DOI:
10.1039/C1PY00271F
(Paper)
Polym. Chem., 2011,
2, 2518-2527
A click chemistry-based “grafting through” approach to the synthesis of a biorelevant polymer brush
Received
14th June 2011
, Accepted 18th July 2011
First published on 19th August 2011
Abstract
A new biorelevant polymer brush showing a polybenzofulvene backbone was synthesized by a “grafting through” approach based on click chemistry and spontaneous polymerization reactions. The easy polymerization of the relatively complex monomer (6-MOEG-9-TM-BF3k) suggests the existence of a particularly efficient recognition process capable of pre-organizing the monomer molecules for the spontaneous polymerization. 13C-NMR spectroscopy as well as UV-vis and fluorescence spectroscopy suggested for poly-6-MOEG-9-TM-BF3k the features of a vinyl (1,2) π-stacked polymer. The new polybenzofulvene derivative was found to interact with water at room temperature to give clear water solutions, but TEM analysis demonstrated the presence of macromolecular aggregates showing dimensions larger than those suggested by SEC-MALS analysis for the isolated macromolecules. DLS studies confirmed the presence of objects showing average dimensions in the range of 200–300 nm and suggested thermoresponsive colloidal properties for poly-6-MOEG-9-TM-BF3kmacromolecules. Finally, owing to its favourable absorption/emission properties and water solubility, the interaction of poly-6-MOEG-9-TM-BF3k with live cells was studied by fluorescence microscopy experiments, which revealed that the polymer brush was unable to enter live cells and alter cell morphology.
1. Introduction
Click chemistry is a concept developed by Sharpless in 20011 to classify organic reactions capable of producing chemical substances rapidly and quantitatively without byproducts and side reactions under mild reaction conditions, and with broad tolerance to functional groups and applicability. Several reaction types have been recognized to possess these prerequisites and all these organic reactions are characterized by a large thermodynamic driving force, which leads to rapid completion with the formation of a single product.1 Among them, the most popular reaction is the Huisgen 1,3-dipolar cycloaddition between organic azides and alkynes that has been improved by means of the introduction of Cu(I) catalysis as realized independently by the Meldal and the Sharpless laboratories.2
Click chemistry has been successfully applied also to polymer synthesis.3 Among many notable applications in polymer synthesis, Gao and Matyjaszewski demonstrated the usefulness of click chemistry in a “grafting onto” approach for the preparation of molecular brushes [or cylindrical polymer brushes (CPBs)]. In this approach, linear poly(2-hydroxyethyl methacrylate) (PHEMA) backbone was functionalized with different side chains (SCs) after derivatization with pentynoic acid and subsequent “click reaction” with suitable azido-terminated polymeric SCs to obtain a variety of CPBs showing a maximum grafting density of 88%.4
In general, cylindrical polymer brushes are formed by a linear polymeric backbone densely grafted (ideally with one SC per monomeric unit) with suitable polymeric side chains and can be synthesized by means of three different approaches: “grafting through” (the polymerization of macromonomers), “grafting onto” (the addition of previously prepared SCs to a polymeric backbone), and “grafting from” (the polymerization of SCs from a macroinitiator backbone).5,6 The particular features shown by CPBs are regulated by the properties of the components (side chains and backbone) and by the grafting density. Thus, side chain length and grafting density along with environment characteristics such as solvent properties, temperature, pH, and ionic strength, may play a key role in affecting the conformational behavior of CPBs.5
Polymerization of PEG-based macromonomers such as oligo(ethylene glycol) methacrylate (OEGMA) has been profitably exploited in the synthesis of polymers bearing densely grafted PEG SCs.7–14 For instance, methacrylates bearing short oligo(ethylene glycol) alkyl ether SCs were polymerized in the presence of anionic initiators to give poly[oligo(ethylene glycol) alkyl ether methacrylate]s characterized by different water solubility and lower critical solution temperature (LCST).11 Moreover, transfer radical polymerization (ATRP) of a commercially available OEGMA derivative was performed in aqueous media to obtain OEGMA polymers showing low polydispersity.12,13ATRP methodology was also employed to polymerize 2-(2-methoxyethoxy)ethyl methacrylate with OEGMA and obtain poly-(MEO2MA-co-OEGMA) copolymers showing controlled chain length, narrow molecular weight distribution, interesting solution properties in water, and tunable thermosensivity.8
Benzofulvene derivative BF1 (Scheme 1) was found to polymerize, in the apparent absence of catalysts or initiators, by the removal of solvents to give a new hydrophobic high-molecular-weight polymer (poly-BF1) showing vinyl structure stabilized by aromatic stacking interactions.15,16 This intriguing finding stimulated the preparation of a series of benzofulvene derivatives BF3 in order to explore the role of substituents of benzofulvene moiety on the polymer properties such as formation, molecular weight, structure, thermoreversibility, and aggregation in nanostructured entities.17 Thus, desmethyl derivative BF3k of the prototypical benzofulvene monomer BF1 was found to form a polymer (poly-BF3k), which showed macromolecular features very similar to those shown by poly-BF1.17
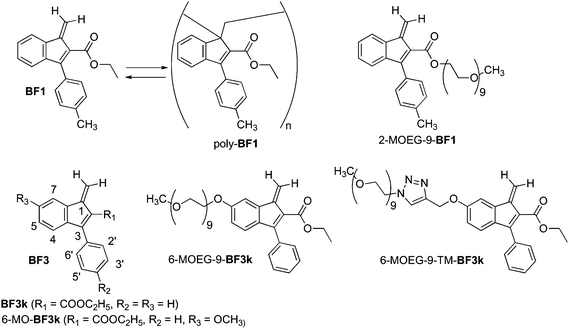 |
| Scheme 1 Structure of some benzofulvene derivatives. | |
The insertion of monomethyl oligo(ethylene glycol) (MOEG) side chains onto polybenzofulvene backbone led to two polymer brushes: poly-2-MOEG-9-BF118 and poly-6-MOEG-9-BF3k.19 The first polymer interacted with water to give a compact physical hydrogel stable to ultrasound exposure, whereas, the physical hydrogel obtained with poly-6-MOEG-9-BF3k was rapidly broken by ultrasound to give a milky dispersion leading to the separation of a transparent hydrogel. In addition, both 1H-NMR analysis and TEM experiments showed the presence of poly-6-MOEG-9-BF3k in the supernatant solution in equilibrium with the hydrogel. These results suggest that the difference in MOEG-9 attachment between poly-2-MOEG-9-BF1 and poly-6-MOEG-9-BF3k produces differences in the behavior of the corresponding hydrogels that may be related to differences in intermolecular interactions and aggregation properties.
