DOI:
10.1039/C4RA09876E
(Paper)
RSC Adv., 2014,
4, 60451-60459
Facile assembly of 1D multinuclear Agn (n = 11, 11, 12) alkynyl chains with CF3COO−/CH3COO− as the auxiliary ligand†
Received
5th September 2014
, Accepted 28th October 2014
First published on 29th October 2014
Abstract
Three 1D multinuclear silver(I) alkynyl compounds, [Ag11(C
CtBu)6(CF3COO)5(CH3OH)]n (1), [Ag11(C
CtBu)7(CH3COO)4]n (2) and [Ag12(C
CtBu)7(CF3COO)5·H2O]n (3), constructed from AgC
CtBu ligand and CF3COO−/CH3COO− auxiliary ligand, have been obtained by changing the type and source of the auxiliary ligand as well as adjusting the pH value in different solvents. The synthesis of 1–3 indicates that this kind of 1D multinuclear Agn (n = 11, 11, 12) alkynyl chain can be isolated through a facile one-pot process. Among these, an infinitely extended 2D supramolecular network is fabricated from 1D chains of 1 via hydrogen bonds, while 3 crystallizes in the chiral space group P21. In addition, the solid state optical diffuse reflection spectra and luminescence spectra of 1–3 have been obtained. Compound 2 is taken as an example to study the electrochemical behaviour of this kind of compound, and the results indicate that 2-CPE possesses good electrocatalytic activity toward the reduction of nitrite.
Introduction
The simple coinage-metal (Cu, Ag, and Au) alkynyl compounds originally studied by Nast and Schindel et al. half a century ago have been widely discussed up to now.1 Simple coinage-metal (Cu, Ag, and Au) alkynyl compounds attract a lot of attention, which not only stems from the structural diversity that they show,2 but also originates from their promising applications as luminescent materials and rigid-rod molecular wires.3 Alkynyl ligands displaying flexible coordination environments can serve as good σ-donors and modest π-acceptors4 and metal–alkynyl σ, π and mixed (σ, π) bonds will be formed between metal centres and alkynyl ligands.5 Silver(Ι) alkynyl compounds have been actively researched among coinage-metal (Cu, Ag, and Au) alkynyl compounds because silver metal has an acceptable price, versatile coordination modes, and a high binding ability to donor ligands.6 Meanwhile, multiple argentophilic interactions exist in the central Ag skeletons during the formation of multinuclear silver(Ι) alkynyl compounds.7 Currently, several active groups, Mak, Wang, Zhao, and Jansen et al. are making contributions to the development and flourishing of multinuclear silver(Ι) alkynyl compounds. Many cases of multinuclear silver(Ι) alkynyl compounds constructed from tBuC
C− ligand and stabilised by other auxiliary ligands with a great diversity of configurations have been documented.8–11
The crystal engineering of supramolecular architectures constructed by metal and organic building blocks has also been rapidly researched.12 Among these, the rational design and synthesis of new extended supramolecular networks with higher dimensionalities by the assembly of low dimensional coordination complexes/polymers built by coordinative covalent bonds via hydrogen bonding interactions are of great interest.13 In fact, silver(Ι) alkynyl compounds have also been proven to be a better system for building supramolecular architectures through hydrogen bonding interactions. It is worth mentioning that Mak and Zhao et al. have reported eight coordination and hydrogen-bonded networks with the tert-Bu-C
C⊃Agn (n = 4, 5) supramolecular synthon and so on.14
Initially, in order to construct multinuclear silver(Ι) alkynyl clusters induced by polyanions in our group,15,16 many attempts were made through changing the type and source of the auxiliary ligand CF3COO−/CH3COO− along with introducing several polyoxometalates (POMs) as template reagents in different solutions. Contrary to expectations, three 1D multinuclear silver(I) alkynyl compounds, [Ag11(C
CtBu)6(CF3COO)5(CH3OH)]n (1), [Ag11(C
CtBu)7(CH3COO)4]n (2) and [Ag12(C
CtBu)7(CF3COO)5·H2O]n (3) have been obtained through a facile one-pot process. An extended 2D supramolecular network is fabricated from 1D covalently-bonded chains of 1 through hydrogen bonds between the coordinated tBuC
C− ligands and CF3COO− auxiliary ligands, while compound 3 crystallizes in the chiral space group P21. Herein, the synthesis, structures, characteristics and properties of 1–3 have been discussed.
Experimental section
Materials and measurements
In this paper, all reagents and solvents for synthesis were obtained from commercial sources and used without further purification, such as 3,3-dimethyl-1-butyne (Aladdin, >95%). All other reagents were of analytical grade and were used as received. [AgC
CtBu]n,17 [Mn12(CH3COO)16(H2O)4O12]·2CH3COOH·4H2O, (NH4)3[CrMo6H6O24]·7H2O and α-K6P2W18O62·14H2O were synthesized according to the literature.18 Elemental analyses (C and H) were performed on a Perkin-Elmer 2400 CHN elemental analyzer. Ag was analyzed on a PLASMA-SPEC(I) ICP atomic emission spectrometer. The FT-IR spectra were recorded from KBr pellets in the range of 4000–400 cm−1 on a Mattson Alpha-Centauri spectrometer. PXRD patterns were recorded on a Siemens D 5005 diffractometer with Cu-Kα (λ = 1.5418 Å) radiation in the range of 3–50 °C. Luminescence was measured on an F-7000 FL Spectrophotometer. Diffuse reflectivity was measured from 200 to 800 nm using barium sulfate (BaSO4) as a standard with 100% reflectance on a Varian Cary 500 UV-Vis spectrophotometer. A CHI 440 Electrochemical Quartz Crystal Microbalance was used for the electrochemical experiments. A conventional three-electrode cell was used at room temperature. The modified electrode was used as the working electrode. A SCE and a platinum wire were 0used as the reference and auxiliary electrodes, respectively.
Caution! Owing to the potential explosive nature of silver(I) alkynyls, great care should be taken, they should be handled with care and only small amounts should be used.
