DOI:
10.1039/C2RA20385E
(Paper)
RSC Adv., 2012,
2, 4415-4420
Simple, efficient and selective colorimetric sensors for naked eye detection of Hg2+, Cu2+ and Fe3+†
Received
2nd March 2012
, Accepted 2nd March 2012
First published on 3rd April 2012
Abstract
Compounds 3 and 4 are all push–pull molecules containing both a donor 1,3-dithiole ring and an acceptor carbonyl group or thiocarboyl group, and their structures confer them with special spectroscopic properties. Their recognition behaviors towards metal ions were explored by UV-vis spectral analysis, and the results showed that combination of 3 and 4 could efficiently detect Hg2+, Cu2+ and Fe3+. The instant color changes induced by the addition of corresponding metal ions make them excellent naked eye sensors for the detection of heavy metal ions.
Indroduction
Heavy metal pollution impacted by human activities such as industrialization and discharge of contaminated sewage has caused great concern.1 Some heavy metal ions are very toxic to the environment and human beings even in low concentrations, for example Hg2+ can trigger several serious disorders, including sensory, motor, and neurological when inhaled by the human body.2 Other heavy metal ions have bio-importance as trace elements but show high toxicity in higher concentrations, such as Cu2+ and Fe3+.3 As a result, many sophisticated analytical tools have been developed to detect different metal ions, including selective fluorescent probes, colorimetric sensors and electrical sensors.4 In this respect, we have been interested in developing a selective colorimetric sensor for the naked eye detection of metal ions due to its simplicity and convenience.
The 1,3-dithiole ring is a good electron donor and can be readily used to extend the π-electron delocalization by linking other conjugated chains to construct highly conjugated systems, which possess good electrical and spectral properties, such as TTF, extended TTF and relative derivatives.5 Active and fast-responding sensors are easily prepared when the 1,3-dithiole ring or its derivatives are connected to appropriate receptor groups as transduction elements.6 Moore and Bryce7 developed several kinds of 1,3-dithiole derivatives as π-electron donors for organic metals in 1991, among which 10-(1,3-dithiol-2-ylidene)anthrancene-9(10H)-one raises our interest due to its specific structure and spectral properties. Due to a resonance contribution of the aromatic dipolar structure, its UV-vis spectrum shows an absorption band at 467 nm and is bright orange in appearance. Zhu et al. have synthesized fluorometric chemodosimeters for Hg2+ ions based on the desulfurization of the thiocarbonyl groups of 1,3-dithiole-2-thione units.8a,8b Considering that the carbonyl group could be possibly generated from the thiocarbonyl group by desulfurization of Hg2+, we designed the analogical molecule 10-(1,3-dithiol-2-ylidene)anthrancene-9(10H)-thione, which behaves a push–pull molecule, bearing both an electron donor 1,3-dithiole ring and an electron acceptor chromophore thiocarbonyl group and shows an absorption band at 579 nm.7,9 We speculate that the sulfur atoms in the conjugated molecule may function as binding sites to coordinate with Hg2+ due to the strong thiophilic affinity of Hg2+, or furthermore Hg2+ may promote desulfurization of the thiocarbonyl group followed by formation of a ketone.8 Whichever interaction between the molecule and metal ions may influence the π-electron delocalization of the whole system, it will consequently lead to changes in absorption properties. Due to the bright color appearance of this conjugated compound, it's likely to work as naked eye sensor. Here, we report the synthesis of the colorimetric sensors and their derivatives by a simple two-step reaction, and explore their recognition behaviors towards various metal ions by UV-vis spectrophotometric detection. By combination of the sensors and their precursors of the ketones, we can selectively detect Hg2+, Cu2+ and Fe3+ using both UV-vis spectra and the naked eye.
