DOI:
10.1039/C0OB00693A
(Communication)
Org. Biomol. Chem., 2011,
9, 66-69
Water-solubilisation and bio-conjugation of a red-emitting BODIPY marker†
Received
7th September 2010
, Accepted 29th October 2010
First published on 18th November 2010
Abstract
The synthesis and preliminary bio-conjugation studies of a novel water-soluble red-emitting di-styryl BODIPY dye are disclosed. Aggregation behaviour of this compound under physiological conditions was suppressed by specific introduction of a di-sulfonated peptide-based linker at the mesophenyl substituent, sultonated styryl arms and short polyethyleneglycol chains at the boron center. Thus, a good quantum yield of 22% in PBS for this red-emitting BODIPY was obtained. Introduction of an activated ester function enabled successful bio-conjugation to monoclonal antibodies and proteins.
Introduction
During the past decade, intensive research efforts have been devoted to the design and synthesis of water-soluble long-wavelength fluorescent organic dyes for use as non-radioactive labels in various bio-analytical, life science and medical applications.1–3 The advantages of a fluorescence occurring in the low-energy region of the electromagnetic spectrum are numerous and have been extensively discussed.1,4 Amongst these advantages is the absence of background absorption, autofluorescence (especially of cell components), and light scattering along with the availability of low-cost sources of irradiation. Several suitable dyes are available but the BODIPY, cyanine and rhodamine families are the most promising owing to their attractive photophysical properties, and their facile chemical transformation.3 However, their prohibitive cost and limited stability have hampered their wide use as markers and photosensitisers.
We and others have noted that long-wavelength BODIPY dyes are often more photo- and chemically stable than cyanine dyes.5 Their preparation by extending the π-conjugation of the central core through Knoevenagel condensation reaction6,7 or Pd-catalysed cross-coupling reactions8 involving the 3,5-pyrrolic positions were more convenient and less time-consuming than the linear synthetic routes proposed for the preparation of rigidified π-extended rhodamine dyes.9 However, unlike to cyanine and rhodamine dyes, only a limited number of water-soluble and bio-conjugatable BODIPY derivatives are now available.10,11 In 2008, Atilgan et al. reported the synthesis of the first water-soluble NIR fluorescent BODIPY dye by tethering short PEG-type linkers onto the meso-phenyl and styryl moieties.12 Further functionalisation with a dipicolylamine unit enabled them to design a sensitive and selective ratiometric chemosensor for Zn(II) ions in ethanol/aqueous buffer. However, the lack of an additional functional group for bio-conjugation prevents its use as a bio-labelling reagent. Recently, anionic and cationic substituted BF2-chelated tetraarylazadipyrromethene derivatives (aza-BODIPY), bearing sulfonic acid, carboxylic acid or quaternary amine moieties have been synthesised by Tasior et al.13 However, the location of two water-solubilising groups on the same side (top or bottom) of the aza-BODIPY scaffold led to amphiphilic-like structures which are fluorescent only in aqueous solutions containing additives that disrupt aggregates. Such aggregation behaviour in water was also observed recently with blue di-styryl BODIPY dyes bearing a tri-sulfonated tail linked in the meso-phenyl substituent.14 To overcome this drawback and to provide highly fluorescent dyes soluble in water and emitting above 600 nm, we have thought to combine two complementary water-solubilisation methods allowing the introduction of (poly)sulfonated linkers in two opposite orientations on amino-alkyne-functionalised BODIPY cores (E-BODIPY) through amidification of the meso-aryl carboxylic acid14 and alkylation of N,N-dimethylpropargylamine moieties with 1,3-propanesultone15 respectively. Herein, we succeed in the application of this original double-sulfonation procedure to the preparation of a novel water-soluble di-styryl BODIPY dye, which exhibits attractive photophysical properties in aqueous media. Furthermore, its potential for effective amine conjugations was illustrated with antibodies and proteins.