X-ray crystallographic analysis
Single-crystal X-ray diffraction data for compounds 1–3 were recorded on a Bruker Apex CCD II area-detector diffractometer with graphite-monochromated Mo-Kα radiation (λ = 0.71073 Å) at room temperature. Absorption corrections were applied using a multi-scan technique and were performed by using the SADABS program. Their structures were solved by the direct method of SHELXS-97 and refined by full-matrix least-square techniques using the SHELXL-97 program within WINGX.19 All of the crystal data and structure refinement details for 1–3 are given in Table 1. Comparative experiments without POMs for the synthesis of 1–3 were performed as shown in Table S1.† Selected bond lengths (Å) and angles (°) for 1–3 are listed in Tables S2–S4.† Powder X-ray diffractions of 1–3 are included in Fig. S1–S3.†
Table 1 Crystal data and structure refinements for compounds 1–3
Compound |
1 |
2 |
3 |
R1 = ∑||Fo| − |Fc||/∑|Fo. wR2 = {∑[w(Fo2 − Fc2)2]/∑[w(Fo2)2]}1/2. |
Empirical formula |
C47H58F15O11Ag11 |
C50H75O8Ag11 |
C52H65F15O11Ag12 |
Formula weight |
2266.47 |
1990.67 |
2445.48 |
Crystal system |
Triclinic |
Triclinic |
Monoclinic |
Space group |
P![[1 with combining macron]](https://www.rsc.org/images/entities/char_0031_0304.gif) |
P![[1 with combining macron]](https://www.rsc.org/images/entities/char_0031_0304.gif) |
P21 |
a (Å) |
14.551(4) |
13.983(5) |
11.490(5) |
b (Å) |
14.805(4) |
14.005(5) |
23.129(5) |
c (Å) |
18.520(7) |
18.653(5) |
14.609(5) |
α (°) |
110.957(4) |
99.434(5) |
90.000(5) |
β (°) |
92.602(4) |
99.090(5) |
106.035(5) |
γ (°) |
117.093(3) |
116.326(5) |
90.000(5) |
V (Å3) |
3211.2(17) |
3117.9(18) |
3731(2) |
Z |
2 |
2 |
2 |
D/g cm−3 |
2.344 |
2.120 |
2.177 |
μ/mm−1 |
3.367 |
3.415 |
3.156 |
F(000) |
2152 |
1912 |
2328 |
Reflection collected |
15 742 |
15 420 |
18 864 |
Unique reflections |
11 016 |
10 520 |
9684 |
Parameters |
492 |
404 |
470 |
Rint |
0.0573 |
0.0523 |
0.0385 |
GOF |
0.950 |
0.993 |
1.036 |
R1a [I > 2σ(I)] |
0.0781 |
0.0639 |
0.0607 |
wR2b (all data) |
0.2233 |
0.1799 |
0.1663 |
Synthesis
[Ag11(C
CtBu)6(CF3COO)5(CH3OH)]n (1). [AgC
CtBu]n (0.0759 g, 0.4016 mmol) was dissolved in methanol (10 mL) under stirring. Then, CF3COOH (0.2 mL, 0.0027 mmol) and [Mn12(CH3COO)16(H2O)4O12]·2CH3COOH·4H2O (0.0219 g, 0.0106 mmol) were added in turn under stirring to form a brown suspension. After stirring for 10 h, the suspension (pH ≈ 1.2) was filtered and the filtrate was evaporated slowly at room temperature in an Erlenmeyer flask. A few weeks later, we unexpectedly achieved compound 1, which was deposited as colorless block crystals. Yield: ca. 16% (based on Ag). Elemental analysis (%) calcd for C47H58F15O11Ag11: C, 24.86; H, 2.57; Ag, 52.26. Found: C, 23.18; H, 2.97; Ag, 53.82. IR (KBr): ν = 1988 cm−1 (vs, C
C); 1683 cm−1 (w, C
O).
[Ag11(C
CtBu)7(CH3COO)4]n (2).
Method 1. [AgC
CtBu]n (0.0588 g, 0.3111 mmol), (NH4)3[CrMo6H6O24]·7H2O (0.0206 g, 0.0172 mmol), Ni(CH3COO)2·4H2O (0.0175 g, 0.0703 mmol), NaClO4·H2O (0.0083 g, 0.0591 mmol) and AgBF4 (0.0173 g, 0.0889 mmol) were added in turn into ethanol–acetonitrile–dimethylformamide (v
:
v
:
v = 1
:
1
:
1) (15 mL) under ultrasonication. The mixture was stirred for 28 h to give a white suspension (pH ≈ 7.4), which was filtered and the filtrate was evaporated slowly at room temperature in an Erlenmeyer flask. A few days later, we had successfully achieved compound 2, which was deposited as colorless block crystals. Yield: ca. 29% (based on Ag).
Method 2. [AgC
CtBu]n (0.0106 g, 0.0561 mmol) was dissolved in a solution of Co(CH3COO)2·4H2O (0.0327 g, 0.1313 mmol) in ethanol (25 mL) under ultrasonication. Then, 1,10-phenanthroline monohydrate (0.0256 g, 0.1291 mmol) was added to the above resulting solution. The mixture was stirred for 20 h to give a light pink solution (pH ≈ 8.5). The solution was evaporated slowly at room temperature in an Erlenmeyer flask. A few days later, we had successfully achieved compound 2, which was deposited as colorless block crystals. Yield: ca. 21% (based on Ag). Elemental analysis (%) calcd for C50H75O8Ag11: C, 30.17; H, 3.80; Ag, 59.61. Found: C, 28.75; H, 4.08; Ag, 58.26. IR (KBr): ν = 2022 cm−1 (vs, C
C); 1661 cm−1 (w, C
O).
[Ag12(C
CtBu)7(CF3COO)5·H2O]n (3). [AgC
CtBu]n (0.0565 g, 0.2989 mmol) was dissolved in a solution of CF3COOAg (0.1099 g, 0.4975 mmol) in methanol (10 mL) under ultrasonication. Then α-K6P2W18O62·14H2O (0.0218 g, 0.0045 mmol) and H2O (2 mL) were added to the above resulting solution. The mixture was stirred for 3 h to give a light yellow suspension. The reaction mixture was transferred to a Teflon-lined stainless autoclave (25 mL) and kept at 85 °C for 22 h. After cooling to room temperature, the solution (pH ≈ 4.7) was filtered and the filtrate was evaporated slowly at room temperature in an Erlenmeyer flask. A few weeks later, we had successfully achieved compound 3, which was deposited as colorless rectangular crystals. Yield: ca. 21% (based on Ag). Elemental analysis (%) calcd for C52H65F15O11Ag12: C, 25.54; H, 2.68; Ag, 52.93. Found: C, 25.88; H, 2.49; Ag, 50.97. IR (KBr): ν = 2007 cm−1 (vs, C
C); 1677 cm−1 (m, C
O).
Results and discussion
The three 1D chain compounds 1–3 were similarly synthesized by a facile one-pot process and their formations were strongly influenced by reaction conditions such as pH value, solvent, and the type and source of auxiliary ligand.
Effect of the pH value
It is well known that the pH can have an impact on the resulting coordination architectures, and optimization of the pH condition may also be considered as a structure-directing factor from the viewpoint of forming hydrogen-bonding supramolecular interactions.20 In this work, the final mixture pH values were approximately 1.0–1.2, 7.4–8.5 and 3.7–4.7 for compounds 1, 2 and 3, respectively (as shown in Scheme 1). The lower pH value for 1 leads to an extended 2D supramolecular network, which is fabricated from the 1D covalently bonded chains of 1 via hydrogen bonds between the coordinated tBuC
C− ligands and CF3COO− auxiliary ligands.
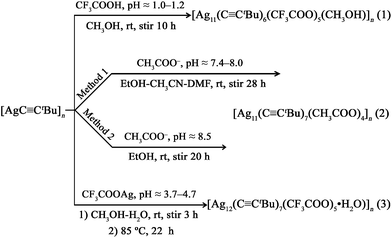 |
| Scheme 1 Synthetic procedures for compounds 1–3. | |
Effect of the solvent
Solvents also have significant effects on the final coordination architectures.21 CH3OH not only serves as an organic solvent which provides the reaction medium but also acts as a ligand by attaching to the central silver skeletons in 1. Additionally, H2O also plays the role of a ligand which coordinates to the central silver skeletons in 3.