Results and discussion
Synthesis
Compound 4a and its derivative 4b were easily synthesized by a two-step reaction. As shown in Scheme 1, thione 2 was reacted with excess anthraquinone 1a and 2,6-dimethoxyl anthraquinone 1b in triethylphosphite at 135 °C for 3 h, yielding ketones 3a and 3b, respectively.10 Remarkably, 3a and 3b were easily converted into thione 4a and 4bvia the reactions with Lawesson's reagent in 80 and 85% yields, respectively.11 Compounds 3a and 3b are both fresh orange solids, which is distinctive compared to the dark blue color of 4a and 4b.
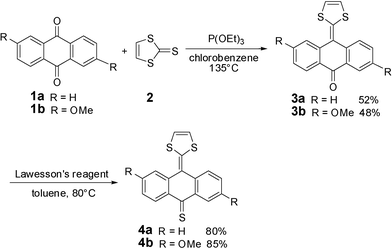 |
| Scheme 1 Synthesis of compounds 4a and 4b. | |
UV-vis spectroscopic recognition of 4a and 4b towards metal ions
Our initial target was to design a selective Hg2+ sensor through combinatorial interaction with sulfur atoms or irreversible desulfurization of the thiocarbonyl group promoted by Hg2+, leading to significant changes in the absorption spectrum which could be easily detected. In fact, when 1 equiv. of various metal ions (Co2+, Ni2+, Ag+, Mn2+, Cu2+, Hg2+, Fe3+, Cd2+, Zn2+, Pb2+, Ba2+, Mg2+, Na+, K+, Cs+) were added to a 50 μM solution of 4a in acetonitrile, it was found that both Hg2+ and Cu2+ induced an instant color change from dark blue to orange while the other metal ions failed to show any significant interference as shown in Fig. 1. Although it is less obvious, we could still note that there's some difference in color between the 4a-Hg2+ and 4a-Cu2+ systems. The absorption behaviors of 4a in the presence of the different metal ions are presented in Fig. 2. Upon the addition of both Hg2+ and Cu2+, a decrease in the absorption band at 579 nm and a concomitant increase of a new band at 263 nm was observed. Interestingly, when EDTA was added to the 4a-Hg2+ and 4a-Cu2+ systems, the color of the solution changed back to the original blue instantly. This may be attributed to the strong complexation of guest ions with EDTA that prevents the interaction of metal ions with the chemodosimeter 4a. These results implied that no desulfurization reaction happened. It seems that the stable conjugated system is more difficult to destroy than simple thioketones. Considering the strong sulfur affinity of Hg2+ and Cu2+, it's easy to conclude that these two metal ions form complexes with compound 4a through reversible chelation interactions. The influence of other metal ions on the recognition of working ions was also considered. As shown in Fig. S5,† the existence of other metal ions causes no interference to recognition of the absorption spectra. There is only a little difference when Hg2+ and Cu2+ are both present in the solution.
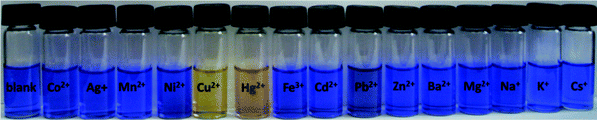 |
| Fig. 1
1 Color changes of the solution of 4a (50 μM) in acetonitrile upon the addition of 1 equiv. of various metal ions. | |
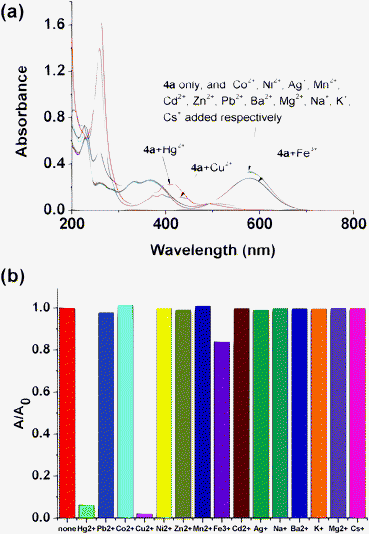 |
| Fig. 2 (a) Absorption spectra of 4a (20 μM) in acetonitrile with the addition of 1 equiv. of various metal ions; (b) comparison of absorbance at 579 nm. | |
To further inquire into the spectral characteristics of thione 4a upon the addition of Cu2+ and Hg2+, the influence of the amount of Cu2+ and Hg2+ on the absorption spectra of 4a were measured. As shown in Fig. 3, with the gradual addition of Hg2+ to 4a, the absorption band at 579 nm decreased gradually in intensity and a new absorption peak at 263 nm was developed. Titration curves at 579 nm obtained from sigmoidal fitting are displayed as the red lines in the inset of Fig. 3.
