DOI:
10.1039/C2RA21713A
(Paper)
RSC Adv., 2012,
2, 10359-10364
Retracted Article: Enhanced adsorption of ionizable aromatic compounds on humic acid-coated carbonaceous adsorbents
Received
6th August 2012
, Accepted 5th September 2012
First published on 5th September 2012
Abstract
Adsorption of ionizable aromatic compounds (IACs) such as 1-naphthylamine and 1-naphthol on Humic acid (HA)-coated graphene oxide nanosheets (GONs), multiwalled carbon nanotubes (MWCNTs), activated carbon (AC), and flake graphite (FG) were investigated by the batch techniques. The adsorption of 1-naphthylamine on four HA-coated carbonaceous adsorbents was weakly depended on pH, whereas the adsorption of 1-naphthol on these HA-coated carbonaceous adsorbents evidently increased with increasing pH from 2.0 to 8.0. The maximum adsorption capacity of HA-coated carbonaceous adsorbents for IACs was in the order of GONs≫MWCNTs>AC>FG, indicating that the GONs can be regarded as a suitable material for the preconcentration and removal of IACs from aqueous solution in environmental pollution management. The main adsorption mechanism between carbonaceous adsorbents and IACs is the hydrophobic effect. This observation is of great implication for the removal of IACs by HA-coated carbonaceous adsorbents in environmental applications.
1. Introduction
Ionizable aromatic compounds (IACs), widely used as an industrial intermediate, are organic contaminants due to their potential harmf to human health such as carcinogens and acute toxicity. Accurate predictions of transport, distribution, accumulation and fate of IACs in the environment has been developed in recent years.1–7 Ro et al.1 put forward that the continuous removal efficiency of 1-naphthylamine was greater than 90% using a fluidized-bed reactor with bio augmentation of slightly contaminated groundwater. Afterwards, Sheng et al.2 further demonstrated that the adsorption behaviors of IACs such as 1-naphthlyamine and 1-naphthol on multiwalled carbon nanotubes (MWCNTs) can be described by Langmuir and Freundlich models very well, and found that MWCNTs exhibited a large adsorption capacity for 1-naphthylamine and 1-naphthol compared to the other IACs, such as phenol. To the author's best knowledge, there is little information available in the literature about the comparison of 1-naphthylamine and 1-naphthol adsorption on four HA-coated carbonaceous adsorbents such as graphene oxide nanosheets (GONs), MWCNTs, activated carbon (AC) and flake graphite (FG).
Graphene, a two-dimensional allotrope of carbon atoms densely packed in a honeycomb crystal lattice, has sparked intense interest in multidisciplinary studies such as physics, chemistry, materials and environmental science since it was found in 2004 by using a simple mechanical method.8–12 Graphene has been used to focus on particular environmental applications such as removing gases,13,14 inorganic contaminants,15–17 radioclides,18 and organic matter19,20 owing to its considerable specific surface area (∼2620 m2 g−1). Sun et al.18 found that the maximum adsorption capacity of Eu(III) on GONs at pH ∼ 6.0 and T = 298 K was about 175.0 mg g−1. In this study, it has been demonstrated that GONs can be a promising adsorbent for the removal of radionuclides by formation of the strong inner-sphere surface complexation.
Accurate prediction and remediation of IACs in (sub)surface environments and development of materials suitable for encapsulation and disposal of IACs requires the fundamental knowledge of adsorption characteristics of IACs to be investigated as well as the knowledge of the type of adsorbents and of their characteristics onto various solid–water interfaces, thereby the adsorption of IACs on four carbonaceous adsorbents have been investigated in this study with the aim of examining the effect of various carbonaceous adsorbents on the adsorption of IACs from aqeuous solution. Four carbonaceous adsorbents were modified by humic acid (HA) in order to enhance the amount of oxygen-containing functional groups on the adsorbent surface. The objectives of this paper were to (1) synthesize HA-coated GON and MWCNT composites and characterize their microscopic and macroscopic surface properties by using scanning electron microscopy (SEM), X-ray diffraction (XRD), Fourier transform infrared (FT-IR) and potentiometric titration techniques; (2) investigate the effects of pH and initial Eu(III) concentrations on 1-naphthylamine and 1-naphthol adsorption on four HA-coated carbonaceous adsorbents by batch technique; (3) discuss the adsorption mechanism between IACs and four HA-coated carbonaceous adsorbents under various pH conditions. This study highlighted the extensive applicability of GONs in the removal of IACs from large volumes of aqueous solutions.