Effect of the type and source of auxiliary ligand
The structural differences among the three multinuclear silver(I) alkynyl compounds 1–3 can also be ascribed to the effect of the type and source of auxiliary ligand. In detail, the type of carboxylate ligand is different between 1, 3 (CF3COO−) and 2 (CH3COO−), while the source of the CF3COO− auxiliary ligands is also different between 1 (CF3COOH) and 3 (CF3COOAg). Although similar Ag11 skeletons of the columnar structures exist in 1 and 2, they finally display different configurations that are not only due to the different pH values and solvents used but also because of the difference between the CF3COO− and CH3COO− auxiliary ligands. Compound 2 provides a simple synthetic protocol for the preparation of stable silver(Ι) alkynyl compounds with higher yield by introducing CH3COO− auxiliary ligands instead of CF3COO−.
Effect of the POMs
POMs were originally introduced as template reagents to induce the formation of core–shell silver(Ι) alkynyl clusters. However, three POMs in different configurations, namely, [Mn12(CH3COO)16(H2O)4O12]·2CH3COOH·4H2O for the synthesis of 1, (NH4)3[CrMo6H6O24]·7H2O for 2, and α-K6P2W18O62·14H2O for 3, were not involved in building the target compounds. In order to understand and confirm what role the three POMs play in constructing compounds 1–3, comparative experiments were performed in the absence of POMs. Fortunately, compounds 1–3 can be obtained in the absence of POMs, because little change was observed in the pH values of the three reaction systems without POMs (as depicted in Table S1†). Furthermore, compound 2 can be synthesized not only by the self-assembly of [AgC
CtBu]n and CH3COO− with/without (NH4)3[CrMo6H6O24]·7H2O (pH ≈ 7.4/8.0) as shown in method 1, but also through the reaction of [AgC
CtBu]n and CH3COO− accompanied by other unreacted reagents replacing (NH4)3[CrMo6H6O24]·7H2O (pH ≈ 8.5) as illustrated in method 2. These results indicate that POMs are not necessary for the preparation of compounds 1–3 since they can be ignored or replaced if the pH values of the reaction systems are well controlled in the appropriate range.
Effect of cobalt/nickel metal cations
Compound 2 can be obtained through the two methods mentioned above, in which two different acetates, Ni(CH3COO)2·4H2O and Co(CH3COO)2·4H2O were used in method 1 and method 2, respectively. Ni(CH3COO)2·4H2O and Co(CH3COO)2·4H2O only act as acetates to provide the CH3COO− auxiliary ligand. Hence, Ni2+ and Co2+ make no difference in the synthesis of 2.
Effect of other factors
The coordination from η2 to η6 of silver ions and the μ2- to μ4-coordination modes of tBuC
C− ligands together with argentophilic interactions also play important roles in the construction of the three silver(Ι) alkynyl compounds 1–3.
From what has been discussed above, we can see that this work implies that the crystallization conditions are important, because the synthesis of these compounds is affected not only by the pH value but also by the solvent, and the type and source of auxiliary ligand in the reaction systems. These results further provide us with a useful and important guide to the synthesis of other silver(Ι) alkynyl compounds by a facile one-pot process.
Powder X-ray diffraction analyses
To characterize the phase purities of compounds 1–3, powder X-ray diffraction (PXRD) patterns have been obtained at ambient temperature. The experimental PXRD patterns of compounds 1–3 are in agreement with the corresponding simulated ones, which demonstrate that the synthesized bulk materials and the measured single crystals are the same (Fig. S1–S3†). By contrast, the slight differences in intensities may be attributed to the preferred orientation of the crystalline powder samples, and the tiny peak shifts may be assigned to the baseline drift of the PXRD diffractometer and different measuring temperatures for single-crystal and powder diffraction.22
Description of the crystal structures of 1–3
[Ag11(C
CtBu)6(CF3COO)5(CH3OH)]n (1). Single-crystal X-ray diffraction analysis reveals that compound 1 crystallizes in the triclinic P
space group with a columnar structure. In a [Ag11(C
CtBu)6(CF3COO)5(CH3OH)] asymmetric unit (Fig. 1a), it consists of eleven Ag(I) ions, six tBuC
C− ligands, five CF3COO− auxiliary ligands and one CH3OH ligand. Herein, Ag1, Ag2, Ag4, Ag5, Ag6 and Ag7 are chelated by tBuC
C−, CF3COO− and CH3OH ligands, while Ag3, Ag8, Ag9, Ag10 and Ag11 are left open for connection to neighbouring asymmetric units through tBuC
C− and CF3COO− ligands. Consequently, as shown in Fig. 1b, a 1D chain in the shape of a columnar structure along the c axis is formed depending on Ag⋯Ag connections (2.849(2)–3.368(2) Å), Ag–C bonds (2.063(15)–2.707(15) Å) and Ag–O bonds (2.212(13)–2.561(13) Å). It can be seen that Ag⋯Ag connections lying in the range 2.849(2)–3.368(2) Å (partly shorter than twice the van der Waals radius for Ag: 3.44 Å) powerfully demonstrate that the skeleton of the columnar structure in 1 is promoted by the existence of important argentophilic Ag⋯Ag connections, which are clearly indicative of the presence of d10–d10 interactions.23 The eleven silver ions display η2 to η6 coordination modes. The ethynide moieties, C11
C12 and C29
C30 adopt the μ3–η1, η1, η2 mode; C17
C18, C35
C36 and C41
C42 take the μ4–η1, η1, η1, η2 mode; and C23
C24 is in the μ4–η1, η1, η2, η2 mode. Among the five bridging trifluoroacetate ligands, O1–O2, O5–O6 and O7–O8 are in the syn-μ3-O, O′, O′ mode, and O3–O4 and O9–O10 are in the μ2-O, O′ mode. In addition, the oxygen atom of one CH3OH molecule is linked to Ag9 with the Ag9–O11 bond length of 2.561(15) Å. Finally, these 1D [Ag11(C
CtBu)6(CF3COO)5(CH3OH)]n chains are further connected by relatively weak hydrogen bonds C28–H28C⋯F4 (H⋯F 2.54 Å) between the coordinated tBuC
C− ligands and CF3COO− auxiliary ligands along the b direction to yield a hydrogen-bonded layer parallel to the bc plane, as shown in Fig. 2.