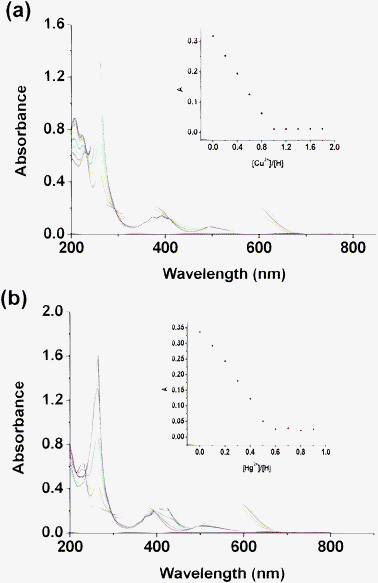 |
| Fig. 3 (a) UV-vis titration of compound 4a (2.0 × 10−5 M in acetonitrile) upon the addition of an acetonitrile solution of Cu(ClO4)2. Inset: absorption at 579 nm vs. the number of equivalents of Cu2+ added. (b) UV-vis titration upon the addition of an acetonitrile solution of Hg(ClO4)2. Inset: absorption at 579 nm vs. the number of equivalents of Hg2+ added. | |
The absorption maximum reaches a saturation state after total addition of about 0.5 equiv. of Hg2+. Job plots (Fig. S8†) further indicated the 1
:
2 binding model of Hg2+-4a. Accordingly, the binding constant Ka was determined to be 9.39 × 1016 M−2.12 Similarly, we found that 4a binds Cu2+ in a 1
:
1 ratio with a binding constant Ka = 3.67 × 107 M−1. The detection limits of 4a towards Hg2+ and Cu2+ were measured to be 1.2 × 10−7 M and 4.0 × 10−7 M, respectively.
The recognition behaviors of compound 4b towards different metal ions was also investigated by UV-vis absorption spectroscopy in acetonitrile, and similar results were acquired (Fig. S7†). Compound 4b exhibited simultaneous sensitivity towards both Cu2+ and Hg2+ by forming complexes with a dramatic change in absorption bands at 579 nm and 276 nm. Titration experiments and Job's method were carried out to find the stoichiometric composition of the complexes; 1
:
1 for Cu2+-4b and 1
:
2 for Hg2+-4b were found (Fig. S9, S10†). The association constant for a 1
:
1 complex between 4b and Cu2+ in acetonitrile was calculated to be 1.12 × 106 M−1, and the association constant for a 1
:
2 complex between 4b and Hg2+ is 2.23 × 1018 M−2. The detection limits of 4b towards Hg2+ and Cu2+ were measured to be 8 × 10−8 M and 6 × 10−8 M, respectively.
NMR studies for the complexation of 4a with Hg2+
The 1H NMR titration experiments of compound 4a in DMSO-d6 before and after the addition of different amounts of Hg2+ ion were further carried out to explore more details about the complexation between 4a and Hg2+. As shown in Fig. 4, the resonance signal of protons on the 1,3-dithiole ring as peak a goes through a significant downfield shift of about 2.57 ppm upon the addition of 0.5 equiv. of Hg2+, and a broadening effect is observed during this process. The resonance of peak e shows downfield shifts of about 0.55 ppm while other peaks show upfield shifts in various degrees. Obvious changes of the chemical shifts indicated that 4a could form a stable complex with Hg2+. While considering that whichever sulfur atom on the 1,3-dithiole ring or thiocarbonyl group binds with Hg2+ may cause similar changes, and that the two molecules binding with the same Hg2+ may also have influences on each other, we need further studies to determine the binding sites.
