DOI:
10.1039/C1RA00652E
(Paper)
RSC Adv., 2012,
2, 480-486
(±)-Camphor-10-sulfonic acid catalyzed direct one-pot three-component Mannich type reaction of alkyl (hetero)aryl ketones under solvent-free conditions: application to the synthesis of aminochromans†
Received
30th August 2011
, Accepted 27th September 2011
First published on 10th November 2011
Abstract
Direct three-component Mannich type reactions of (hetero)aromatic ketones with aromatic aldehydes and aromatic amines were efficiently catalyzed by camphor-10-sulfonic acid at ambient temperature under solvent free conditions to afford β-amino ketones in good to excellent yields. Syntheses of aminochromans were accomplished from one of the Mannich adducts via an unprecedented route involving intramolecular etherification with CuI/8-hydroxyquinoline.
Introduction
The Mannich reaction, involving carbon–carbon bond formation between an enolizable ketone (nucleophile) and a Schiff base (electrophile) is an atom-economic process and provides one of the most basic and useful methods for the synthesis of β-amino carbonyl compounds.1 These are important synthetic intermediates for various valuable pharmaceuticals and bio-active natural products.2 The classical Mannich reaction is plagued by drastic reaction conditions, substrate limitations, and long reaction times.3 To overcome the drawbacks of the classical method, the Lewis acid catalyzed condensation between silyl enol ethers or silyl ketene acetals and preformed imines has been developed.4 However, many imines tend to be unstable during purification by chromatography, distillation or prolonged storage. Thus, from a practical point of view, it is desirable to use three component one pot strategy, involving the in situ formation of imine intermediate from aldehydes and amines followed by the reaction with the nucleophile to afford the desired product. However, many Lewis acids cannot be used in Mannich type reactions because they decompose or deactivate in the presence of amines and water produced during the formation of imine. In continuation of our efforts towards the development of newer synthetic methodologies, we report herein an efficient camphorsulfonic acid (CSA) catalyzed one-pot three-component Mannich type reaction of alkyl (hetero)aryl ketones with aldehydes and amines.
Although, most of the reports for direct three component one-pot Mannich reaction deal with acyclic and alicyclic ketones as nucleophiles,5 only a few reports are available involving aromatic ketones as nucleophiles. This may be due to the poor nucleophilicity of aromatic ketones as compared to that of acyclic/alicyclic ketones. To increase the nucleophilicity, silyl enolates of aromatic ketones were used.6 But, there is still a drawback in that the silyl enolates have to be prepared from the corresponding carbonyl compounds under anhydrous conditions. Few catalysts, including H3PW12O40,7HClO4–SiO2,8NbCl5,9BiCl3,10DBSA,11 and SnCl2,12etc. have been developed for direct three component one-pot procedures using aromatic ketones as nucleophiles. However, most of these reported procedures suffer from disadvantages such as use of strong acid, expensive catalysts, toxic reagents, long reaction times, low yields etc. Therefore, there is still a demand for the development of effective protocols for the synthesis of aromatic ketone derived β-amino carbonyl compounds under mild conditions using inexpensive catalysts.
Results and discussion
Currently, there is a growing effort on the development of economical and environmentally benign processes based on the principles of green chemistry. Use of metal free small organic molecules as catalysts has been a strategy to develop green organic reactions. It was therefore, of interest to use CSA as an acid catalyst in Mannich type reaction.
The preliminary reaction on the (±) CSA-catalyzed (10 mol%) two-component Mannich reaction of acetophenone (1a, 1.1 equiv) and N-benzylideneaniline under solvent free conditions got completed in 4 h at ambient temperature to afford the corresponding β-amino ketone (4a) in 90% yield.
Encouraged by this result, the three component one-pot Mannich reaction of 1a, with benzaldehyde (2a) and aniline (3a) was investigated under identical reaction conditions (Table 1). Gratifyingly, the reaction proceeded smoothly to afford the adduct 4a with comparable yield (89%) as that obtained in a two component reaction. In order to find out the optimal catalyst loading, the above three-component reaction was carried out with 2–10 mol% of (±) CSA (Table 1). It appears that 5 mol% of catalyst loading is the suitable choice to get the best result in terms of both the yield and reaction time and the same catalyst loading was used for all subsequent studies. Use of (1S)-(+)-camphor-10-sulfonic acid as catalyst (5 mol%) in the above reaction also afforded 4a in similar yield (90%). However, no chiral induction in 4a was observed. To demonstrate the preparative utility of this protocol, the reaction of 1a with 2a and 3a using 5 mol% of (±) CSA was carried out on 0.1 mol scale under solvent free conditions. To our satisfaction, the reaction proceeded smoothly to afford 4a in 89% isolated yield within 4 h. In the absence of the catalyst 4a was not formed at all and both 1a and N-benzylideneaniline were recovered (Table 1, entry 1).
Entry |
(±) CSA (mol%) |
Time (h) |
% Yielda of 4a |
Isolated yield (with respect to aniline).
1a and N-benzylideneaniline were recovered.
|
1 |
0 |
6 |
—b |
2 |
2 |
5 |
78 |
3 |
5 |
4 |
88 |
4 |
10 |
4 |
89 |
Encouraged by the above result, the efficiency of CSA was compared with some of the reported catalysts in the three-component coupling of 1a, 2a and 3a under identical reaction conditions and the results are summarized in Table 2. Thus, use of BiCl3, NbCl5, HClO4–SiO2, and SnCl2 as catalysts yielded the adduct 4a in 69%, 50%, 68% and 53% yields respectively. On the basis of the above results, it was concluded that CSA is indeed superior to many of the reported catalysts.
Table 2 Screening of catalysts for the three-component reaction of 1a, 2a and 3a at ambient temperature under solvent-free conditions
Entry |
Catalyst (mol%) |
Time (h) |
% Yielda |
Isolated yield (with respect to aniline).
|
1 |
BiCl3 (5.0) |
4.0 |
69.0 |
2 |
NbCl5 (5.0) |
4.0 |
50.0 |
3 |
HClO4–SiO2 (5.0) |
4.0 |
68.0 |
4 |
SnCl2 (5.0) |
4.0 |
53.0 |
5
|
(±)-CSA (5.0) |
4.0 |
88.0 |
In an effort to extend and explore the scope and limitations of the present protocol, different aromatic aldehydes and aromatic amines with both electron-donating and electron-withdrawing groups were selected to undergo one-pot three-component Mannich reaction with (hetero)aromatic ketones under optimized conditions (Scheme 1). The results of this study are summarized in Table 3. In all the cases smooth reaction were observed to afford the β-amino ketones 4b–q in moderate to high yields (Table 3, entries 2–17). However, the reaction time for acetophenone with substituted aromatic aldehydes and substituted aromatic amines were longer as compared to that of benzaldehyde and aniline. In contrast to aromatic ketones, reactions with heterocyclic ketone such as 2-acetyl pyridine (1g) was much faster to yield the adducts in high yields (Table 3, entries 16–17).