2. Experimental
2.1. Materials
The GONs and MWCNTs were synthesized by modified Hummers method21 and the chemical vapour deposition (CVD) method22 respectively. Briefly, the GONs were obtained from the strong oxidization of flake graphite by using KMnO4 and concentrated H2SO4 under ultrasonic conditions, then the suspension was washed several times and centrifuged at 18
000 rpm for 60 min. The MWCNTs were prepared by CVD of acetylene at 760 °C under H2(g) conditions using Ni–Fe nanoparticles as catalysts, then the desired material was obtained by adding 3.0 mol L−1 HNO3 solution to remove the amorphous carbon and the catalysts Ni and Fe impurities. More details on preparation of GONs and MWCNTs can be found in our previous literature elsewhere.2,15,16,18,19,22 The other two comparative adsorbents of FG and AC supplied by Nanotech Chemicals (Shanghai, China), were used in this research without the further purification.
HA was extracted from the soil of Hua-Jia county (Gansu, China), and was characterized in previous literature in detail.23 HA-coated GONs, MWCNTs, AC and FG were obtained by mixing the HA suspension and four carbonaceous adsorbents under vigorously stirring conditions for 24 h.
2.2. Characterization
The SEM images of GONs and MWCNTs were obtained on a field emission scanning electron microscope (FEI-JSM 6320F). The GONs, MWCNTs, AC and FG were mounted for XRD analysis with a Rapid-II powder diffractometer (D/RAPID II, Japan) using Cu-Kα radiation (λ = 0.15406 nm) with a 0.02° step size and a 2 s step time. The MWCNTs and GONs were also investigated using Fourier transform infrared (FTIR) spectroscopy (FTIR, PE2000) in pressed KBr pellets. Briefly, the samples were washed three times with ethanol, and dried at 333 K for 24 h. The FTIR cell was flushed with N2 gas for 10 min prior to scanning to remove atmospheric water vapour and CO2 from the spectrophotometer. The spectral resolution was set to 1 cm−1, and 150 scans were collected for each spectrum. The potentiometric acid–base titration was performed using a computer-controlled titration system (DL50 Automatic Titrator, Mettler Toledo). Briefly, these carbonaceous adsorbents were spiked into 0.01 mol L−1 NaClO4 background electrolyte at room temperature, and purged with Ar(g) to exclude atmospheric CO2 (g). The initial pH of suspension was adjusted to acidic conditions (pH∼3.0) by adding HClO4 solution under vigorous stirring, and then the suspension was slowly titrated to alkaline conditions (pH∼11.0) with a nomalized NaOH titrant at a variable increment. The data sets of pH versus the net consumption of H+ or OH− were used to obtain intrinsic acidity constants.
The pH-dependent adsorptions and adsorption isotherm experiments were conducted with aluminum foil-lined Teflon screw caps to prevent degradation of IACs during adsorption experiments. An aliquot of the suspension (the range of solid/liquid ratio was from 0.01 to 1.0 g L−1) with background electrolyte (0.01 mol L−1 NaClO4) were spiked into a batch of 10 mL glassed centrifuge tubes. The preliminary kinetics indicated that 1 day was adequate for the suspenstion to obtain equilibrium (data no shown), therefore the individual effluent samples were shaken for 2 days to achieve a sufficiently steady-state condition. The pH of suspensions were adjusted to be in the range from 2.0 to 8.0 by adding negligible volume of 0.01 mol L−1 HClO4 or 0.1 mol L−1 NaOH solution using a glassy combination electrode (pH/conductivity meter, Fisher Scientific). The suspension was filtered in series through 0.2 μm syringe filter after centrifuging it at 3800 rpm for 10 min. The batch adsorption isotherms were prepared using the same procedures as that in batch pH experiments and equilibrated for 2 days with various IAC concentrations. The concentration of 1-naphthylamine and 1-naphthol were detected by variable UV-VIS spectrophotometer operated at 212 nm and 320 nm, respectively. The detection limit using this protocol was approximately 0.05 mg L−1.