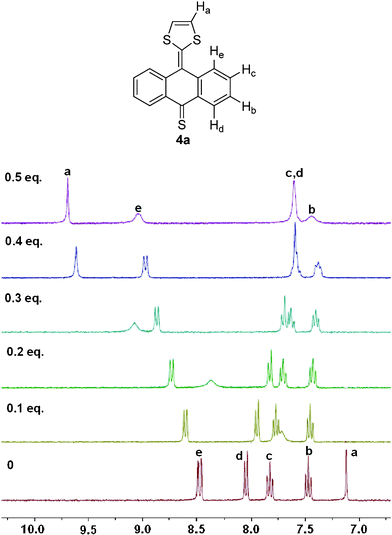 |
| Fig. 4
1H NMR titration spectra of compound 4a in DMSO-d6 upon the addition of Hg2+ from 0 to 0.5 equivalents. | |
UV-vis spectroscopic recognition of 3a–b towards metal ions
Given the chelating interaction between compound 4a and the two relative metal ions, we assume that ketone 3a, which has an analogical structure, may exhibit similar properties to those of 4a. As shown in Fig. 5, the selectivity of ketone 3a to various metal ions was examined by adding 2 equiv. of metal perchlorate salts to compound 3a in acetonitrile and collecting the resulting UV-vis spectra. It's interesting to find that compound 3a showed a marked selectivity for Cu2+ and Fe3+ leading to a dramatic decrease of the absorption band at 467 nm, while Hg2+ shows no interference. The orange solution is bleached completely to colorless after the addition of Cu2+ and Fe3+ to the solution, which can be easily observed by the naked eye as revealed in Fig. 6. The titration of compound 3a with increasing amounts of Cu2+ and Fe3+ is shown in Fig. 7, and 2 equiv. of metal ions have been added until the absorbance intensity is saturated. The strange ratio and no clear isosbestic points indicate that it's not a simple association interaction between ketone 3a and the two relative metal ions. Actually, we found a new compound formed by TLC detection and isolated it from the mixed solution. The NMR spectra and EI mass both verified that this new compound is the original material anthraquinone 1a. The results illuminate that the oxidative activation of Cu2+ and Fe3+ induces the breaking of the double bond between the anthraquinone moiety and the 1,3-dithiole ring, destroying the conjugated system and consequently leading to the color changes. The response was so fast that no 3a was detected by TLC just after the addition of a saturation volume of metal ions and short stirring for 5 s.
 |
| Fig. 6 Color changes of the solution of 3a (50 μM) in acetonitrile upon the addition of 2 equiv. of various metal ions. | |
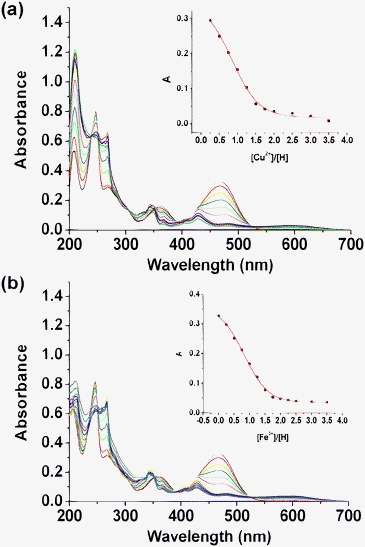 |
| Fig. 7 (a) UV-vis titration of compound 3a (2.0 × 10−5 M) in acetonitrile upon addition of an acetonitrile solution of Cu(ClO4)2. Inset: absorption at 467 nm vs. the number of equivalents of Cu2+ added. (b) UV-vis titration upon the addition of an acetonitrile solution of Fe(ClO4)3. Inset: absorption at 467 nm vs. the number of equivalents of Fe3+ added. | |
The ion recognition properties of compound 3b were also examined. Under the same conditions, Cu2+ and Fe3+ promote dramatic changes in the absorption spectra, and bleaching of the orange solution of 3b to colorless is readily observed visually. Moreover, 2,6-dimethoxyl anthraquinone 1b formed in the reaction can also be detected and isolated.