Entry |
Ketone
|
Aldehyde
|
Amine
|
Time (h) |
Product |
% Yieldb |
Reaction conditions: 1.2 equiv of ketone, 1.1 equiv of aromatic aldehyde, 1.0 equiv of aromatic amine and 5 mol% of CSA at ambient temperature.
Isolated yield (with respect to aromatic amines).
|
1 |
1a
|
2a
|
3a
|
4 |
4a
|
88 |
2 |
1a
|
2b
|
3a
|
8 |
4b
|
83 |
3 |
1a
|
2c
|
3a
|
8 |
4c
|
75 |
4 |
1a
|
2d
|
3a
|
6 |
4d
|
88 |
5 |
1a
|
2a
|
3b
|
15 |
4e
|
100 |
6 |
1a
|
2b
|
3c
|
24 |
4f
|
42 |
7 |
1a
|
2e
|
3c
|
48 |
4g
|
60 |
8 |
1a
|
2f
|
3c
|
17 |
4h
|
75 |
9 |
1a
|
2g
|
3d
|
24 |
4i
|
82 |
10 |
1b
|
2a
|
3a
|
24 |
4j
|
51 |
11 |
1b
|
2g
|
3d
|
24 |
4k
|
60 |
12 |
1c
|
2a
|
3a
|
20 |
4l
|
37 |
13 |
1d
|
2a
|
3a
|
20 |
4m
|
60 |
14 |
1e
|
2a
|
3a
|
20 |
4n
|
53 |
15 |
1f
|
2a
|
3a
|
20 |
4o
|
62 |
16 |
1g
|
2a
|
3a
|
1 |
4p
|
90 |
17 |
1g
|
2b
|
3a
|
1 |
4q
|
84 |
Oxygen-heterocycles are featured in many natural products and medicinally relevant compounds that display a diverse range of interesting biological activities.13 Among various oxygen-heterocycles, chromans and in particular 4-aminochroman derivatives have attracted considerable attention in recent years as they have been used as modulators of calcium and potassium channels affecting cardiac activity of the blood pressure.14 In view of their pharmaceutical importance, the synthesis of 4-aminochroman skeleton has become an interesting and challenging task for synthetic organic chemists.15
Earlier, Buchwald and co-workers demonstrated an elegant protocol for carbon-oxygen bond forming reaction between aryl bromides/iodides and aliphatic alcohols to produce aromatic ethers using CuI/tetramethyl-1,10-phenanthroline combination as catalyst.16 However, the reactivity of aryl bromides in this system was more poor than their iodide counterparts. Subsequently, Hu et al.17 reported an alternate protocol using CuI/8- hydroxyquinoline as catalyst for carbon-oxygen bond forming reaction between alcohols and aryl bromides. Keeping this in view, we felt that the Mannich adduct derived bromo substituted β-aminoalcohol in principle should undergo intramolecular etherification to afford 4-aminochroman skeleton. To this end, the Mannich adduct 4h was reduced with NaBH4 to yield a mixture of syn- and anti-isomers of bromo substituted β-aminoalcohol (5a and 5b) which were separated by column chromatography. To our delight, both 5a and 5b underwent intramolecular etherification using CuI/8-hydroxyquinoline combination as catalyst affording 4-aminochromans 6a and 6b respectively albeit in moderate yields (Scheme 2). As the relative stereochemistry of 6a and 6b could not be ascertained from their 1H NMR spectral data, we opted for XRD spectra to determine the relative stereochemistry between C(2) and C(4). Although suitable single crystals of 6a were obtained, we failed to get good quality crystals of 6b. Single crystal XRD data of 6a revealed its cis-geometry [(2R*,4R*)-3,4-dihydro-N-(4-methoxyphenyl)-4-amino-2-phenyl-2H-chromene] (Fig. 1).
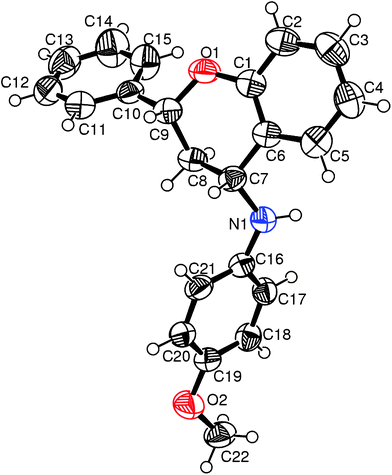 |
| Fig. 1 ORTEP diagram of compound 6a. | |
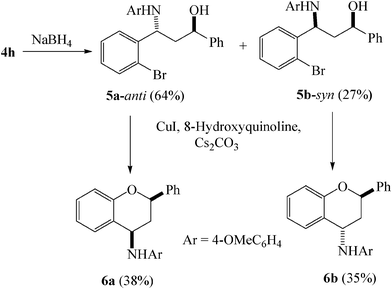 |
| Scheme 2 Application of the Mannich adduct in the synthesis of O-heterocycles. | |
On the basis of the XRD data of 6a, compound 6b was designated as trans-isomer [(2R*,4S*)-3,4-dihydro-N-(4-methoxyphenyl)-4-amino-2-phenyl-2H-chromene]. Accordingly compounds 5a and 5b were designated as anti-[(1R*,3R*)-3-(4-methoxyphenylamino)-3-(2-bromophenyl)-1-phenyl-propan-1-ol] and syn-isomer [(1R*,3S*)-3-(4-methoxyphenylamnio)-3-(2-bromophenyl)-1-phenyl-propan-1-ol] respectively. Thus a single Mannich adduct could be successfully converted to two geometrical isomers of 4-aminochromans. It is important to mention that although various catalysts have been developed and successfully used for Mannich type reaction so far, there is no report on the utilization of the aromatic ketone derived Mannich adduct in the synthesis of O-heterocycles. So far, the only intramolecular route for the synthesis of 4-aminochroman by Samanta et al. involved indium-mediated cyclization of chiral hydrazones.15d
Conclusion
In conclusion, we have developed a very simple, efficient and practical method for the one-pot three-component Mannich reaction of (hetero)aromatic ketones with aromatic aldehydes and aromatic amines using (±)-camphor-10-sulfonic acid as catalyst. The significant features of the protocol include solvent-free conditions, inexpensive catalyst, mild reaction conditions, easy work up and high yields of the products which make it a valuable contribution for the synthesis of β-amino carbonyl compounds. As an application, the bromo substituted β-amino ketone (derived from acetophenone and 2-bromobenzaldehyde and 4-methoxy aniline) was first reduced to the corresponding bromo benzylic alcohols which were subsequently converted into 4-aminochromans via intramolecular etherification with CuI/8-hydroxyquinoline. Application of this reaction to the preparation of biologically relevant compounds is currently underway.
