DOI:
10.1039/C4AY01940G
(Paper)
Anal. Methods, 2015,
7, 253-259
A high-throughput method for the determination of quinolones in different matrices by ultra-high performance liquid chromatography with fluorescence detection
Received
18th August 2014
, Accepted 27th October 2014
First published on 31st October 2014
Abstract
Ultra-high performance liquid chromatography coupled to fluorescence detection has been proposed for the determination of thirteen quinolones of human and veterinary use (danofloxacin, sarafloxacin, difloxacin, flumequine, norfloxacin, pipemidic acid, enoxacin, lomefloxacin, marbofloxacin, ciprofloxacin, enrofloxacin, moxifloxacin and oxolinic acid). Sample treatment consisted of a modified method based on salting-out assisted liquid–liquid extraction, which involves the use of magnesium sulphate and sodium chloride as salting-out agents. To demonstrate the applicability of the method, it was characterized for three different matrices of interest (milk, urine and environmental water), obtaining very low limits of quantification (0.2–192 μg L−1). The precision of the method was evaluated in terms of repeatability and intermediate precision, and the results were acceptable in all cases (relative standard deviations lower than 11%). Recovery studies were performed on the three matrices, obtaining values between 71% and 104%.
Introduction
Quinolones are some of the most used antibiotics in human medicine, mainly to treat urinary infections. They are also widely applied in veterinary medicine to treat and prevent bacterial infections and as a consequence, residues in foodstuffs can appear, causing allergic reactions or antibiotic resistance in humans. As an example, when the time allowed between animal drug administration and milking is too short, quinolone residues may be found in milk.1 Consequently, the European Union (EU) has established maximum residue limits (MRLs) for several quinolones in foodstuffs of animal origin through the Commission Regulation no. 37/2010
2 and subsequent amendments.
On the other hand, and regarding human treatments, quinolones are excreted with urine mostly unchanged. So, their determination in this matrix allows the performance of pharmacokinetic studies.
Moreover, pharmaceuticals (including antibiotics) used in livestock production and human medicine can reach the environment via domestic and hospital sewage, industrial discharges or from medicated domestic animals among other sources [http://www.epa.gov/ppcp/]. These products can potentially contaminate the surface, ground, and wastewater and even drinking water.3 Their frequent detection in aquatic environments has led to their consideration as “emerging pollutants”.4,5 Among them, water contamination with quinolones has been reported in different publications at concentrations from ng L−1 to mg L−1.6–10 Therefore, milk, human urine and environmental water are three matrices of interest regarding contamination by quinolones, and reliable and efficient methods for their monitoring are required.
In the last few years, different approaches have been reported for the determination of quinolones, such as micellar liquid chromatography (MLC) with fluorescence detection (FL),11,12 capillary liquid chromatography (capillary LC) with laser induced fluorescence detection (LIF),13 capillary electrophoresis with UV-Vis,14–17 mass spectrometry (MS)18 or chemiluminescence detection.19,20 However, the most usually reported methods are those based on LC with either FL or UV-Vis, which have been applied for the determination of quinolones in milk,21–25 urine26,27 and water;26,28 LC coupled to tandem mass spectrometry (MS/MS) has also been widely applied for the determination of quinolones in water,29–31 including multiresidue analysis with other drug contaminants,32 and in milk.33–39 In the last few years, ultra-high performance liquid chromatography (UHPLC) coupled to MS/MS has been applied for determining these contaminants in milk,39–41 biological fluids42 and water.6,43–47 UHPLC has also been coupled to high resolution mass spectrometry for the multiresidue determination of antibiotics, including quinolones.48–53 Nevertheless, there are few methods reporting the coupling of UHPLC with FL for the determination of quinolones.46
Regarding sample treatment, solid-phase extraction (SPE) is by far the most frequently reported technique for the extraction of quinolones from milk,18,23,38,39,41,49,52 urine19 and water6,17,28,29,32,43,44,46 and even used as an on-line sample treatment technique in LC.26 Other sample preparations include liquid–liquid extraction (LLE)11,15,21,33,35 and QuEChERS for milk analysis;13,37 dispersive liquid–liquid microextraction (DLLME),45 ultrasound-assisted extraction (USE)31 or microwave-assisted extraction (MAE)31 for water analysis; or MEPS® SGE Micro Extraction by Packed Sorbent for urine.27
However, more efficient, multiclass, rapid and environmentally friendly extraction systems are demanding. An increasingly popular treatment technique is the so called salting-out assisted liquid–liquid extraction (SALLE). This technique is based on LLE, in which the addition of an appropriate amount of a salt to a mixture of an aqueous sample and water-miscible organic solvent causes separation of the solvent from the mixture and thus the formation of a two-phase system and simultaneously the target analytes are separated into the organic phase.54 The method is simple, fast, cheap and safe and the obtained extracts could be directly injected or evaporated and reconstituted into a suitable solvent before being injected into HPLC, CE or GC instruments. Some of the organic solvents used in SALLE are acetonitrile, acetone, ethyl acetate and isopropanol and the salts commonly used are magnesium sulphate, ammonium sulphate, calcium chloride, potassium carbonate and calcium sulphate.55 This methodology has been scarcely used for the determination of quinolones.36,47,53,56 However, SALLE has never been applied in combination with UHPLC–FL.
