DOI:
10.1039/C1PY00300C
(Paper)
Polym. Chem., 2011,
2, 2543-2547
pH-triggered micellar membrane for controlled release microchips†
Received
2nd July 2011
, Accepted 1st August 2011
First published on 16th September 2011
Abstract
A pH-responsive membrane based on polystyrene-b-poly(4-vinylpyridine) (PS-b-P4VP) block copolymer was developed on a model glass microchip as a promising controlled polymer delivery system. The PS-b-P4VP copolymer assembles into spherical and/or worm-like micelles with styrene block cores and pyridine coronas in selective solvents. The self-assembled worm-like morphology exhibited pH-responsive behaviour due to the protonation of the P4VP block at low pH and it's deprotonation at high pH and thus constituting a switchable “off/on” system. Doxorubicin (Dox) was used as cargo to test the PS-b-P4VP membrane. Luminescence experiments indicated that the membrane was able to store Dox molecules within its micellar structure at neutral pH and then release them as soon as the pH was raised to 8.0. The performance of the cast membrane was predictable and most importantly reproducible. The physiochemical and biological properties were also investigated carefully in terms of morphology, cell viability and cell uptake.
Introduction
Controlled release systems are common in a variety of areas, including engineering, daily-chemistry and biomedicine.1–4 Recent development and advances in the field of microfabrication have made a new class of controlled release system, namely, microchips become possible for controlled drug release. The microchip system has a unique array of reservoirs, which enables features not possible with other drug delivery technologies,5–9 such as: 1) delivery of multiple drugs; 2) administration of several drug formulation in a single implantation procedure; 3) controlling the microchip size to make local chemical delivery possible. An advantage of local drug delivery is a high concentration of drug can be achieved at the site where it is needed, while keeping the systemic concentration of the drug at a low level.
The block copolymer polystyrene-b-poly(4-vinylpyridine) (PS-b-P4VP) was chosen for the membrane material due to its reliable operation, stability, and predictability. Moreover, PS-b-P4VP chemistry is well established including the phase separation behaviour of the copolymer during its assembly.10–19 When dissolved in well-selected solvents PS-b-P4VP can form well-defined micelles with styrene block constituting the cores and vinylpyridine chains the coronas. Upon pH change, the P4VP block can be protonated or deprotonated, providing an off/on switch mechanism for access to the PS cores. That is, when the pH increases to above the pKa of pyridine, the P4VP block is deprotonated and tends to shrink, thus exposing the payload encapsulated within the PS core and causing its release. At low pH, the pyridine groups are protonated and the blocks extend to minimize repulsion between like charges, thus forming a protective network and preventing the payload release from the PS core. In this pH range, release can be designed to occur within specific areas of the body.20,21 The human pancreas secretes an impressive amount of sodium bicarbonate–rich fluid with each meal, thus creating a basic environment in the body. The majority of fluid and bicarbonate in pancreatic juice arises from the pancreatic duct cells even though they compose only about 5% of the pancreatic mass.20 The ultimate goal of our work is to develop a microfabricated device with the ability to store and release multiple chemical substances on demand using a pH triggering mechanism.
Experimental section
Materials
Polystyrene-b-poly(4-vinylpyridine) block copolymer (PS43k-b-P4VP59k) was synthesized by sequential anionic polymerization of styrene followed by 4-vinylpyridine (4VP).22GPC and NMR measurements confirmed the structure and the block ratio of the copolymer (ESI Fig. S1 and S2†). PS120k-b-P4VP25k was purchased from Polymer Source, Inc., Canada. Doxorubicin hydrochloride (Dox·HCl) purchased from Sigma-Aldrich is converted to Doxorubicin (Dox) before loading experiments by adding triethylamine. N,N-Dimethylformamide (DMF) and all buffer solutions were purchased from Sigma-Aldrich, USA and used without further purification. The THP-1 cells (Human acute monocytic leukemia cell line) were grown in RPM1 1640 supplemented with 10% fetal calf serum plus 2 mM L-glutamine.