Experimental
General information
Melting points were determined using Fisher-Johns melting point apparatus and are uncorrected. IR spectra were scanned with a Jasco FT IR 4100 spectrophotometer. The 1H and 13C NMR spectra were recorded with a Bruker AC 200 spectrometer. Spectra were referenced to residual chloroform (δ 7.25 ppm, 1H; 77.0 ppm, 13C). Low resolution mass spectra were recorded with a Varian 500 mass spectrometer (ESI). High resolution mass spectra were recorded with a Q-TOF (YA-105) micromass spectrometer (ESI, Ar). All reactions were carried out under an argon atmosphere. (±)-Camphor-10-sulfonic acid (CSA) was purchased from Aldrich, USA and was used as such. Spectral data of known compounds was in accordance with those reported in the literature while the unknown compounds were characterized by IR, NMR and HRMS data.
To a mixture of 1a (1.32 g, 11 mmol) and N-benzylideneaniline (1.81 g, 10 mmol), (±)-CSA (0.23 g, 10 mol%) was added and stirred at ambient temperature. After completion of the reaction (monitored by TLC), the thick mass was quenched with 10% aqueous NaHCO3 solution. The pale yellow solid was filtered, washed with water and dried in air. Recrystallization of the crude product from ethyl acetate-hexane mixture yielded pure adduct 4a as a colorless solid in 90% yield.
Colorless solid; mp 169–170 °C (lit.18 169–170 °C); IR (CHCl3): υ 3384, 3019, 2399, 1671, 1600, 1509, 1449, 1417, 1291, 1214, 1077, 1002, 856, 756, 668 cm−1; 1H NMR (200 MHz, CDCl3): δ 3.63 (d, J = 6.5 Hz, 2H), 5.02 (t, J = 6.5 Hz, 1H), 6.68–6.80 (m, 3H), 7.07–7.15 (m, 2H), 7.22–7.55 (m, 8H), 7.89 (d, J = 8.0 Hz, 2H); 13C NMR (50 MHz, CDCl3): δ 46.0, 55.3, 114.4, 118.4, 126.5, 127.4, 128.2, 128.7, 128.8, 129.1, 133.4, 136.6, 142.4, 146.2, 198.1.
General procedure for one-pot three-component Mannich reaction under solvent-free conditions
To a mixture of aniline/substituted aniline (5 mmol), benzaldehyde/substituted benzaldehyde (5.5 mmol) and methyl (hetero)aryl ketone (6 mmol), (±)-CSA (0.06 g, 0.25 mmol) was added and the mixture was stirred at ambient temperature. After completion of the reaction (disappearance of aromatic amine; monitored by TLC), the thick mass was quenched with 10% aqueous NaHCO3 solution, extracted with ethyl acetate, washed with water, brine and dried (Na2SO4). Removal of solvent yielded the crude product which was purified by crystallization/column chromatography over silica gel to give the pure adducts 4b–q. The identity and purity of the products are confirmed by IR, 1H NMR, 13C NMR, and HRMS (for unknown compounds) spectroscopic analysis.
Colorless solid; 83% yield; mp 128–129 °C (lit.8 130–131 °C); IR (CHCl3): υ 3394, 3019, 1681, 1601, 1504, 1448, 1214, 1010, 755, 695, 666 cm−1; 1H NMR (200 MHz, CDCl3): δ 3.51 (d, J = 6.2 Hz, 2H), 4.96 (t, J = 6.2 Hz, 1H), 6.60 (d, J = 7.8 Hz, 2H), 6.77 (t, J = 7.3 Hz, 1H), 7.12 (t, J = 7.6 Hz, 2H), 7.30–7.53 (m, 7H), 7.88 (d, J = 7.4 Hz, 2H); 13C NMR (50 MHz, CDCl3): δ 45.6, 55.2, 114.9, 119.2, 121.3, 128.1, 128.5, 128.7, 129.2, 131.9, 133.6, 136.5, 141.1, 145.4, 197.6; MS (ESI) m/z 381 (M + 2, 71), 379 (M, 98), 354 (13), 325 (13), 283 (22), 281 (13), 265 (13), 262 (86), 260 (100), 150 (20), 138 (27), 122 (20), 116 (37), 105 (76).
Colorless solid; 75% yield; mp 115–116 °C (lit.18 117–118 °C); IR (CHCl3): υ 3399, 3019, 2399, 1682, 1601, 1504, 1448, 1407, 1215, 1013, 825, 756, 690 cm−1; 1H NMR (200 MHz, CDCl3): δ 3.56 (d, J = 6.4 Hz, 2H), 5.00 (t, J = 6.4 Hz, 1H), 6.65 (d, J = 7.9 Hz, 2H), 6.77 (t, J = 7.3 Hz, 1H), 7.13 (t, J = 7.8 Hz, 2H), 7.26–7.62 (m, 7H), 7.87–7.91 (m, 2H); 13C NMR (50 MHz, CDCl3): δ 46.0, 54.3, 114.0, 118.1, 127.8, 128.1, 128.7, 128.9, 129.1, 133.0, 133.4, 136.7, 141.5, 146.7, 197.8; MS (ESI) m/z 337 (M + 2, 23), 335 (M, 58), 218 (34), 217 (21), 216 (100), 105 (37).
3-(3-Methoxyphenyl)-1-phenyl-3-(phenylamino)propan-1-one (4d)
Colorless solid; 88% yield; mp 106–107 °C; IR (CHCl3): υ 3406, 3017, 2938, 2835, 1683, 1601, 1505, 1465, 1449, 1435, 1216, 1181, 1046, 871, 692 cm−1; 1H NMR (200 MHz, CDCl3): δ 3.47–3.54 (m, 2H), 3.78 (s, 3H), 4.99 (t, J = 6.9 Hz, 1H), 6.61–6.81 (m, 4H), 7.03–7.29 (m, 5H), 7.41–7.58 (m, 3H), 7.92 (d, J = 7.3 Hz, 2H); 13C NMR (50 MHz, CDCl3): δ 46.1, 55.0, 55.1, 112.3, 112.6, 114.1, 118.0, 118.7, 128.1, 128.6, 129.0, 129.7, 133.3, 136.8, 144.6, 146.8, 160.0, 198.1; MS (ESI) m/z 331 (M, 46), 212 (100), 105 (23); HRMS: m/z calcd for C22H22NO2 (M + H): 332.1651; found: 332.1645.