In this work, we propose a method based on UHPLC–FL for the simultaneous determination of 13 quinolones of veterinary and/or human use – danofloxacin (DANO), enrofloxacin (ENRO), ciprofloxacin (CIPRO), sarafloxacin (SARA), difloxacin (DIFLO), flumequine (FLUME), enoxacin (ENO), oxolinic acid (OXO), moxifloxacin (MOXI), lomefloxacin (LOME), marbofloxacin (MARBO), pipemidic acid (PIPE), norfloxacin (NOR) –, using SALLE as a sample treatment technique with magnesium sulphate and sodium chloride as salting-out agents and 5% formic acid in acetonitrile (MeCN) as an extraction solvent. To demonstrate the applicability of the method it was fully validated in three different matrices of interest: bovine milk, human urine and environmental water sampled at the exit of a fish farm. The combination of this sample treatment with a high efficiency technique such as UHPLC–FL is an environmentally friendly alternative to the determination of quinolones, as the consumption of organic solvents is reduced in both steps of the method (sample treatment and determination), being in agreement with the new trends of green analytical chemistry.57,58
Material and methods
Chemicals and reagents
All reagents were of analytical reagent grade, solvents were of LC grade and quinolones were of analytical standard grade. MeCN, sodium hydroxide, sodium chloride, magnesium sulphate and sodium dihydrogen phosphate monohydrate were supplied by Panreac (Madrid, Spain). Formic acid was obtained from Merck (Darmstadt, Germany).
Standards of DANO, SARA, and DIFLO were supplied by Riedel-de Haën (Seelze, Germany); FLUME, NOR, PIPE, LOME and ENO by Sigma-Aldrich (St. Louis, MO, USA); and MARBO, CIPRO, ENRO, MOXI and OXO by Fluka (Steinheim, Germany). Individual stock standard solutions (100 mg L−1) of each quinolone were prepared by dissolving the appropriate amount of each analyte in MeCN/0.02% formic acid aqueous solution (50/50) and were stored in the dark at −20 °C.
A 0.1 M phosphate buffer solution (pH 7.1) was prepared by dissolving an adequate amount of NaH2PO4·H2O in water and the pH was adjusted with 4 M NaOH solution.
Ultrapure water (18.2 MΩ cm−1, Milli-Q Plus system, Millipore Bedford, MA, USA) was used throughout the work.
Syringe filters (25 mm with a 0.2 μm nylon membrane from Agela Technologies, DE, USA) were used for filtration of extracts prior to the injection into the chromatographic system, while nylon filters (47 mm, 0.2 μm from Supelco, Bellefonte, PA, USA) were used for filtration of water samples.
Samples
Bovine milk samples were collected from local markets (Granada, Spain). Urine samples were collected from a healthy male volunteer and obtained within institutional guidelines. Water samples were collected at the exit of a fish farm located in Granada (Spain), using pre-cleaned amber glass bottles. The samples were filtered to eliminate the suspended solid matter and macro-particle impurities. All the samples were stored in a freezer at −18 °C until analysis.
Instruments and equipment
Chromatographic separation and detection were performed on an XLC Extreme Pressure LC system (two pumps, oven, auto sampler, mixer and degasser units) from Jasco (Easton, MD, USA) coupled to a fluorescence detector (Jasco X-LC 3120FP). ChromNAV software (1.09.03 version, Jasco) was used for data acquisition and processing.
The separation of the quinolones was achieved using a Poroshell 120 EC-C18 column (50 × 2.1 mm, 2.7 μm) from Agilent Technologies (Waldbronn, Germany).
A Universal 320R centrifuge (HettichZentrifugen, Tuttlingen, Germany), a vortex-2 Genie (Scientific Industries, Bohemia, NY, USA) and an evaporator system (System EVA-EC, VLM GmbH, Bielefeld, Germany) were also used for sample preparation. A pH-meter with a resolution of ±0.01 pH unit (Crison model pH 2000, Barcelona, Spain) was also used.
Sample treatment
The extraction of quinolones from water, urine and milk samples was achieved using a SALLE procedure, based on partitioning via salting-out. A volume of 5 mL of different samples (urine, milk and filtered water) was place in a 50 mL screw cap test tube with conical bottom and the pH was adjusted to 7 by adding 5 mL of 0.1 M phosphate buffer solution. Then, 10 mL of 5% formic acid in MeCN was added and the mixture was homogenized in a vortex mixer. The extraction kit (containing 1 g of NaCl and 4 g of MgSO4) was added and the tube was shaken vigorously for 1 min. The sample was centrifuged at 9000 rpm and 4 °C for 5 min and 4 mL of the upper MeCN layer was transferred to a vial, dried at 35 °C under a stream of nitrogen and re-dissolved in 0.5 mL of H2O/MeCN/formic acid (88/10/2), considering the nature of the compounds in terms of solubility and the composition of the mobile phase.47 Before injection into the UHPLC system, the obtained extracts were filtered in order to reduce the risk of column blockage.