The work described in the present paper shows that the insertion of a triazolomethyl (TM) spacer in the SCs of poly-6-MOEG-9-BF3k leads to the water-soluble polymer brush poly-6-MOEG-9-TM-BF3k. This polymer was prepared by means of a “grafting through” approach in which a copper(I)-catalyzed alkyne-azide 1,3-dipolar cycloaddition (CuAAC) step was followed by the spontaneous polymerization of macromonomer 6-MOEG-9-TM-BF3k. These key steps of poly-6-MOEG-9-BF3k synthesis share most of the main features of the click reactions, and the spontaneous polymerization appears to be driven by an effective monomer recognition/interaction as it occurs in “affinity polymerization” reactions.
2. Results and discussion
2.1. Synthesis and spontaneous polymerization of benzofulvene macromonomer 6-MOEG-9-TM-BF3k
Benzofulvene macromonomer 6-MOEG-9-TM-BF3k was synthesized starting from indenone 1,20 which was reacted with propargyl bromide to obtain compound 2 (Scheme 2). Propargyl indenone 2 was reacted with a MOEG-9 chain bearing an azide group (MOEG-9-N33) in THF in the presence of Cu(Ph3P)3Br and DIPEA to give indenone derivative 3. This compound was submitted to the same reaction sequence used for the synthesis of BF1. The reaction of 3 with trimethylaluminium gave the expected indenol derivative 4, which was dehydrated with p-toluenesulfonic acid (PTSA) to give macromonomer 6-MOEG-9-TM-BF3k. Benzofulvene monomer 6-MOEG-9-TM-BF3k polymerized, in the apparent absence of catalysts, upon solvent removal to give the corresponding polymer. Very interestingly, the increase in the structural complexity produced by the TM-spacer insertion in macromonomer SC did not affect the monomer interactions leading to polymerization. This result suggests the existence of a very effective recognition process capable of pre-organizing the monomer molecules for spontaneous polymerization.
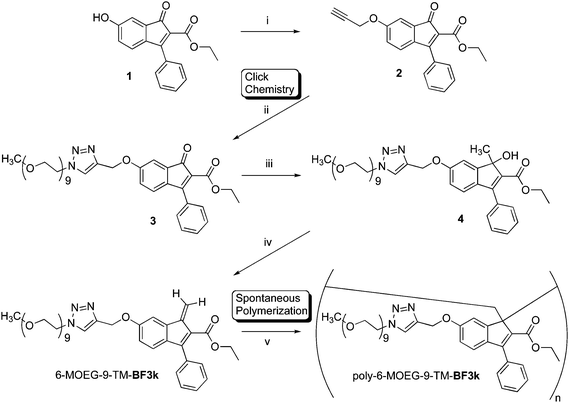 |
| Scheme 2 Grafting through approach used in the synthesis of molecular brush poly-6-MOEG-9-TM-BF3k. Reagents: (i) propargyl bromide, K2CO3, NaI, DMF; (ii, Click Chemistry) CH3(OCH2CH2)9N3, Cu(PPh3)3Br, DIPEA, THF; (iii) Al(CH3)3, CH2Cl2; (iv) PTSA, CHCl3; (v, Spontaneous Polymerization) solvent elimination. | |
2.2. Properties of poly-6-MOEG-9-TM-BF3k
Poly-6-MOEG-9-TM-BF3k showed an excellent solubility in the most common organic solvents. In dichloromethane or chloroform the polymer dissolved very rapidly, while a more gradual dissolution of the polymer was observed in water. For instance, ca. 10 mg of the polymer was dissolved in 1 mL of water and the dissolution was complete after a few minutes of sonication at room temperature to obtain a transparent solution. Remarkably, the comparison of this behavior with that observed with parent macromolecule poly-6-MOEG-9-BF3k suggests that SC elongation increases the interaction with water and alters the aggregation propensity. We assume that the insertion of TM-spacers increases the efficiency of the interaction between MOEG-9 SCs (hydrophilic domain) and water by separating the hydrophilic domain from the hydrophobic one (polybenzofulvene backbone).
2.2.1. Characterization of molecular weight distribution
The characterization of molecular weight distribution (MWD) in a poly-6-MOEG-9-TM-BF3k sample was performed by means of a multi-angle laser light scattering (MALS) detector on-line to a size exclusion chromatography (SEC) system using a (8
:
2) mixture of THF-(DMF + 0.01M LiBr) as the mobile phase. The analysis showed a Mw of 358 kg mol−1 and a polydispersity index (PDI = Mw/Mn = 3.9) consistent with the data obtained with poly-6-MOEG-9-BF3k (Table 1). The average size of the macromolecular radius of gyration (Rg) was approximately 15.5 nm and the conformation plot (Rg = KMα) was linear with slope α = 0.55 typical of polymers showing random coil conformations in a good solvent. However, the SEC trace suggested a tendency to aggregate for poly-6-MOEG-9-TM-BF3k, and this result stimulated an extensive characterization of the polymer behavior in solution by dynamic light scattering (DLS) and transmission electron microscopy (TEM, see below).
