DOI:
10.1039/C2RA20445B
(Communication)
RSC Adv., 2012,
2, 6397-6400
Pd(0) NPs: a novel and reusable catalyst for the synthesis of bis(heterocyclyl)methanes in water†
Received
10th March 2012
, Accepted 23rd May 2012
First published on 24th May 2012
Abstract
A ligand free, Pd-nanoparticle catalyzed synthesis of bis(heterocyclyl)methane derivatives is described herein. The advantages of this method lie in its simplicity, cost effectiveness and environmentally friendliness. The process is very green, as water is exploited as the reaction medium.
Introduction
Metal particles in the nanometer range exhibit quantum mechanical properties that make them promising candidates for applications in nanotechnology. The last decade has witnessed tremendous growth in the field of nanoscience and nanotechnology. Easy access to nanoparticles (NPs) has prompted investigations into their applications in catalysis. Several reports have shown their remarkable level of performance as catalysts in terms of selectivity, reactivity, and improved product yield.1 The properties of metal nanoparticles, such as palladium, gold and platinum, may well differ markedly from those of the respective bulk metals.2 These metal nanoparticles exhibit size-induced quantum-size effects (i.e. electron confinement and surface effect)2,3 and can be exploited for a number of advanced functional applications, such as sensors,4 electronics and catalysis.5,6 In general, the catalytic properties of metal nanoparticles are a function of their size, crystal lattice parameters, and properties of carrier systems. As part of our interest in this area, we initiated an investigation to explore the potential of metal nanoparticles for C–C bond formation. Recently, much attention has been directed toward the synthesis of bis(heterocyclyl)methanes because of their diverse biological and pharmacological activities.7–13 Bis(heterocyclyl)methanes constitute building blocks in natural and unnatural porphyrins.14 Because of their biological activity, several different methods have been developed, such as Amberlyst 15,15 Zn(Proline)2,16 Montmorillonite clay,17 InCl3,18 TiO2/SO4,19 phosphomolybdic acid20 and ethyl ammonium nitrate (EAN).21 Although most of these processes offer distinct advantages, at the same time they suffer from certain drawbacks such as longer reaction times, use of organic solvents, unsatisfactory yields, high costs, harsh reaction conditions, use of stoichiometric amounts, as well as environmentally toxic catalysts.17,18 Thus, the development of simple, highly efficient methodologies remains desirable.
Green chemical reactions have become a preeminent issue in recent decades. Reactions in aqueous media are considerably more safe, non-toxic, environmentally friendly and inexpensive. At the beginning of this century, a shift in emphasis in chemistry was apparent, with the desire to develop environmentally benign routes using non-toxic reagents, solvents and catalysts. Organic solvents represent the biggest pollution problem in many synthetic organic processes, and the development of efficient synthetic methodologies for organic reactions in the absence of organic solvents is an important challenge. An ideal organic reaction would proceed in neat substrate(s) or an environmentally benign solvent, such as water.22 The design of synthetic methods for the preparation of privileged bis(heterocyclyl)methanes and the use of water as the reaction medium constitute a very important direction in green chemistry.
To the best of our knowledge, however, any palladium nanoparticle promoted synthesis of bis(heterocyclyl)methane derivatives has not been reported up to now. In the present work, we report a novel, ligand-free protocol for the synthesis of bis(heterocyclyl)methanes catalyzed by palladium(0) nanoparticles in water.
Result and discussion
As a part of our ongoing interest in the palladium nanoparticle catalyzed reaction,23 we had the opportunity to further explore its catalytic activity toward the synthesis of bis(heterocyclyl)methanes. Recently it has been proven that Pd(0) nanoparticles as catalysts offer great opportunities for a wide range of applications in organic synthesis, such as the Heck reaction, Sonogashira coupling, hydrogenation of olefins and Hiyama cross-coupling etc.24 In our present study, catalytically active Pd(0) nanoparticles were synthesized in the presence of PdCl2, tetrabutyl ammonium bromide (TBAB) and sodium carbonate (Na2CO3). We assume that Pd2+ was reduced to its zero-valent state in water due to the transfer of two electrons from the Br− ion of TBAB.25b,c Under these reaction conditions, palladium nanoparticles were stabilized by TBAB and sodium carbonate acted as a base.25
The formation of Pd nanoparticles was detected by transmission electron microscopy (TEM) (Fig. 1) and X-ray diffraction (XRD) (Fig. 2). The TEM micrograph showed that palladium nanoparticles had an average size of 20–50 nm. The presence of some larger particles should be attributed to aggregation or overlapping of smaller particles.