3. Results and discussion
3.1. Characterization
The characterization results of SEM, XRD and FT-IR of HA-coated four carbonaceous adsorbents are shown in Fig. 1, and surface properties of carbonaceous adsorbents are also tabulated in Table 1. The SEM images (Fig. 1A and 1B) show that the GONs are closely agglomerated together by randomly and thin aggregated sheets, but the curled and entangled tube-like shape MWCNTs are irregularly accumulated. For FG, AC and MWCNTs, the diffraction peak at 2θ = 26° (d = 0.34 nm) is related to the basal spacing (002) of the graphite plane. For GONs, however, the only weak and broad peak is observed at 2θ = 10° (d = 0.86 nm). The increasing c-axis spacing is due to the occurrence of massive oxygen-containing functional groups on the surface of the GONs.19 No change in XRD patterns for HA-coated and pristine carbonaceous adsorbents (data not shown) are observed in the experimental system. According to the FTIR spectrum (Fig. 1D), MWCNTs have characteristic bands of C
O at 1740 cm−1, C
C at 1625 cm−1, C–O–C at 1450 cm−1, C–C at 1040 and 1100 cm−1. For GONs, however, the characteristic bands of C–O at 1220 cm−1, C
C at 1625 cm−1 and C
O at 1740 cm−1 were observed. According to the results of the potentiometric titration (Table 1), the surface reactive site density of HA-coated GONs (95.82 mmol g−1) is larger than that of HA-coated FG (0.142 mmol g−1). It is observed that the surface reactive site density of HA-coated GONs is more than an order of magnitude larger than that of pristine GONs (2.36 mmol g−1).16 Theoretically, the pHPZC of adsorbents were obtained from the zero points at surface charge (Q), but there are a large number of functional groups on the graphene surface, so the point of net zero charge (pHPNZC) rather than point of zero charge (pHPZC) is employed to depict the surface property of adsorbents exactly. The pHPNZC is corroborated to be 7.1 for GONs at I = 0.01 mol L−1 NaClO4, which is slightly bigger than that of the other carbonaceous adsorbents. The change of surface acidity coefficients (ΔpK = pK2−pK1) of carbonaceous adsorbents are also computed in Table 1. The larger ΔpK values of FG (∼2.4) established predominant distribution of reactive sites on outer surface sites of bulk particles rather than inner surface sites compared to AC, MWCNTs and GONs.
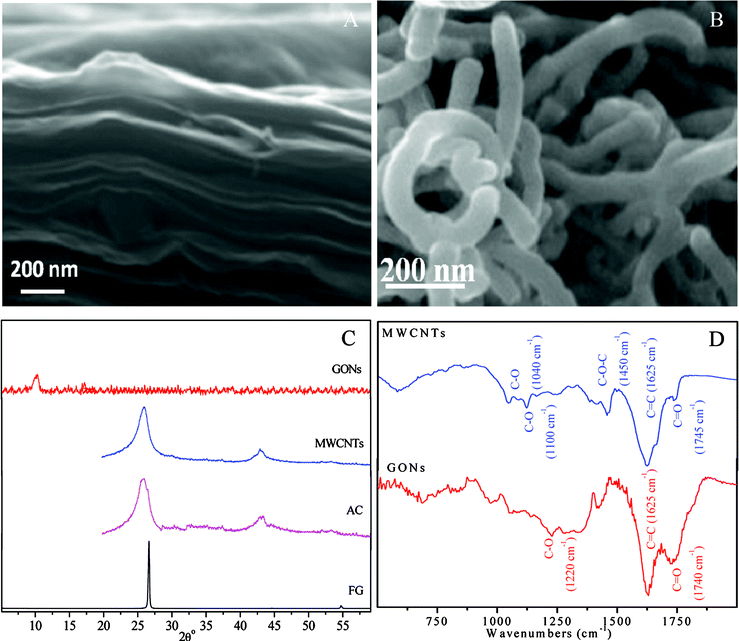 |
| Fig. 1 The characterization of carbon materials. (A) and (B): SEM images of GONs and MWCNTs, respectively; (C): XRD patterns of carbon materials; (D): the FTIR of GONs and MWCNTs. | |
Table 1 Surface density, acidity constants (pK1 and pK2), pHPZNC and specific surface area for AC, EG, MWCNTs and GONs
Samples |
Surface density (mmol g−1) |
pK
1
|
pK
2
|
pH
PNZC
|
S
BET
(m2 g−1) |
FG |
0.142 |
3.3 |
5.7 |
4.5 |
12.4 |
AC |
0.745 |
3.9 |
6.1 |
5.0 |
65 |
MWCNTs |
21.24 |
4.2 |
6.8 |
5.5 |
72 |
GONs |
95.82 |
6.8 |
7.4 |
7.1 |
120 |
3.2. Breakthrough of IACs adsorption