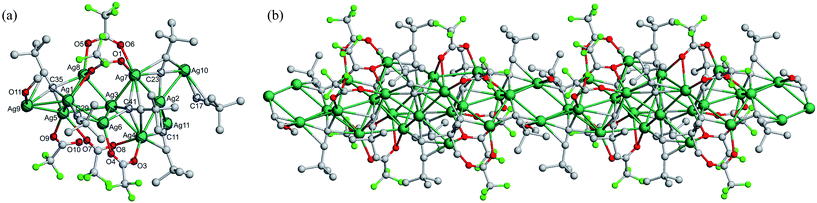 |
| Fig. 1 (a) Atom labeling ball-and-stick model of the asymmetric unit in 1. (b) Standard ball-and-stick model of a 1D chain in 1. Hydrogen atoms are omitted for clarity. Color code: Ag, sea green; C, gray-25%; O, red; F, bright green. | |
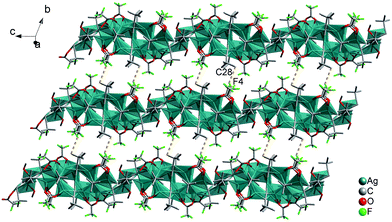 |
| Fig. 2 Polyhedron/standard ball-and-stick model of the 2D layer in 1 interwoven by hydrogen bonds parallel to the bc plane. Hydrogen atoms are omitted for clarity. Color code: Ag polyhedron, teal; C, gray-40%; O, red; F, bright green. | |
[Ag11(C
CtBu)7(CH3COO)4]n (2). Single-crystal X-ray diffraction analysis reveals that compound 2 crystallizes in the triclinic P
space group, and eleven Ag(I) ions, seven tBuC
C− ligands and four CH3COO− auxiliary ligands can be seen in an asymmetric unit. Herein, the η2–η5 coordination modes of the silver ions and five types of coordination modes for the ethynide moieties are observed in 2. The ethynide moieties; C27
C28 bridges two silver ions in the μ2–η1, η1 mode; C9
C10 and C21
C22 connect three silver ions in theμ3–η1, η1, η1 mode; C12
C13 and C18
C19 link three silver ions in the μ3–η1, η1, η2 mode; C15
C16 concatenates four silver ions in the μ4–η1, η1, η1, η1 mode; and C24
C25 is bound to four silver ions in the μ4–η1, η1, η1, η2 mode to take the shape of tert-Bu-C
C⊃Agn (n = 2, 3, 4) units. Then, these units approach each other so that they coalesce by sharing Ag⋯Ag connections (2.8986(18)–3.376(2) Å) to generate a [Ag11(C
CtBu)7]4+ segment. This [Ag11(C
CtBu)7]4+ segment is further stabilized by four bridging CH3COO− ligands in the syn-μ3-O, O′, O′ mode to make up a [Ag11(C
CtBu)7(CH3COO)4] aggregation (Fig. 3a). Such Ag11 aggregations are fused through Ag⋯Ag connections, Ag–O bonds (2.247(13)–2.596(14) Å) and Ag–C bonds (2.046(15)–2.703(15) Å) to produce an infinite 1D coordination column along the c axis (Fig. 3b). Although tBuC
C− and CH3COO− ligands are coordinated to Ag11 skeletons and warp the column up, tert-butyl groups and the CH3 moieties protrude on both sides of this 1D chain to prevent further linkage.
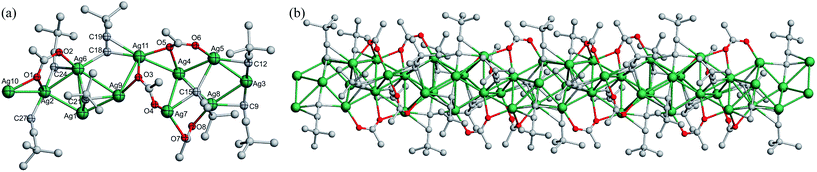 |
| Fig. 3 (a) Atom labeling ball-and-stick model of the asymmetric unit in 2. (b) Standard ball-and-stick model of a 1D chain in 2. Hydrogen atoms are omitted for clarity. Color code: Ag, sea green; C, gray-25%; O, red. | |
[Ag12(C
CtBu)7(CF3COO)5·H2O]n (3). Compound 3 crystallizes in the monoclinic P21 space group. An asymmetric unit comprises a [Ag12(C
CtBu)7(CF3COO)5·H2O] aggregation (Fig. 4a), which is made up of a central Ag12 skeleton surrounded by seven tBuC
C− ligands, five CF3COO− ligands and one aqua ligand with Ag⋯Ag connections ranging from 2.788(2) to 3.334(2) Å. The twelve silver ions are in the η2–η5 coordination modes. The ethynide moieties, C11
C12, C23
C24, C47
C48 adopt the μ3–η1, η1, η1 ligation mode; C17
C18, C35
C36 take the μ3–η1, η1, η2 ligation mode; C41
C42 exhibits the μ4–η1, η1, η1, η1 ligation mode; and C29
C30 is in the μ4–η1, η1, η1, η2 ligation mode to make connections around the Ag12 skeleton. As for the five CF3COO− ligands, except for the O9–O10 group in the syn-μ3-O, O′, O′ mode and the O7–O8 group in the μ1-O mode, the other groups lying above and below the Ag12 skeleton span the Ag⋯Ag edges through the μ2-O, O′ mode. The Ag–C bonds range from 1.959(15) to 2.708(18) Å, and the Ag–O bonds are in the range of 2.128(16)–2.584(17) Å. In addition, an aqua ligand coordinates with Ag12 with the Ag12–O11W bond length of 2.52(3) Å. Then, a 1D chain along the a axis made up of inversion-related [Ag12(C
CtBu)7(CF3COO)5·H2O] aggregations bridged pairwise is formed (Fig. 4b).
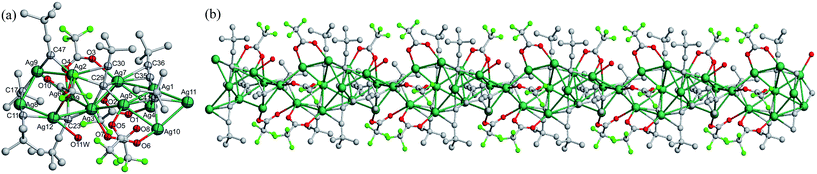 |
| Fig. 4 (a) Atom labeling ball-and-stick model of the asymmetric unit in 3. (b) Standard ball-and-stick model of a 1D chain in 3. Hydrogen atoms are omitted for clarity. Color code: Ag, sea green; C, gray-25%; O, red; F, bright green. | |
Solid state optical diffuse reflection spectra of 1–3
Some multinuclear silver clusters have been reported to be promising semiconductive materials.16 Therefore, the solid state optical diffuse reflection spectra of 1–3 were measured at room temperature to obtain their band gaps (Eg, photoresponse wavelength region). The Eg term is defined as the intersection point between the energy axis and the line extrapolated from the linear portion of the adsorption edge in a plot of the Kubelka–Munk function F versus energy E.24 Absorption data were calculated from the reflectance using the Kubelka–Munk function. As depicted in Fig. S4–S6,† the UV-vis-NIR absorption spectra of 1–3 in the solid state display absorption peaks at ca. 425, 442 and 438 nm, and the energy gaps of 1–3 (see inset of Fig. S4–S6†) were determined to be 3.60, 2.73 and 2.92 eV, respectively. The results indicate that compounds 1–3 are a kind of potential wide band gap semiconductor material.