Instruments and methods
Doxorubicin (Dox) was measured using a Cary 5000 UV-Vis spectrophotometer at 485 nm. Scanning Electron Microscopy (SEM) images were obtained using a Quanta 600 FEG scanning electron microscope (SEM) from FEI® company, USA. Atomic Force Microscopy (AFM) topographies were performed using an Agilent 5400 SPM atomic force microscopy (AFM) from Agilent Technologies, Inc, USA, operating in the tapping mode, with scan range 90 μm × 90 μm × 8 μm. Silicon tips with a radius of 7 nm, a spring constant of 2 Nm-1, and resonance frequency of 70–80 kHz were used, after calibration with gold nanoparticles (diameter of 5 nm) to evaluate the tip radius. The 1H NMR spectrum was recorded on Bruker Avance III (400 MHz) spectrometer, using CDCl3 as the solvent. The GPC measurement for the synthesized polymer was performed on Agilent 1200 series apparatus equipped with a Refractive Index (RI) detector and Agilent PL-gel MIXED-C column, using polystyrene as a standard and tetrahydrofuran (THF) as eluent at a flow rate of 1.0 mL min−1 at 25 °C. Confocal microscopy images were taken using a Confocal Microscope (LSM 710) from Zeiss.
Dox-loaded pH responsive membrane preparation
We chose Dox as the payload because it is not only a common anti-cancer drug but also a fluorescent agent, which enables close monitoring of its release and cell uptake. The Dox-loaded membranes were cast from polymer solutions containing the PS-b-P4VP block copolymer with different concentrations of Dox in DMF. The solvent was left exposed in air for up to 1 min following immersing in water at room temperature. The membrane was cast on glass plates using casting blades with a 200 μm gate height at room temperature (ca. 20 °C) and 60% relative humidity. The control membrane was cast using the same method.
Morphology observation
Before Scanning Electron Microscopy (SEM) observation, the samples were coated with Au by sputtering using a K575X Emitech equipment. To measure the film thickness and the cross section, the membrane was randomly fractured at room temperature. The surface morphology of the membrane was also characterized using atomic force microscopy (AFM), using silicon tips on a cantilever and spring constants ranging between 20.0 and 80.0 nm were used.
Dox release
The pH-responsive payload release behaviour of the membrane was studied using an UV-spectrophotometer. Briefly, 2 mg of dried Dox-loaded membrane was immersed into a vial containing 2 mL of buffer solution (at pH value ranging from 2.0 to 8.0). At selected time intervals, the buffered solution (1 mL) inside the vial was removed from the vial for UV-Vis analysis and replaced by an equal volume of freshly buffered solution. The amount of released Dox was analyzed with the UV spectrophotometer using the previously established calibration. Each sample was measured three times.
Cell viability evaluation
After the THP-1 (Human acute monocytic leukemia cell line) were grown to certain amount (1 × 104 mL−1) in plastic culture bottle, 1 mL of the medium was taken out to centrifuge and re-suspended in 1 ml fresh medium and incubated with blank membrane without drug at different pH values (3.0, 6.8 and 9.0) in 24-well plates for 3 and 24 h. At selected time intervals, 20 μL medium was taken out and treated with Trypan blue for cell counting and assessment of cell viability.
Cell uptake study
THP-1
cells (1 × 104 mL−1) were treated with Dox-loaded membrane (Dox concentration 20 μg mL−1) in 24-well plates at pH 9.0 and at pH 7.4 (control experiment) for 3 h and 5 h. Then the cells were centrifuged and washed with PBS twice and re-suspended into PBS solution. 150 μL of suspension was taken out for cytospin, finally, the slides were observed by confocal laser scanning microscopy.
Result and discussion
Fabrication of Dox-loaded micellar membrane
In short, DMF solution of the designated PS-b-P4VP and Dox was cast on a model glass microchip. The microchip was then immersed in deionized water and then dried at room temperature (Fig. 1). PS-b-P4VP of different concentration (10, 20 and 25 wt%) and hydrophobic/hydrophilic ratios (PS43k-b-P4VP59k and PS120k-b-P4VP25k) were used in the preparation of the cast membrane.