UHPLC–FL analysis
Separation was performed in a partially porous column (Poroshell 120 EC-C18, 50 × 2.1 mm, 2.7 μm). The mobile phase consisted of a 0.1% formic acid aqueous solution pH 4.75 (solvent A), and MeCN (solvent B). Gradient mode was selected with the following program: 0 min, 5% B; 6 min, 21% B; 7 min, 90% B. Finally it was back to 5% B in 1 min and maintained for 3 min for column equilibration. The flow rate was 500 μL min−1, column oven temperature was 35 °C and injection volume was 5 μL.
Detection was achieved using a fluorescence detector with the following multi-wavelength excitation/emission program: λex = 278 nm and λem = 466 nm from the start to 6.5 min for detection of all quinolones except FLUME, which was detected using λex = 325 nm and λem = 366 nm. The fluorimeter worked at gain × 100.
Results and discussion
Optimization of sample preparation
The sample treatment was based on partitioning via salting-out, similar to the first step of the QuEChERS sample preparation. The SALLE procedure was adapted from a method proposed in our laboratory for the determination of quinolones in water by UHPLC–MS/MS.47 To carry out an efficient extraction of the analytes, pH 7 was required, so the pH of the sample was adjusted using 0.1 M phosphate buffer solution. The percentage of formic acid in the extraction solvent was optimized (0–5%), and 5% formic acid was selected as optimum. Moreover, the volume of the MeCN supernatant layer to be dried after the SALLE (between 1 and 4 mL) and the volume of solution for the reconstitution before injection (between 0.5 and 1 mL) were optimized, in order to achieve the limits of quantification (LOQs) as low as possible and compatible with minimizing matrix effects. Finally, 4 mL of the upper MeCN layer was transferred to a vial, dried at 35 °C under a stream of nitrogen and re-dissolved in 0.5 mL of H2O/MeCN/formic acid (88/10/2).
Although in most cases a clean-up step was not necessary, as extracts were clean enough for quantification purposes, PIPE and NOR could not be determined in urine samples, due to the existence of interference peaks co-migrating with the analytes. This problem could be probably avoided by using MS as a more selective detection system47 but further work should be carried out for the study of the matrix effect for this kind of sample.
A typical chromatogram corresponding to a spiked water sample under the optimum conditions is shown in Fig. 1, showing the effectiveness of the SALLE procedure in the determination of quinolones.
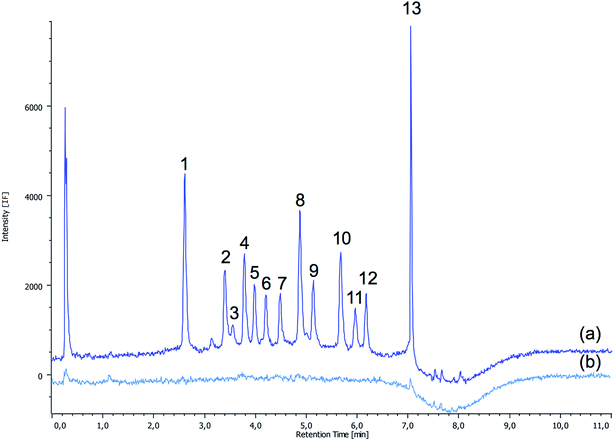 |
| Fig. 1 Chromatograms of: (a) the water sample subjected to the proposed method: sample spiked with 100 μg L−1 of PIPE, MARBO, OXO, MOXI, and FLUME; 250 μg L−1 of ENO; 10 μg L−1 of NOR, CIPRO, LOME, ENRO, SARA, and DIFLO; 1 μg L−1 of DANO; (b) blank sample. (1: PIPE, 2: MARBO, 3: ENO, 4: NOR, 5: CIPRO, 6: LOME, 7: DANO, 8: ENRO, 9: SARA, 10: DIFLO, 11: OXO, 12: MOXI, and 13: FLUME.) | |
Characterization of the method
In order to check the suitability of the method for the determination of thirteen quinolones in milk, urine and environmental water samples, an exhaustive characterization was carried out. So, linear dynamic ranges, limits of detection (LODs) and quantification (LOQs), precision and trueness were evaluated for each matrix.
Calibration curves and performance characteristics
Calibration curves were obtained using milk, urine and water samples spiked at eight different concentration levels, prepared in duplicate, submitted to the subsequent sample treatment and injected in triplicate. The statistical parameters were calculated by least-square regression, and LODs and LOQs were considered as 3 × S/N ratio and 10 × S/N ratio, respectively. Satisfactory determination coefficients confirmed that analytical responses were linear over the studied ranges. Table 1 summarizes the results.