Luminescence properties of 1–3
Because compounds with d10-transition metal centers, such as copper, silver and gold, have excellent luminescence properties,25 the luminescence spectra of 1–3 in the solid state have been obtained at room temperature. Compounds 1–3 show similar luminescence spectra with a maximum emission band at ca. 421 nm and several shoulder peaks upon excitation at 365 nm (Fig. S7–S9†). With reference to our previous spectroscopic work on related polynuclear silver clusters26 and the luminescence spectra of the AgC
CtBu ligand in the solid state (Fig. S10†), the emissions of 1–3 are probably ligand-to-metal-charge-transfer (LMCT) transitions.
Electrochemical behavior of 2
Because compounds 1–3 have similar structures as well as consisting of the same silver(Ι) ions and tBuC
C− ligands, compound 2 is taken as an example to study in detail the electrochemical behaviour of this kind of compound. Hence, a 2-bulk-modified carbon paste electrode (2-CPE) was fabricated as the working electrode to study the electrochemical properties of this type of silver(I) alkynyl compound.27 As shown in Fig. 5, the voltammetric behavior of 2-CPE in a 0.01 M H2SO4 + 0.5 M Na2SO4 aqueous solution at a scan rate of 0.1 V s−1 displays an obvious redox couple in the potential range of 0 to 600 mV. The mean peak potential E1/2 = (Epa + Epc)/2 is 267 mV for 2-CPE, which is similar to the AgC
CtBu ligand (E1/2 = 285 mV) (vs. SCE). Thus, the redox peak for 2 is attributed to Ag+/Ag0 couples, but the slightly different peak potentials between 2 and the AgC
CtBu ligand should be affected by the final composition of 2.9f,10a
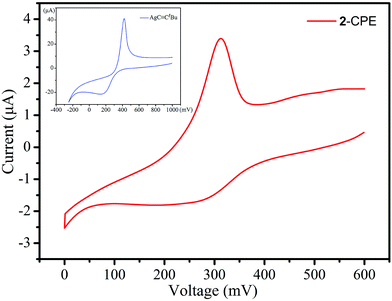 |
| Fig. 5 Cyclic voltammogram of 2-CPE (0 to 600 mV) in a 0.01 M H2SO4 + 0.5 M Na2SO4 aqueous solution. Scan rate: 100 mV s−1. The inset shows the cyclic voltammogram of the AgC CtBu ligand (−250 to 1000 mV) in a 0.01 M H2SO4 + 0.5 M Na2SO4 aqueous solution. | |
The effect of scan rate on the electrochemical behavior of 2-CPE was investigated in the potential range of 0 to 600 mV in a 0.01 M H2SO4 + 0.5 M Na2SO4 aqueous solution. As shown in Fig. 6, with the scan rate increasing from 120 to 280 mV s−1, the peak potential changes gradually: the cathodic peak potential gradually shifts in the negative direction and the corresponding anodic peak potential shifts in the positive direction. The inset of Fig. 6 shows that the peak currents are proportional to the scan rate up to 280 mV s−1 for 2-CPE, suggesting that the redox process for 2-CPE is surface-controlled.28
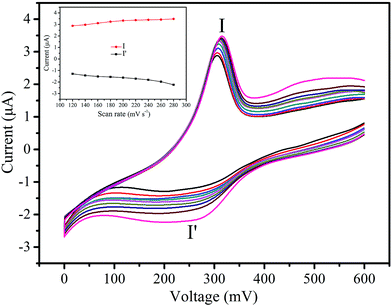 |
| Fig. 6 Cyclic voltammograms of the 2-CPE in the 0.01 M H2SO4 + 0.5 M Na2SO4 aqueous solution at different scan rates (from inner to outer: 120, 140, 160, 180, 200, 220, 240, 260, 280 mV s−1). The inset shows the plots of the anodic and cathodic peak currents against scan rates. | |
As we know, the electroreduction of nitrite requires a large overpotential and no response has been observed in the potential range of 0 to 600 mV on a bare CPE in a solution containing nitrite. However, 2-CPE displays excellent electrocatalytic activity toward the reduction of nitrite, as shown in Fig. 7.29 With the addition of nitrite, the reduction peak current increased while the corresponding oxidation peak current decreased, suggesting that 2-CPE possesses good electrocatalytic activity toward the reduction of nitrite.
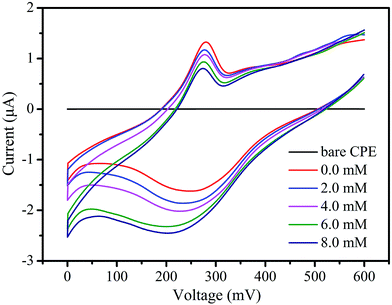 |
| Fig. 7 Cyclic voltammograms of 2-CPE in a 0.01 M H2SO4 + 0.5 M Na2SO4 aqueous solution containing 0.0–8.0 mM KNO2 and a bare CPE in a 1.0 mM KNO2 + 0.01 M H2SO4 + 0.5 M Na2SO4 aqueous solution (scan rate: 100 mV s−1). | |
Conclusions
In summary, the preparation of multinuclear silver(Ι) alkynyl compounds constructed from tBuC
C− ligand with a wide variety of structures is affected by many factors, such as the pH value of the reaction system, solvent, the type and source of auxiliary ligand, and so on. In this paper, three 1D silver(Ι) alkynyl compounds, [Ag11(C
CtBu)6(CF3COO)5(CH3OH)]n (1), [Ag11(C
CtBu)7(CH3COO)4]n (2) and [Ag12(C
CtBu)7(CF3COO)5·H2O]n (3) have been acquired by changing the type (CF3COO−/CH3COO−) and source of auxiliary ligand along with adjusting the pH values in different solvents. Notably, an extended 2D supramolecular network is fabricated from 1D covalently-bonded chains of 1 via hydrogen bonds. Furthermore, the synthesis, structures, characteristics and properties of 1–3 have been discussed. Further work on the construction of multinuclear silver(I) alkynyl compounds is underway in our group.
Acknowledgements
This work was financially supported by the NSFC of China (no. 21471027, 21171033, 21131001, 21222105), the National Key Basic Research Program of China (no. 2013CB834802), the Foundation for Author of National Excellent Doctoral Dissertation of P.R. China (FANEDD) (no. 201022), The Science and Technology Development Planning of Jilin Province (no. 20140203006GX), Changbai mountain scholars of Jilin Province and FangWu distinguished young scholar of NENU, and the Fundamental Research Funds for the Central Universities (12SSXT142).