Table 1 Macromolecular features of the newly synthesized poly 6-MOEG-9-TM-BF3k compared to those shown by previously reported poly-6-MOEG-9-BF3k, poly-6-MO-BF3k, and poly-BF3k
Polymer
|
M
p/kg mol−1 |
M
w/kg mol−1a |
PDIb |
R
g/nmc |
M
w: weight-average molecular weight.
PDI: the polydispersity index PDI = Mw/Mn where Mn denotes the numeric-average molecular weight.
R
g: radius of gyration i.e. the dimension of the macromolecules.
|
Poly-6-MOEG-9-TM-BF3k |
193 |
358 |
3.9 |
15.5 |
Poly-6-MOEG-9-BF3k |
527 |
456 |
1.8 |
20.8 |
Poly-6-MO-BF3k |
312 |
347 |
4.3 |
|
Poly-BF3k |
|
1593 |
3.7 |
44.6 |
2.3. Structure of poly-6-MOEG-9-TM-BF3k
2.3.1.
NMR
.
Previous 13C-NMR studies carried out with poly-6-MOEG-9-BF3k and poly-2-MOEG-9-BF1 suggested that the presence of MOEG side chain does not affect the vinyl (1,2) polymerization mechanism.18,19 In order to establish whether the insertion of TM-spacer has effects on the poly-6-MOEG-9-TM-BF3k structure, the 13C-NMR spectrum of this polymer was compared to that of poly-6-MOEG-9-BF3k (Fig. 1). The comparison shows the evident correspondence between poly-6-MOEG-9-TM-BF3k and poly-6-MOEG-9-BF3kspectra in the low-field portion containing C-1′ and C-3 signals (which may be affected by the competing 1,4-polymerization) and supports the hypothesis on the retention of the spontaneous 1,2-polymerization mechanism in these benzofulvene carboxylate derivatives. Moreover, the 13C-NMR spectrum of poly-6-MOEG-9-TM-BF3k differs from that of poly-6-MOEG-9-BF3k by the presence of additional signals attributable to TM-spacer and by the up-field shift of the signal attributed to the methylene carbon of MOEG SC directly attached to the triazole ring (around 51 ppm in poly-6-MOEG-9-TM-BF3k).
 |
| Fig. 1
13C-NMR spectrum (CDCl3) of poly-6-MOEG-9-TM-BF3k compared with those of previously reported poly-6-MOEG-9-BF3k, poly-6-MO-BF3k, and model compound 5.19,20 | |
2.3.2. Absorption and emission spectroscopy.
The UV-vis absorption spectrum of poly-6-MOEG-9-TM-BF3k is perfectly comparable to those of both the parent macromolecule poly-6-MOEG-9-BF3k and the model compound 5.19,20 The presence of TM-spacer appears unable to affect the UV-vis absorption of the chromophore. The spectrum of poly-6-MOEG-9-TM-BF3k appears to be quite independent of solvent polarity (compare the yellow trace obtained with dichloromethane with the violet trace obtained with water as the solvent) and shows the existence of two absorption components (one centered around 240 nm attributable to the 3-phenylindene moiety and a second centered at about 310 nm related to the presence of a p-methoxycinnamate moiety) as in the case of model compound 5. But the direct comparison of spectra obtained at the same concentration, indicates a significant hypochromism for poly-6-MOEG-9-TM-BF3k with respect to chromophore model 5 that resulted as slightly superior to that observed in parent macromolecule poly-6-MOEG-9-BF3k (Fig. 2).
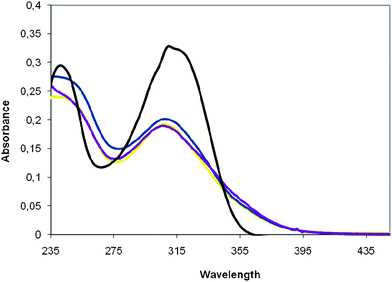 |
| Fig. 2 Comparison of the absorption spectra of poly-6-MOEG-9-TM-BF3k in CH2Cl2 (yellow), poly-6-MOEG-9-BF3k in CH2Cl2 (blue), chromophore model 5 in CH2Cl2 (black), and poly-6-MOEG-9-TM-BF3k in H2O (violet). The spectra were obtained at room temperature and normalized with respect to the concentration (ca. 1.7 × 10−5 mol L−1). | |
Also, the emission spectrum of poly-6-MOEG-9-TM-BF3k is very similar to that of the parent macromolecule poly-6-MOEG-9-BF3k and shows a main peak centered at about 460 nm, bearing a shoulder at about 500 nm (Fig. 3).
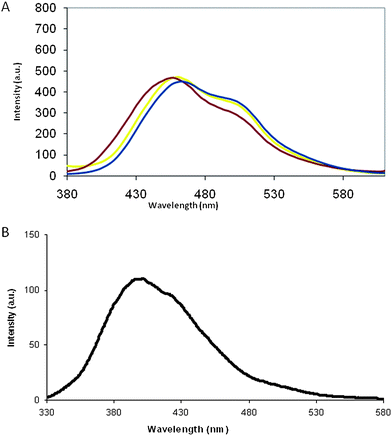 |
| Fig. 3 (A) Comparison of the emission spectra of poly-6-MOEG-9-TM-BF3k in CH2Cl2 (yellow), poly-6-MOEG-9-BF3k in CH2Cl2 (blue), and poly-6-MOEG-9-TM-BF3k in H2O (violet). The spectra were obtained at room temperature (excitation wavelength was 320 nm) and normalized with respect to the concentration (1.7 × 10−5 mol L−1). (B) Emission spectrum of chromophore model 5. The spectrum was obtained in dichloromethane (concentration ca. 2.7 × 10−5 mol L−1) at room temperature. The excitation wavelength was 310 nm. | |
When the emission spectrum of poly-6-MOEG-9-TM-BF3k in dichloromethane is compared to the one obtained with 5, a significant red-shift becomes apparent. This shift, along with the hypochromism discussed above, suggests that the presence of the TM-spacer does not alter the degree of chromophore stacking assumed for poly-BF1 congeners.16–19
Differing from the absorption spectrum, the emission of poly-6-MOEG-9-TM-BF3k appears to be slightly dependent on solvent polarity (weak solvatochromic effect). In fact, the comparison of the yellow trace obtained with dichloromethane to violet trace obtained with water as the solvent shows both a decrease in the shoulder intensity and a slight blue-shift of the main component. This result can be interpreted in terms of effects of solvent polarity on the excited state energy or different stacking related to different conformational preferences induced by the solvent, but more detailed studies are needed to address the question.