It is found that environmental variables such as pH can potentially affect the adsorption behaviors. The adsorption of 1-naphthylamine and 1-naphthol on FG, AC, MWCNTs and GONs as a function of pH at constant temperature and ionic strength conditions were investigated in Fig. 2. The adsorption of 1-naphthylamine on four carbonaceous adsorbents is independent of pH, whereas 1-naphthol adsorption increases with increasing pH from 2.0 to 8.0. The maximum adsorption of 1-naphthylamine and 1-naphthol on GONs is observed compared to the other carbonaceous adsorbents, which is approximately five times that of FG. In contrast, FG exhibited much lower adsorption affinity for the 1-naphthylamine molecule mainly due to the molecular sieving effect.6 It was postulated that the less polar 1-naphthylamine is absorbed by physical forces and possibly the formation of coordination complexes with exchangeable cations, while the 1-naphthol is absorbed by a combination of protonation and consequent ion exchange reactions, the relative importance of each process being determined by pH, exchangeable cation and the characteristics of the adsorbate molecule. In an attempt to account for the adsorptive differences between 1-naphthylamine and 1-naphthol, the two main reasons are that: 1) It is found that 1-naphthylamine is a less potent adsorbate due to the low polarity of 1-naphthylamine relative to 1-naphthol, and thus 1-naphthylamine exhibited as a weak competitor against water for adsorption on adsorbent surfaces; 2) Lesser adsorption of 1-naphthylamine might be ascribed to an increase of electrostatic repulsion, carboxylic groups weakening the π–π interaction and water adsorption. The extraordinarily strong adsorption affinity makes flake graphite, in particular graphene, promising candidates as special adsorbents for IACs in wastewater treatment.
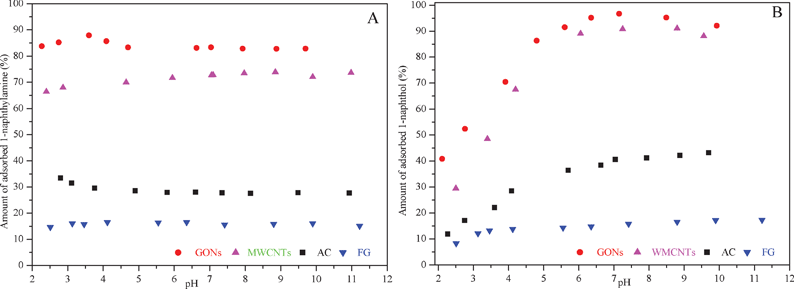 |
| Fig. 2 The effect of pH on the adsorption of 1-naphthylamine (A) and 1-naphthol (B) on FG, AC, MWCNTs and GONs, CIAC = 100 mg L−1, T = 293 K, I = 0.01 mol L−1 NaClO4. | |
Adsorption isotherms are employed to depict the distribution of IACs between a solid sorbent and the solution in equilibrium over a range of concentrations at constant temperature. For four carbonaceous adsorbents, it is observed that the adsorption amount for 1-naphthylamine is higher than 1-naphthol (Fig. 3). It can be seen that adsorption isotherms appear to be linear at low concentration, but tend to become increasingly nonlinear as the concentration of chemicals in the aqueous phase increases. The maximum adsorption capacity was in the order of GONs≫MWCNTs>AC>FG. The adsorption isotherms of 1-naphthylamine and 1-naphthol on the four carbonaceous adsorbents were fitted by Langmuir and Freundlich models respectively, and selected parameters derived from the Langmuir and Freundlich models are listed in Table 2. It can be seen that adsorption isotherms of 1-naphthylamine and 1-naphthol on four carbonaceous adsorbents are consistent with the Freundlich model very well in terms of the correlation coefficient (R2). As shown in Table 2, the adsorption capacity of GONs (qmax = 434.78 mg g−1) calculated from the Langmuir model is more than thirty times as much as FG (qmax = 23.36 mg g−1). The maximum adsorption capacity of HA-coated GONs (qmax = 434.78 mg g−1 