Table 1 Statistics and performance characteristics of the method for each matrix
Matrix |
Analyte |
Linear range (μg L−1) |
R
2
|
LOD (μg kg−1) |
LOQ (μg kg−1) |
Milk |
PIPE |
4.8–1500 |
0.997 |
1.4 |
4.7 |
MARBO |
30–1500 |
0.998 |
8.7 |
29 |
ENO |
99–1500 |
0.996 |
29 |
96 |
NOR |
0.33–150 |
0.997 |
0.10 |
0.32 |
CIPRO |
1.8–150 |
0.998 |
0.52 |
1.8 |
LOME |
1.4–150 |
0.996 |
0.42 |
1.4 |
DANO |
0.17–15 |
0.996 |
0.05 |
0.17 |
ENRO |
0.79–150 |
0.997 |
0.23 |
0.77 |
SARA |
2.4–150 |
0.996 |
0.70 |
2.3 |
DIFLO |
1.2–150 |
0.997 |
0.34 |
1.2 |
OXO |
15–1500 |
0.993 |
4.3 |
15 |
MOXI |
16–1500 |
0.998 |
4.7 |
16 |
FLUME |
11–1500 |
0.997 |
3.1 |
11 |
Matrix |
Analyte |
Linear range (μg L−1) |
R
2
|
LOD (μg L−1) |
LOQ (μg L−1) |
Urine |
MARBO |
28–1500 |
0.998 |
8.4 |
28 |
ENO |
192–1500 |
0.981 |
58 |
192 |
CIPRO |
2.6–150 |
0.992 |
0.78 |
2.6 |
LOME |
2.3–150 |
0.990 |
0.68 |
2.3 |
DANO |
0.47–15 |
0.995 |
0.14 |
0.47 |
ENRO |
1.8–150 |
0.998 |
0.56 |
1.8 |
SARA |
5.0–150 |
0.997 |
1.5 |
5.0 |
DIFLO |
2.4–150 |
0.996 |
0.70 |
2.4 |
OXO |
67–1500 |
0.995 |
20 |
67 |
MOXI |
45–1500 |
0.997 |
13 |
45 |
FLUME |
17–1500 |
0.998 |
5.0 |
17 |
Water |
PIPE |
5.8–1500 |
0.993 |
1.8 |
5.8 |
MARBO |
11–1500 |
0.992 |
3.4 |
11 |
ENO |
136–1500 |
0.979 |
40 |
136 |
NOR |
1.0–150 |
0.996 |
0.30 |
1.0 |
CIPRO |
3.1–150 |
0.978 |
0.94 |
3.1 |
LOME |
1.8–150 |
0.991 |
0.55 |
1.8 |
DANO |
0.29–15 |
0.995 |
0.09 |
0.29 |
ENRO |
0.72–150 |
0.991 |
0.22 |
0.72 |
SARA |
2.9–150 |
0.988 |
0.87 |
2.9 |
DIFLO |
1.0–150 |
0.994 |
0.30 |
1.0 |
OXO |
21–1500 |
0.993 |
6.2 |
21 |
MOXI |
22–1500 |
0.985 |
6.5 |
22 |
FLUME |
6.0–1500 |
0.991 |
1.8 |
6.0 |
With the low LOQs obtained, the quinolones with an established MRL in milk (namely, DANO, MARBO, ENRO and CIPRO) could be determined at concentrations lower than limits established by the current legislation (75 μg kg−1 for MARBO, 100 μg kg−1 for the sum of CIPRO and ENRO, 30 μg kg−1 for DANO and 50 μg kg−1 for FLUME; DIFLO and OXO application is forbidden for animal produced milk intended for human consumption2).
Although the LOQs were slightly higher for urine, they were far below the concentrations of quinolones usually found in this matrix after oral administration (mg L−1 range).42,59
Regarding the LOQs obtained for environmental waters, they were low enough to allow their quantification in this type of environmental samples.
Precision study
The precision of the whole method was evaluated in terms of repeatability (intraday precision) and reproducibility (interday precision). The repeatability was assessed for milk, urine and water samples by application of the whole procedure on the same day to samples spiked at three different concentration levels of each quinolone (except ENO in urine, where only two concentrations were tested, because the lowest concentration level considered was below the LOQ for this analyte). Each sample was processed in triplicate and injected also in triplicate. The reproducibility was evaluated with a similar procedure, spiking and analysing five samples in different days. The results, expressed as %RSD of peak areas are shown in Table 2. In all cases, values lower than 11% were obtained.
Table 2 Precision study (%RSD of peak areas)a,b,c
Matrix |
Analyte |
Repeatability (n = 9) |
Reproducibility (n = 15) |
Level 1 |
Level 2 |
Level 3 |
Level 1 |
Level 2 |
Level 3 |
Level 1: PIPE, MARBO, OXO, MOXI and FLUME: 50 μg L−1; ENO: 100 μg L−1; NOR, CIPRO, LOME, ENRO, SARA, and DIFLO: 5 μg L−1; DANO 0.5 μg L−1; OXO in urine: 100 μg L−1.
Level 2: PIPE, MARBO, ENO, OXO, MOXI and FLUME: 250 μg L−1; NOR, CIPRO, LOME, ENRO, SARA, and DIFLO: 25 μg L−1; DANO 2.5 μg L−1.
Level 3: PIPE, MARBO, ENO, OXO, MOXI and FLUME: 750 μg L−1; NOR, CIPRO, LOME, ENRO, SARA, and DIFLO: 75 μg L−1; DANO 7.5 μg L−1.