2.3.3. MALDI-TOF mass spectrometry.
The structure and chemical composition of neat poly-6-MOEG-9-TM-BF3k were also studied by matrix-assisted laser desorption time-of-flight mass spectrometry (MALDI-TOF MS)21,22 in positive ions mode, using 2-(4-hydroxylphenylazo)benzoic acid (HABA) as a matrix in the presence of sodium trifluoroacetate as cationizating agent. A typical mass spectrum recorded in linear mode is displayed in Fig. 4. It shows clusters of homologous peaks separated by a molecular mass of 783.9 ± 0.2 uma, corresponding to the mass of the repeating unit of the analyzed polymer, in agreement with the synthetic procedure (Scheme 2). The spectrum shows poly-6-MOEG-9-TM-BF3k oligomers from monomer to the nonamer. Each cluster of peaks presents the protonated and sodiated ions of the polymer chains terminated with hydrogen at both ends (H/H; species A), as well as those of the oxidized benzofulvene unit having one aldehyde moiety (species B). This can be seen in the inset in Fig. 4, which displays the experimental and calculated portions of the mass spectra due to dimers A2 and B2 with a molar ratio of 60
:
40. The monomer may be formed by depolymerization of the polymer induced by laser radiation or by processes occurring during the preparation procedure used for MALDI analyses. The mass spectrum shows also some clusters of peaks corresponding to the adduct of the oligo-6-MOEG-9-TM-BF3k chains (species A and B) with the HABA matrix; these peaks are labelled with the symbol * in Fig. 4.
Previous studies showed that poly-benzofulvene derivatives are characterized by a depolymerization15,17–19 similar to the unzipping behavior typical of cyanoacrylates.23,24 Therefore, in order to ascertain the influence of the insertion of TM-spacer on the thermo-induced depolymerization of poly-6-MOEG-9-TM-BF3k, a solution of this polymer (5.0 mg in 0.5 mL of nitrobenzene-d5) was heated at 150 °C and 1H-NMR spectra were acquired at regular time intervals. Fig. 5 shows that poly-6-MOEG-9-TM-BF3k depolymerized quite slowly at 150 °C, reaching an apparent equilibrium (after ca. 72 h, Table 2) characterized by the presence of a considerable amount of the monomer. By way of comparison, poly-6-MOEG-9-BF3kdepolymerization reached an apparent equilibrium after ca 48 h, whereas, unsubstituted poly-BF3k was reported to show an almost complete depolymerization after 3–6 h heating at 150 °C.19 Thus, the insertion of TM-spacer appears to slow the depolymerization process in agreement with the previously observed slowing effect caused by chain elongation.19
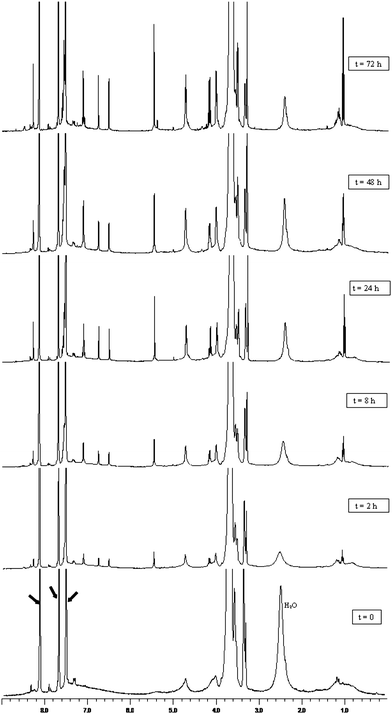 |
| Fig. 5 Thermoinduced depolymerization of poly-6-MOEG-9-TM-BF3k followed by 1H-NMR (400 MHz). A solution of 5.0 mg of poly-6-MOEG-9-TM-BF3k in 0.5 mL of nitrobenzene-d5 was heated at 150 °C, and 1H-NMR spectra were recorded at regular time intervals. In order to appreciate the variation in monomer concentration, the signals attributable to the vinylene group were integrated, and the integral values were compared to that of the lowest field signal of nondeuterated nitrobenzene, which was considered as an internal standard. Integrals are omitted in the figure for the sake of clarity. The arrows indicate the solvent peaks and H2O the water peak. | |
Table 2 Comparison of thermo-induced depolymerization of poly-6-MOEG-9-TM-BF3k with those shown by previously reported poly-6-MOEG-9-BF3k, poly-6-MO-BF3k, poly-BF3k, followed by 1H-NMR spectroscopy
Polymer
|
Thermo-induced depolymerization at 150 °C by 1H-NMRa |
In the thermoreversibility experiments, samples of the polymers (5.0 mg) were dissolved into nitrobenzene-d5 (0.5 mL) and heated in an oil bath thermostatted at 150 °C; the 1H-NMR experiments were performed at regular time intervals.
|
Poly-6-MOEG-9-TM-BF3k |
Apparent equilibrium after 72 h |
Poly-6-MOEG-9-BF3k |
Apparent equilibrium after 48 h |
Poly-6-MO-BF3k |
Apparent equilibrium after 8–24 h |
Poly-BF3k |
Depol. almost complete after 3–6 h |
2.5. Macromolecular structure of poly-6-MOEG-9-TM-BF3k
A clear water solution of poly-6-MOEG-9-TM-BF3k was observed by transmission electron microscopy (TEM) after negative staining with uranyl acetate. The images obtained (Fig. 6) show the presence of a cluster of macromolecular aggregates. The dimensions of the isolated macromolecules determined by SEC-MALS in a good solvent (radius of gyration around 15 nm) suggest that the aggregates observed by TEM analysis are formed by a number of single macromolecules. However, because of a tendency to aggregate, shown by poly-6-MOEG-9-TM-BF3k, the large polymer aggregates could be formed as a possible result of a collapse occurring in the sample preparation for TEM experiments.