for 1-naphthol) is higher than that of pristine GONs (qmax = 346 mg g−1 for 1-naphthol).19 Sheng et al.2 also found that the maximum adsorption capacity of MWCNTs for 1-naphthlyamine and 1-naphthol at 298 K were 58.82 and 54.4 mg g−1 respectively, which are lower than those of HA-coated MWCNTs (qmax = 90.91 and 97.091 mg g−1 for 1-naphthlyamine for 1-naphthol, respectively). The enhanced adsorption of HA-coated carbonaceous adsorbents could be due to the occurrence of more oxygen-containing functional groups by coating HA on these carbonaceous adsorbents. It has been documented previously that HA molecules present long flexible aliphatic chains in the molecule, which would show high affinity to IACs probably due to strong electrostatic interactions. The delicate differences in maximum adsorption capacity for the adsorption of 1-naphthylamine and 1-naphthol on FG and AC are observed, whereas selective adsorption of 1-naphthylamine and 1-naphthol on MWCNTs and GONs are observed. The results of the present work indicate that the adsorption selectivity and efficiency can be improved through specific carbonaceous adsorbents.
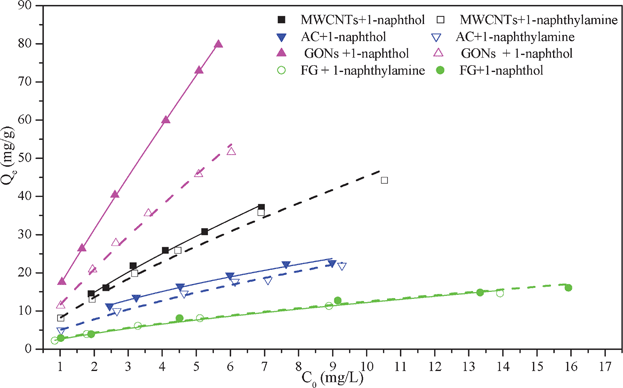 |
| Fig. 3 The isotherms of 1-naphthylamine and 1-naphthol adsorption on AC, MWCNTs, FG and GONs, T = 293 K, pH = 5.0 ± 0.1, I = 0.01 mol L−1 NaClO4. | |
Adsorbents |
Adsorbate |
Adsorption isotherms |
Langmuir |
Freundlich |
K
a
(L mg−1) |
q
max
(mg g−1) |
R2 |
lnK
F
(mg1−n·Ln·g−1) |
1/n |
R2 |
1-naphthylamine |
FG |
9.242 |
25.253 |
0.9813 |
1.028 |
0.6527 |
0.9909 |
|
AC |
7.29 |
38.167 |
0.9836 |
1.607 |
0.6782 |
0.9955 |
|
MWCNTs |
11.04 |
90.91 |
0.9830 |
2.10 |
0.7443 |
0.9956 |
|
GONs |
14.66 |
178.57 |
0.9845 |
2.455 |
0.8501 |
0.9961 |
|
|
|
|
|
|
|
|
1-naphthol |
FG |
8.9556 |
23.36 |
0.9849 |
0.9546 |
0.675 |
0.9957 |
|
AC |
5.836 |
38.17 |
0.9902 |
1.950 |
0.555 |
0.9887 |
|
MWCNTs |
11.223 |
97.09 |
0.9662 |
2.181 |
0.751 |
0.9935 |
|
GONs |
25.304 |
434.78 |
0.9834 |
2.8246 |
0.901 |
0.9999 |
Differences between adsorption of IACs on GONs and adsorption on the other carbonaceous adsorbents such as FG, AC and MWCNTs have been examined over a relatively wide range of pH from 2.0 to 12.0. It is determined that the extent of adsorption of IACs on the four carbonaceous adsorbents depends on the physical and chemical properties of the adsorbents. The adsorption magnitude of organic compounds with different chemical character is governed by the degree of water solubility, the dissociation constant of the adsorbate, and the pH of the aqueous solution. Numerous studies have shown that the adsorption of organic chemicals on carbon materials24 were corroborated by water adsorption, dispersive–repulsive interactions, and physicochemical properties of adsorbate and adsorbents such as pore structure, functional groups, pHPZC, and specific surface area. When carbonaceous adsorbents were fully hydrated, adsorption of IACs by adsorbents becomes relatively insignificant presumably because water is preferentially adsorbed by adsorbents. Therefore, the main adsorption mechanism between carbonaceous adsorbents and IACs is the hydrophobic effect.