While it has the same 1,3-dithiole ring as 4a, compound 3a has no interaction with Hg2+. The different responses indirectly indicate that the site of 4a binding with Hg2+ and Cu2+ should be the sulfur atom on the thiocarbonyl group, otherwise Hg2+ will also bind with 3a and result in absorption changes. The decomposition caused by Cu2+ and Fe3+ to 3a but not to 4a also shows the higher stability of 4a over 3a. Interestingly, it seems easy to distinguish Hg2+, Cu2+, and Fe3+ ions through combination of compounds 3 and 4 due to their different recognition behaviors towards metal ions. Although the introduction of two methoxyl groups to 3a and 4a doesn't cause obvious changes in detection of metal ions, we can utilize these two derivation sites to incorporate other functional groups to modify the sensors' water solubility for further applications in real life.
Conclusions
Simple push–pull compounds were found to be able to recognize Hg2+, Cu2+ and Fe3+ selectively. The coordination model between metal ions and thione 4, and the reaction model induced by metal ions to ketone 3 were further described. The absorption changes and significant color changes after the addition of metal ions to the solution of compounds 3 and 4 showed that they could serve as selective and efficient colorimetric sensors for the detection of Hg2+, Cu2+ and Fe3+ by not only UV-vis spectra but also the naked eye method. Further studies will focus on enhancing their water solubility and exploring their potential applications in biological or environmental chemistry.
Experimental section
General
Melting points were recorded on an electrothermal melting point apparatus and are uncorrected. 1H and 13C NMR spectra were obtained by a Bruker DMX300 NMR. EI-MS spectra were obtained by a Waters GCT mass spectrometer. Elemental analyses were performed by the Analytical Laboratory of the Institute of Chemistry, CAS. Absorption spectra were measured on a UV-vis spectrophotometer (Shimadzu UV-2401PC) in acetonitrile of HPLC grade using a 10 mm path length quartz cell. Perchlorate (Co2+, Ni2+, Ag+, Mn2+, Cu2+, Hg2+, Fe3+, Cd2+, Zn2+, Pb2+, Ba2+, Mg2+, Na+, K+, Cs+) were used as the metal cation source, and their stock solutions were prepared by dissolution in acetonitrile (1 × 10−2 M in CH3CN). During the titration, different amounts of metal perchlorate were added by microsyringe to a solution of sensors (2 × 10−5 M in CH3CN) accordingly to result in requisite mole ratios, and the total volume of the solution was maintained at 3 mL in each case. Absorption spectra were taken just after short stirring. Job plots were measured according to the method for continuous variations of mole fraction of metal ions while the total concentration of metal ion and the sensor is kept at 2 × 10−5 M. The binding constant was calculated by Hyperquad software.
Synthesis of 3a.