The prepared Pd NPs were used as a catalyst in the synthesis of bis(heterocyclyl)methane derivatives. As a model reaction, we selected 2 equiv. of 4-hydroxy coumarin 1 (mmol), 1 equiv. of benzaldehyde 5a (mmol) and Pd NPs (0.045 mmol) in water and it afforded an 89% yield of the product 6a (Scheme 1). The structure was determined from the analytical and spectral data. In order to study the generality of this procedure a series of biscoumarols was synthesized. The results are listed in Table 1. Several activated and deactivated aromatic aldehydes offered a high yield of the product with high purity. With these encouraging results, we turn to explore the scope of the reaction using other C–H activated compounds such as indole, dimedone and pyrazolone using aryl aldehydes under the optimized reaction conditions. The results are summarized in Table 1. All the products were characterized by elemental analysis and spectroscopic data with those that are reported in the literature.15–24 From Table 1, it is evident that an aromatic aldehyde containing either an electron donating or an electron withdrawing substituent provides good yields within a short reaction time.
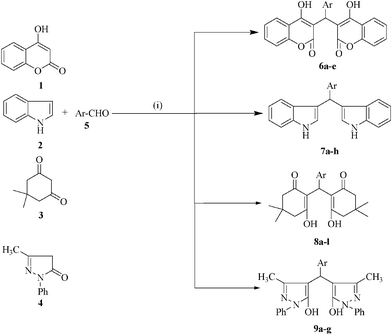 |
| Scheme 1 Reagents and conditions: (i) PdNPs, water, refluxed, 0.3–1.3 h. | |
Entry |
Ar–CHO |
Product |
Time (h) |
Yield (%)a |
m.p. (°C) |
Isolated yields.
|
1 |
C6H5 |
6a
|
0.6 |
89 |
228–229 |
2 |
4-NO2C6H4 |
6b
|
0.5 |
92 |
230–232 |
3 |
4-CH3C6H4 |
6c
|
0.6 |
88 |
274–276 |
4 |
4-OCH3C6H4 |
6d
|
0.5 |
87 |
246–247 |
5 |
4-BrC6H4 |
6e
|
0.5 |
90 |
258–260 |
6 |
C6H5 |
7a
|
1.2 |
88 |
149–150 |
7 |
4-CH3C6H4 |
7b
|
1.3 |
86 |
96–98 |
8 |
4-NO2C6H4 |
7c
|
1 |
88 |
219–221 |
9 |
4-FC6H4 |
7d
|
1 |
87 |
69–71 |
10 |
4-CNC6H4 |
7e
|
1 |
89 |
120–122 |
11 |
4-BrC6H4 |
7f
|
1 |
90 |
75–77 |
12 |
4-ClC6H4 |
7g
|
1.1 |
91 |
78–79 |
13 |
C6H10 |
7h
|
1.3 |
86 |
223–224 |
14 |
C6H5 |
8a
|
0.3 |
88 |
199–201 |
15 |
4-OCH3C6H4 |
8b
|
0.4 |
86 |
230–231 |
16 |
3-NO2C6H4 |
8c
|
0.4 |
88 |
157–159 |
17 |
3-FC6H4 |
8d
|
0.4 |
90 |
118–120 |
18 |
4-BrC6H4 |
8e
|
0.3 |
90 |
221–223 |
19 |
2-CH3C6H4 |
8f
|
0.3 |
87 |
157–159 |
20 |
4-CNC6H4 |
8g
|
0.3 |
91 |
209–211 |
21 |
3-ClC6H4 |
8h
|
0.3 |
88 |
175–177 |
22 |
4-N(CH3)2C6H4 |
8i
|
0.5 |
86 |
227–229 |
23 |
4-CH3C6H4 |
8j
|
0.4 |
86 |
208–210 |
24 |
4-NO2C6H4 |
8k
|
0.3 |
90 |
230–232 |
25 |
4-ClC6H4 |
8l
|
0.3 |
90 |
210–212 |
26 |
C6H5 |
9a
|
1.0 |
89 |
171–173 |
27 |
2-ClC6H4 |
9b
|
1.3 |
86 |
198–200 |
28 |
4-FC6H4 |
9c
|
1.0 |
87 |
180–182 |
29 |
4-NO2C6H4 |
9d
|
1.0 |
90 |
224–226 |
30 |
4-BrC6H4 |
9e
|
1.1 |
88 |
182–184 |
31 |
4-ClC6H4 |
9f
|
1.2 |
87 |
200–202 |
32 |
4-CH3C6H4 |
9g
|
1.3 |
86 |
199–201 |
A plausible mechanism for the synthesis of bis(heterocyclyl)methanes by palladium nanoparticles is shown in Scheme 2. The electrophilicity of the carbonyl carbon atom of both the aldehyde and active methylene compounds is increased due to coordination with the palladium nanoparticles. The aromatic aldehydes and active methylene compounds undergo Knoevenagel condensation followed by Michael addition, and tautomerization furnished the desired products.