|
Milk |
PIPE |
7.2 |
3.8 |
4.5 |
8.8 |
5.6 |
7.3 |
MARBO |
7.5 |
7.7 |
3.6 |
9.0 |
7.7 |
7.8 |
ENO |
8.8 |
6.8 |
8.9 |
9.2 |
10.5 |
9.9 |
NOR |
5.9 |
3.2 |
4.1 |
9.7 |
3.6 |
6.4 |
CIPRO |
4.9 |
2.6 |
4.7 |
9.3 |
7.8 |
8.9 |
LOME |
7.3 |
3.7 |
4.6 |
9.6 |
6.6 |
7.0 |
DANO |
7.5 |
3.9 |
4.8 |
8.5 |
9.3 |
8.1 |
ENRO |
6.6 |
2.9 |
4.0 |
8.2 |
6.6 |
8.8 |
SARA |
5.7 |
3.9 |
4.8 |
9.2 |
4.6 |
7.0 |
DIFLO |
5.8 |
3.6 |
3.5 |
10.1 |
5.9 |
7.2 |
OXO |
6.7 |
4.4 |
7.2 |
9.3 |
7.2 |
9.0 |
MOXI |
2.4 |
3.9 |
5.5 |
6.6 |
5.5 |
10.5 |
FLUME |
7.2 |
5.8 |
7.6 |
8.8 |
6.2 |
5.8 |
Urine |
MARBO |
8.7 |
8.1 |
4.1 |
9.8 |
9.9 |
5.2 |
ENO |
— |
7.4 |
6.2 |
— |
9.4 |
8.9 |
CIPRO |
8.9 |
5.1 |
5.3 |
9.8 |
9.6 |
6.8 |
LOME |
9.2 |
7.5 |
6.3 |
10.9 |
7.5 |
8.5 |
DANO |
7.3 |
5.9 |
4.0 |
10.2 |
8.2 |
7.2 |
ENRO |
7.7 |
9.2 |
4.2 |
9.8 |
9.1 |
5.7 |
SARA |
9.9 |
6.4 |
3.5 |
8.6 |
6.9 |
7.3 |
DIFLO |
9.4 |
8.2 |
3.5 |
8.0 |
9.9 |
6.3 |
OXO |
8.4 |
5.6 |
3.2 |
9.5 |
9.5 |
9.3 |
MOXI |
9.0 |
4.3 |
6.0 |
10.0 |
7.6 |
9.8 |
FLUME |
4.9 |
6.0 |
2.8 |
5.1 |
8.7 |
3.6 |
Water |
PIPE |
3.9 |
4.2 |
3.0 |
7.8 |
4.3 |
7.8 |
MARBO |
8.0 |
5.6 |
1.9 |
8.7 |
8.3 |
5.0 |
ENO |
9.7 |
9.3 |
5.7 |
9.8 |
9.6 |
6.1 |
NOR |
7.6 |
4.7 |
2.9 |
8.3 |
5.7 |
6.6 |
CIPRO |
9.5 |
9.2 |
3.3 |
9.5 |
10.2 |
6.7 |
LOME |
8.3 |
8.6 |
3.0 |
8.6 |
9.3 |
5.3 |
DANO |
5.4 |
6.5 |
3.7 |
5.6 |
10.3 |
7.4 |
ENRO |
7.7 |
3.9 |
3.7 |
8.3 |
6.5 |
4.9 |
SARA |
7.0 |
6.0 |
5.4 |
7.9 |
9.3 |
5.8 |
DIFLO |
7.2 |
5.7 |
2.5 |
8.5 |
10.2 |
4.8 |
OXO |
6.9 |
8.5 |
7.5 |
7.2 |
8.7 |
8.9 |
MOXI |
7.8 |
4.2 |
6.6 |
7.0 |
6.3 |
8.0 |
FLUME |
3.9 |
4.8 |
6.7 |
4.3 |
5.3 |
9.4 |
Recovery studies
In order to check the trueness of the proposed method, recovery experiments were carried out on bovine milk, human urine and water samples, previously analysed in order to check the presence of quinolones. None of them gave a positive result above the LODs of the method. These samples were spiked at three different concentration levels (except ENO in urine, avoiding the lowest level, and PIPE and NOR in urine, because of the interferences found), processed as described previously and injected in triplicate into the UHPLC–FL system. The results are shown in Table 3 and as can be seen, very good recoveries were obtained (between 71.2% and 103.8%).
Table 3 Recovery (%) study (% RSD of peak areas is given in parentheses, n = 9)a,b,c
Analyte |
Milk |
Urine |
Water |
Level 1 |
Level 2 |
Level 3 |
Level 1 |
Level 2 |
Level 3 |
Level 1 |
Level 2 |
Level 3 |
Level 1: PIPE, MARBO, OXO, MOXI and FLUME: 50 μg L−1; ENO: 100 μg L−1; NOR, CIPRO, LOME, ENRO, SARA, and DIFLO: 5 μg L−1; DANO 0.5 μg L−1; OXO in urine: 100 μg L−1.
Level 2: PIPE, MARBO, ENO, OXO, MOXI and FLUME: 250 μg L−1; NOR, CIPRO, LOME, ENRO, SARA, and DIFLO: 25 μg L−1; DANO 2.5 μg L−1.