Colorless solid; 100% yield; mp 180–181 °C; IR (CHCl3): υ 3368, 3019, 1665, 1593, 1576, 1504, 1447, 1407, 1312, 1283, 1070, 916, 848 cm−1; 1H NMR (200 MHz, CDCl3): δ 3.65 (d, J = 6.2 Hz, 2H), 4.97 (t, J = 6.2 Hz, 1H), 6.61 (d, J = 8.6 Hz, 2H), 7.18–7.61 (m, 10H), 7.88 (d, J = 7.5 Hz, 2H); 13C NMR (50 MHz, CD3COCD3): δ 47.3, 54.7, 116.0, 127.5, 127.8, 128.9, 129.3, 129.4, 132.2, 133.8, 138.1, 144.4, 148.1, 197.7; MS (ESI) m/z 381 (M + 2, 32), 380 (M + 1, 23), 379 (M, 35), 311 (16), 262 (85), 261 (19), 260 (100), 105 (65); HRMS: m/z calcd for C21H19NOBr (M + H): 380.0650; found: 380.0648.
3-(4-Methoxyphenylamino)-3-(4-bromophenyl)-1-phenylpropan-1-one (4f)
Colorless solid; 42% yield; mp 114–115 °C; IR (CHCl3): υ 3389, 3061, 3013, 2951, 2832, 1904, 1682, 1602, 1510, 1485, 1448, 1330, 1298, 1234, 1178, 1071, 1036, 818, 763, 689 cm−1; 1H NMR (200 MHz, CDCl3): δ 3.45–3.49 (m, 2H), 3.69 (s, 3H), 4.89 (t, J = 6.5 Hz, 1H), 6.52–6.58 (m, 2H), 6.63–6.73 (m, 2H), 7.30–7.37 (m, 2H), 7.40–7.48 (m, 4H), 7.53–7.61 (m, 1H), 7.87–7.90 (m, 2H); 13C NMR (50 MHz, CDCl3): δ 45.9, 54.8, 55.4, 114.6, 115.2, 120.7, 127.9, 128.1, 128.4, 131.5, 133.2, 136.4, 140.7, 142.2, 152.3, 197.6; MS (ESI) m/z 411 (M + 2, 74), 410 (M + 1, 77), 409 (M, 100), 292 (23), 290 (27), 105 (14); HRMS: m/z calcd for C22H21NO2Br (M + H): 410.0756; found: 410.0752.
3-(4-Methoxyphenylamino)-3-(3-nitrophenyl)-1-phenylpropan-1-one (4g)
Colorless solid; 60% yield; mp 76–77 °C; IR (CHCl3): υ 3394, 3014, 2934, 2832, 1681, 1596, 1530, 1448, 1351, 1239, 1099, 1035, 821, 760, 686 cm−1; 1H NMR (200 MHz, CDCl3): δ 3.50 (d, J = 6.1 Hz, 2H), 3.68 (s, 3H), 4.08 (br, 1H), 5.05 (t, J = 6.1 Hz, 1H), 6.52 (d, J = 8.9 Hz, 2H), 6.70 (d, J = 9.0 Hz, 2H), 7.39–7.60 (m, 4H), 7.79–7.91 (m, 3H), 8.04–8.08 (m, 1H), 8.32 (s, 1H); 13C NMR (50 MHz, CDCl3): δ 45.8, 54.8, 55.5, 114.7, 115.4, 121.5, 122.3, 128.0, 128.7, 129.6, 133.0, 133.6, 136.3, 140.3, 145.6, 148.5, 152.6, 197.3; MS (ESI) m/z 377 (M + 1, 65), 376 (M, 100), 273 (13), 257 (61), 210 (14), 164 (12), 105 (24); HRMS: m/z calcd for C22H21N2O4 (M + H): 377.1501; found: 377.1505.
3-(4-Methoxyphenylamino)-3-(2-bromophenyl)-1-phenylpropan-1-one (4h)
Colorless solid; 75% yield; mp 60–61 °C; IR (CHCl3): υ 3396, 3015, 2933, 2832, 2400, 1966, 1682, 1596, 1512, 1463, 1440, 1291, 1216, 1180, 1098, 819, 761, 689 cm−1; 1H NMR (200 MHz, CDCl3): δ 3.42–3.54 (m, 1H), 3.69 (s, 3H), 3.74–3.85 (m, 1H), 5.28–5.34 (m, 1H), 6.58–6.72 (m, 4H), 7.07–7.14 (m, 1H), 7.22–7.30 (m, 1H), 7.40–7.47 (m, 2H), 7.53–7.60 (m, 2H),7.68–7.72 (m, 1H), 7.95 (d, J = 7.5 Hz, 2H); 13C NMR (50 MHz, CDCl3): δ 43.9, 54.9, 55.5, 114.7, 115.0, 122.6, 127.9, 128.2, 128.4, 128.5, 128.7, 133.0, 133.3, 136.6, 140.6, 141.3, 152.4, 198.3; MS (ESI) m/z 412 (M + 3, 65), 411 (M + 2, 37), 410 (M + 1, 65), 357 (17), 326 (18), 293 (16), 292 (95), 291 (20), 290 (100), 105 (12); HRMS: m/z calcd for C22H21NO2Br (M + H): 410.0756; found: 410.0748.
3-(4-Chlorophenylamino)-3-(4-nitrophenyl)-1-phenylpropan-1-one (4i)
Yellow solid; 82% yield; mp 127–128 °C (lit.12 129–131 °C); IR (CHCl3): υ 3394, 3019, 2927, 1679, 1598, 1495, 1348, 1214, 1180, 1093, 855, 754, 688 cm−1; 1H NMR (200 MHz, CDCl3): δ 3.52 (d, J = 6.0 Hz, 2H), 5.05 (t, J = 6.0 Hz, 1H), 6.46 (d, J = 8.6 Hz, 2H), 7.04 (d, J = 8.6 Hz, 2H), 7.41–7.48 (m, 2H), 7.58–7.62 (m, 3H), 7.88 (d, J = 7.3 Hz, 2H), 8.16 (d, J = 8.5 Hz, 2H); 13C NMR (50 MHz, CDCl3): δ 45.4, 54.0, 114.8, 122.8, 123.9, 127.3, 128.0, 128.7, 128.9, 133.7, 136.1, 144.9, 147.0, 150.2, 197.0; MS (ESI) m/z 383 (M + 3, 26), 382 (M + 2, 29), 381 (M + 1, 58), 380 (M, 75), 292 (12), 286 (8), 263 (49), 262 (17), 261 (100), 105 (9).