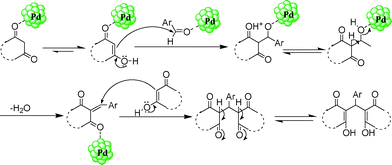 |
| Scheme 2 Plausible reaction mechanism. | |
Domino Knoevenagel condensation/Michael addition of 4-hydroxy coumarin and benzaldehyde using Pd(0) nanoparticles was investigated with various solvents, including acetonitrile (CH3CN), dichloromethane (DCM), and tetrahydrofuran (THF). Significant improvement was achieved in DCM and THF (Table 2, entries 3 and 2) but the best result was observed when we used water as a medium.
Table 2 Optimization of the solvent effecta
Entry |
Solvent |
Temp./°C |
Time (min) |
Yield (%)b |
Reagents and conditions: 4-hydroxy coumarin (2 mmol), benzaldehyde (1 mmol), PdCl2 (0.04 mmol), TBAB (1 mmol), Na2CO3 (3 mmol).
Isolated yields
|
1 |
MeCN |
82 |
80 |
60 |
2 |
THF |
66 |
85 |
66 |
3 |
DCM |
39 |
90 |
71 |
4 |
H2O |
100 |
60 |
89 |
5 |
H2O |
100 |
70 |
89 |
In transition metal catalyzed reactions, ligands play an important role. Various phosphine and nonphosphine ligands for palladium catalyzed coupling reactions are described in the literature (Table 3). Most of these ligands are air and moisture sensitive, difficult to prepare, and expensive. Thus, catalysis under ligand-free conditions is an area of high importance.26
Table 3 Comparison of the results for the synthesis of bis(heterocyclyl) methanes with other catalysts
The promising nature of the Pd nanoparticle catalyst in this work is due to many factors. One is the low catalyst loading, and the others are reusability of the catalyst, short reaction time, easy and simple reaction procedure and, moreover, it is environmentally friendly.
To determine the appropriate concentration of palladium nanoparticles, we have investigated the model reaction at different concentrations of catalyst such as 0.02, 0.04 and 0.06 mmol. The best yield was found using 0.04 mmol Pd nanoparticles. Moreover, we found that the yield was obviously affected by the amount of catalyst loaded. The use of a larger amount of catalyst (Table 4, entries 4 and 5) did not improve the product yield. The reaction was also carried out in the absence of Pd-NPs and it furnished only a trace (∼15%) amount of the desired product. Thus, the catalyst plays an important role in the product yield.
Entry |
Catalyst loading (mmol) |
Yield (%) |
1 |
No catalyst |
15 |
2 |
0.02 |
45 |
3 |
0.04 |
89 |
4 |
0.06 |
89 |
5 |
0.08 |
90 |
The reusability of the catalyst (Pd-NPs) was examined. It was found that the catalyst can be reused for another four consecutive runs under similar reaction conditions. After each run, the reaction mixture was centrifuged at 4000 rpm at room temperature for 3–5 min and filtered. Then the residue was washed with water and reused. The yield of 6a decreased slightly with reuse of the Pd nanoparticles (Fig. 3).