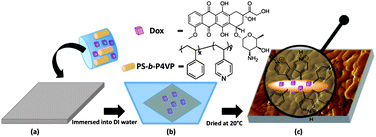 |
| Fig. 1 Schematic representation of Dox-loaded micellar membrane. | |
Morphology of the cast membrane
The PS-b-P4VP block copolymer can form micelles with different morphologies when they are dissolved into selective solvents.23–27 We first investigated the effect of different copolymer concentration on morphology. The well-defined micelles that present a “spherical” structure were found on the surface of the cast membrane based on PS43k-b-P4VP59k when the concentration was 10 wt% (Fig. 2a). DMF proved to be the preferred solvent for the pyridine block. When the PS-b-P4VP block copolymer is dissolved in DMF, the styrene block tends to avoid the unfavourable contact with DMF-rich medium and assemble into spherical core, while the pyridine block is preferentially exposed to the solvent and forms to the coronal shell. Consequently, the micelles with styrene block cores and pyridine coronas were formed quickly in order to minimize the free energy when the solution was immersed into water. When the concentration of the copolymer was increased, the self-assembled morphology had a transition from spherical nanomicelles at 10 wt%, to co-existent nanospheres and worm-like micelles 20 wt% (Fig. 2b), and to worm-like micelles when the concentration was increased to 25 wt% (Fig. 2c). These worm-like micelles are entangled and their extremities are hardly discernible. Moreover, their cross-section is slightly larger than the diameter of the micelles made with lower concentration. This is probably due to the different affinity of the PS block to the ambient water and the aggregation of the nanoparticles when the concentration changes. Similar morphological changes were observed for polystyrene-block-poly(ethylene oxide) micelles in N,N-dimethylformamide DMF/water.28 The cross section of an 80 μm thick membrane was observed by SEM (ESI Fig. S3†) showing the presence of a matrix of imperfect interconnected cylinders which may be due to the micelles regular packing and stacking. The membranes were further confirmed by AFM topographies. Nodules with heights of 10∼60 nm which were similar to sphere morphologies protruded above the surface when the polymer concentration was 10 wt% (Fig. 2d). In addition worm-like micelles were observed for the membrane when the polymer concentration reached to 25 wt% which was consistent with the results of the SEM (Fig. 2e).
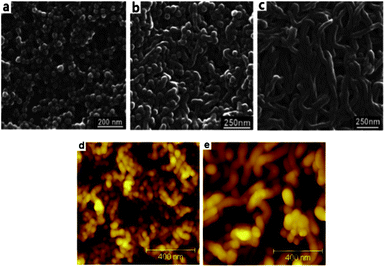 |
| Fig. 2
SEM images of the membrane cast from PS43k-b-P4VP59k DMF solution with different concentrations (a) 10 wt%, (b) 20 wt%, (c) 25 wt% at room temperature. AFM height topographies of the membrane cast from (d) 10 wt% and (e) 25 wt%. | |
We also investigated the effect of the hydrophobic/hydrophilic ratio of copolymer on the morphology. For this purpose two types of polymers were used PS43k-b-P4VP59k and PS120k-b-P4VP25k. The SEM images of the cast membrane showed formation of compact and tiny worm-like micelles when the PS120k-b-P4VP25k copolymer was used (Fig. 3a). The diameter of the cylinders was obviously smaller than that obtained with the PS43k-b-P4VP59k (Fig. 3b). It is known that when the weight fraction of the hydrophilic block of the block copolymer is less than ∼50%, the worm-like micelles that are microns in length and similar in diameter to the spheres are the predominant morphology for a variety of diblock copolymers.29,30 Casting the membrane when the concentration of the polymer is 10% proved to be very fragile which makes it unsuitable for continuous experiments.
