DOI:
10.1039/C4AY02197E
(Paper)
Anal. Methods, 2015,
7, 244-252
Simultaneous determination and pharmacokinetic study of P-hydroxybenzaldehyde, 2,3,5,4′-tetrahydroxystilbene-2-O-β-glucoside, emodin-8-O-β-D-glucopyranoside, and emodin in rat plasma by liquid chromatography tandem mass spectrometry after oral administration of Polygonum multiflorum
Received
22nd September 2014
, Accepted 30th October 2014
First published on 19th November 2014
Abstract
A rapid and specific liquid chromatography tandem mass spectrometry (LC-MS/MS) method was developed for the simultaneous analysis of P-hydroxybenzaldehyde, 2,3,5,4′-tetrahydroxystilbene-2-O-β-glucoside, emodin-8-O-β-D-glucopyranoside, and emodin in rat plasma, and it was applied to the pharmacokinetics (PK) studies of the four compounds from the herb Polygonum multiflorum. The analysis was carried out on a Phenomenex Hydro-RP C18 (150 × 2.0 mm, 4 μm) reversed-phase column by gradient elution with a flow rate of 0.4 mL min−1. All of the analytes including internal standards (I.S.) were monitored by selected reaction monitoring with an electrospray ionization source. Linear responses were obtained for P-hydroxybenzaldehyde, 2,3,5,4′-tetrahydroxystilbene-2-O-β-glucoside, emodin-8-O-β-D-glucopyranoside, and emodin ranging from 1 to 1000 ng mL−1. The intra-day and inter-day precisions (RSD) were less than 8.61% and 8.75%, respectively. The extraction recovery ranged from 77.00 ± 5.54 to 91.30 ± 2.96, and the I.S. was 85.82 ± 3.58%. Stability studies showed that the accuracies of P-hydroxybenzaldehyde, 2,3,5,4′-tetrahydroxystilbene-2-O-β-glucoside, emodin-8-O-β-D-glucopyranoside, and emodin ranged from 94.66 ± 3.54 to 106.84 ± 6.91; the matrix effects ranged from 92.14 ± 3.63 to 103.98 ± 8.71. The validated method was successfully used to determine the concentration–time profiles of P-hydroxybenzaldehyde, 2,3,5,4′-tetrahydroxystilbene-2-O-β-glucoside, emodin-8-O-β-D-glucopyranoside, and emodin.
1. Introduction
Polygonum multiflorum, also known as fo-ti or Hou Shou Wu, is one of the most popular traditional Chinese medicines, and it is an ingredient in many Chinese patent medicines and prescriptions. It was widely used in China for many centuries to treat various diseases commonly associated with aging. Recent research proved that it exhibits antioxidative activity,1–4 mainly due to the flavonoid and the phenolic acid constituents. The pharmacological effects of stilbene in P. multiflorum have been reported to be anti-aging5–10 and liver-protective.11,12 Anthraquinones, another main component of this plant, also have many biological activities, such as anti-cancer,13–19 anti-developmental anomalies,20 and anti-tension.21 Recently, there have been an increasing number of reports on the adverse effects of P. multiflorum, with the herb exhibiting not only hepatotoxicity, but also bone marrow suppression and a possible drug interaction with warfarin. The long-term use of P. multiflorum may lead to hepatoxicity and renal toxicity, which have been described in detail by the U.S. National Toxicology Program.22
P. multiflorum contains the compounds P-hydroxybenzaldehyde, 2,3,5,4′-tetrahydroxystilbene-2-O-β-glucoside, and emodin, a cathartic, and emodin-8-O-β-D-glucopyranoside. In this study, a rapid, specific, and sensitive LC-MS/MS method was developed and validated for simultaneous determination of P-hydroxybenzaldehyde, 2,3,5,4′-tetrahydroxystilbene-2-O-β-glucoside, emodin-8-O-β-D-glucopyranoside, and emodin in rat plasma using hyperin as an internal standard (I.S.), and it was applied to the pharmacokinetics (PK) study after oral administration of an extract of P. multiflorum.