1-(4-Chlorophenyl)-3-phenyl-3-(phenylamino)propan-1-one (4j)
Colorless solid; 51% yield; mp 120–121 °C (lit.18 119–120 °C); IR (CHCl3): υ 3377, 3019, 2922, 2399, 1918, 1683, 1601, 1589, 1504, 1431, 1358, 1215, 1177, 1094, 832, 755, 699 cm−1; 1H NMR (200 MHz, CDCl3): δ 3.55–3.58 (m, 2H), 5.02 (t, J = 6.5 Hz, 1H), 6.67–6.81 (m, 3H), 7.10–7.17 (m, 2H), 7.24–7.47 (m, 7H), 7.80 (d, J = 8.4 Hz, 2H); 13C NMR (50 MHz, CDCl3): δ 46.0, 55.2, 114.2, 118.3, 126.4, 127.5, 128.8, 129.0, 129.1, 129.5, 135.2, 139.9, 142.5, 146.5, 197.0; MS (ESI) m/z 337 (M + 2, 11), 335 (M, 39), 320 (25), 319 (14), 318 (72), 212 (26), 183 (13), 182 (100), 141 (10), 139 (20).
3-(4-Chlorophenylamino)-1-(4-chlorophenyl)-3-(4-nitrophenyl)propan-1-one (4k)
Yellow solid; 60% yield; mp 125–126 °C; IR (CHCl3): υ 3396, 3019, 2908, 2850, 1671, 1590, 1402, 1348, 1215, 1178, 856, 700 cm−1; 1H NMR (200 MHz, CDCl3): δ 3.51 (m, 2H), 5.05 (t, J = 6.2 Hz, 1H), 6.48 (d, J = 8.7 Hz, 2H), 7.06 (d, J = 8.7 Hz, 2H), 7.41–7.45 (m, 2H), 7.61 (d, J = 8.7 Hz, 2H), 7.80–7.84 (m, 2H), 8.18 (d, J = 8.7 Hz, 2H); 13C NMR (50 MHz, CDCl3): δ 45.3, 54.5, 115.3, 123.7, 124.1, 127.5, 129.2, 129.5, 134.6, 140.4, 144.5, 147.4, 149.7, 195.8; HRMS: m/z calcd for C21H17N2O3Cl2 (M + H): 415.0616; found: 415.0618.
1-(4-Fluorophenyl)-3-phenyl-3-(phenylamino)propan-1-one (4l)
Colorless solid; 37% yield; mp 119–120 °C; IR (CHCl3): υ 3399, 3015, 2956, 2925, 2855, 1684, 1600, 1505, 1409, 1358, 1215, 1156, 839, 758 cm−1; 1H NMR (200 MHz, CDCl3): δ 3.49 (d, J = 6.4 Hz, 2H), 5.00 (t, J = 6.4 Hz, 1H), 6.59–6.74 (m, 3H), 7.05–7.19 (m, 4H), 7.23–7.35 (m, 3H), 7.44 (d, J = 7.0 Hz, 2H), 7.88–7.95 (m, 2H); 13C NMR (50 MHz, CDCl3): δ 46.0, 54.9, 113.9, 115.5, 115.9, 117.9, 126.3, 127.4, 128.7, 129.1, 130.7, 130.9, 133.2, 142.7, 146.8, 163.3, 168.3, 196.5; MS (ESI) m/z 320 (M + 1, 86), 302 (26), 285 (12), 183 (16), 182 (100), 123 (48); HRMS: m/z calcd for C21H19NOF (M + H): 320.1451; found: 320.1449.
1-(4-Methoxyphenyl)-3-phenyl-3-(phenylamino)propan-1-one (4m)
Colorless solid; 60% yield; mp 124–125 °C; IR (CHCl3): υ 3380, 3021, 2921, 1658, 1600, 1511, 1454, 1407, 1215, 1028, 833, 746, 688 cm−1; 1H NMR (200 MHz, CDCl3): δ 3.40–3.62 (m, 2H), 3.87 (s, 3H), 4.98 (t, J = 6.8 Hz, 1H), 6.64–6.77 (m, 3H), 6.91(d, J = 8.9 Hz, 2H), 7.07–7.16 (m, 2H), 7.23–7.36 (m, 3H), 7.44–7.48 (m, 2H), 7.87–7.91 (d, J = 8.9 Hz, 2H); 13C NMR (50 MHz, CDCl3): δ 45.9, 55.3, 55.4, 113.9, 114.1, 117.9, 126.4, 127.2, 128.7, 129.0, 130.0, 130.5, 143.0, 146.9, 163.8, 196.7; HRMS: m/z calcd for C22H22NO2 (M + H): 332.1651; found: 332.1637.
1-(3-Methoxyphenyl)-3-phenyl-3-(phenylamino)propan-1-one (4n)
Colorless solid; 53% yield; mp 104-105 °C; IR (CHCl3): υ 3398, 3020, 2835, 1681, 1600, 1504, 1452, 1428, 1267, 1216, 1042, 751, 692 cm−1; 1H NMR (200 MHz, CDCl3): δ 3.45–3.66 (m, 2H), 3.83 (s, 3H), 5.02 (t, J = 6.7 Hz, 1H), 6.64–6.78 (m, 3H), 7.08–7.16 (m, 3H), 7.24–7.38 (m, 4H), 7.44–7.49 (m, 4H); 13C NMR (50 MHz, CDCl3): δ 46.2, 55.2, 55.4, 112.5, 114.1, 118.1, 119.9, 120.8, 126.4, 127.4, 128.8, 129.1, 129.6, 138.2, 142.8, 146.7, 160.0, 198.0; HRMS: m/z calcd for C22H22NO2 (M + H): 332.1651; found: 332.1638.
1-(2-Methoxyphenyl)-3-phenyl-3-(phenylamino)propan-1-one (4o)
Colorless solid; 62% yield; mp 105–106 °C; IR (CHCl3): υ 3419, 3018, 2840, 1670, 1599, 1505, 1455, 1436, 1295, 1215, 1024, 755, 668 cm−1; 1H NMR (200 MHz, CDCl3): δ 3.57–3.66 (m, 2H), 3.91 (s, 3H), 4.93–5.00 (m, 1H), 6.61–6.75 (m, 3H), 6.94–7.01 (m, 2H), 7.06–7.13 (m, 2H), 7.19–7.35 (m, 3H), 7.43–7.52 (m, 3H), 7.63 (dd, J = 7.9, 2.0 Hz, 1H); 13C NMR (50 MHz, CDCl3): δ 51.3, 55.2, 55.5, 111.6, 113.8, 117.6, 120.9, 126.4, 127.0, 128.3, 128.6, 129.0, 130.5, 133.7, 143.3, 147.1, 158.4, 200.5; HRMS: m/z calcd for C22H22NO2 (M + H): 332.1651; found: 332.1638.