7
Anthraquinone 1a (0.8 g, 3.84 mmol) was dissolved in a mixed solution of triethylphosphite (5 mL) and chlorobenzene (15 mL). The mixture solution was degassed and stirred under argon at 135 °C whereupon thione 2 (0.264 g, 2.0 mmol) was added as a solid in small portions over 1.5 h, affording a dark red solution. Then another potion of 1a (0.4 g, 1.92 mmol) was added once, and more thione 2 (0.264 g, 2.0 mmol) in small portions was added over the next 1.5 h. The reaction mixture was cooled to room temperature, and filtered to remove the excess anthraquinone. The filtrate was condensed under reduced pressure, and methanol (30 mL) was added to give a red precipitate. The precipitate was filtered, washed with methanol, dried in vacuo, and purified by flash column chromatography (silica gel, dichloromethane
:
PE 1
:
1) to afford 3a as an orange powder (0.59 g, 50%). Mp: 219–221 °C; MS (EI): m/z 294 (M+); 1H NMR (300 MHz, CDCl3): δ 8.27 (dd, J = 7.8, 1.4 Hz, 2H), 7.95 (d, J = 7.9 Hz, 2H), 7.65 (td, J = 7.8, 1.5 Hz, 2H), 7.42 (td, J = 7.8, 0.9 Hz, 2H), 6.47 (s, 2H).
Synthesis of 4a.
7
A mixture of ketone 3a (29.4 mg, 0.1 mmol) and Lawesson's reagent (60.6 mg, 0.15 mmol) in dry toluene (10 mL) was stirred at 80 °C for 2 h. It was then cooled, poured over a silica plug, and washed with toluene until all the brown product had eluted. The filtrate was evaporated under vacuum, and the residue was then purified by flash column chromatography eluting with petroleum–dichloromethane mixture (1
:
1, v/v), affording compound 4a (22 mg, 80%) as a dark-blue solid. Mp: 215–217 °C; MS (EI): m/z 310 (M+); 1H NMR (300 MHz, CDCl3): δ 8.56 (dd, J = 8.1, 1.0 Hz, 2H), 7.93 (d, J = 8.0 Hz, 2H), 7.72–7.61 (m, 2H), 7.42–7.30 (m, 2H), 6.52 (s, 2H).
Synthesis of 3b.
Compound 3b as a bright orange solid was prepared in a similar manner as described for 3a from 402 mg of 1b (1.5 mmol) and 132 mg of 2 (1 mmol), Yield: 48% (169.9 mg). Mp: 232 °C. MS (EI): m/z 354 (M+); UV-vis (CH3CN): λmax 465, 350, 258, 214 nm. 1H NMR (300 MHz, CDCl3): δ 8.25 (d, J = 8.7 Hz, 1H), 7.88 (d, J = 8.7 Hz, 1H), 7.76 (d, J = 2.9 Hz, 1H), 7.36 (d, J = 2.4 Hz, 1H), 7.19 (dd, J = 8.7, 2.9 Hz, 1H), 6.94 (dd, J = 8.7, 2.4 Hz, 1H), 6.47 (s, 2H), 3.95 (d, J = 12.1 Hz, 6H). 13C NMR (75 MHz, CDCl3): δ 182.1, 162.6, 158.0, 143.5, 141.4, 132.8, 132.2, 129.7, 127.5, 124.4, 119.2, 118.0, 117.8, 112.8, 110.9, 109.3, 77.2, 55.7, 55.6. Anal. Calcd for C19H14OS2: C, 64.38; H, 3.98; S, 18.09. Found: C, 64.35; H, 3.99; S, 18.07.
Synthesis of 4b.
Thione 4b as a green blue solid was prepared following the procedure as described for 4a. Yield: 85%. Mp: 233 °C. MS (EI): m/z 370 (M+). UV-vis (CH3CN): λmax 235, 363, 579 nm. 1H NMR (300 MHz, DMSO-d6): δ 8.51 (d, J = 9.0 Hz, 1H), 8.01 (dd, J = 7.7, 5.9 Hz, 2H), 7.40 (m, 2H), 7.17–6.99 (m, 3H), 3.93 (d, J = 29.1 Hz, 6H). 13C NMR (75 MHz, DMSO-d6): δ 209.5, 162.9, 157.4, 150.2, 148.6, 138.2, 134.9, 132.4, 132.0, 127.1, 126.3, 120.4, 119.1, 117.3, 114.5, 111.4, 109.0, 55.9, 55.4. Anal. Calcd for C19H14S3O2·0.05H2O: C, 61.44; H, 3.83; S, 25.90. Found: C, 61.42; H, 3.84; S, 25.88.