2. Experimental
2.1. Chemicals and reagents
Emodin and 2,3,5,4′-tetrahydroxystilbene-2-O-β-glucoside were purchased from Shanghai Standard Biotech Co., Ltd. (Shanghai, China), hyperin was purchased from the National Institute for the Control of Pharmaceutical and Biological Products (Beijing, China), and emodin-8-O-β-D-glucopyranoside and P-hydroxybenzaldehyde were purchased from Shanghai Yuanye Bio-Technology Co., Ltd. (Shanghai, China). The purities of all standards were not less than 98% and were suitable for LC-MS/MS analysis. Methanol (HPLC-grade) was purchased from Fisher Scientific (Fair Lawn, NJ, USA). HPLC-quality water was obtained using a Cascada™ IX water purification system purchased from Pall Corporation (Port Washington, NY, USA). Polygonum multiflorum was purchased from Beijing Tongrentang Co., Ltd (Beijing, China). Solid phase extraction (SPE) C18-Max (100 mg mL−1; 100 pk; phase type: octadecyl; surface area: 531 m2 g−1; pore diameter: 60 Å; pore volume: 0.8 cm3 g−1; average particle size: 50.9 μ) cartridges were purchased from Grace Corporation (Deerfield, IL, USA). All the other chemicals were analytical grade and used without further purification.
2.2. Instrumentation and chromatography
Identification and quantification of analytes were carried out using an Agilent 1290 (Agilent, USA) liquid chromatograph equipped with a QQQ (Agilent 6460, USA) tandem mass spectrometer. The separation of all samples was obtained with a Phenomenex Hydro-RP C18 reversed-phase column (150 × 2.0 mm, 4 μm). The auto-sampler was set at 20 °C, and the gradient elution was employed with 0.1% formic acid methanol solution as solvent A and 0.1% formic acid aqueous solution as solvent B. The gradient program was as follows: 0–1 min, 20–40% A; 1–4 min, 40–60% A; 4–5 min, 60–100% A; 5–7 min, 100% A; 7–7.1 min, 100–20% A; 7.1–10 min, 20% A. The flow rate was set at 0.4 mL min−1, and the injection volume was 10 μL. The total run time was 10 min for each sample.
Negative ion ionization was used for the detection of P-hydroxybenzaldehyde, 2,3,5,4′-tetrahydroxystilbene-2-O-β-glucoside, emodin-8-O-β-D-glucopyranoside, emodin, and the I.S. due to their higher sensitivity than that of the positive mode. The TIC chromatograms and chemical structures of P-hydroxybenzaldehyde, 2,3,5,4′-tetrahydroxystilbene-2-O-β-glucoside, emodin-8-O-β-D-glucopyranoside, emodin, and the I.S. are shown in Fig. 1. The nebulizer was set at 45 psi, and the capillary was at 3500 V. High-purity nitrogen served as both the nebulizing and dry gas. The gas temperature was held at 350 °C, and the gas flow rate was 8 L min−1. Other parameters were also optimized for maximum sensitivity (Table 1).
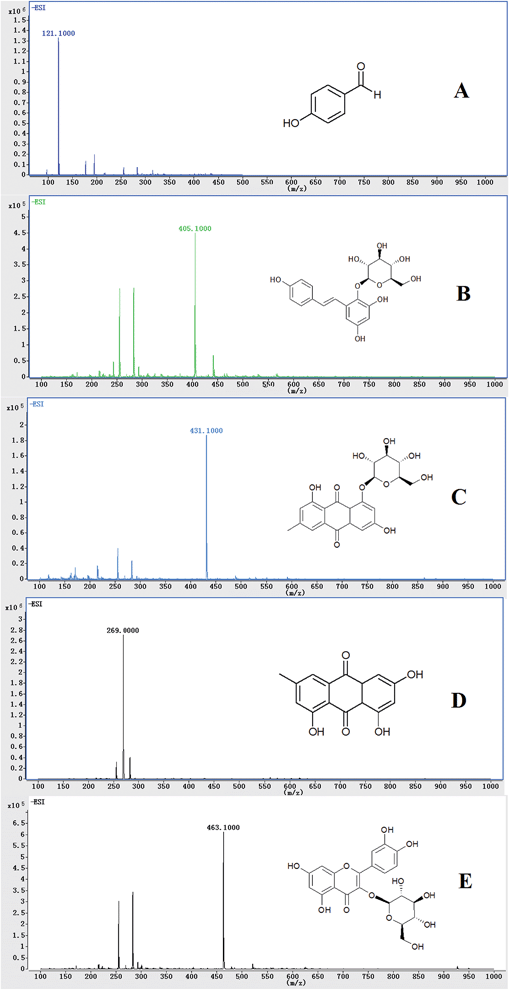 |
| Fig. 1 MS spectra and chemical structures of P-hydroxybenzaldehyde (A), 2,3,5,4'-tetrahydroxystilbene-2-o-β-glucoside (B), emodin-8-o-β-D-glucopyranoside (C), emodin (D), and I.S. (E). | |