3-Phenyl-3-(phenylamino)-1-(pyridin-2-yl)propan-1-one (4p)
Colorless solid; 90% yield; mp 120–121 °C; IR (CHCl3): υ 3394, 3374, 3019, 2923, 1688, 1602, 1511, 1495, 1318, 1215, 1078, 994, 863 cm−1; 1H NMR (200 MHz, CDCl3): δ 3.62–3.94 (m, 2H), 5.02 (dd, J = 8.4, 5.2 Hz, 1H), 6.56–6.71 (m, 3H), 7.07 (t, J = 7.6 Hz, 2H), 7.18–7.34 (m, 3H), 7.45–7.51 (m, 3H), 7.78–7.88 (m, 1H), 8.02 (d, J = 7.9 Hz, 1H), 8.71 (d, J = 4.6 Hz, 1H); 13C NMR (50 MHz, CDCl3): δ 45.7, 55.4, 113.6, 117.4, 122.1, 126.4, 127.1, 127.2, 128.6, 128.9, 136.9, 143.1, 146.9, 148.8, 153.3, 199.7; HRMS: m/z calcd for C20H19N2O (M + H): 303.1497; found: 303.1484.
3-(4-Bromophenyl)-3-(phenylamino)-1-(pyridin-2-yl)propan-1-one (4q)
Colorless solid; 84% yield; mp 116–117 °C; IR (CHCl3): υ 3395, 3019, 2399, 2332, 1696, 1602, 1506, 1486, 1315, 1215, 1071, 995, 876 cm−1; 1H NMR (200 MHz, CDCl3): δ 3.58–3.91 (m, 2H), 4.96 (dd, J = 8.2, 5.2 Hz, 1H), 6.55 (d, J = 7.9 Hz, 2H), 6.69 (t, J = 7.6 Hz, 1H), 7.04–7.17 (m, 2H), 7.34–7.52 (m, 5H), 7.79–7.87 (m, 1H), 8.01 (d, J = 7.7 Hz, 1H), 8.70 (d, J = 4.3 Hz, 1H); 13C NMR (50 MHz, CDCl3): δ 45.5, 54.9, 113.6, 117.7, 120.8, 122.2, 127.3, 128.2, 129.0, 131.7, 137.0, 142.2, 146.6, 148.8, 153.1, 199.3; MS (ESI) m/z 382 (M + 2, 43), 381 (M + 1, 97), 380 (M, 72), 365 (19), 363 (15), 290 (66), 288 (55), 272 (65), 263 (23), 262 (84), 260 (100), 235 (13), 225 (16), 197 (28), 124 (23), 122 (81); HRMS: m/z calcd for C20H18N2OBr (M + H): 381.0602; found: 381.0600.
Typical procedure for the syntheses of β-aminoalcohols 5a and 5b from 4h
To a stirred solution of 4h (4.87 g, 11.9 mmol) in dry methanol (25 ml) at 0–5 °C was added NaBH4 (0.45 g, 11.9 mmol) portion wise. After stirring for 2.5 h at 0 °C, the reaction mixture was quenched with saturated aqueous NH4Cl solution. Methanol was removed under vacuo, extracted with ethyl acetate, washed with water, brine and dried (Na2SO4). Removal of solvent yielded the crude product, which was purified by silica gel column chromatography to afford pure β-aminoalcohols 5a (64% yield) and 5b (27% yield).
(1R*,3R*)-3-(4-Methoxyphenylamino)-3-(2-bromophenyl)-1-phenyl-propan-1-ol (anti-isomer) (5a): yellow oil; IR (neat): υ 3427, 3061, 3028, 2830, 1882, 1809, 1619, 1514, 1454, 1232, 1024, 913 cm−1; 1H NMR (200 MHz, CDCl3): δ 2.21–2.28 (m, 2H), 3.68 (s, 3H), 4.93–5.01 (m, 2H), 6.49 (d, J = 8.9 Hz, 2H), 6.67 (d, J = 8.9 Hz, 2H), 7.03–7.10 (m, 1H), 7.19–7.35 (m, 6H), 7.47–7.52 (m, 2H); 13C NMR (50 MHz, CDCl3): δ 43.8, 55.5, 71.9, 114.7, 114.8, 122.7, 125.6, 127.3, 127.5, 127.8, 128.3, 132.9, 140.6, 141.8, 144.0, 152.0; HRMS: m/z calcd for C22H23NO2Br (M + H): 412.0912; found: 412.0906.
(1R*,3S*)-3-(4-Methoxyphenylamino)-3-(2-bromophenyl)-1-phenyl-propan-1-ol (syn-isomer) (5b) yellow oil; IR (neat): υ 3378, 3062, 2929, 1618, 1604, 1566, 1513, 1463, 1237, 1036, 914, 754 cm−1; 1H NMR (200 MHz, CDCl3): δ 2.00–2.19 (m, 2H), 3.70 (s, 3H), 4.92 (dd, J = 9.7, 3.1 Hz, 1H), 5.06 (dd, J = 8.8, 3.4 Hz, 1H), 6.51 (d, J = 8.8 Hz, 2H), 6.71 (d, J = 8.8 Hz, 2H), 7.01–7.09 (m, 1H), 7.18–7.43 (m, 7H), 7.52 (d, J = 7.9 Hz, 1H); 13C NMR (50 MHz, CDCl3): δ 45.7, 55.5, 58.2, 74.3, 114.7, 115.4, 122.7, 125.5, 127.4, 127.6, 128.3, 132.8, 140.7, 142.3, 144.2, 152.4; HRMS: m/z calcd for C22H23NO2Br (M + H): 412.0912; found: 412.0910.
Typical procedure for the synthesis of 4-aminochroman 6a from β-aminoalcohol 5a
To a mixture of CuI (0.02 g, 0.1 mmol), 8-hydroxyquinoline (0.03 g, 0.2 mmol) and Cs2CO3 (1.30 g, 4 mmol) at ambient temperature under argon, was added a solution of compound 5a (0.82 g, 2 mmol) in dry toluene (5 ml). The reaction mixture was further refluxed for 24 h when TLC showed the total consumption of 5a. The reaction mixture was brought to room temperature and diluted with dichloromethane (20 ml). The brown slurry was passed through Celite pad to remove the inorganic salts. Dichloromethane was removed under vacuo and the crude residue was purified by silica gel column chromatography to afford pure 4-aminochroman 6a in 38% yield.
(2R*,4R*)-3,4-Dihydro-N-(4-methoxyphenyl)-4-amino-2-phenyl-2H-chromene (6a).