Acknowledgements
We thank the National Natural Science Foundation of China (21072220), Beijing National Laboratory for Molecular Sciences, and the National Basic Research Program (2011CB932501) for financial support.
References
- J. O. Duruibe, M. O. C. Ogwuegbu and J. N. Egwurugwu, Int. J. Phys. Sci., 2007, 2, 112–118 Search PubMed.
-
(a) A. Renzoni, F. Zino and E. Franchi, Environ. Res., 1998, 77, 68–72 CrossRef CAS;
(b) O. Malm, Environ. Res., 1998, 77, 73–78 CrossRef CAS.
-
(a) L. M. Gaetke and C. K. Chow, Toxicology, 2003, 189, 147–163 CrossRef CAS;
(b) P. G. Welsh, J. Liptona, C. A. Mebaneb and J. C. A. Marr, Ecotoxicol. Environ. Saf., 2008, 69, 199–208 CrossRef CAS;
(c) J. B. George, Chem. Res. Toxicol., 2010, 23, 319–326 CrossRef;
(d) A. Shander, M. D. Cappellini and L. T. Goodnough, Vox Sang., 2009, 97, 185–197 CrossRef CAS.
-
(a) L. Prodi, F. Bolletta, M. Montalti and N. Zaccheroni, Coord. Chem. Rev., 2000, 205, 59–83 CrossRef CAS;
(b) R. Sheng, P. Wang and S. Li, Org. Lett., 2008, 10, 5015–5018 CrossRef CAS;
(c) Q-Y. Cao, M. H. Lee and J. S. Kim, Tetrahedron Lett., 2011, 52, 2786–2789 CrossRef CAS;
(d) J.-M. Liu, Q.-Y. Zheng, J.-L. Yang, C.-F. Chen and Z.-T. Huang, Tetrahedron Lett., 2002, 43, 9209–9212 CrossRef CAS;
(e) Q.-Y. Chen and C.-F. Chen, Tetrahedron Lett., 2005, 46, 165–168 CrossRef CAS;
(f) G.-K. Li, Z.-X. Xu, C.-F. Chen and Z.-T. Huang, Chem. Commun., 2008, 1774–1776 RSC;
(g) X. F. Guo, X. H. Qian and L. H. Jia, J. Am. Chem. Soc., 2004, 126, 2272–2273 CrossRef CAS;
(h) X. J. Zhu, S. T. Fu, W. K. Wong, J. P. Guo and W. Y. Wong, Angew. Chem., Int. Ed., 2006, 45, 3150–3154 CrossRef CAS;
(i) S. Yoon, E. W. Miller, Q. He, P. H. Do and C. J. Chang, Angew. Chem., Int. Ed., 2007, 46, 6658–6661 CrossRef CAS;
(j) J. Y. Kwon, Y. J. Jang, Y. J. Lee, K. M. Kim, M. S. Seo, W. Nam and J. Y. Yoon, J. Am. Chem. Soc., 2005, 127, 10107–10111 CrossRef CAS;
(k) J. H. Huang, Y. F. Xu and X. H. Qian, J. Org. Chem., 2009, 74, 2167–2170 CrossRef CAS;
(l) Z. Q. Hu, C. S. Lin, X. M. Wang, L. Ding, C. L. Cui, S. F. Liu and H. Y. Lu, Chem. Commun., 2010, 46, 3765–3767 RSC;
(m) Z. Q. Hu, X. M. Wang, Y. C. Feng, L. Ding and H. Y. Lu, Dyes Pigm., 2011, 88, 257–261 CrossRef CAS;
(n) X. Chen, J. Jia, H. Ma, S. Wang and X. Wang, Anal. Chim. Acta, 2009, 632, 9–14 CrossRef CAS;
(o) W. Shi and H. Ma, Chem. Commun., 2008, 1856–1858 RSC;
(p) N. Kaur and S. Kumar, Chem. Commun., 2007, 3069–3070 RSC;
(q) N. Kaur and S. Kumar, Tetrahedron Lett., 2008, 49, 5067–5069 CrossRef CAS.