Level 3: PIPE, MARBO, ENO, OXO, MOXI and FLUME: 750 μg L−1; NOR, CIPRO, LOME, ENRO, SARA, and DIFLO: 75 μg L−1; DANO 7.5 μg L−1.
|
PIPE |
85.9 (7.2) |
94.3 (3.8) |
82.5 (4.5) |
— |
— |
— |
87.2 (3.9) |
79.9 (4.2) |
94.6 (3.0) |
MARBO |
86.5 (7.5) |
82.8 (7.7) |
84.2 (3.6) |
94.1 (8.7) |
96.1 (8.1) |
84.5 (4.1) |
87.2 (8.0) |
100.8 (5.6) |
86.6 (1.9) |
ENO |
85.5 (8.8) |
80.2 (6.8) |
86.0 (8.9) |
— |
74.7 (7.4) |
90.8 (6.2) |
81.3 (9.7) |
93.2 (9.3) |
89.2 (5.7) |
NOR |
81.0 (5.9) |
83.1 (3.2) |
86.3 (4.1) |
— |
— |
— |
99.7 (7.6) |
89.1 (4.7) |
71.2 (2.9) |
CIPRO |
92.8 (4.9) |
81.6 (2.6) |
89.8 (4.7) |
102.2 (8.9) |
95.8 (5.1) |
85.2 (5.3) |
90.1 (9.5) |
85.2 (9.2) |
80.0 (3.3) |
LOME |
83.7 (7.3) |
86.1 (3.7) |
82.3 (4.6) |
98.9 (7.7) |
92.9 (7.5) |
89.8 (6.3) |
93.5 (8.3) |
96.2 (8.6) |
80.2 (3.0) |
DANO |
82.3 (7.5) |
82.1 (3.9) |
83.8 (4.8) |
87.6 (7.3) |
103.8 (5.9) |
84.4 (4.0) |
84.6 (5.4) |
92.6 (6.5) |
94.8 (3.7) |
ENRO |
80.0 (6.6) |
85.0 (2.9) |
85.7 (4.0) |
95.1 (7.7) |
96.4 (9.2) |
84.3 (4.2) |
85.9 (7.7) |
98.0 (3.9) |
91.4 (3.7) |
SARA |
87.1 (5.7) |
85.1 (3.9) |
81.9 (4.8) |
87.8 (9.9) |
96.7 (6.4) |
86.3 (3.5) |
81.1 (7.0) |
84.6 (6.0) |
84.0 (5.4) |
DIFLO |
97.9 (5.8) |
88.5 (3.6) |
85.6 (3.5) |
83.7 (9.4) |
102.9 (8.2) |
84.8 (3.5) |
71.6 (7.2) |
99.4 (5.7) |
83.7 (2.5) |
OXO |
87.7 (6.7) |
88.8 (4.4) |
81.6 (7.2) |
92.7 (8.4) |
102.1 (5.6) |
82.4 (3.2) |
71.2 (6.9) |
95.8 (8.5) |
91.6 (7.5) |
MOXI |
83.1 (2.4) |
86.3 (3.9) |
83.3 (5.5) |
90.6 (9.0) |
95.6 (4.3) |
88.6 (6.0) |
72.2 (7.8) |
96.1 (4.2) |
86.3 (6.6) |
FLUME |
85.9 (7.2) |
87.8 (5.8) |
81.6 (7.6) |
84.8 (4.9) |
95.2 (6.0) |
81.2 (2.8) |
72.4 (3.9) |
89.5 (4.8) |
92.3 (6.7) |
Conclusions
A rapid and simple UHPLC–FL method using SALLE as the sample treatment technique has been proposed for the determination of 13 quinolones in milk and water samples, and 11 quinolones in urine. The quinolones were separated and detected in less than 7 min. To the best of our knowledge, this is the first time that SALLE and UHPLC–FL have been combined for the determination of quinolones. The method has been fully validated in the three matrices. Matrix-matched calibration curves were established and the LODs and LOQs were in all cases below the maximum levels established by EU regulation for milk; in the case of urine, the LOQs were far below the concentrations of quinolones usually found in urine after oral administration and quantification in the low parts per billion range was achieved for water samples. The combination of SALLE as the sample treatment technique with the high efficiency and sensitivity provided by the UHPLC–FL coupling makes the proposed method a simple, quick and an environmentally friendly alternative to the determination of quinolones in a great variety of samples, reducing drastically the consumption of organic solvents in both steps of the method (sample treatment and determination). In spite of the lower LOQs obtained with the previous UHPLC–MS/MS method for the control of quinolone residues in water samples,47 the proposed method is presented as a less expensive and powerful methodology when MS is not available, showing satisfactory results in its application to other complex matrixes such as milk or urine.
Acknowledgements
NAM thanks the Andalusia Government for a postdoctoral grant. JFHP thanks the Ministry of Economy and Competitiveness of the Spanish Government for a Juan de la Cierva postdoctoral contract. The authors gratefully acknowledge the financial support of the Andalusia Government (Excellence Project Ref: P12-AGR-1647).
Notes and references
- A. Junza, S. Barbosa, M. R. Codony, A. Jubert, J. Barbosa and D. Barrón, J. Agric. Food Chem., 2014, 62, 2008 CrossRef CAS PubMed.
- Commission Regulation (EU) no. 37/2010 on pharmacologically active substances and their classification regarding maximum residue limits in foodstuffs of animal origin, 2010, L15, 1.
- Z. Q. Ye, H. S. Weinberg and M. T. Meyer, Anal. Chem., 2007, 79, 1135 CrossRef CAS PubMed.
- N. J. Morley, Environ. Toxicol. Pharmacol., 2009, 27, 161 CrossRef CAS PubMed.
- V. Christen, S. Hickmann, B. Rechenberg and K. Fent, Aquat. Toxicol., 2010, 96, 167 CrossRef CAS PubMed.