It also has been widely accepted that the strong adsorption of IACs on the carbonaceous adsorbents were mainly attributed to the π–π electron-donor–acceptor (EDA) interaction between IACs and the highly polarizable graphene sheets.6,24,25–27 The carboxylic groups on the surfaces of the carbonaceous adsorbents can act as electron withdrawing groups localizing electron from π–system of graphene, which might be expected to interfere with π–π dispersion forces between the aromatic ring of IACs and the graphitic structure of carbonaceous adsorbents. Previous studies on the adsorption of aromatics on AC suggested that surface oxygen complexes increased the affinity of water to activated carbon, which reduced the accessibility for aromatic adsorbates, thus leading to the lower adsorption capacity. This phenomenon has been observed by many researchers.25,28 Chen et al.29 have proposed that the strong adsorptive interaction between hydroxyl-substituted aromatics and CNTs was mainly due to the electron-donating effect of the hydroxyl group, which caused a strong EDA interaction between the adsorbates and the π-electron depleted regions on the graphene surfaces of the CNTs. In addition to the EDA interaction, van der Waals force and H-bonding are likely to play an extra important role in the mechanism contributing to the strong adsorption of 1-naphthylamine, especially on the O-functionality-abundant carbon nanotubes. Furthermore, electrostatic interaction may depend on other important factors that are responsible for the adsorption of IACs on these carbonaceous adsorbents.
4. Conclusion
In this paper, GONs and MWCNTs were synthesized and characterized by SEM, XRD, FT-IR and potentiometric titration. The adsorption of 1-naphthylamine on FG, AC, MWCNTs and GONs is weakly dependent of pH, whereas 1-naphthol adsorption increases with increasing pH from 2.0 to 8.0. The adsorption of 1-naphthylamine on four carbonaceous adsorbents is higher than 1-naphthol. The maximum adsorption capacity of HA-coated carbonaceous adsorbents for IACs was in the order of GONs≫MWCNTs>AC>FG. The interaction mechanism between carbonaceous adsorbents and IACs was determined to depend on the hydrophobic effect, as well as EDA interaction, van der Waals force, H-bonding and electrostatic interaction. According to documented investigation, it is reported that the adsorption capacity of GONs is the highest than any of various adsorbents currently used. One can see that GONs may be the most suitable materials for the removal of IACs in environmental pollution cleanup. Although GONs are relatively more expensive than other natural adsorbents, GONs will be synthesized in large scale and at a low price in the near future with the rapid development of technology. The high adsorption capacity of GONs for IACs assures their application in the real world. This paper highlights the possible application of GONs in the removal of IACs from large volumes of aqueous solutions in environmental pollution cleanup.
Acknowledgements
Financial supports from NSFC (21207135, 21207136, 21071147, 21071107, 20971126), 973 projects (2011CB933700), and Natural Science Foundation of Anhui Province (Grant No:1208085QB32) are acknowledged.
References
- K. S. Ro, R. W. Babcock and M. K. Stenstrom, Water Res., 1997, 31, 1687–1693 CrossRef CAS.
- G. D. Sheng, D. D. Shao, X. M. Ren, X. Q. Wang, J. X. Li, Y. X. Chen and X. K. Wang, J. Hazard. Mater., 2010, 178, 505–516 CrossRef CAS.
- K. Fu and R. G. Luthy, J. Environ. Eng., 1986, 112, 346–366 CrossRef.
- J. J. Hassett and W. L. Banwart, Soil Sci. Soc. Am. Madison, WI, 1989, 31–44 CAS.
- D.H. Lin and B. S. Xing, Environ. Sci. Technol., 2008, 42, 7254–7259 CrossRef CAS.