Table 1 Optimized multiple reaction monitoring parameters for the detection of analytes and internal standards (*quantitative ion)
Compound |
Precursor ion (m/z) |
Product ion (m/z) |
Fragmentor |
CE |
Dwell |
P-hydroxybenzaldehyde |
121 |
92* |
80 |
20 |
100 |
2,3,5,4′-tetrahydroxystilbene-2-O-β-glucoside |
405 |
243* |
135 |
10 |
100 |
|
143 |
|
60 |
|
Emodin-8-O-β-D-glucopyranoside |
431 |
269* |
180 |
20 |
100 |
|
224 |
|
50 |
|
Emodin |
269 |
225* |
135 |
20 |
100 |
|
195 |
|
40 |
|
Hyperin (I.S.) |
463 |
300* |
160 |
20 |
100 |
|
271 |
|
40 |
|
2.3. Preparation of calibration standards and quality control (QC) samples
The primary stock solutions of P-hydroxybenzaldehyde, 2,3,5,4′-tetrahydroxystilbene-2-O-β-glucoside, emodin-8-O-β-D-glucopyranoside, emodin, and the I.S. were dissolved in methanol at a concentration of 0.1 mg mL−1 and stored at −20 °C. The maximum preservation time was 30 days. A series of working standard solutions of analytes ranging from 10 ng mL−1 to 10 μg mL−1 and the I.S. solution at 5 μg mL−1 were prepared by diluting their respective stock solutions with methanol–water (1
:
4, v/v). Calibration standards were prepared using blank rat plasma spiked with P-hydroxybenzaldehyde, 2,3,5,4′-tetrahydroxystilbene-2-O-β-glucoside, emodin-8-O-β-D-glucopyranoside, and emodin working solutions to yield concentrations of 1 to 1000 ng mL−1.
QC samples were prepared in the same way as the calibration samples, representing three levels of concentrations of P-hydroxybenzaldehyde, 2,3,5,4′-tetrahydroxystilbene-2-O-β-glucoside, emodin-8-O-β-D-glucopyranoside, and emodin in plasma at 5, 100, and 1000 ng mL−1 respectively.
2.4. Sample preparation
An aliquot of 100 μL rat plasma was transferred to a 1.5 mL plastic tube to which was added 10 μL I.S. for processing. After vortexing for 30 s, the plasma sample was added to the SPE cartridge, which was preconditioned with methanol and then water. The cartridge was washed with 1.0 mL water, sucked dry, and eluted with 1.0 mL aliquots of methanol; the flow rate was 1 mL min−1. The eluate was evaporated to dryness under a gentle stream of N2 at 40 °C using a Zymark TurboVap LV drying system (Westborough, MA, USA). The residue was reconstituted with 100 μL methanol. 10 μL of the sample was injected into the LC-MS/MS system for analysis.
2.5. Method validation
This method was validated according to the International Conference on Harmonization of Technical Requirements for Registration of Pharmaceuticals for Human Use (ICH) document with respect to selectivity, linearity, precision, accuracy, recovery, matrix effects, and stability.
2.5.1. Selectivity.
The selectivity study was performed to investigate whether endogenous constituents and other substances existing in samples will interfere with the detection of analytes and the I.S. The selectivity of this method was established by comparing the blank plasma from six different sources that contain analytes to the I.S., particularly to detect interference from endogenous components.