Colorless solid; mp 118–119 °C; IR (CHCl3): υ 3399, 3064, 2922, 1609, 1579, 1511, 1484, 1232, 1035, 916, 759, 698 cm−1; 1H NMR (200 MHz, CDCl3): δ 1.93–2.17 (m, 1H), 2.61 (ddd, J = 13.4, 5.8, 1.9 Hz, 1H), 3.75 (s, 3H), 4.95 (dd, J = 10.7, 5.8 Hz, 1H), 5.20 (dd, J = 11.3, 1.9 Hz, 1H), 6.70–6.82 (m, 4H), 6.91–6.98 (m, 2H), 7.18–7.22 (m, 1H), 7.31–7.43 (m, 5H), 7.66 (d, J = 7.6 Hz, 1H); 13C NMR (50 MHz, CDCl3): δ 37.3, 50.4, 55.8, 77.5, 115.1, 115.3, 116.9, 120.9, 124.7, 126.0, 127.5, 128.0, 128.6, 128.8, 140.8, 141.0, 152.7, 155.1; HRMS: m/z calcd for C22H22NO2 (M + H): 332.1651; found: 332.1635.
(2R*,4S*)-3,4-Dihydro-N-(4-methoxyphenyl)-4-amino-2-phenyl-2H-chromene (6b).
Viscous oil; 35% yield; IR (CHCl3): υ 3402, 3013, 2916, 1608, 1509, 1460, 1218, 1034, 769 cm−1; 1H NMR (200 MHz, CDCl3): δ 2.06–2.21 (m, 1H), 2.41–2.49 (m, 1H), 3.78 (s, 3H), 4.55–4.58 (m, 1H), 5.22 (dd, J = 11.5, 1.8 Hz, 1H), 6.72 (d, J = 8.8 Hz, 2H), 6.84 (d, J = 8.8 Hz, 2H), 6.96–7.02 (m, 2H), 7.22–7.45 (m, 7H); 13C NMR (50 MHz, CDCl3): δ 35.4, 49.0, 55.8, 73.6, 115.2, 117.4, 120.8, 122.2, 126.2, 127.9, 128.5, 129.4, 130.5, 141.0, 153.0, 155.2; HRMS: m/z calcd for C22H22NO2 (M + H): 332.1651; found: 332.1645.
Single crystal X-ray structural studies of 6a were performed on a CCD Oxford Diffraction XCALIBUR-S diffractometer. Data were collected at 293(2) K using graphite-monochromated Mo-Kα radiation (λα = 0.71073 Å). The structure was solved by direct methods using SHELXS-97 and refined by full matrix least-squares with SHELXL-97, refining on F2. The positions of all the atoms were obtained by direct methods. All non-hydrogen atoms were refined anisotropically. Formula: C22H21NO2, M = 331.40, colorless crystals, crystal size: 0.32 × 0.28 × 0.23 mm3, orthorhombic, space group: Pcab, cell parameters: a = 8.5808(7) Å, b = 46.211(4) Å, c = 8.7745 Å, α = 90°, β = 90°, γ = 90°, V = 3479.3(5) Å3, ρcalcd = 1.265 mg m−3, μ = 0.081 mm−1, Z = 8, of 24296 reflections, 3063 were unique. CCDC-828034 contains the supplementary crystallographic data (excluding structure factors) for the structure in this paper. Copies of these data can be obtained, free of charge from The Cambridge Crystallographic Data Centre via www.ccdc.cam.ac.uk/data_request/cif.
References
-
(a) M. Arend, B. Westermann and N. Risch, Angew. Chem., Int. Ed., 1998, 37, 1044 CrossRef;
(b) S. Kobayashi and H. Ishitani, Chem. Rev., 1999, 99, 1069 CrossRef CAS;
(c) A. Cordova, Acc. Chem. Res., 2004, 37, 102 CrossRef CAS;
(d) D. J. Hart and D.-C. Ha, Chem. Rev., 1989, 89, 1447 CrossRef CAS;
(e) K. Juhl, N. Gathergood and K. A. Jorgensen, Angew. Chem., Int. Ed., 2001, 40, 2995 CrossRef CAS;
(f)
E. F. Kleinman, in Comprehensive Organic Synthesis, ed. B. M. Trost and I. Fleming, Pergamon, New York, 1991, 2, ch 4.1, pp. 893 Search PubMed.
-
(a) R. Müller, H. Goesmann and H. Waldmann, Angew. Chem., Int. Ed., 1999, 38, 184 CrossRef;
(b) M. Suginome, L. Uehlin and M. Murakami, J. Am. Chem. Soc., 2004, 126, 13196 CrossRef CAS;
(c) W. Notz, F. Tanaka, S.-I. Watanabe, N. S. Chowdari, J. M. Turner, R. Thayumanvan and C. F. Barbas, J. Org. Chem., 2003, 68, 9624 CrossRef CAS;
(d) N. S. Joshi, L. R. Whitaker and M. B. Francis, J. Am. Chem. Soc., 2004, 126, 15942 CrossRef CAS;
(e) G. B. Evans, R. H. Furneaux, P. C. Tyler and V. L. Schramm, Org. Lett., 2003, 5, 3639 CrossRef CAS;
(f) M. Liu and M. P. Sibi, Tetrahedron, 2002, 58, 7991 CrossRef CAS.
-
R. A. Volkmann, in Comprehensive Organic Synthesis, ed. B. M. Trost and I. Fleming, Pergamon, New York, 1991, Vol. 1, pp. 355 Search PubMedand references cited therein..
-
(a) T.-P. Loh, S. B. K. W. Liung, K.-L. Tan and L.-L. Wei, Tetrahedron, 2000, 56, 3227 CrossRef CAS;
(b) K. Manabe, Y. Mori and S. Kobayashi, Tetrahedron, 2001, 57, 2537 CrossRef CAS.
-
(a) R. I. Kureshy, S. Agrawal, S. Saravanan, N. H. Khan, A. K. Shah, S. H. R. Abdi, H. C. Bajaj and E. Suresh, Tetrahedron Lett., 2010, 51, 489 CrossRef CAS;
(b) P. Goswami and B. Das, Tetrahedron Lett., 2009, 50, 2384 CrossRef CAS;
(c) H. Wu, X.-M. Chen, Y. Wan, L. Ye, H.-Q. Xin, H.-H. Xu, C.-h. Yue, L.-I. Pang, R. Ma and D.-Q. Shi, Tetrahedron Lett., 2009, 50, 1062 CrossRef CAS;
(d) C. Mukhopadhyay, A. Datta and R. J. Butcher, Tetrahedron Lett., 2009, 50, 4246 CrossRef CAS;
(e) S. Wang, S. Matsumura and K. Toshima, Tetrahedron Lett., 2007, 48, 6449 CrossRef CAS;
(f) M. L. Kantam, C. V. Rajasekhar, G. Gopikrishna, K. R. Reddy and B. M. Choudary, Tetrahedron Lett., 2006, 47, 5965 CrossRef CAS;
(g) T. Ollevier, E. Nadeau and A.-A. Guay-Bégin, Tetrahedron Lett., 2006, 47, 8351 CrossRef CAS;
(h) P. Shukla, N. Chattopadhyay and S. K. Nayak, Natl. Pdt. Commun., 2009, 4, 247 CAS;
(i) T. Ollevier and E. Nadeau, J. Org. Chem., 2004, 69, 9292 CrossRef CAS.