Results and discussion
The preparation of the target red emitter 8 required the two starting materials 2 and 316 (Scheme 1). The key step involved a Knoevenagel reaction in a mixture of toluene and piperidine leading to the blue derivative 4 in 22% yield after careful column chromatography. The mono-substituted side-product (magenta dye) could not be properly purified due to contamination of the aldehyde derivative 2. The unreacted starting material 3 is conveniently recovered during the purification process and recycled (20%). The next step involved the Grignard reagent EtMgC
CCH2OCH2CH2OMe allowing substitution of the fluorine atoms, leading to derivative 5 in 70% isolated yield.17 Use of a carboalkoxylation reaction promoted by low-valent palladium provided quantitatively the ethyl ester 6. The next step involved quaternisation of the tertiary amines with an excess of 1,3-propanesultone in 1,2-dichloroethane as solvent. The resulting solid was recrystallised in adequate solvents to provide the bis-sulfobetain 7 in 60%. Finally, saponification of the ethyl ester was performed under standard conditions to give the acid 8 as the main compound. Notice that the reverse order for the two-reaction sequence (i.e., saponification followed by sulfonation with 1,3-propanesultone) did not provide the expected derivative dye 8. All derivatives were unambiguously characterised by NMR, ESI-MS, UV-vis and steady-state emission and all data are in agreement with the assigned structures (see ESI†).
![Reagents and conditions: (i) 1-dimethylamino-2-propyne, benzene, triethylamine, [Pd(PPh3)4] (6 mol%), 60 °C, quant. yield; (ii) cmpds 2 (2.2 equiv.) and 3 (1 equiv.), toluene, piperidine, 140 °C, dryness, 22%; (iii) 3-(2-methoxyethoxy)-1-propyne, EtMgBr, anhydrous THF, 60 °C; then cmpd 4 in anhydrous THF, 60 °C, 70%; (iv) EtOH, TEA, CO flux 1 atm, 60 °C, [Pd(PPh3)2Cl2] (10 mol%), quant. yield; (v) 1,3-propanesultone (10 equiv.), C2H2Cl2, 60 °C, 60%; (vi) EtOH, NaOH (10 equiv.), rt, then pH 7.0 adjusted with 2% aq. HCl; (vii) TSTU (7.4 equiv.), DIEA (4.0 equiv.), NMP, rt, 1h; then (α-sulfo-β-alanine)2 (9.0 equiv.) in 0.24 M aq. NaHCO3, pH 8.2, 4 °C, overnight, 47%.](/image/article/2011/OB/c0ob00693a/c0ob00693a-s1.gif) |
| Scheme 1
Reagents and conditions: (i) 1-dimethylamino-2-propyne, benzene, triethylamine, [Pd(PPh3)4] (6 mol%), 60 °C, quant. yield; (ii) cmpds 2 (2.2 equiv.) and 3 (1 equiv.), toluene, piperidine, 140 °C, dryness, 22%; (iii) 3-(2-methoxyethoxy)-1-propyne, EtMgBr, anhydrous THF, 60 °C; then cmpd 4 in anhydrous THF, 60 °C, 70%; (iv) EtOH, TEA, CO flux 1 atm, 60 °C, [Pd(PPh3)2Cl2] (10 mol%), quant. yield; (v) 1,3-propanesultone (10 equiv.), C2H2Cl2, 60 °C, 60%; (vi) EtOH, NaOH (10 equiv.), rt, then pH 7.0 adjusted with 2% aq. HCl; (vii) TSTU (7.4 equiv.), DIEA (4.0 equiv.), NMP, rt, 1h; then (α-sulfo-β-alanine)2 (9.0 equiv.) in 0.24 M aq. NaHCO3, pH 8.2, 4 °C, overnight, 47%. | |
Synthesis of water-soluble BODIPY dye 9 through post-synthetic derivatisation with α-sulfo-β-alanine dipetide linker
The BODIPY carboxylic acid 8 was converted quantitatively into the corresponding N-hydroxysuccinimidyl (NHS) ester by treatment with peptide coupling uronium reagent TSTU and DIEA in dry NMP. Thereafter, the crude mixture of NHS ester was subjected to aminolysis with dipeptide (α-sulfo-β-alanine)2 in aqueous bicarbonate buffer to give the water-soluble BODIPY dye 9 (Scheme 1). RP-HPLC purification using aqueous triethylammonium bicarbonate (TEAB) buffer and acetonitrile as eluents, followed by desalting on ion-exchange resin Dowex H+ provided 9 in a pure form (isolated yield: 47%).