Notes and references
-
(a) R. Nast and H. Schindel, Z. Anorg. Allg. Chem., 1963, 326, 201 CrossRef CAS;
(b) R. Nast, Coord. Chem. Rev., 1982, 47, 89 CrossRef CAS;
(c) H. Schmidbaur, Chem. Soc. Rev., 1995, 24, 391 RSC;
(d) V. W.-W. Yam, W. K.-M. Fung and K.-K. Cheung, Angew. Chem., Int. Ed. Engl., 1996, 35, 1110 CrossRef;
(e) T. C. W. Mak and L. Zhao, Chem.–Asian J., 2007, 2, 456 CrossRef CAS PubMed;
(f) Y.-P. Xie and T. C. W. Mak, J. Am. Chem. Soc., 2011, 133, 3760 CrossRef CAS PubMed;
(g) C.-Y. Gao, L. Zhao and M.-X. Wang, J. Am. Chem. Soc., 2011, 133, 8448 CrossRef CAS PubMed;
(h) C. S. Ma, C. T.-L. Chan, W.-M. Kwok and C.-M. Che, Chem. Sci., 2012, 3, 1883 RSC;
(i) P. Maity, T. Wakabayashi, N. Ichikuni, H. Tsunoyama, S. H. Xie, M. Yamauchi and T. Tsukuda, Chem. Commun., 2012, 48, 6085 RSC;
(j) L. Rodríguez, M. Ferrer, R. Crehuet, J. Anglada and J. C. Lima, Inorg. Chem., 2012, 51, 7636 CrossRef PubMed.
-
(a) G.-C. Guo, G.-D. Zhou and T. C. W. Mak, J. Am. Chem. Soc., 1999, 121, 3136 CrossRef CAS;
(b) H. Lang, D. S. A. George and G. Rheinwald, Coord. Chem. Rev., 2000, 206–207, 101 CrossRef CAS;
(c) L. Zhao and T. C. W. Mak, J. Am. Chem. Soc., 2005, 127, 14966 CrossRef CAS PubMed;
(d) T. C. W. Mak, X.-L. Zhao, Q.-M. Wang and G.-C. Guo, Coord. Chem. Rev., 2007, 251, 2311 CrossRef CAS PubMed;
(e) B. Li, S.-Q. Zang, R. Liang, Y.-J. Wu and T. C. W. Mak, Organometallics, 2011, 30, 1710 CrossRef CAS;
(f) P.-S. Cheng, S. Marivel, S.-Q. Zang, G.-G. Gao and T. C. W. Mak, Cryst. Growth Des., 2012, 12, 4519 CrossRef CAS.
-
(a) Y.-Y. Lin, S.-W. Lai, C.-M. Che, K.-K. Cheung and Z.-Y. Zhou, Organometallics, 2002, 21, 2275 CrossRef CAS;
(b) M. P. Cifuentes and M. G. Humphrey, J. Organomet. Chem., 2004, 689, 3968 CrossRef CAS PubMed;
(c) V. W.-W. Yam and K. M.-C. Wong, Top. Curr. Chem., 2005, 257, 1 CrossRef CAS;
(d) Q.-H. Wei, G.-Q. Yin, L.-Y. Zhang and Z.-N. Chen, Inorg. Chem., 2006, 45, 10371 CrossRef CAS PubMed;
(e) Z.-N. Chen, N. Zhao, Y. Fan and J. Ni, Coord. Chem. Rev., 2009, 253, 1 CrossRef CAS PubMed;
(f) B. R. Buckley, A. N. Khan and H. Heaney, Chem.–Eur. J., 2012, 18, 3855 CrossRef CAS PubMed;
(g) I. O. Koshevoy, Y.-C. Chang, A. J. Karttunen, S. I. Selivanov, J. Jänis, M. Haukka, T. Pakkanen, S. P. Tunik and P.-T. Chou, Inorg. Chem., 2012, 51, 7392 CrossRef CAS PubMed;
(h) M. C. Blanco, J. Cámara, M. C. Gimeno, A. Laguna, S. L. James, M. C. Lagunas and M. D. Villacampa, Angew. Chem., Int. Ed., 2012, 51, 9777 CrossRef CAS PubMed.
-
(a) D. Rais, D. M. P. Mingos, R. Vilar, A. J. P. White and D. J. Williams, J. Organomet. Chem., 2002, 652, 87 CrossRef CAS;
(b) S.-Q. Zang and T. C. W. Mak, Inorg. Chem., 2008, 47, 7094 CrossRef CAS PubMed;
(c) L. Zhao and T. C. W. Mak, Inorg. Chem., 2009, 48, 6480 CrossRef CAS PubMed.
-
(a) L. Zhao, W.-Y. Wong and T. C. W. Mak, Chem.–Eur. J., 2006, 12, 4865 CrossRef CAS PubMed;
(b) S. C. K. Hau and T. C. W. Mak, Chem.–Eur. J., 2013, 19, 5387 CrossRef CAS PubMed.
-
(a) G.-C. Guo and T. C. W. Mak, Angew. Chem. Int. Ed., 1998, 37, 3183 CrossRef CAS;
(b) C.-M. Che, M.-C. Tse, M. C. W. Chan, K.-K. Cheung, D. L. Phillips and K.-H. Leung, J. Am. Chem. Soc., 2000, 122, 2464 CrossRef CAS;
(c) P.-S. Cheng, S. Marivel, S.-Q. Zang, G.-G. Gao and T. C. W. Mak, Cryst. Growth Des., 2012, 12, 4519 CrossRef CAS.
-
(a) G.-G. Gao, P.-S. Cheng and T. C. W. Mak, J. Am. Chem. Soc., 2009, 131, 18257 CrossRef CAS PubMed;
(b) J. Qiao, K. Shi and Q.-M. Wang, Angew. Chem., Int. Ed., 2010, 49, 1765 CrossRef CAS PubMed;
(c) G. Li, Z. Lei and Q.-M. Wang, J. Am. Chem. Soc., 2010, 132, 17678 CrossRef CAS PubMed.
-
(a) D. Rais, D. M. P. Mingos, R. Vilar, A. J. P. White and D. J. Williams, Organometallics, 2000, 19, 5209 CrossRef CAS;
(b) X.-D. Chen, M. Du and T. C. W. Mak, Chem. Commun., 2005, 4417 RSC;
(c) S.-D. Bian, J.-H. Jia and Q.-M. Wang, J. Am. Chem. Soc., 2009, 131, 3422 CrossRef CAS PubMed;
(d) F. Gruber, M. Schulz-Dobrick and M. Jansen, Chem.–Eur. J., 2010, 16, 1464 CrossRef CAS PubMed;
(e) C.-Y. Gao, L. Zhao and M.-X. Wang, J. Am. Chem. Soc., 2012, 134, 824 CrossRef CAS PubMed.
-
(a) D. Rais, J. Yau, D. M. P. Mingos, R. Vilar, A. J. P. White and D. J. Williams, Angew. Chem., Int. Ed., 2001, 40, 3464 CrossRef CAS;
(b) O. M. Abu-Salah, M. H. Ja’far, A. R. Al-Ohaly, K. A. Al-Farhan, H. S. Al-Enzi, O. V. Dolomanov and J. A. K. Howard, Eur. J. Inorg. Chem., 2006, 2353 CrossRef CAS;
(c) M.-L. Chen, X.-F. Xu, Z.-X. Cao and Q.-M. Wang, Inorg. Chem., 2008, 47, 1877 CrossRef CAS PubMed;
(d) S.-D. Bian, H.-B. Wu and Q.-M. Wang, Angew. Chem., Int. Ed., 2009, 48, 5363 CrossRef CAS PubMed;
(e) G.-G. Gao, P.-S. Cheng and T. C. W. Mak, J. Am. Chem. Soc., 2009, 131, 18257 CrossRef CAS PubMed;
(f) J. Qiao, K. Shi and Q.-M. Wang, Angew. Chem., Int. Ed., 2010, 49, 1765 CrossRef CAS PubMed;
(g) F. Gruber and M. Jansen, Angew. Chem., Int. Ed., 2010, 49, 4924 CrossRef CAS PubMed.