2.5.2. Linearity and LLOQ.
The linearity was determined by the observed peak area ratios of analytes to the I.S. versus the spiked concentrations of analytes based on calibration curves that contained at least six points. The curves were fitted by a weighted least-squares linear regression method through the measurement of the peak-area ratio of the analytes to the I.S. The acceptance criterion for a calibration curve was a correlation coefficient (r) of 0.99 or better. The deviation criteria of these back-calculated concentrations from the spiked concentrations were set within ±15%, except for the LLOQ (lower limit of quantification), which was defined as the lowest concentration in the calibration curve with acceptable relative standard deviation that was within 20% and accuracy within 100 ± 20%.
2.5.3. Precision and accuracy.
Precision and accuracy assays were carried out in six replicates at three QC levels on the same day and three batches on three consecutive validation days. The assay accuracy was expressed as (observed concentration/nominal concentration) × 100%. The intra- and inter-day precisions were expressed as the relative standard deviation (RSD). The precisions did not exceed 15%, and the accuracies were within 85–115%.
2.5.4. Extraction recovery and matrix effect.
The extraction recoveries of P-hydroxybenzaldehyde, 2,3,5,4′-tetrahydroxystilbene-2-O-β-glucoside, emodin-8-O-β-D-glucopyranoside, emodin, and the I.S. were determined by comparing the responses from blank plasma samples spiked with analytes and I.S. before extraction with those from blank plasma samples spiked after extraction. This experiment was performed at three QC levels with six replicates. Matrix effects (ME) were evaluated by comparing the peak areas of post-extraction blank plasma spiked with concentrations of QC samples with the areas obtained by direct injection of corresponding standard solutions.
2.5.5. Stability.
The stability of the analytes in plasma was investigated under the following conditions: 24 h at 20 °C, three freeze–thaw cycles, stored at −20 °C for a month, and post-preparation stored at 20 °C for 24 h. The analytes were considered stable when 85–115% of the initial concentrations was found.
2.6. Pharmacokinetics
2.6.1. Animals.
Male Sprague-Dawley rats (250 g ± 10 g) were obtained from Vital River Laboratories (Beijing, P.R. China). Rats were housed in a temperature- and humidity-controlled environment and maintained on a 12 hour light/dark cycle for three days with free access to food and water. All experimental procedures followed the National Institutes of Health Guide for the Care and Use of Laboratory Animals and were approved by the Committee on Animal Care and Usage of the Beijing University of Chinese Medicine.
2.6.2. Dosing and sampling.
100 g of P. multiflorum was decocted with 70% ethanol (1000 mL) twice, each time for 1.5 h. The extractions were combined and concentrated to 100 mL. The concentrated solution was orally administered to six rats at a dose of 10 g per kg of body weight, which was computed based on the quantity of crude material. The dose was equivalent to 20 times the upper dosage for humans as described in the Chinese Pharmacopoeia. This is a common hepatotoxic dose of P. multiflorum.23–27
Blood samples (0.5 mL) were collected from the ocular fundus veins of rats before administration and after 5, 10, 20, 30, 45 min, 1, 2, 4, 8, 12, and 24 h. The blood samples were immediately centrifuged at 6
000 rpm for 10 min to separate out plasma and then stored at −20 °C until analysis.
2.6.3. Pharmacokinetic data analysis.
The pharmacokinetic parameters of P-hydroxybenzaldehyde, 2,3,5,4′-tetrahydroxystilbene-2-O-β-glucoside, emodin-8-O-β-D-glucopyranoside, and emodin were calculated with Kinetica 4.4 software (Thermo Scientific, USA). Non-compartmental analysis was used to determine standard pharmacokinetic parameters of analytes. The data are presented as the mean ± SD.
3. Results and discussion
3.1. Method development
Mobile phases (methanol–water, acetonitrile–water, methanol–0.1% formic acid aqueous solution, methanol–0.01% ammonia aqueous solution, and 0.1% formic acid methanol solution–0.1% formic acid aqueous solution) were examined and compared in order to obtain good chromatographic behavior and appropriate ionization. It was observed that the 0.1% formic acid methanol solution–0.1% formic acid aqueous solution provided the best performance, and this is in accordance with previous studies that determined that adding 0.1% formic acid in the mobile phase can improve the detection sensitivity of the instrument for the analytes.28–30 The gradient elution of the mobile phase was used for narrowing the peaks, improving the resolution of analytes and the I.S., and decreasing the chromatographic running time.