-
(a) C. Wang, M. R. Bryce, A. S. Batsanov and J. A. K. Howard, Chem.–Eur. J., 1997, 3, 1679–1690 CrossRef CAS;
(b) C. Rovira, Chem. Rev., 2004, 104, 5289–5317 CrossRef CAS;
(c) F. Gustavo, M. P. Emilio, S. Luis and M. Nazario, J. Am. Chem. Soc., 2008, 130, 2410–2411 CrossRef.
-
(a) M. R. Bryce, A. S. Batsanov, T. Finn, T. K. Hansen, A. J. Moore, J. A. K. Howard, M. Kamenjicki, I. K. Lednev and S. A. Asher, Eur. J. Org. Chem., 2001, 933–940 CrossRef CAS;
(b) H. A. Zamani, A. Nezhadali and M. Saghravanian, Anal. Lett., 2008, 41, 2727–2742 CrossRef CAS;
(c) M. R. Bryce, B. Johnston, R. Kataky and K. Tothb, Analyst, 2000, 125, 861–866 RSC;
(d) S-G. Liu, H. Liu, K. Bandyopadhyay, Z. Gao and L. Echegoyen, J. Org. Chem., 2000, 65, 3292–3298 CrossRef CAS.
- A. J. Moore and M. R. Bryce, J. Chem. Soc., Perkin Trans. 1, 1991, 1, 157–168 RSC.
-
(a) G. Zhang, D. Zhang, X. Yin, S. Yang, Z. Shuai and D. Zhu, Chem. Commun., 2005, 2161–2163 RSC;
(b) H. Lu, Y. Tang, W. Xu, D. Zhang, S. Wang and D. Zhu, Macromol. Rapid Commun., 2008, 29, 1467–1471 CrossRef CAS;
(c) G. Zhang, D. Zhang, X. Guo and D. Zhu, Org. Lett., 2004, 6, 1209–1212 CrossRef CAS;
(d) X. Li, G. Zhang, H. Ma, D. Zhang, J. Li and D. Zhu, J. Am. Chem. Soc., 2004, 126, 11543–11548 CrossRef CAS;
(e) M. Y. Chae and A. W. Czarnik, J. Am. Chem. Soc., 1992, 114, 9704–9705 CrossRef CAS;
(f) K. C. Song, J. S. Kim, S. M. Park, K-C. Chung, S. Ahn and S. K. Chang, Org. Lett., 2006, 8, 3413–3416 CrossRef CAS;
(g) L. Liu, G. Zhang, J. Xiang, D. Zhang and D. Zhu, Org. Lett., 2008, 10, 4581–4584 CrossRef CAS.
- D. Masuda, H. Wakabayashi, H. Miyamae, H. Teramae and K. Kobayashi, Tetrahedron Lett., 2008, 49, 4342–4345 CrossRef CAS.
- C. A. Christensen, A. S. Batsanov and M. R. Bryce, J. Org. Chem., 2007, 72, 1301–1308 CrossRef CAS.
- N. P. Kyle, G. Kamil, N. Colin and T. Noah, Org. Lett., 2009, 11, 2225–2228 CrossRef.
-
(a)
H.-J. Schneider and A. K. Yatsimirsky, Principles and methods in supramolecular chemistry, John Wiley and Sons, New York, 2000 Search PubMed;
(b)
K. A. Connors, Binding constants, John Wiley and Sons, New York, 1987 Search PubMed;
(c) C.-F. Chen and Q.-Y. Chen, New J. Chem., 2006, 30, 143–147 RSC.
|
This journal is © The Royal Society of Chemistry 2012 |
Click here to see how this site uses Cookies. View our privacy policy here.