Photophysical properties of water-soluble BODIPY dye 9 in physiological conditions
As expected, sulfonated BODIPY dye 9 was found to be perfectly soluble in water and related aqueous buffers in the concentration range (1 μM to 10 mM) suitable for bio-labelling applications.18 The photophysical properties of this novel water-soluble long-wavelength fluorophore was evaluated under simulated physiological conditions (i.e., phosphate buffered saline (PBS), pH 7.5). Its spectral properties (Fig. 1, Table 1 and ESI†) in the red region correspond very closely to those previously reported for the class of di-styryl BODIPY chromophores in organic solvents.7 Furthermore, this fluorophore exhibits a strong fluorescence emission in physiological conditions with a 22% quantum yield. Interestingly, this value is comparable to those obtained for the most popular and widely used water-soluble red (or far-red) fluorescent labelling reagents belonging to the CyDye™ family and namely Cy 5.0 and Cy 5.5 (20% and 23% in PBS respectively).19,20 Further evidence for the lack of non-fluorescent aggregates of H-type, was provided by the excitation spectra of 9 in PBS which perfectly match the absorption spectra (see ESI†). This latter result and the good 22% quantum yield clearly demonstrate that the site-specific introduction of two different sets of sulfonated residues on each side of a di-styryl BODIPY core enables the generation of water-soluble and non-amphiphilic BODIPY derivatives.
Table 1 Spectral properties of the water-soluble BODIPY 9 and proteins labelled with this dye or Cy 5.0 in PBS, and fluorophore to protein molar ratios (F/P)a
Free or conjugated fluorescent dye |
λ
max, abs/nm |
ε/dm3 mol−1 cm−1 |
λ
max, em/nm |
Φ
F
b
|
F/P |
In the table the absorption signal arising from the protein, ∼280 nm in all cases, is omitted.
Determined at 25 °C by using sulfoindocyanine dye Cy 5.0 (ΦF = 20% in PBS at 25 °C) as a standard.19
|
9
|
368, 642 |
76 860, 55 080 |
655 |
0.22 |
— |
mAb-BODIPY
|
370, 603, 650 |
— |
657 |
0.07 |
5.66 |
mAb-Cy 5.0 |
610, 650 |
— |
667 |
0.12 |
2.75 |
BSA-BODIPY
|
372, 603, 651 |
— |
657 |
0.06 |
2.30 |
BSA-Cy 5.0 |
609, 651 |
— |
663 |
0.03 |
0.73 |
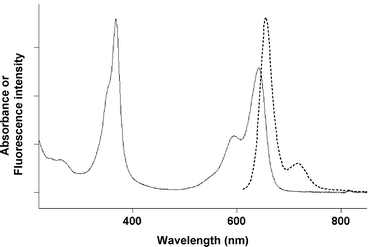 |
| Fig. 1 Spectral properties of BODIPY dye 9 in PBS at 25 °C. Normalised absorption (—) and emission ( ) spectra (Ex. 600 nm). | |
Since the water-soluble BODIPY dye 9 was functionalised with a free carboxylic acid group, its ability to label proteins and antibodies through reactions of its active ester with the NH2 groups of ε-lysine residues present in these biopolymers, was considered. BSA and the commonly used monoclonal antibody (mAb) 12CA5 that recognises the influenza hemagglutinin (HA) epitope tag,21 were chosen as the protein and the antibody respectively for the bio-conjugation experiments. To compare the labelling performances of this novel red-fluorescent marker with those of classic CyDye™ reagents, similar reactions were performed with sulfoindocyanine dye Cy 5.0 under the same experimental conditions. To avoid possible deleterious effects of organic solvents and additives towards mAb (or protein) structure and functional activity, a carboxylic acid activation protocol performed in water and involving the use of 1-ethyl-3-(3-dimethylaminopropyl) carbodiimide (EDC) and sulfo-NHS instead of TSTU/DIEA in a polar aprotic solvent was preferred. BSA and anti-HA mAb were labelled through overnight incubation with a 13- and 31-fold molar excess of BODIPY dye sulfo-NHS ester in PBS (pH 7.5) respectively. The