-
(a) Y.-P. Xie and T. C. W. Mak, Chem. Commun., 2012, 48, 1123 RSC;
(b) S. C. K. Hau, P.-S. Cheng and T. C. W. Mak, J. Am. Chem. Soc., 2012, 134, 2922 CrossRef CAS PubMed;
(c) Y.-P. Xie and T. C. W. Mak, Inorg. Chem., 2012, 51, 8640 CrossRef CAS PubMed;
(d) Y.-P. Xie and T. C. W. Mak, Angew. Chem., Int. Ed., 2012, 51, 8783 CrossRef CAS PubMed;
(e) Y.-Y. Li, F. Gao, J. E. Beves, Y.-Z. Li and J.-L. Zuo, Chem. Commun., 2013, 49, 3658 RSC;
(f) Z.-G. Jiang, K. Shi, Y.-M. Lin and Q.-M. Wang, Chem. Commun., 2014, 50, 2353 RSC.
- S.-D. Bian and Q.-M. Wang, Chem. Commun., 2008, 5586 RSC.
-
(a) M. Fujita, Y. J. Kwon, S. Washizu and K. Ogura, J. Am. Chem. Soc., 1994, 116, 1151 CrossRef CAS;
(b) H. Oshio, Y. Saito and T. Ito, Angew. Chem., Int. Ed. Engl., 1997, 36, 2673 CrossRef CAS;
(c) P. J. Hagrman, D. Hagrman and J. Zubieta, Angew. Chem., Int. Ed., 1999, 38, 2638 CrossRef;
(d) J. S. Seo, D. Whang, H. Lee, S. I. Jun, J. Oh, Y. J. Jeon and K. Kim, Nature, 2000, 404, 982 CrossRef CAS PubMed;
(e) E. Coronado, J. R. Galán-Mascarós, C. J. Gómez-García and V. Laukhin, Nature, 2000, 408, 447 CrossRef CAS PubMed;
(f) Y. H. Wang, L. Y. Feng, Y. G. Li, C. W. Hu, E. B. Wang, N. H. Hu and H. Q. Jia, Inorg. Chem., 2002, 41, 6351 CrossRef CAS PubMed.
-
(a) D. Braga, F. Grepioni and G. R. Desiraju, Chem. Rev., 1998, 98, 1375 CrossRef CAS PubMed;
(b) H. L. Li, M. Eddaoudi, M. O’Keeffe and O. M. Yaghi, Nature, 1999, 402, 276 CrossRef CAS PubMed;
(c) S. S.-Y. Chui, S. M.-F. Lo, J. P. H. Charmant, A. G. Orpen and I. D. Williams, Science, 1999, 283, 1148 CrossRef CAS;
(d) D. Braga and F. Grepioni, Acc. Chem. Res., 2000, 33, 601 CrossRef CAS PubMed;
(e) J. C. MacDonald, P. C. Dorrestein, M. M. Pilley, M. M. Foote, J. L. Lundburg, R. W. Henning, A. J. Schultz and J. L. Manson, J. Am. Chem. Soc., 2000, 122, 11692 CrossRef CAS;
(f) B. J. Holliday and C. A. Mirkin, Angew. Chem., Int. Ed., 2001, 40, 2022 CrossRef CAS;
(g) D. Armentano, G. D. Munno, F. Lloret, A. V. Palii and M. Julve, Inorg. Chem., 2002, 41, 2007 CrossRef CAS PubMed;
(h) J.-M. Lehn, Science, 2002, 295, 2400 CrossRef CAS PubMed.
-
(a) S.-Q. Zang, L. Zhao and T. C. W. Mak, Organometallics, 2008, 27, 2396 CrossRef CAS;
(b) L. Zhao, C.-Q. Wan, J. Han, X.-D. Chen and T. C. W. Mak, Chem.–Eur. J., 2008, 14, 10437 CrossRef CAS PubMed;
(c) B. Li, R.-W. Huang, H.-C. Yao, S.-Q. Zang and T. C. W. Mak, CrystEngComm, 2014, 16, 723 RSC.
-
(a) K. Zhou, C. Qin, H.-B. Li, L.-K. Yan, X.-L. Wang, G.-G. Shan, Z.-M. Su, C. Xu and X.-L. Wang, Chem. Commun., 2012, 48, 5844 RSC;
(b) K. Zhou, X.-L. Wang, C. Qin, H.-N. Wang, G.-S. Yang, Y.-Q. Jiao, P. Huang, K.-Z. Shao and Z.-M. Su, Dalton Trans., 2013, 42, 1352 RSC;
(c) K. Zhou, C. Qin, X.-L. Wang, L.-K. Yan, K.-Z. Shao and Z.-M. Su, CrystEngComm, 2014, 16, 10376 RSC.
-
(a) K. Zhou, C. Qin, X.-L. Wang, K.-Z. Shao, L.-K. Yan and Z.-M. Su, CrystEngComm, 2014, 16, 7860 RSC;
(b) K. Zhou, C. Qin, X.-L. Wang, K.-Z. Shao, L.-K. Yan and Z.-M. Su, Dalton Trans., 2014, 43, 10695 RSC;
(c) K. Zhou, Y. Geng, L.-K. Yan, X.-L. Wang, X.-C. Liu, G.-S. Shan, K.-Z. Shao, Z.-M. Su and Y.-N. Yu, Chem. Commun., 2014, 50, 11934 RSC;
(d) K. Zhou, C. Qin, L.-K. Yan, W.-E. Li, X.-L. Wang, H.-N. Wang, K.-Z. Shao and Z.-M. Su, Dyes and Pigments, 2015, 113, 299 CrossRef CAS PubMed.
-
(a) L. Zhao, C.-Q. Wan, J. Han, X.-D. Chen and T. C. W. Mak, Chem.–Eur. J., 2008, 14, 10437 CrossRef CAS PubMed;
(b) Z.-G. Jiang, K. Shi, Y.-M. Lin and Q.-M. Wang, Chem. Commun., 2014, 50, 2353 RSC.
-
(a) T. Lis, Acta Crystallogr., Sect. B: Struct. Crystallogr. Cryst. Chem., 1980, 36, 2042 CrossRef;
(b) K. Nomiya, T. Takahashi, T. Shirai and M. Miwa, Polyhedron, 1987, 6, 213 CrossRef CAS;
(c) W. G. Klemperer and O. M. Yaghi, Inorg. Synth., 1990, 27, 105 Search PubMed.
