097 CrossRef CAS;
(d) E. Katz, O. Lioubashevsky and I. Willner, J. Am. Chem. Soc., 2004, 126, 15520–15532 CAS;
(e) E. Katz, R. Baron, I. Willner, N. Richke and R. D. Levine, ChemPhysChem, 2005, 6, 2179–2189 CrossRef CAS.
-
(a) A. N. Shipway, M. Lahav, R. Gabai and I. Willner, Langmuir, 2000, 16, 8789–8795 CrossRef CAS;
(b) M. A. Olson, A. Coskun, R. Klajn, L. Fang, S. K. Dey, K. P. Browne, B. A. Grzybowski and J. F. Stoddart, Nano Lett., 2009, 9, 3185–3190 CrossRef CAS;
(c) R. Klajn, M. A. Olson, P. J. Wesson, L. Fang, A. Coskun, A. Trabolsi, J. F. Stoddart and B. A. Grzybowski, Nat. Chem., 2009, 1, 733–738 Search PubMed.
- Using BHEAN directly as the template for cyclisation was also investigated. The reaction resulted in a low yield (<10%), whether carried out under UHP or atmospheric pressure. The reason is presumably because of the nucleophilic nature of the amino groups present in BHEAN.
- J. W. Choi, A. H. Flood, D. W. Steuerman, S. Nygaard, A. B. Braunschweig, N. N. P. Moonen, B. W. Laursen, Y. Luo, E. Delonno, A. J. Peters, J. O. Jeppesen, K. Xe, J. F. Stoddart and J. R. Heath, Chem.–Eur. J., 2006, 12, 261–279 CrossRef CAS.
- Crystal data for [BHEAN⊂CBPQT]·4PF6·3MeCN: C18H26N2O4⊂C36H32N4·4PF6·6C2H3N, M = 1681.27, monoclinic, a = 13.845(1), b = 22.974(3), c = 13.206(1) Å, β = 116.740(7)°, U = 3751.7(7) Å3, T = 100(2) K, space group P21/c, Z = 2 (BHEAN⊂CBPQT4+ lies on an inversion center), 6425 independent reflections, ρ = 1.488 g cm−3, μ(Cuκα) = 1.96 mm−1, 6425 independent observed reflections, 5276 reflections with I > 2σ(I), Rint = 0.032, R[F2 > 2σ(F2)] = 0.050, wR(F2) = 0.137. CCDC 764119.
- The mean interplanar separation between the 1,5-diaminonaphthalene residue and the bipyridinum unit is 3.24 Å.
- The [C–H⋯O] interactions happen between the Ha protons on the bipyridinium unit and the middle oxygen atom of the polyether chains of the naphthalene-based unit. The [C–H⋯π] hydrogen bonding geometries are [C⋯O], [H⋯O] distances, [C–H⋯O] angles: 3.39 Å, 2.61 Å, and 139.6°.
- The [H⋯π] distances associated with the [C–H⋯π] interactions are 2.55 Å and the associated [C–H⋯π] angles are 147°.
- Crystal data for CBPQT·4PF6: C36H32N4·4PF6, M = 1100.54, monoclinic, a = 7.316(1), b = 13.756(1), c = 20.634(2) Å, β = 97.195(8)°, U = 2060.4(4) Å3, T = 100(2) K, space group P21/c, Z = 2 (CBPQT4+ lies on an inversion center), ρ = 1.774 g cm−3, μ(Cuκα) = 3.05 mm−1, 3430 independent observed reflections, 2180 reflections with I > 2σ(I), Rint = 0.099, R[F2 > 2σ(F2)] = 0.068, wR(F2) = 0.197. CCDC 766073.
- The π–π distance between the para-phenylene units and adjacent pyridinium rings is 3.71 Å.
- In addition to CBQPT·4PF6, we have explored the purification of various other tetracationic cyclophanes and functionalised “blue boxes” in our laboratories. The method described in this manuscript is universally applicable to all those compounds.
|
This journal is © The Royal Society of Chemistry 2010 |
Click here to see how this site uses Cookies. View our privacy policy here.