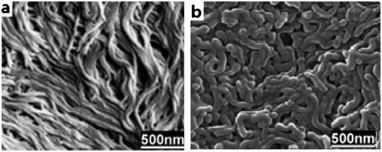 |
| Fig. 3
SEM images of the membrane cast from (a) PS120k-b-P4VP25k and (b) PS43k-b-P4VP59k DMF solution with concentration of 25 wt%. | |
Investigation of morphology dependent Dox release
To investigate the pH responsive behaviour of the membrane in relation to the morphology and concentration, Doxorubicin (Dox) was loaded into the membrane at a loading level of 2.5 wt%, which means that every 2 mg of Dox-loaded membrane contain 50 μg Dox. Release experiments were carried with UV monitoring at a series of pH values (pH 3.0 to 8.0). The membranes were prepared from 25 wt% solutions for both copolymers with different hydrophobic/hydrophilic block ratios. As shown in Fig. 4a the copolymer PS120k-b-P4VP25k (block ratio is 5.6, compact worm-like micelles Fig. 3a), showed a negligible amount of Dox release at all pH values. In contrast, the PS43k-b-P4VP59k copolymer (block ratio is 0.8), showed a typical two-phase-release profile at pH values above 7.0. A relatively rapid release in the first stage was followed by a sustained and slow release over a prolonged time of up to 2 days. Slow sustained release is highly desirable in drug delivery microchip, as these implantable devices are expected to consistently perform for a longer period of time. Five sets of data were collected over 20 h as a proof of concept. At pH 4.0 to 7.0, negligible amounts of Dox were released for the whole duration of the experiment. When the pH value increased to 9.0, about 10 wt% was released after 5 h incubation in buffer solution. The pyridine block of the copolymer, which forms the corona shell of the micelle, is deprotonated at high pH and the pyridine molecules tend to shrink, thus exposing the Dox-loaded PS core to the buffer solution. At the same time, the shrinked corona induces the expansion of gaps between worm-like micelles, leading to the increase of diffusible ability of embedded Dox in the membrane to the buffer solution. However, at low pH value, the pyridine groups are protonated and the blocks extend to minimize charge repulsion. This means that the pyridine molecules spread over each other and form a protective network to prevent the Dox release from the PS core and also prevent released Dox diffused out of the membrane.31 Using the copolymer with higher hydrophobic/hydrophilic ratio leads to a stronger solid hydrophobic core and a compact aggregate with Dox, which prevents release of the encapsulated drug even when the P4VP corona is protonated. This pH-dependent release behaviour is of particular interest in achieving specific site-targeted drug delivery with micelles. While a faster drug release is desired once the micelle particles reach the specific pathological site where pH value is higher than that in the normal tissue. Therefore, this pH sensitive membrane can be used to achieve specific drug delivery relying on the variation in pH values.
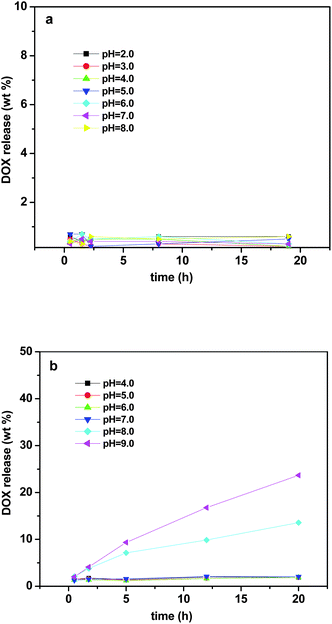 |
| Fig. 4 Dox release profiles of membrane based on (a) PS120k-b-P4VP25k and (b) PS43k-b-P4VP59k at different pH value. | |
Cell viability test
To test the viability and safety of the micellar membrane, cell lines of human origin such THP-1 cells (Human acute monocytic leukemia cell line) were used. A trypan blue exclusion test was used to determine the number of viable cells present in the THP-1 cell suspension after incubation with the cast membrane based on PS43k-b-P4VP59k copolymer. This test is based on the principle that live cells possess intact cell membranes that exclude certain dyes, such as trypan blue, whereas dead cells do not. In this test, 1 mL of cells suspension (1 × 105 mL−1) was simply mixed with dye after incubation with blank cast membrane at different pH values (7.4 (control), and 9.0) for 3 and 24 h, then visually examined to determine whether cells take up or exclude dye under microscopy. As shown in Fig. 5, at both pH 7.4 (the medium itself, same level as physiological environment) and pH 9.0, the cells were active and alive after incubation with blank membrane even for 24 h, indicating the membrane is safe to use in controlled drug release microchips.
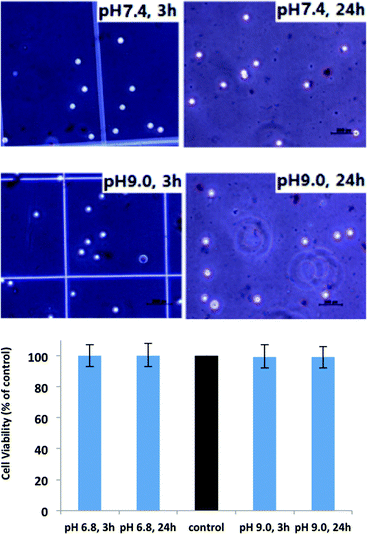 |
| Fig. 5
THP-1
Cell viability of the membrane casted from PS43k-b-P4VP59k incubated in medium with different pH values for 3h and 24 h. | |
Cell uptake study
Confocal microscopy was performed to investigate the cell uptake of Dox released from the Dox-loaded membrane at basic pH. Since Dox itself is fluorescent, it was used directly to measure cellular uptake without additional markers. Therefore, the fluorescence intensity is proportional to the amount of Dox internalized by Fig. 6. As shown in Fig. 6, the red fluorescence was observed in the cytoplasm after incubation for 3 h, and higher concentrations of released Dox was found in cytoplasm after incubation for 5 h (medium pH 9), indicating the consistent release from the membrane as predicted by the drug release profile. It is noted that no fluorescence was observed after the cells were treated with Dox-loaded membrane in control medium (pH 7.4) for 3 h and 5 h, indicating no drug released at this point, or too small an amount of drug to detect was released.