resulting protein fluorescent conjugates were purified by size-exclusion chromatography over a Sephadex®G-25 column. Table 1 reports the absorption/emission wavelengths (see Fig. 2 and ESI† for the corresponding spectra), quantum yields of fluorescent proteins in PBS, together with the attached fluorophore to protein molar ratios (F/P), estimated from the relative intensities of protein and dye absorptions. The F/P values achieved with meso-derivatised BODIPY dye 9 were significantly higher than those achieved with Cy 5.0, indicating a greater reactivity of its sulfo-NHS ester. Indeed, the added (α-sulfo-β-alanine)-dipeptidyl spacer enables extension of the bioconjugatable CO2H group away from sterically hindered di-styryl BODIPY core, thus ensuring a better capability for the acylation of primary amines within proteins, especially under pH neutral conditions. When compared to the non-covalently bound poly-sulfonated BODIPY dye 9, the absorption maxima of the fluorescent protein conjugates is slightly red-shifted by about 8 nm whereas emission maxima remain unchanged (Fig. 2).
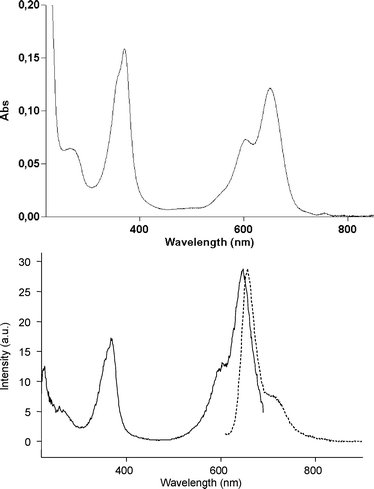 |
| Fig. 2 Top. Absorption spectrum of BSA-BODIPY conjugate in PBS at 25 °C. Bottom. Normalised emission ( ) (Ex. 600 nm) and excitation (—) (Em. 700 nm) spectra of BSA-BODIPY conjugate in PBS at 25 °C. | |
The drop in quantum yield observed after bio-conjugation is likely explained by dye to protein interactions favouring in certain cases electron transfer to aromatic amino acids or dye to dye interactions leading to non-emissive aggregates, in the same manner as observed for DNA biopolymers.22 We also notice that this binding-induced reduction in emission is also observed with protein-Cy 5.0 conjugates (it is even less pronounced for labelled anti-HA mAb) and has been already reported for other bioanalytical relevant fluorescent labels including Cy 5.0, Cy 5.5 and the Alexa Fluor®dyes.23 However, these preliminary results show for the first time that it is possible to get red-emitting fluorescent protein conjugates by using a water-soluble di-styryl BODIPY dye. Furthermore, the fluorescence emission of these bio-conjugates is observed in aqueous buffers without adding aggregate disrupting additives.
Conclusion and future work
In summary, a promising new class of water-soluble fluorescent labels based on a di-styryl E-BODIPY scaffold, has been developed. The use of sequential derivatisations of its N,N-dimethylpropargylamine linker arms and meso-phenyl carboxylic acid with 1,3-propanesultone and the dipeptide (α-sulfo-β-alanine)2 has led for the first time to a poly-sulfonated red-emitting BODIPY dye which does not aggregate in aqueous solutions. The successful post-synthetic sulfonation of highly-functionalised BODIPY cores clearly demonstrates the versatility of this easy-to-implement water-solubilising method. In this context, we believe that this procedure has a strong potential to enhance the water solubility of promising but highly hydrophobic fluorescent and/or redox active multi-component systems such as “cascatelle” dyes24 or through-bond energy transfer cassettes.25 As proteins labels, water-soluble BODIPY dyes, such as 9, are more or less equivalent to the commercial sulfoindocyanine dye Cy 5.0. However, to improve the brightness of the resulting BODIPY–protein conjugates, further sulfonation of our water-soluble derviatives in the 2- and 6-positions through the Boyer–Burgess method11,26 and/or the size increase of the BODIPY bio-conjugatable linker are currently under investigation.