- G. M. Sheldrick, SHELXL-97, Program for X-ray Crystal Structure Refinement, University of Göttingen, Göttingen, Germany, 1997 Search PubMed.
-
(a) S.-L. Li, K. Tan, Y.-Q. Lan, J.-S. Qin, M.-N. Li, D.-Y. Du, H.-Y. Zang and Z.-M. Su, Cryst. Growth Des., 2010, 10, 1699 CrossRef CAS;
(b) X. X. Xu, X. Zhang, X. X. Liu, T. Sun and E. B. Wang, Cryst. Growth Des., 2010, 10, 2272 CrossRef CAS;
(c) Z. Su, J. Fan, T. Okamura, W.-Y. Sun and N. Ueyama, Cryst. Growth Des., 2010, 10, 3515 CrossRef CAS;
(d) X.-L. Wang, J. Luan, F.-F. Sui, H.-Y. Lin, G.-C. Liu and C. Xu, Cryst. Growth Des., 2013, 13, 3561 CrossRef CAS;
(e) H.-M. Zhang, Y.-C. He, J. Yang, Y.-Y. Liu and J.-F. Ma, Cryst. Growth Des., 2014, 14, 2307 CrossRef CAS.
-
(a) G.-S. Yang, Z.-L. Lang, H.-Y. Zang, Y.-Q. Lan, W.-W. He, X.-L. Zhao, L.-K. Yan, X.-L. Wang and Z.-M. Su, Chem. Commun., 2013, 49, 1088 RSC;
(b) Y.-C. He, J. Guo, H.-M. Zhang, J.-F. Ma and Y.-Y. Liu, CrystEngComm, 2014, 16, 5450 RSC.
- X.-L. Wang, F.-F. Sui, H.-Y. Lin, J.-W. Zhang and G.-C. Liu, Cryst. Growth Des., 2014, 14, 3438 CAS.
-
(a) T. L. Zhang, J. X. Kong, Y. J. Hu, X. G. Meng, H. B. Yin, D. S. Hu and C. P. Ji, Inorg. Chem., 2008, 47, 3144 CrossRef CAS PubMed;
(b) D. Sun, Z.-H. Wei, D.-F. Wang, N. Zhang, R.-B. Huang and L.-S. Zheng, Cryst. Growth Des., 2011, 11, 1427 CrossRef CAS;
(c) D. Sun, F.-J. Liu, R.-B. Huang and L.-S. Zheng, Inorg. Chem., 2011, 50, 12393 CrossRef CAS PubMed;
(d) S. Yuan, Y.-K. Deng, X.-P. Wang and D. Sun, New J. Chem., 2013, 37, 2973 RSC;
(e) B. Li, J.-H. Liao, Y.-J. Li and C. W. Liu, CrystEngComm, 2013, 15, 6140 RSC;
(f) D. Sun, H. Wang, H.-F. Lu, S.-Y. Feng, Z.-W. Zhang, G.-X. Sun and D.-F. Sun, Dalton Trans., 2013, 42, 6281 RSC;
(g) H. Wang, C.-Q. Wan and T. C. W. Mak, Dalton Trans., 2014, 43, 7254 RSC.
-
(a) G. D. Santis, L. Fabbrizzi, M. Licchelli, A. Poggi and A. Taglietti, Angew. Chem., Int. Ed. Engl., 1996, 35, 202 CrossRef;
(b) Q. G. Wu, M. Esteghamatian, N.-X. Hu, Z. Popovic, G. Enright, Y. Tao, M. D’Iorio and S. N. Wang, Chem. Mater., 2000, 12, 79 CrossRef CAS;
(c) J. E. McGarrah, Y.-J. Kim, M. Hissler and R. Eisenberg, Inorg. Chem., 2001, 40, 4510 CrossRef CAS.
-
(a) M.-L. Chen, X.-F. Xu, Z.-X. Cao and Q.-M. Wang, Inorg. Chem., 2008, 47, 1877 CrossRef CAS PubMed;
(b) M.-X. Yang, L.-J. Chen, S. Lin, X.-H. Chen and H. Huang, Dalton Trans., 2011, 40, 1866 RSC.
-
(a) V. W.-W. Yam, K. K.-W. Lo, W. K.-M. Fung and C.-R. Wang, Coord. Chem. Rev., 1998, 171, 17 CrossRef CAS;
(b) J.-H. Jia and Q.-M. Wang, J. Am. Chem. Soc., 2009, 131, 16634 CrossRef CAS PubMed;
(c) S. Perruchas, C. Tard, X. F. L. Goff, A. Fargues, A. Garcia, S. Kahlal, J.-Y. Saillard, T. Gacoin and J.-P. Boilot, Inorg. Chem., 2011, 50, 10682 CrossRef CAS PubMed;
(d) D. Sun, L. L. Zhang, H. F. Lu, S. Y. Feng and D. F. Sun, Dalton Trans., 2013, 42, 3528 RSC;
(e) B. Li, R.-W. Huang, J.-H. Qin, S.-Q. Zang, G.-G. Gao, H.-W. Hou and T. C. W. Mak, Chem.–Eur. J., 2014, 20, 12416 CAS.
-
(a) X.-L. Wang, H.-Y. Lin, B. Mu, A.-X. Tian, G.-C. Liu and N.-H. Hu, CrystEngComm, 2011, 13, 1990 RSC;
(b) X.-L. Wang, B. Mu, H.-Y. Lin, G.-C. Liu, A.-X. Tian and S. Yang, CrystEngComm, 2012, 14, 1001 RSC;
(c) X.-L. Wang, B. Mu, H.-Y. Lin, S. Yang, G.-C. Liu, A.-X. Tian and J.-W. Zhang, Dalton Trans., 2012, 41, 11074 RSC;
(d) X. L. Wang, C. Xu, H. Y. Lin, G. C. Liu, J. Luan and Z. H. Chang, RSC Adv., 2013, 3, 3592 RSC.
-
(a) E. H. Kim, D. I. Kim, H. S. Lee, J. C. Byun, J. H. Choi and Y. C. Park, Polyhedron, 2007, 26, 85 CrossRef CAS PubMed;
(b) X. L. Wang, H. Y. Zhao, H. Y. Lin, G. C. Liu, J. N. Fang and B. K. Chen, Electroanalysis, 2008, 20, 1055 CrossRef CAS.
-
(a) B. Keita and L. Nadjo, J. Electroanal. Chem., 1987, 227, 77 CrossRef CAS;
(b) X.-L. Wang, B. Mu, H.-Y. Lin and G.-C. Liu, J. Organomet. Chem., 2011, 696, 2313 CrossRef CAS PubMed;
(c) X. L. Wang, J. Luan, H. Y. Lin, Q. L. Lu, C. Xu and G. C. Liu, Dalton Trans., 2013, 42, 8375 RSC.
Footnote |
† Electronic supplementary information (ESI) available. CCDC 926484, 926485 and 926482. For ESI and crystallographic data in CIF or other electronic format see DOI: 10.1039/c4ra09876e |
|
This journal is © The Royal Society of Chemistry 2014 |
Click here to see how this site uses Cookies. View our privacy policy here.