- L. L. Ji, W. Chen, L. Duan and D. Q. Zhu, Environ. Sci. Technol., 2009, 43, 2322–2327 CrossRef CAS.
- J. Wei, R. Zhu, J. Zhu, F. Ge, P. Yuan, H. He and C. Ming, J. Hazard. Mater., 2009, 166, 195–199 CrossRef CAS.
- K. S. Kim, Y. Zhao, H. Jang, S. Y. Lee, J. M. Kim, K. S. Kim, J. H. Ahn, P. Kim, J. Y. Choi and B. H. Hong, Nature, 2009, 457, 706–710 CrossRef CAS.
- Y. B. Zhang, Y. W. Tan, H. L. Stormer and P. Kim, Nature, 2005, 438, 201–204 CrossRef CAS.
- A. K. Geim and K. S. Novoselov, Nat. Mater., 2007, 6, 183–191 CrossRef CAS.
- C. Lee, X. D. Wei, J. W. Kysar and J. Hone, Science, 2008, 321, 385–388 CrossRef CAS.
- T. Ohta, A. Bostwick, T. Seyller, K. Horn and E. Rotenberg, Science, 2006, 313, 951–954 CrossRef CAS.
- B. Huang, Z. Y. Li, Z. R. Liu, G. Zhou, S. G. Hao, J. Wu, B. L. Gu and W. H. Duan, J. Phys. Chem. C, 2008, 112, 13442–13446 CAS.
- H. G. Romero, P. Joshi, A. K. Gupta, H. R. Gutierrez, M. W. Cole, S. A. Tadigadapa and P. C. Eklund, Nanotechnology, 2009, 20, 245501–245508 CrossRef.
- G. X. Zhao, X. M. Ren, X. Gao, X. L. Tan, J. X. Li, C. L. Chen, Y. Y. Huang and X. K. Wang, Dalton Trans., 2011, 40, 10945–10952 RSC.
- G. X. Zhao, J. X. Li, X. M. Ren, C. L. Chen and X. K. Wang, Environ. Sci. Technol., 2011, 45, 10454–10462 CrossRef CAS.
- S. Vasudevan and J. Lakshmi, RSC Adv., 2012, 2, 5234–5242 RSC.
- Y. B. Sun, Q. Wang, C. L. Chen, X. L. Tan and X. K. Wang, Environ. Sci. Technol., 2012, 46, 6020–6027 CrossRef CAS.
- G. X. Zhao, L. Jiang, Y. D. He, J. X. Li, H. L. Dong and X. K. Wang, Adv. Mater., 2011, 23, 3959–3963 CrossRef CAS.
- J. D. Wuest and A. Rochefort, Chem. Commun., 2010, 46, 2923–2325 RSC.
- W. S. Hummers and R. E. Offeman, J. Am. Chem. Soc., 1958, 80, 1339–1339 CrossRef CAS.
- X. K. Wang, C. L. Chen, W. P. Hu, A. P. Ding, D. Xu and X. Zhou, Environ. Sci. Technol., 2005, 39, 2856–2860 CrossRef CAS.
- Z. Y. Tao, J. Zhang and J. Zhai, Anal. Chim. Acta, 1999, 395, 199–203 CrossRef CAS.
- D. Q. Zhu and J. J. Pignatello, Environ. Sci. Technol., 2005, 39, 2033–2041 CrossRef CAS.
- M. Franz, H. A. Arafat and N. G. Pinto, Carbon, 2000, 38, 1807–1019 CrossRef CAS.
-
R. P. Schwarzenbach, P. M. Gschwend and D. M. Imboden, Wiley-Inter-science, New York 2003, 2nd ed, 52–78 Search PubMed.
- K. Yang and B. Xing, Environ. Pollut., 2007, 145, 529–537 CrossRef CAS.
- A. S. Gnihotri and M. Rostam-Abadi, Environ. Sci. Technol., 1968, 2, 291–297 CrossRef.
- W. Chen, L. Duan, L. L. Wang and D. Q. Zhu, Environ. Sci. Technol., 2008, 42, 6862–6868 CrossRef CAS.
|
This journal is © The Royal Society of Chemistry 2012 |
Click here to see how this site uses Cookies. View our privacy policy here.