Acknowledgements
This work was supported by the Agence Nationale de la Recherche (Programme Blanc 2009, ANR-09-BLAN-0081-01) especially for a PhD grant to Cédrik Massif, La Région Haute-Normandie via the CRUNCh program (CPER 2007-2013), and Institut Universitaire de France (IUF). We thank Dr Guillaume Clavé for the synthesis of sulfoindocyanine dye Cy 5.0, Dr Hervé Volland (iBiTecS, LERI, CEA-Saclay) for kind gift of anti-HA mAb clone 12CA5, and Professor Jack Harrowfield (CNRS-ISIS, Strasbourg) for commenting on the manuscript before publication.
Notes and references
- A. Gómez-Hens and M. P. Aguilar-Caballos, TrAC, Trends Anal. Chem., 2004, 23, 127 CrossRef.
-
(a)
R. P. Haugland, The Handbook. A Guide to Fluorescent Probes and Labeling Technologies, 11th edn, Invitrogen, Oregon, 2006 Search PubMed;
(b) C.-H. Tung, Biopolymers, 2004, 76, 391 CrossRef CAS.
- L. D. Lavis and R. T. Raines, ACS Chem. Biol., 2008, 3, 142 CrossRef CAS.
- V. Buschmann, K. D. Weston and M. Sauer, Bioconjugate Chem., 2003, 14, 195 CrossRef CAS.
-
(a) A. Loudet and K. Burgess, Chem. Rev., 2007, 107, 4891 CrossRef CAS;
(b) G. Ulrich, R. Ziessel and A. Harriman, Angew. Chem., Int. Ed., 2008, 47, 1184 CrossRef CAS.
- O. Buyukcakir, O. A. Bozdemir, S. Kolemen, S. Erbas and E. U. Akkaya, Org. Lett., 2009, 11, 4644 CrossRef CAS.
- P. Didier, G. Ulrich, Y. Mély and R. Ziessel, Org. Biomol. Chem., 2009, 7, 3639 RSC.
-
(a) L. Jiao, C. Yu, T. Uppal, M. Liu, Y. Li, Y. Zhou, E. Hao, X. Hu and M. G. H. Vicente, Org. Biomol. Chem., 2010, 8, 2517 RSC;
(b) V. Leen, E. Braeken, K. Luckermans, C. Jackers, M. Van Der Auweraer, N. Boens and W. Dehaen, Chem. Commun., 2009, 4515 RSC;
(c) T. Rohand, W. Qin, N. Boens and W. Dehaen, Eur. J. Org. Chem., 2006, 4658 CrossRef CAS.
- J. Liu, Z. Diwu, W.-Y. Leung, Y. Lu, B. Patch and R. P. Haugland, Tetrahedron Lett., 2003, 44, 4355 CrossRef CAS.
-
(a) M. Tasior and D. F. O'Shea, Bioconjugate Chem., 2010, 21, 1130 CrossRef CAS;
(b) M. Brellier, G. Duportail and R. Baati, Tetrahedron Lett., 2010, 51, 1269 CrossRef CAS;
(c) O. Dilek and S. L. Bane, Bioorg. Med. Chem. Lett., 2009, 19, 6911 CrossRef CAS;
(d) L. Jiao, J. Li, S. Zhang, C. Wei, E. Hao and M. G. H. Vicente, New J. Chem., 2009, 33, 1888 RSC;
(e) L. Li, B. Nguyen and K. Burgess, Bioorg. Med. Chem. Lett., 2008, 18, 3112 CrossRef CAS;
(f) C. Thivierge, R. Bandichhor and K. Burgess, Org. Lett., 2007, 9, 2135 CrossRef CAS;
(g) N. J. Meltola, R. Wahlroos and A. E. Soini, J. Fluoresc., 2004, 14, 635 CrossRef CAS.