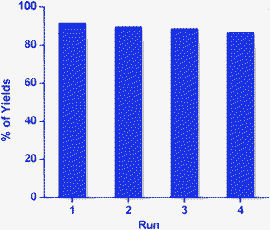 |
| Fig. 3 Reusability of the Pd NPs. | |
Experimental
Melting points were determined in open capillaries and are uncorrected. IR spectra were recorded on a Spectrum BX FT-IR, Perkin Elmer (υmax in cm−1) on KBr disks. 1H NMR and 13C NMR (400 MHz and 100 MHz respectively) spectra were recorded on a Bruker Avance II-400 spectrometer in CDCl3 (chemical shifts in δ with TMS as internal standard). Mass spectra were recorded on a Waters ZQ-2695. Transmission electron microscopy (TEM) was recorded on a JEOL JSM 100CX. XRD was recorded on a Bruker D8 XRD instrument SWAX. CHN were recorded on a CHN-OS analyzer (Perkin Elmer 2400, Series II). Silica gel G (E-mark, India) was used for TLC. Hexane refers to the fraction boiling between 60 °C and 80 °C.
A mixture of PdCl2 (0.045 mmol), TBAB (1.145 mmol) and Na2CO3 (3.435 mmol) in doubly distilled water (10 ml) was refluxed for 4 h. Then the reaction mixture was cooled to room temperature, centrifuged at 4000 rpm for 3 min and filtered. The residue was washed with distilled water (3 × 10 ml) and collected and dried. The particle size was determined by TEM and XRD.
Synthesis procedure for the synthesis of bis(heterocycyl)methane (6a–e, 7a–h, 8a–l, 9a–g)
A mixture of PdCl2 (0.045 mmol), TBAB (1.145 mmol) and Na2CO3 (3.435 mmol) in water (10 ml) was refluxed for 4 h. The C–H activated compound (4-hydroxy coumarin 1, indole 2, dimedone 3, or 3-methyl-1-phenyl-1H-pyrazol-5(4H)-one 4) (2 mmol) and an aryl aldehyde (1 mmol) 5 were added to it and the reaction was continued for the time mentioned in Table 1. After completion (TLC), the reaction mixture was cooled to room temperature, centrifuged at 4000 rpm for 3 min and filtered. The filtrate was extracted with ethyl acetate (3 × 10 ml) and the combined organic extract was washed with water (3 × 10 ml) and brine (10 ml) and dried over anhydrous Na2SO4. After removing the solvent, the crude product was purified by column chromatography over silica gel (60–120 mesh) using ethyl acetate and hexane as the eluent, to afford the pure product (6a–e, 7a–h, 8a–l, 9a–g).
Spectral data of compounds 6a, 7a, 8a and 9a
4-Hydroxy-3-((4-hydroxy-2-oxo-2H-chromen-3-yl)(phenyl)methyl)2H-chromen-2-one (6a).
IR (KBr): 3443, 1672, 1613 cm−1. 1H NMR (CDCl3, 400 MHz): δ = 11.46 (s, 1H), 11.23 (s, 1H), 7.16–7.99 (m, 13 H), 6.03 (s, 1H); ESI-MS: m/z 413 [M + H]+. Anal. Calcd for C25H16O6: C, 72.81; H, 3.91. Found: C, 72.92; H, 4.05.
3-((1H-Indol-3-yl)(phenyl)methyl)-1H-indole (7a).
IR (KBr): 3403, 2925, 1639 cm−1. 1H NMR (400 MHz, CDCl3): δ 5.81 (s, 1H), 6.57 (s, 2H), 6.94 (t, 2H, J = 7.6 Hz), 7.32–7.07 (m, 9H), 7.83 (br, s, 2H). 13C (100 MHz, CDCl3): δ 40.2, 111.1, 119.2, 119.6, 119.9, 121.9, 123.7, 126.2, 127.0, 128.3, 128.7, 136.6, 144.0; ESI-MS: m/z 323 [M + H]+. Anal. Calcd for C23H18N2: C, 85.68, H, 5.63; N, 8.69. Found: C, 85.57; H, 5.51; N, 8.52.
2,2′-Arylmethylene bis(3-hydroxy-5,5-dimethyl-2-cyclohexene-1-one) (8a).
IR (KBr): 3432, 2962, 1592 cm−1. 1H NMR (400 MHz, CDCl3): δ = 11.91 (s, 1H), 7.28–7.08 (m, 5H), 5.54 (s, 1H), 2.48–2.29 (m, 8H), 1.23 (s, 6H), 1.10 (s, 6H). 13C NMR (100 MHz, CDCl3): δ = 190.5, 189.4, 138.0, 128.2, 126.7, 125.8, 115.5, 47.0, 46.4, 32.7, 31.4, 29.6, 27.3; ESI-MS: m/z 369 [M + H]+. Anal. Calcd for C23H28O4: C, 74.97; H, 7.66. Found: C, 74.92; H, 7.73.