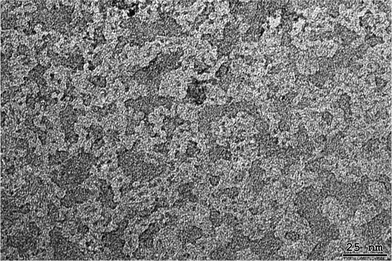 |
| Fig. 6 Structure of macromolecular aggregates found by TEM analysis of poly-6-MOEG-9-TM-BF3k solution in water. | |
In order to evaluate this hypothesis, dynamic light scattering (DLS) studies were performed by using polymer solutions in water in the concentration range of 0.1–1.0 mg mL−1 and at 25 and 37 °C. Very interestingly, DLS measurements of clear solutions at 25 °C revealed the presence of objects showing average dimension of 245 nm with a PDI value of 0.43. When the temperature was raised to 37 °C the average dimension of the objects present in the polymer dispersion increased to 302 nm and PDI decreased to 0.20 (see DLS curves in Fig. 7). Actually, this finding is consistent with the macroscopic observation that the polymer solution, at a minimum concentration of 1.0 mg mL−1, became slightly opalescent when heated at 37 °C.
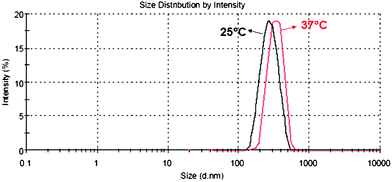 |
| Fig. 7 Comparison of the size distribution curves of poly-6-MOEG-9-TM-BF3k recorded at 25 and 37 °C by DLS (sample concentration 0.1 mg mL−1). | |
These results appear to agree with the information obtained with TEM experiments and for poly-6-MOEG-9-TM-BF3kwater solutions suggest the features of a thermoresponsive colloidal dispersion. Moreover, the low Z-potential value measured (−1.24 mV) is consistent with the pronounced aggregation liability shown by this polymer brush.
2.6. Interaction of poly-6-MOEG-9-TM-BF3k with live cells
The absorption/emission features of poly-6-MOEG-9-TM-BF3k were considered to be compatible with its employment in a fluorescence microscopy apparatus with standard filters commonly available for use with fluorescence stain DAPI (4′-6-diamidino-2-phenylindole). Thus, the interaction of poly-6-MOEG-9-TM-BF3k with HEK293T cells was investigated by fluorescence microscopy. Cells were plated on coverslips and allowed to grow overnight. Coverslips were then washed and incubated with polymer solutions (0.017, 0.17, and 1.7 mg mL−1) in phosphate buffered saline (PBS) for 30 minutes at 4 °C, room temperature, and 37 °C. Cells were washed with PBS and mounted in order to be observed with a fluorescence microscope. The presence of the polymer was easily detected in the solutions by fluorescence microscopy, but it was not found in the cells after the polymer solution has been eliminated. This result suggests that the polymer, probably because of its tendency to aggregate, is unable to interact and cross the membrane of HEK293T cells in the present experimental conditions. Moreover, the cell morphology was not altered by the presence of the polymer, and this result suggests the absence of toxic effects (Fig. 8).
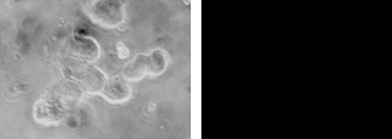 |
| Fig. 8 The left panel shows a phase-contrast image of a group of HEK293T cells, in the right panel, the same cells are imaged after excitation at 365 nm. | |
3. Conclusions
A new biorelevant polymer brush, based on the polybenzofulvene backbone, was prepared by means of a “grafting through” approach in which the most popular click chemistry CuAAC reaction was used to assemble the precursor of macromonomer 6-MOEG-9-TM-BF3k. This was found to polymerize spontaneously in the apparent absence of catalysts or initiators suggesting the existence of a particularly efficient recognition process capable of pre-organizing the monomer molecules for the polymerization (“affinity polymerization”). The results described in the present paper demonstrate that the insertion of a TM-spacer in the side chains of poly-6-MOEG-9-BF3k leads to the water-soluble poly-6-MOEG-9-TM-BF3k showing structural features very similar to those shown by the parent macromolecules. In particular, 13C-NMR spectroscopy as well as UV-vis and fluorescence spectroscopy showed a close similarity between poly-6-MOEG-9-TM-BF3k and poly-6-MOEG-9-BF3k. SEC-MALS studies for poly-6-MOEG-9-TM-BF3k showed a high molecular weight and a large PDI. In the depolymerization experiments performed in deuterated nitrobenzene as the solvent, poly-6-MOEG-9-TM-BF3k showed a behavior similar to that shown by the parent macromolecule poly-6-MOEG-9-BF3k. TEM analysis of poly-6-MOEG-9-TM-BF3k solutions in water demonstrated the presence of a cluster of macromolecular aggregates showing dimensions larger than those suggested by SEC-MALS analysis for the isolated macromolecules. DLS studies confirmed the presence of objects showing average dimensions in the range of 200–300 nm and suggested thermoresponsive colloidal properties for poly-6-MOEG-9-TM-BF3kmacromolecules.