- L. Li, J. Han, B. Nguyen and K. Burgess, J. Org. Chem., 2008, 73, 1963 CrossRef CAS.
- S. Atilgan, T. Ozdemir and E. U. Akkaya, Org. Lett., 2008, 10, 4065 CrossRef CAS.
- M. Tasior, J. Murtagh, D. O. Frimannsson, S. O. McDonnell and D. F. O'Shea, Org. Biomol. Chem., 2010, 8, 522 RSC.
- S. L. Niu, G. Ulrich, R. Ziessel, A. Kiss, P.-Y. Renard and A. Romieu, Org. Lett., 2009, 11, 2049 CrossRef CAS.
- S.-L. Niu, G. Ulrich, P. Retailleau, J. Harrowfield and R. Ziessel, Tetrahedron Lett., 2009, 50, 3840 CrossRef CAS.
- T. N. Singh-Rachford, A. Haefele, R. Ziessel and F. N. Castellano, J. Am. Chem. Soc., 2008, 130, 16164 CrossRef CAS.
- C. Goze, G. Ulrich and R. Ziessel, Org. Lett., 2006, 8, 4445 CrossRef CAS.
- M. S. T. Goncalves, Chem. Rev., 2009, 109, 190 CrossRef CAS.
- R. B. Mujumdar, L. A. Ernst, S. R. Mujumdar, C. J. Lewis and A. S. Waggoner, Bioconjugate Chem., 1993, 4, 105 CrossRef CAS.
- S. R. Mujumdar, R. B. Mujumdar, C. M. Grant and A. S. Waggoner, Bioconjugate Chem., 1996, 7, 356 CrossRef CAS.
-
(a) C. Chidley, K. Mosiewicz and K. Johnsson, Bioconjugate Chem., 2008, 19, 1753 CrossRef CAS;
(b) D. Maurel, J. Kniazeff, G. Mathis, E. Trinquet, J.-P. Pin and H. Ansanay, Anal. Biochem., 2004, 329, 253 CrossRef CAS.
- J. P. Rostron, G. Ulrich, P. Retailleau, A. Harriman and R. Ziessel, New J. Chem., 2005, 29, 1241 RSC.
- J. Pauli, R. Brehm, M. Spieles, W. A. Kaiser, I. Hilger and U. Resch-Genger, J. Fluoresc., 2010, 20, 681 CrossRef CAS.
-
(a) C. Goze, G. Ulrich and R. Ziessel, J. Org. Chem., 2007, 72, 313 CrossRef CAS;
(b) G. Ulrich, C. Goze, M. Guardigli, A. Roda and R. Ziessel, Angew. Chem., Int. Ed., 2005, 44, 3694 CrossRef CAS.
-
(a) W. Lin, L. Yuan, Z. Cao, Y. Feng and J. Song, Angew. Chem., Int. Ed., 2010, 49, 375 CAS;
(b) G. Barin, M. D. Yilmaz and E. U. Akkaya, Tetrahedron Lett., 2009, 50, 1738 CrossRef CAS;
(c) G.-S. Jiao, L. H. Thoresen, T. G. Kim, W. C. Haaland, F. Gao, M. R. Topp, R. M. Hochstrasser, M. L. Metzker and K. Burgess, Chem.–Eur. J., 2006, 12, 7816 CrossRef CAS.
- J. H. Boyer, A. M. Haag, G. Sathyamoorthi, M. L. Soong, K. Thangaraj and T. G. Pavlopoulos, Heteroat. Chem., 1993, 4, 39 CrossRef CAS.
Footnotes |
† Electronic supplementary information (ESI) available: Detailed synthetic procedures and characterisation data for compounds 2–9 and fluorescent bio-conjugates. See DOI: 10.1039/c0ob00693a |
‡ These authors contributed equally to this work. |
|
This journal is © The Royal Society of Chemistry 2011 |
Click here to see how this site uses Cookies. View our privacy policy here.