- A. Jia, Y. Wan, Y. Xiao and J. Hu, Water Res., 2012, 46, 387 CrossRef CAS PubMed.
- R. López-Roldán, M. López de Alda, M. Gros, M. Petrovic, J. Martín-Alonso and D. Barceló, Chemosphere, 2010, 80, 1337 CrossRef PubMed.
- M. J. Martínez-Bueno, M. J. Gómez, S. Herrera, M. D. Hernando, A. Agüera and A. R. Fernández-Alba, Environ. Pollut., 2012, 164, 267 CrossRef PubMed.
- F. Adachi, A. Yamamoto, K. I. Takakura and R. Kawahara, Sci. Total Environ., 2013, 444, 508 CrossRef CAS PubMed.
- W. Li, Y. Shi, L. Gao, J. Liu and Y. Cai, Chemosphere, 2012, 89, 1307 CrossRef CAS PubMed.
- M. Rambla-Alegre, M. A. Collado-Sánchez, J. Esteve-Romero and S. Carda-Broch, Anal. Bioanal. Chem., 2011, 400, 1303 CrossRef CAS PubMed.
- M. Rambla-Alegre, J. Esteve-Romero and S. Carda-Broch, J. Chromatogr. B: Anal. Technol. Biomed. Life Sci., 2009, 877, 3975 CrossRef CAS PubMed.
- M. Lombardo-Agüí, L. Gámiz-Gracia, C. Cruces-Blanco and A. M. García-Campaña, J. Chromatogr. A, 2011, 1218, 4966 CrossRef PubMed.
- I. S. Ibarra, J. A. Rodríguez, M. E. Páez-Hernández, E. M. Santos and J. M. Miranda, Electrophoresis, 2012, 33, 2041 CrossRef CAS PubMed.
- L. Vera-Candioti, A. C. Olivieri and H. C. Goicoechea, Talanta, 2010, 82, 213 CrossRef CAS PubMed.
- A. R. Solangi, M. I. Bhanger, S. Q. Memon, M. Y. Khuhawar and A. Mallah, J. AOAC Int., 2009, 92, 1382 CAS.
- M. R. Alcaráz, L. Vera-Candioti, M. J. Culzoni and H. C. Goicoechea, Anal. Bioanal. Chem., 2014, 406, 2571 CrossRef PubMed.
- F. J. Lara, A. M. García-Campaña, F. Alés-Barrero, J. M. Bosque-Sendra and L. E. García-Ayuso, Anal. Chem., 2006, 78, 7665 CrossRef CAS PubMed.
- Z. Fu, Y. Liu, L. Wang and Y. Wang, Chromatographia, 2009, 69, 1101 CAS.
- Y. M. Liu, Y. X. Jia and W. Tian, J. Sep. Sci., 2008, 31, 3765 CrossRef CAS PubMed.
- H. J. Cho, H. Yi, S. M. Cho, D. G. Lee, K. Cho, A. M. Abd El-Aty, J. H. Shim, S. H. Lee, J. Y. Jeong and H. C. Shin, J. Sep. Sci., 2010, 33, 1034 CrossRef CAS PubMed.
- M. M. Zheng, R. Gong, X. Zhao and Y. Q. Feng, J. Chromatogr. A, 2010, 1217, 2075 CrossRef CAS PubMed.
- M. D. Marazuela and M. C. Moreno-Bondi, J. Chromatogr. A, 2004, 1034, 25 CrossRef CAS PubMed.
- A. L. Cinquina, P. Roberti, L. Giannetti, F. Longo, R. Draisci, A. Fagiolo and N. R. Brizioli, J. Chromatogr. A, 2003, 987, 221 CrossRef CAS.
- N. Bilandžić, B. S. Kolanović, I. Varenina, G. Scortichini, L. Annunziata, M. Brstilo and N. Rudan, Food Control, 2011, 22, 1941 CrossRef PubMed.
- F. J. Lara, M. del Olmo-Iruela and A. M. García-Campaña, J. Chromatogr. A, 2013, 1310, 91 CrossRef CAS PubMed.
- S. Rani, A. Kumar, A. K. Malik and B. Singh, J. AOAC Int., 2012, 95, 261 CrossRef CAS PubMed.
- M. D. Gil García, A. Belmonte Gallegos, R. Santiago Valverde and M. Martínez Galera, J. Sep. Sci., 2012, 35, 823 CrossRef PubMed.
- J. L. Zhou, K. Maskaoui and A. Lufadeju, Anal. Chim. Acta, 2012, 731, 32 CrossRef CAS PubMed.
- M. H. Wang and S. P. Wang, J. Sep. Sci., 2012, 35, 702 CrossRef CAS PubMed.
- N. Dorival-García, A. Zafra-Gómez, F. J. Camino-Sánchez, A. Navalón and J. L. Vílchez, Talanta, 2013, 106, 104 CrossRef PubMed.
- J. L. Zhou and Y. Kang, J. Sep. Sci., 2013, 36, 564 CrossRef CAS PubMed.
- R. Pereira Lopes, D. Vasconcellos Augusti, F. Alves Santos, E. Azevedo Vargas and R. Augusti, Anal. Methods, 2013, 5, 5121 RSC.