MS spectra were studied in both positive and negative modes. The negative mode was employed in our study, as it had better sensitivity of ion response than the positive mode.28–30 The CE and fragmentor value were optimized based on the intensity of the product ion of analytes.
It is necessary to use an I.S. to obtain high accuracy while performing MS quantitation. An appropriate I.S. will control variability in extraction, HPLC injection, and ionization. In this study, we compared the response of rhapontin and hyperin, and the result reflected that there was increased response and sensitivity of hyperin as compared to rhapontin. Therefore, hyperin was finally selected as the I.S. due to its structural similarity with the analytes and high recovery.
3.2. Method validation
3.2.1. Specificity.
This study confirms the specificity and matrix effect of this method. The specificity of the method was evaluated by analyzing individual blank plasma samples from six different sources. All samples were found to have no interferences from endogenous substances at the retention times of either the analytes or the I.S. The result is shown in Fig. 2.
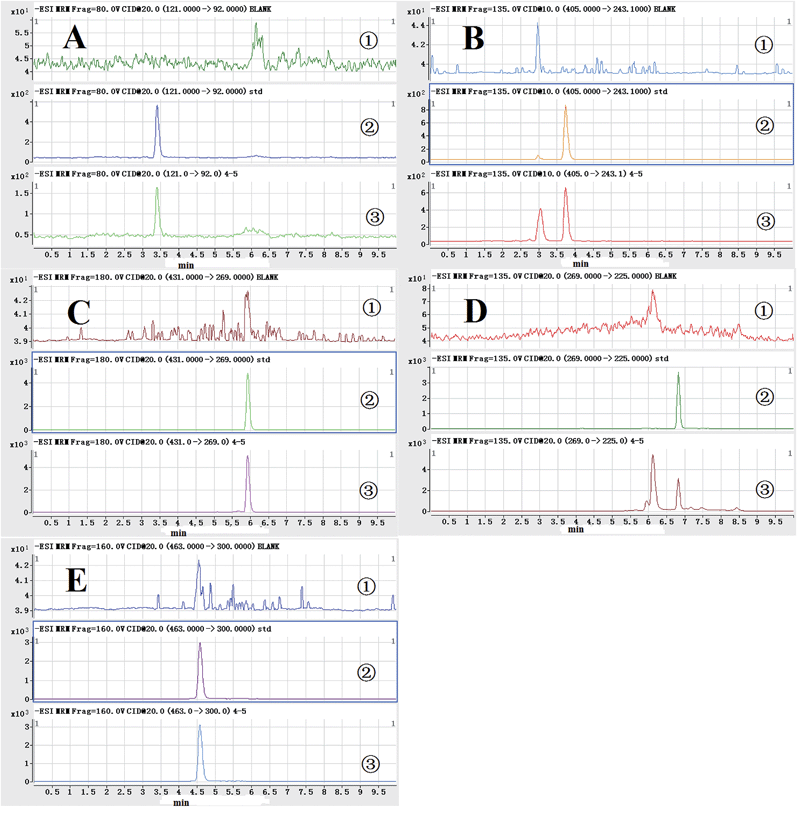 |
| Fig. 2 Representative MRM chromatograms of P-hydroxybenzaldehyde (A), 2,3,5,4′-tetrahydroxystilbene-2-O-β-glucoside (B), emodin-8-O-β-D-glucopyranoside (C), emodin (D), and I.S. (E). ① Blank plasma; ② Blank plasma spiked four analytes (100 ng mL−1) and I.S. ③ Plasma sample after oral administration for 45 min. | |
3.2.2. Linearity and LLOQ.
In the present study, calibration curves were established within the range of 1–1000 ng mL−1, representing a high correlation coefficient, and each range has at least six concentrations. The typical equation of the calibration curves is shown in Table 2, where Y is the peak-area ratio of analytes to I.S. and X is the plasma concentration of analytes, respectively.