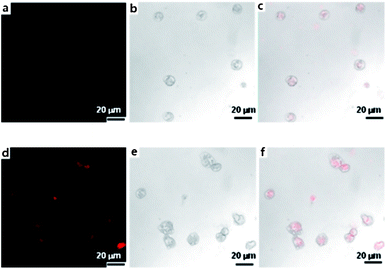 |
| Fig. 6 Confocal images of THP-1 cells after incubating (medium with pH 9.0) with Dox-loaded membrane (PS43k-b-P4VP59k). (a–c) CLSM of Dox fluorescence, DIC and merged images of Dox fluorescence and DIC in THP-1 cells treated with membrane for 3 h, (d–f) for 5 h. Scale bar is 20 μm in all images. | |
Conclusions
In summary, a pH-responsive membrane based on PS-b-P4VP copolymer assembly was developed for controlled drug release. As shown by SEM, in selective solvents the PS-b-P4VP copolymer can form spherical or worm-like micelles with inner cores consisting of styrene block cores and coronas made of the pyridine components. The assembled morphology depends on the concentration and block ratio of the PS/P4VP copolymer. The hydrophobic PS core encapsulates the hydrophobic drug Dox, while the P4VP block can either protect or expose the PS core according to pH of the surrounding medium to control the release of the loaded drug. Cell viability tests revealed that the membrane has no toxicity at a wide range of pH values. It is important to mention that using polystyrene-b-(4-vinylpyridine) membrane for medical devices is not an obvious choice due to toxicities that may be associated with these blocks. However, this polymer was chosen as a safe (Fig. 6), but a non-biodegradable membrane as needed for a delivery microchip with consistent operation. Moreover, the effect of morphology and concentration on the operation of the membrane provides a good reference for designing more biocompatible membranes in the future. The cell uptake study evaluated the membrane did have appropriate drug release at basic pH which was consistent with the results obtained from the drug release profile. This pH-responsive polymer provides a practical, safe and effective solution for the production of reliable membranes for future use in drug delivery microchips.
Acknowledgements
The research presented is fully sponsored by King Abdullah University of Science and Technology (KAUST). The authors thank his excellency minister Ali Ibrahim Al Naimi for his constant support to the research environment at KAUST.
References
-
L. Liu, J. Kost, L. Fishman Marshall and B. Hicks Kevin, New Delivery Systems for Controlled Drug Release from Naturally Occurring Materials, American Chemical Society, 2008, vol. 992, pp. 265–281 Search PubMed.
- S. M. Barnard and D. R. Walt, Science, 1991, 251, 927–929 CAS.
- R. Langer, Science, 2001, 293, 58–59 CrossRef CAS.
- K. E. Uhrich, S. M. Cannizzaro, R. S. Langer and K. M. Shakesheff, Chem. Rev., 1999, 99, 3181–3198 CrossRef CAS.
- A. C. R. Grayson, I. S. Choi, B. M. Tyler, P. P. Wang, H. Brem, M. J. Cima and R. Langer, Nat. Mater., 2003, 2, 767–772 CrossRef CAS.
- Y. W. Li, R. S. Shawgo, B. Tyler, P. T. Henderson, J. S. Vogel, A. Rosenberg, P. B. Storm, R. Langer, H. Brem and M. J. Cima, J. Controlled Release, 2004, 100, 211–219 CrossRef CAS.
- A. Giese, T. Kucinski, U. Knopp, R. Goldbrunner, W. Hamel, H. M. Mehdorn, J. C. Tonn, D. Hilt and M. Westphal, J. Neuro-Oncol., 2004, 66, 351–360 CrossRef.