Owing to the similarities existing between the absorption/emission spectra of poly-6-MOEG-9-TM-BF3k and the fluorescence microscopy reagent DAPI, the interaction of the polymer with live cells was investigated by fluorescence microscopy. These experiments revealed that in the condition used, the polymer brush was unable to enter live cells and to alter cell morphology, suggesting the absence of toxic effects. These features make this polymer brush potentially useful as a scaffold in the development of new macromolecular nanocarriers.
4. Experimental section
Synthesis
Melting points were determined in open capillaries in a Gallenkamp apparatus and were uncorrected. UV/vis spectra were recorded with a Shimadzu 260 spectrophotometer and the emission spectra were performed by means of a Perkin Elmer LS45 instrument. Merck silica gel 60 (230–400 mesh) was used for column chromatography. Merck TLC plates, silica gel 60 F254 were used for TLC. NMR spectra were recorded with a Bruker AC200, a Varian Mercury-300, a Bruker DRX-400 AVANCE, a Bruker DRX-500 AVANCE, or a Bruker DRX-600 AVANCE spectrometer in the indicated solvents (TMS as internal standard): the values of the chemical shifts are expressed in ppm and the coupling constants (J) in Hz. An Agilent 1100 LC/MSD operating with an electrospray source was used in mass spectrometry experiments.
28-Azido-2,5,8,11,14,17,20,23,26-nonaoxaoctacosane (MOEG-9-N3)
A mixture of 2,5,8,11,14,17,20,23,26-nonaoxaoctacosan-28-yl methanesulfonate25 (1.5 g, 3.0 mmol) in DMF (15 mL) with NaN3 (0.39 g, 6.0 mmol) and Bu4NBr (0.039 g, 0.12 mmol) was stirred at 120 °C for 24 h. The reaction mixture was then concentrated under reduced pressure and the resulting residue was treated with CH2Cl2 (25 mL). The solid was filtered off and the filtrate was washed with water, dried over sodium sulfate and evaporated under reduced pressure. Purification of the residue by flash chromatography with ethyl acetate-methanol (9
:
1) as the eluent gave MOEG-9-N33 (0.93 g, yield 68%) as a pale yellow oil. 1H-NMR (400 MHz, CDCl3): 3.22 (m, 5H), 3.51 (m, 2H), 3.62 (m, 32H). 13C-NMR (100 MHz, CDCl3): 50.68, 59.00, 70.02, 70.62, 71.93. MS(ESI): m/z 476 (M + Na+).
A mixture of indenone 120 (0.70 g, 2.4 mmol) in DMF (10 mL) containing K2CO3 (1.0 g, 7.24 mmol), NaI (0.54 g, 3.6 mmol), and propargyl bromide (0.26 mL, 2.9 mmol) was stirred overnight at room temperature. The reaction mixture was then diluted with a saturated NH4Cl solution and extracted with ethyl acetate. The organic layer was dried over sodium sulfate and concentrated under reduced pressure. Purification of the residue by flash chromatography with petroleum ether (40–60°C) -ethyl acetate (1
:
1) as the eluent gave the expected indenone derivative 2 (0.64 g, yield 80%) as a red solid. An analytical sample was obtained by recrystallization from n-hexane (red crystals, mp 111–112 °C). 1H-NMR (400 MHz, CDCl3): 1.13 (t, J = 7.1, 3H), 2.54 (t, J = 2.3, 1H), 4.16 (q, J = 7.1, 2H), 4.71 (d, J = 2.3, 2H), 6.91 (dd, J = 8.2, 2.4, 1H), 7.08 (d, J = 8.2, 1H), 7.21 (d, J = 2.4, 1H), 7.48 (m, 5H). MS (ESI): m/z 355 (M + Na+).
Ethyl 6-[[1-(2,5,8,11,14,17,20,23,26-nonaoxaoctacosan-28-yl)-1H-1,2,3-triazol-4-yl]methoxy]-1-oxo-3-phenyl-1H-indene-2-carboxylate (3)
A mixture of indenone 2 (0.48 g, 1.44 mmol) in THF (20 mL) with Cu(Ph3P)3Br (0.027 g, 0.029 mmol), DIPEA (0.005 mL, 0.029 mmol) and MOEG-9-N33 (0.66 g, 1.46 mmol) was heated under reflux for 18 h and then concentrated under reduced pressure. The residue was purified by flash chromatography with ethyl acetate-methanol (9
:
1) as eluent to obtain compound 3 (0.52 g, yield 46%) as a red oil. 1H-NMR (400 MHz, CDCl3): 1.12 (t, J = 7.1, 3H), 3.34 (s, 3H), 3.50–3.61 (m, 32H), 3.85 (t, J = 4.8, 2H), 4.15 (q, J = 7.1, 2H), 4.53 (t, J = 4.8, 2H), 5.22 (s, 2H), 6.95 (dd, J = 8.1, 2.0, 1H), 7.06 (d, J = 8.1, 1H), 7.21 (d, J = 2.0, 1H), 7.48 (m, 5H), 7.87 (s, 1H). MS (ESI): m/z 808 (M + Na+).