4,4′-(Arylmethylene)bis(1H-pyrazol-5-ols) (9a).
IR (KBr): 3450, 2925, 1600 cm−1. 1H NMR (CDCl3 + DMSO-d6, 400 MHz): δ = 2.26 (s, 6H), 4.84 (s, 1H), 7.09–7.39 (m, 11H), 7.66 (d, J = 8.0 Hz, 4H), 13.38 (brs, 2H). ESI-MS m/z 437 [M + H]+. Anal calcd for C27H24N4O2: C, 74.29; H, 5.54; N, 12.84. Found: C, 74.18; H, 5.42; N, 12.67.
Conclusions
In conclusion, we have demonstrated an efficient and simple method for the preparation of bis(heterocyclyl)methane derivatives using simple and readily available starting materials. Prominent among the advantages of this new method are novelty, operational simplicity, environmentally friendliness, good yields and reusability of the catalyst.
Acknowledgements
We thank the Department of Chemistry and Sophisticated Analytical and Instrumentation Facility (SAIF) of North-Eastern Hill University and IASC (Kolkata) for providing necessary facilities and the UGC, New Delhi for supporting this work under Special Assistance Programme (SAP). We are grateful to the referees for their valuable comments and constructive suggestions on our manuscript. We are thankful to Dr Rama Ranjan Bhatacharya and M. Saha acknowledges the financial support from Department of Biotechnology (Sanctioned no: BT/28/NE/TBP/2010).
References
-
(a) D. Astruc, Inorg. Chem., 2007, 46, 1884 CrossRef CAS;
(b) L.-S. Zhong, J.-S. Hu, Z.-M. Cui, L.-J. Wan and W.-G. Song, Chem. Mater., 2007, 19, 4557 CrossRef CAS;
(c) D. Astruc, F. Lu and J. R. Aranzaes, Angew. Chem., Int. Ed., 2005, 44, 7852 CrossRef CAS.
- C. Burda, X. Chen, R. Narayana and M. A. El-Sayed, Chem. Rev., 2005, 105, 1025 CrossRef CAS.
- P. V. Kamat, J. Phys. Chem. B, 2002, 106, 7729 CrossRef CAS.
- F. Frederix, J. Friedt, K. Choi, W. Laureyn, A. Campitelli, D. Mondelares, G. Maes and G. Borghs, Anal. Chem., 2003, 75, 6894 CrossRef CAS.
- S. Praharaj, S. Nath, S. K. Ghosh, S. Kundu and T. Pal, Langmuir, 2004, 20, 9889 CrossRef CAS.
- T. Itoh and T. Mase, Org. Lett., 2007, 7, 3687 CrossRef.
- T. Sone, Y. Ohba and R. Watanabe, Bull. Chem. Soc. Jpn., 1989, 62, 1346 CrossRef CAS.
-
(a) V. K. Gupta, M. K. Pal and A. K. Singh, Talanta, 2009, 79, 528 CrossRef CAS;
(b) Z. Hu, J. L. Atwood and M. P. Cava, J. Org. Chem., 1994, 59, 8071 CrossRef CAS.
-
(a) J. Zheng, R. Wen, X. Luo, G. Lin, J. Zhang, L. Xu, L. Guo and H. Jiang, Bioorg. Med. Chem. Lett., 2006, 16, 225 CrossRef CAS;
(b) S. Sasaki, N. Ishibashi, T. Kuwamura, H. Sano, M. Matoba, T. Nisikawa and M. Maeda, Bioorg. Med. Chem. Lett., 1998, 8, 2983 CrossRef CAS.
-
(a) T. Zhao, Z. Wei, Y. Song, W. Xu, W. Hu and D. Zhu, J. Mater. Chem., 2007, 17, 4377 RSC;
(b) T. Benincori, S. Rizzio and F. Sannicolo, Macromolecules, 2003, 36, 5114 CrossRef CAS.
- A. R. Katritzky, L. Xie and W.-Q. Fan, J. Org. Chem., 1993, 58, 4376 CrossRef CAS.
- D. A. Stetsenko, E. N. Lubyako, V. K. Potapov, T. L. Azhikima and E. D. Sverdlov, Tetrahedron Lett., 1996, 37, 3571 CrossRef CAS.
-
(a) F. Cherioux, L. Guyard and P. Audebert, Adv. Mater., 1998, 10, 1013 CrossRef CAS;
(b) S. Brasselet, F. Cherioux, P. Audebert and J. Zyss, Chem. Mater., 1999, 11, 1915 CrossRef CAS.