-
(a) T. Ollevier and E. Nadeau, Synlett, 2006, 219 CrossRef CAS;
(b) S. Iimura, D. Nobutou, K. Manabe and S. Kobayashi, Chem. Commun., 2003, 1644 RSC;
(c) B. C. Ranu, S. Samanta and S. K. Guchhait, Tetrahedron, 2002, 58, 983 CrossRef CAS.
- N. Azizi, L. Torkiyan and M. R. Saidi, Org. Lett., 2006, 8, 2079 CrossRef CAS.
- M. A. Bigdeli, F. Nemati and G. H. Mahdavinia, Tetrahedron Lett., 2007, 48, 6801 CrossRef CAS.
- R. Wang, B. G. Li, T. K. Huang, L. Shi and X. X. Lu, Tetrahedron Lett., 2007, 48, 2071 CrossRef CAS.
- H. Li, H.-y. Zeng and H.-w. Shao, Tetrahedron Lett., 2009, 50, 6858 CrossRef CAS.
- K. Manabe and S. Kobayashi, Org. Lett., 1999, 1, 1965 CrossRef CAS.
- M. Wang, Z. Song, X. Wan and S. Zhao, Monatsh. Chem., 2009, 140, 1205 CrossRef CAS.
-
(a)
E. E. Schweizer and D. Meeder-Nycz, in Chemistry of Heterocyclic Compounds: Chromenes, Chromanones and Chromones, ed. G. P. Ellis, Wiley Interscience, New York, 1977, vol. 31, pp. 11 Search PubMed;
(b)
B. A. Keay and P. W. Dibble, in Comprehensive Heterocyclic Chemistry II, ed. A. R. Katrizky, C. W. Rees and E. F. V. Scriven, ed. Pergamon, Oxford, UK, 1996, vol. 2, pp. 395 Search PubMed;
(c) K. C. Nicolaou, J. A. Pfefferkorn, A. J. Roecker, G.-Q. Cao, S. Barluenga and H. J. Mitchell, J. Am. Chem. Soc., 2000, 122, 9939 CrossRef CAS;
(d) G. Zeni and R. C. Larock, Chem. Rev., 2004, 104, 2285 CrossRef CAS;
(e) R. Bergmann and R. Gericke, J. Med. Chem., 1990, 33, 492 CrossRef CAS;
(f) H. C. Shen, Tetrahedron, 2009, 65, 3931 CrossRef CAS.
-
(a) G. C. Rovnyak, S. Z. Ahmed, C. Z. Ding, S. Dzwonczyk, F. N. Ferrara, W. G. Humphreys, G. J. Grover, D. Santafianos, K. S. Atwa, A. J. Baird, L. G. McLaughlin, D. E. Normandin, P. G. Sleph and S. C. Traeger, J. Med. Chem., 1997, 40, 24 CrossRef CAS;
(b) J. M. Evans, C. S. Fake, T. C. Hamilton, R. H. Poyser and E. A. Watts, J. Med. Chem., 1983, 26, 1582 CrossRef CAS.
-
(a) X.-F. Wang, J. An, X.-X. Zhang, F. Tan, J.-R. Chen and W.-J. Xiao, Org. Lett., 2011, 13, 808 CrossRef CAS;
(b) M. Rueping and M.-Y. Lin, Chem.–Eur. J., 2010, 16, 4169 CrossRef CAS;
(c) L. Bernardi, M. Comes-Franchini, M. Fochi, V. Leo, A. Mazzanti and A. Ricci, Adv. Synth. Catal., 2010, 352, 3399 CrossRef CAS;
(d) D. Samanta, R. B. Kargbo and G. R. Cook, J. Org. Chem., 2009, 74, 7183 CrossRef CAS;
(e) P. Holmberg, L. Tedenborg, S. Rosqvist and A. M. Johansson, Bioorg. Med. Chem. Lett., 2005, 15, 747 CrossRef CAS;
(f) E. A. Voight, J. F. Daanen, S. M. Hannick, B. H. Shelat, F. A. Kerdesky, D. J. Plata and M. E. Kort, Tetrahedron Lett., 2010, 51, 5904 CrossRef CAS;
(g) M. Anniyappan, D. Muralidharan and P. T. Perumal, Tetrahedron, 2002, 58, 10301 CrossRef CAS;
(h) J. Wang, F.-X. Xu, X.-F. Lin and Y.-G. Wang, Tetrahedron Lett., 2008, 49, 5208 CrossRef CAS;
(i) M. Anniyappan, D. Muralidharan, P. T. Perumal and J. J. Vittal, Tetrahedron, 2004, 60, 2965 CrossRef CAS;
(j) J. S. Yadav, B. V. S. Reddy, C. Madhuri, G. Sabitha, B. Jagannadth, S. K. Kumar and A. C. Kunwar, Tetrahedron Lett., 2001, 42, 6381 CrossRef CAS;
(k) J. S. Yadav, B. V. S. Reddy, K. C. Sekhar and V. Geetha, Tetrahedron Lett., 2001, 42, 4405 CrossRef CAS;
(l) R. S. Kumar, R. Nagarajan and P. T. Perumal, Synthesis, 2004, 949 CAS;
(m) V. Recuero, G. Gonzalo, R. Brieva and G. Vicente, Eur. J. Org. Chem., 2006, 4224 CrossRef CAS;
(n) K. Huang, F. G. Merced, M. Ortiz-Marciales, H. J. Meléndez, W. Correa and M. D. Jesús, J. Org. Chem., 2008, 73, 4017 CrossRef CAS.
-
(a) M. Wolter, G. Nordmann, G. E. Job and S. L. Buchwald, Org. Lett., 2002, 4, 973 CrossRef CAS;
(b) R. A. Altman, A. Shafir, A. Choi, P. A. Lichtor and S. L. Buchwald, J. Org. Chem., 2008, 73, 284 CrossRef CAS.
- J. Niu, P. Guo, J. Kang, Z. Li, J. Xu and S. Hu, J. Org. Chem., 2009, 74, 5075 CrossRef CAS.
- W.-B. Yi and C. Cai, J. Fluorine Chem., 2006, 127, 1515 CrossRef CAS.
|
This journal is © The Royal Society of Chemistry 2012 |
Click here to see how this site uses Cookies. View our privacy policy here.