Ethyl 6-[[1-(2,5,8,11,14,17,20,23,26-nonaoxaoctacosan-28-yl)-1H-1,2,3-triazol-4-yl]methoxy]-1-hydroxy-1-methyl-3-phenyl-1H-indene-2-carboxylate (4)
To a mixture of indenone derivative 3 (0.46 g, 0.59 mmol) in CH2Cl2 (15 mL) was added a solution (2M in THF) of Al(CH3)3 (0.60 mL, 1.2 mmol) and the resulting mixture was stirred at room temperature for 30 min. The Al(CH3)3 excess was cautiously decomposed with a 30% NaOH solution until the gas evolution ceased. The mixture was filtered and the filtrate was dried over sodium sulfate and evaporated under reduced pressure. Purification of the residue by flash chromatography with ethyl acetate-methanol (9
:
1) as the eluent gave compound 4 (0.21 g, yield 44%) as a pale yellow oil. 1H-NMR (400 MHz, CDCl3): 1.03 (t, J = 7.1, 3H), 1.74 (s, 3H), 3.35 (s, 3H), 3.51–3.70 (m, 32H), 3.87 (t, J = 4.9, 2H), 4.10 (m, 2H), 4.54 (t, J = 4.9, 2H), 5.25 (s, 2H), 6.89 (dd, J = 8.2, 2.2, 1H), 7.04 (d, J = 8.4, 1H), 7.22 (d, J = 2.0, 1H), 7.40 (m, 5H), 7.84 (s, 1H). MS (ESI): m/z 824 (M + Na+).
Ethyl 6-[[1-(2,5,8,11,14,17,20,23,26-nonaoxaoctacosan-28-yl)-1H-1,2,3-triazol-4-yl]methoxy]-1-methylene-3-phenyl-1H-indene-2-carboxylate (6-MOEG-9-TM-BF3k)
A mixture of indenol derivative 4 (0.17 g, 0.21 mmol) in CDCl3 (4.2 mL) with the catalytic amount of p-toluenesulfonic acid monohydrate (PTSA) (0.021 g, 0.11 mmol) was heated under reflux for 3 h and cooled to room temperature. The reaction mixture was washed with a saturated solution of NaHCO3 and dried over sodium sulfate to afford a stock (about 0.05 M) solution of the monomer 6-MOEG-9-TM-BF3k that was stored under an argon atmosphere. 1H-NMR (400 MHz, CDCl3): 1.04 (t, J = 7.1, 3H), 3.37 (s, 3H), 3.48–3.69 (m, 32H), 3.87 (t, J = 4.9, 2H), 4.10 (q, J = 7.1, 2H), 4.56 (t, J = 4.9, 2H), 5.28 (s, 2H), 6.34 (s, 1H), 6.60 (s, 1H), 6.91 (dd, J = 8.4, 2.1, 1H), 7.11 (d, J = 8.4, 1H), 7.40 (m, 6H), 7.86 (s, 1H). MS (ESI): m/z 806 (M + Na+).
Poly[ethyl 6-[[1-(2,5,8,11,14,17,20,23,26-nonaoxaoctacosan-28-yl)-1H-1,2,3-triazol-4-yl]methoxy]-1-methylene-3-phenyl-1H-indene-2-carboxylate] (poly-6-MOEG-9-TM-BF3k)
The solution of benzofulvene monomer 6-MOEG-9-TM-BF3k in chloroform was concentrated under reduced pressure to give a viscous oil, which was dissolved in chloroform and newly evaporated (the dissolution/evaporation procedure was repeated three times). The final residue was purified by washing with diethyl ether and dried under reduced pressure to obtain the poly-6-MOEG-9-TM-BF3k (yield 44%) as a pale yellow oil. 1H-NMR (400 MHz, CDCl3): 0.1–1.1 (br m, 3H), 3.3 (s, 3H), 3.4–5.5 (br m, 42H), 5.6–8.2 (br m, 9H).
SEC-MALS
The MWD characterization of poly-6-MOEG-9-TM-BF3k was performed by a MALS light scattering photometer on-line to a SEC chromatographic system. The SEC-MALS system and the corresponding experimental conditions were identical to those used in our previous study16,17 and are not reported in detail here.
MALDI-TOF mass spectra of poly-6-MOEG-9-TM-BF3k were recorded in reflectron and linear mode, using a Voyager-DE STR (Perseptive Biosystem) mass spectrometer, equipped with a nitrogen laser emitting at 337 nm, with a 3 ns pulse width, and working in positive ion mode. The accelerating voltage was 20 KV, the grid voltage and delay time (delayed extraction, time lag) were optimized for each sample to achieve a higher mass resolution, expressed as the molecular weight of a given ion divided by the full width at half maximum (FWHM). For all spectra, a mass resolution of about 1000 ÷ 1500 g mol−1 was obtained. The laser irradiance was maintained slightly above the threshold. 2-(4-Hydroxylphenylazo)benzoic acid (HABA) 0.1 M in THF, and 1,8,9 anthracenetriol (dithranol) were used as matrices in the presence of CF3COONa (10−2 M in THF) and CF3COOAg (10−3 M in THF) salts, respectively, as cationizating agents (doping agents). Typically, 0.5 μL of a CHCl3 solution of copolymer (5 mg mL−1) was mixed with 0.5 μL or 1.5 μL of a matrix solution and 0.5 μL of a salt solution, and then spotted on the MALDI sample holder and slowly dried to allow matrix crystallization. The better spectra were recorded using a (matrix solution)/(polymer solution) ratio of 3/1. The mass peaks corresponding to the macromolecular species were measured with an accuracy of ± 0.5 g mol−1. The spectra were calibrated using an external calibration file made by MALDI analysis of a Ny6 sample terminated with only amino groups and having a Mn of about 3000 g mol−1, appropriately synthesized. The better spectra were recorded using HABA as the matrix and CF3COONa as the doping agent.
Dynamic light scattering (DLS) studies and Z-potential measurements
DLS studies and Z-potential measurements were performed at 25 and 37 °C using a Malvern Zetasizer NanoZS instrument, fitted with a 532 nm laser at a fixed scattering angle of 90°. The polymer samples were molecularly dissolved into double distilled water (pH ≈ 6) at concentrations ranging from 0.1 to 1.0 mg mL−1 and the intensity-average hydrodynamic diameter (size in nm) and polydispersity index (PDI) were obtained by cumulant analysis of the correlation function. The Z-potential (mV) was calculated from the electrophoretic mobility using the Smoluchowsky relationship and assuming that Ka ≫ 1 (where K and a are the Debye–Hückel parameter and particle radius, respectively). Each experiment was performed in triplicate.