-
(a) E. Vogel, Pure Appl. Chem., 1990, 62, 557 CrossRef CAS;
(b) E. Vogel, N. Lux, J. Dorr, T. Pelster, T. Berg, H.-S. Bohm, F. Behrens, J. Lex, D. Bremm and G. Hohlneicher, Angew. Chem., Int. Ed., 2000, 39, 1101 CrossRef CAS;
(c) J. L. Sessler and D. Seidel, Angew. Chem., Int. Ed., 2003, 42, 5134 CrossRef CAS.
- K. Singh, S. Sharma and A. Sharma, J. Mol. Catal. A: Chem., 2011, 34, 347 Search PubMed.
- Z. N. Siddiqui and F. Farooq, Catal. Sci. Technol., 2011, 1, 810 Search PubMed.
- A. K. Maiti and P. Bhattacharyya, J. Chem. Res. (S), 1997, 424 RSC.
- G. Babu, N. Sridhar and P. T. Perumal, Synth. Commun., 2000, 30, 1609 CrossRef CAS.
- T.-S. Jin, J.-S. Zhang, A.-Q. Wang and T.-S. Li, Synth. Commun., 2005, 35, 2339 CrossRef CAS.
- P. K. R. Phatagav, V. S. Padalkar, V. D. Gupta, V. S. Patil, P. G. Umape and N. Sekar, Synth. Commun., 2012, 42, 1349 CrossRef.
- S. A. R. Mulla, A. Sudalal, M. Y. Pathan, S. A. Siddique, S. M. Inamdar, S. S. Chavan and R. S. Reddy, RSC Adv., 2012, 2 10.1039/c2ra00849a.
- Y. R. Butler and A. G. Coney, Chem. Rev., 2010, 110, 6302 CrossRef.
- M. Saha and A. K. Pal, Tetrahedron Lett., 2011, 52, 4872 CrossRef CAS.
- D. Srimani, A. Bej and A. Sarkar, J. Org. Chem., 2010, 75, 4296 CrossRef CAS.
-
(a) L. Adak, K. Chattopadaya and B. C. Ranu, J. Org. Chem., 2009, 74, 3928 CrossRef;
(b) M. T. Reetz and E. Westerman, Angew. Chem., Int. Ed., 2000, 39, 165 CrossRef CAS;
(c) M. T. Reetz and M. Masse, Adv. Mater., 1999, 11, 773 CrossRef CAS;
(d) B. C. Ranu, K. Chattopadhyay and L. Adak, Org. Lett., 2007, 9, 4595 CrossRef CAS;
(e) T. Jeffery, Tetrahedron, 1996, 52, 10113 CrossRef CAS.
-
(a) R. M. Crooks, M. Zhao, L. Sun, V. Chechik and L. K. Yeung, Acc. Chem. Res., 2001, 34, 181 CrossRef CAS;
(b) M. Moreno-Manas and R. Pleixats, Acc. Chem. Res., 2003, 36, 638 CrossRef CAS.
- H. Hagiwara, N. Fujimoto and T. Suzuki, Heterocycles, 2000, 53, 549 CrossRef CAS.
- H. Mehrabi and H. Abusaidi, J. Iran. Chem. Soc., 2010, 7, 890 CrossRef CAS.
- A. Davoodnia, Bull. Korean Chem. Soc., 2011, 32, 4286 CrossRef CAS.
- M. Kidwai, V. Bansal, P. Mothsra, S. Saxena, R. K. Somvanshi, S. Dey and T. P. Singh, J. Mol. Catal. A: Chem., 2007, 268, 76 CrossRef CAS.
- K. Nawaz, M. A. Munawar and M. Siddiq, J. Chem. Soc. Pak., 1991, 13, 273 Search PubMed.
- P. Singh, P. Kumar, A. Katyal, R. Kalra, S. K. Dass, S. Prakash and R. Chandra, Catal. Lett., 2010, 134, 303 CrossRef CAS.
- V. Padalkar, K. Phatangare, S. Takale, R. Pisal and A. Chaskar, J. Saudi Chem. Soc., 2012 DOI:10.1016/j.jscs.2011.12.015.
|
This journal is © The Royal Society of Chemistry 2012 |
Click here to see how this site uses Cookies. View our privacy policy here.