- G. Y. Kim, B. M. Tyler, M. M. Tupper, J. M. Karp, R. S. Langer, H. Brem and M. J. Cima, J. Controlled Release, 2007, 123, 172–178 CrossRef CAS.
- J. T. Santini, A. C. Richards, R. Scheidt, M. J. Cima and R. Langer, Angew. Chem., Int. Ed., 2000, 39, 2397–2407 Search PubMed.
- S. Park, J.-Y. Wang, B. Kim and T. P. Russell, Nano Lett., 2008, 8, 1667–1672 CrossRef CAS.
- W. Lee, X. Zhang and R. M. Briber, Polymer, 2010, 51, 2376–2382 CrossRef CAS.
- W. van Zoelen, E. Polushkin and G. ten Brinke, Macromolecules, 2008, 41, 8807–8814 CrossRef CAS.
- S. C. Chen, S. W. Kuo, U. S. Jeng, C. J. Su and F. C. Chang, Macromolecules, 2010, 43, 1083–1092 CrossRef CAS.
- H. W. Shen, L. F. Zhang and A. Eisenberg, J. Am. Chem. Soc., 1999, 121, 2728–2740 CrossRef CAS.
- S. Forster, M. Zisenis, E. Wenz and M. Antonietti, J. Chem. Phys., 1996, 104, 9956–9970 CrossRef.
- F. Calderara and G. Riess, Macromol. Chem. Phys., 1996, 197, 2115–2132 CrossRef CAS.
- J. P. Spatz, S. Mossmer and M. Moller, Angew. Chem., Int. Ed. Engl., 1996, 35, 1510–1512 CrossRef CAS.
- J. P. Spatz, S. Sheiko and M. Moller, Macromolecules, 1996, 29, 3220–3226 CrossRef CAS.
- C. J. Clarke, A. Eisenberg, J. LaScala, M. H. Rafailovich, J. Sokolov, Z. Li, S. Qu, D. Nguyen, S. A. Schwarz, Y. Strzhemechny and B. B. Sauer, Macromolecules, 1997, 30, 4184–4188 CrossRef CAS.
- H. Ishiguro, M. C. Steward, R. W. Wilson and R. M. Case, J. Physiol. (London), 1996, 495, 179–191 CAS.
- D. Tuveson and D. Hanahan, Nature, 2011, 471, 316–317 CrossRef CAS.
- J. Y. Zhu, A. Eisenberg and R. B. Lennox, J. Am. Chem. Soc., 1991, 113, 5583–5588 CrossRef CAS.
- K. Kataoka, A. Harada and Y. Nagasaki, Adv. Drug Delivery Rev., 2001, 47, 113–131 CrossRef CAS.
- D. E. Discher and A. Eisenberg, Science, 2002, 297, 967–973 CrossRef CAS.
- T. Smart, H. Lomas, M. Massignani, M. V. Flores-Merino, L. R. Perez and G. Battaglia, Nano Today, 2008, 3, 38–46 CrossRef CAS.
- G. Battaglia and A. J. Ryan, Nat. Mater., 2005, 4, 869–876 CrossRef CAS.
- M. C. Orilall and U. Wiesner, Chem. Soc. Rev., 2011, 40, 520–535 RSC.
- P. Bhargava, Y. Tu, J. X. Zheng, H. Xiong, R. P. Quirk and S. Z. D. Cheng, J. Am. Chem. Soc., 2007, 129, 1113–1121 CrossRef CAS.
- F. Ahmed and D. E. Discher, J. Controlled Release, 2004, 96, 37–53 CrossRef CAS.
- F. H. Meng, C. Hiemstra, G. H. M. Engbers and J. Feijen, Macromolecules, 2003, 36, 3004–3006 CrossRef CAS.
- S. P. Nunes, R. Sougrat, B. Hooghan, D. H. Anjum, A. R. Behzad, L. Zhao, N. Pradeep, I. Pinnau, U. Vainio and K.-V. Peinemann, Macromolecules, 2010, 43, 8079–8085 CrossRef CAS.
Footnote |
† Electronic supplementary information (ESI) available: GPC and 1H NMR characterization of PS43k-b-P4VP59k. Cross-section of the membrane cast from 25 wt% PS43k-b-P4VP59k DMF solution. See DOI: 10.1039/c1py00300c |
|
This journal is © The Royal Society of Chemistry 2011 |
Click here to see how this site uses Cookies. View our privacy policy here.