Interaction with live cells
HEK293T
cells were plated on coverslips and allowed to grow overnight. Coverslips were washed and incubated with 0.017, 0.17, and 1.7 mg mL−1 of poly-6-MOEG-9-TM-BF3k in PBS for 30 minutes at 4 °C, room temperature, and 37 °C. Cells on coverslips were washed with PBS and mounted in order to be observed with an Axioplan Epifluorescence Microscope (Zeiss).
Acknowledgements
Thanks are due to Italian MUR (Ministero dell'Università e della Ricerca) for financial support. Prof. Stefania D'Agata D'Ottavi's careful reading of the manuscript is also acknowledged.
References
- H. C. Kolb, M. G. Finn and K. B. Sharpless, Angew. Chem., Int. Ed., 2001, 40, 2004–2021 CrossRef CAS.
- M. Meldal and C. W. Tornøe, Chem. Rev., 2008, 108, 2952–3015 CrossRef CAS.
- T. P. Lodge, Macromolecules, 2009, 42, 3827–3829 CrossRef CAS.
- H. Gao and K. Matyjaszewski, J. Am. Chem. Soc., 2007, 129, 6633–6639 CrossRef CAS.
- S. S. Sheiko, B. S. Sumerlin and K. Matyjaszewski, Prog. Polym. Sci., 2008, 33, 759–785 CrossRef CAS.
- G. Cavallaro, M. Licciardi, M. Di Stefano, G. Pitarresi and G. Giammona, Macromolecules, 2009, 42, 3247–3257 CrossRef CAS.
- J.-F. Lutz, J. Andrieu, S. Uzgiin, C. Rudolph and S. Agarwal, Macromolecules, 2007, 40, 8540–8543 CrossRef CAS.
- J.-F. Lutz and A. Hoth, Macromolecules, 2006, 39, 893–896 CrossRef CAS.
- J. K. Oh, K. Min and K. Matyjaszewski, Macromolecules, 2006, 39, 3161–3167 CrossRef CAS.
- L. Tao, G. Mantovani, F. Lecolley and D. M. Haddleton, J. Am. Chem. Soc., 2004, 126, 13220–13221 CrossRef CAS.
- T. Ishizone, A. Seki, M. Hagiwara, S. Han, H. Yokoyama, A. Oyane, A. Deffieux and S. Carlotti, Macromolecules, 2008, 41, 2963–2967 CrossRef CAS.
- X.-S. Wang and S. P. Armes, Macromolecules, 2000, 33, 6640–6647 CrossRef CAS.
- X.-S. Wang, S. F. Lascelles, R. A. Jackson and S. P. Armes, Chem. Commun., 1999, 1817–1818 RSC.
- M. Zhang and A. H. E. Müller, J. Polym. Sci., Part A: Polym. Chem., 2005, 43, 3461–3481 CrossRef CAS.
- A. Cappelli, G. Pericot Mohr, M. Anzini, S. Vomero, A. Donati, M. Casolaro, R. Mendichi, G. Giorgi and F. Makovec, J. Org. Chem., 2003, 68, 9473–9476 CrossRef CAS.
- A. Cappelli, M. Anzini, S. Vomero, A. Donati, L. Zetta, R. Mendichi, M. Casolaro, P. Lupetti, P. Salvatici and G. Giorgi, J. Polym. Sci., Part A: Polym. Chem., 2005, 43, 3289–3304 CrossRef CAS.
- A. Cappelli, S. Galeazzi, G. Giuliani, M. Anzini, A. Donati, L. Zetta, R. Mendichi, M. Aggravi, G. Giorgi, E. Paccagnini and S. Vomero, Macromolecules, 2007, 40, 3005–3014 CrossRef CAS.
- A. Cappelli, S. Galeazzi, G. Giuliani, M. Anzini, M. Grassi, R. Lapasin, G. Grassi, R. Farra, B. Dapas, M. Aggravi, A. Donati, L. Zetta, A. C. Boccia, F. Bertini, F. Samperi and S. Vomero, Macromolecules, 2009, 42, 2368–2378 CrossRef CAS.
- A. Cappelli, M. Paolino, P. Anzini, G. Giuliani, S. Valenti, M. Aggravi, A. Donati, R. Mendichi, L. Zetta, A. C. Boccia, F. Bertini, F. Samperi, S. Battiato, E. Paccagnini and S. Vomero, J. Polym. Sci., Part A: Polym. Chem., 2010, 48, 2446–2461 CrossRef CAS.
- J. H. Ahn, M. S. Shin, S. H. Jung, S. K. Kang, K. R. Kim, S. D. Rhee, W. H. Jung, S. D. Yang, S. J. Kim, J. R. Woo, J. H. Lee, H. G. Cheon and S. S. Kim, J. Med. Chem., 2006, 49, 4781–4784 CrossRef CAS.
-
G. Montaudo, M. S. Montaudo and F. Samperi, in Mass Spectrometry of Polymers, ed. G. Montaudo and R. P. Lattimer, CRC Press, Boca Raton, 2002, ch. 2 and 10 Search PubMed.
- G. Montaudo, M. S. Montaudo and F. Samperi, Prog. Polym. Sci., 2006, 31, 277–357 CrossRef CAS.
- C. Vauthier, C. Dubernet, E. Fattal, H. Pinto-Alphandary and P. Couvreur, Adv. Drug Delivery Rev., 2003, 55, 519–548 CrossRef CAS.
- D. C. Pepper, J. Appl. Polym. Sci.: Appl. Polym. Symp., 1978, 62, 65–77 CAS.
-
J. Riggs-Sauthier and B.-L. Deng, PCT Int. Appl., 2008112261, 18 Sept. 2008.
|
This journal is © The Royal Society of Chemistry 2011 |
Click here to see how this site uses Cookies. View our privacy policy here.