- K. Bousova, H. Senyuva and K. Mittendorf, Food Addit. Contam., 2012, 29, 1901 CrossRef CAS PubMed.
- M. Gaugain-Juhel, B. Delepine, S. Gautier, M. P. Fourmond, V. Gaudin, D. Hurtaud-Pessel, E. Verdon and P. Sanders, Food Addit. Contam., 2009, 26, 1459 CrossRef CAS PubMed.
- T. Nakajima, C. Nagano, M. Kanda, H. Hayashi, T. Hashimoto, S. Kanai, Y. Matsushima, Y. Tateishi, T. Sasamoto and I. Takano, Food Hyg. Saf. Sci., 2013, 54, 335 CrossRef CAS.
- E. Karageorgou, A. Myridakis, E. G. Stephanou and V. Samanidou, J. Sep. Sci., 2013, 36, 2020 CrossRef CAS PubMed.
- D. A. Bohm, C. S. Stachel and P. Gowik, J. Chromatogr. A, 2009, 1216, 8217 CrossRef CAS PubMed.
- A. Junza, R. Amatya, D. Barrón and J. Barbosa, J. Chromatogr. B: Anal. Technol. Biomed. Life Sci., 2011, 879, 2601 CrossRef CAS PubMed.
- C. Robert, N. Gillard, P. Y. Brasseur, G. Pierret, N. Ralet, M. Dubois and Ph. Delahaut, Food Addit. Contam., 2013, 30, 443 CrossRef CAS PubMed.
- H. Zhang, Y. Ren and X. Bao, J. Pharm. Biomed. Anal., 2009, 49, 367 CrossRef CAS PubMed.
- R. Cazorla-Reyes, R. Romero-González, A. Garrido-Frenich, M. A. Rodríguez-Maresca and J. L. Martínez-Vidal, J. Pharm. Biomed. Anal., 2014, 89, 203 CrossRef CAS PubMed.
- F. Tamtam, F. Mercier, J. Eurin, M. Chevreuil and B. Le Bot, Anal. Bioanal. Chem., 2009, 393, 1709 CrossRef CAS PubMed.
- E. Gracia-Lor, J. V. Sancho, R. Serrano and F. Hernández, Chemosphere, 2012, 87, 453 CrossRef CAS PubMed.
- A. V. Herrera-Herrera, J. Hernández-Borges, T. M. Borges-Miquel and M. A. Rodríguez-Delgado, J. Pharm. Biomed. Anal., 2013, 75, 130 CrossRef CAS PubMed.
- M. Seifrtová, J. Aufartová, J. Vytlacilová, A. Pena, P. Solich and L. Nováková, J. Sep. Sci., 2010, 33, 2094 CrossRef PubMed.
- M. Lombardo-Agüí, C. Cruces-Blanco, A. M. García-Campaña and L. Gámiz-Gracia, J. Sep. Sci., 2014, 37, 2145 CrossRef PubMed.
- D. Ortelli, E. Cognard, P. Jan and P. Edder, J. Chromatogr. B: Anal. Technol. Biomed. Life Sci., 2009, 877, 2363 CrossRef CAS PubMed.
- X. J. Deng, H.-Q. Yang, J. Z. Li, Y. Song, D. H. Guo, Y. Luo, X. N. Du and T. Bo, J. Liq. Chromatogr. Relat. Technol., 2011, 34, 2286 CrossRef CAS.
- A. Kaufmann and S. Walker, J. Chromatogr. A, 2013, 1292, 104 CrossRef CAS PubMed.
- Z. Herrera Rivera, E. Oosterink, L. Rietveld, F. Schoutsen and L. Stolker, Anal. Chim. Acta, 2011, 700, 114 CrossRef CAS PubMed.
- X. L. Hou, G. Chen, L. Zhu, T. Yang, J. Zhao, L. Wang and Y. L. Wu, J. Chromatogr. B: Anal. Technol. Biomed. Life Sci., 2014, 962, 20 CrossRef CAS PubMed.
- A. Kaufmann, P. Butcher, K. Maden, S. Walker and M. Widmer, Anal. Chim. Acta, 2014, 820, 56 CrossRef CAS PubMed.
- G. G. Noche, M. E. Fernández Laespada, J. L. Pérez Pavón, B. Moreno Cordero and S. Muniategui Lorenzo, J. Chromatogr. A, 2011, 1218, 6240 CrossRef CAS PubMed.
- J. Liu, M. Jiang, G. Li, L. Xu and M. Xie, Anal. Chim. Acta, 2010, 679, 74 CrossRef CAS PubMed.
- Q. Xia, Y. Yang and M. Liu, Spectrochim. Acta, Part A, 2012, 96, 358 CrossRef CAS PubMed.
- M. Tobiszewski, A. Mechlińska and J. Namieśnik, Chem. Soc. Rev., 2010, 39, 2869 RSC.
- A. Gałuszka, Z. Migaszewski and J. Namieśnik, TrAC, Trends Anal. Chem., 2013, 50, 78 CrossRef PubMed.
- S. Wei, J. Lin, H. Li and J. M. Lin, J. Chromatogr. A, 2007, 1163, 333 CrossRef CAS PubMed.
|
This journal is © The Royal Society of Chemistry 2015 |
Click here to see how this site uses Cookies. View our privacy policy here.