Table 2 The calibration curves and LLOQ for P-hydroxybenzaldehyde, 2,3,5,4′-tetrahydroxystilbene-2-O-β-glucoside, emodin-8-O-β-D-glucopyranoside, and emodin. The data are represented as the mean ± SD, n = 3
Compound |
Typical equations |
R
2
|
Weight |
Concentration range |
LLOQ (n = 3) |
Found conc. |
Accuracy (%) |
Precision (RSD%) |
P-hydroxybenzaldehyde |
Y = 0.7694 × X + 0.0046 |
0.9974 |
1/X |
1–1000 ng |
0.93 ± 0.095 |
93.33 ± 9.47 |
10.15 |
2,3,5,4′-tetrahydroxystilbene-2-O-β-glucoside |
Y = 1.0063 × X + 0.0098 |
0.9981 |
1/X |
1–1000 ng |
1.02 ± 0.069 |
101.57 ± 6.86 |
6.75 |
Emodin-8-O-β-D-glucopyranoside |
Y = 6.0385 × X + 0.0049 |
0.9991 |
1/X |
1–1000 ng |
0.90 ± 0.083 |
90.29 ± 8.32 |
9.22 |
Emodin |
Y = 3.8954 × X + 0.0100 |
0.9997 |
1/X |
1–1000 ng |
0.97 ± 0.056 |
96.69 ± 5.63 |
5.82 |
The LLOQ for P-hydroxybenzaldehyde, 2,3,5,4′-tetrahydroxystilbene-2-O-β-glucoside, emodin-8-O-β-D-glucopyranoside, and emodin was 1 ng mL−1, which is sensitive enough for the pharmacokinetic study of these compounds in rat plasma. The precision and accuracy at this concentration level were acceptable, as shown in Table 2.
3.2.3. Precision and accuracy.
The intra-day and inter-day precision and accuracy of the method were investigated by analyzing QC samples (5, 100, and 1000 ng mL−1). All the values are shown in Table 3. The intra-day RSD was below 8.61%, and the inter-day RSD was below 8.75%. The accuracies were within 93.0–106.0%. The method was proved to be highly accurate and precise.
Table 3 Accuracy and precision for the analysis of P-hydroxybenzaldehyde, 2,3,5,4′-tetrahydroxystilbene-2-O-β-glucoside, emodin-8-O-β-D-glucopyranoside, and emodin in rat plasma
Compound |
Added concentration (ng mL−1) |
Intra-day (n = 6) |
Inter-day (n = 18) |
Found concentration (ng mL−1) (mean ± SD) |
Accuracy (%) |
Precision (RSD%) |
Found concentration (ng mL−1) (mean ± SD) |
Accuracy (%) |
Precision (RSD%) |
P-hydroxybenzaldehyde |
5 |
5.20 ± 0.45 |
104.08 ± 8.96 |
8.61 |
5.27 ± 0.34 |
105.37 ± 6.80 |
6.45 |
100 |
107.64 ± 4.93 |
107.64 ± 4.93 |
4.58 |
107.95 ± 4.98 |
107.95 ± 4.98 |
4.62 |
1000 |
999.01 ± 34.11 |
99.90 ± 3.41 |
3.41 |
1022.15 ± 41.50 |
102.25 ± 4.15 |
4.06 |
2,3,5,4′- Tetrahydroxystilbene-2-O-β-glucoside |
5 |
4.75 ± 0.31 |
95.08 ± 6.21 |
6.53 |
5.10 ± 0.45 |
102.00 ± 8.92 |
8.75 |
100 |
97.14 ± 6.26 |
97.14 ± 6.26 |
6.44 |
99.36 ± 5.17 |
99.36 ± 5.17 |
5.20 |
1000 |
982.34 ± 24.08 |
98.23 ± 2.41 |
2.45 |
984.45 ± 29.90 |
98.45 ± 2.99 |
3.04 |
Emodin-8-O-β-D-glucopyranoside |
5 |
4.81 ± 0.29 |
96.23 ± 5.85 |
6.08 |
4.91 ± 0.31 |
98.19 ± 6.33 |
6.45 |
100 |
95.91 ± 3.18 |
95.91 ± 3.18 |
3.32 |
97.57 ± 5.51 |
97.57 ± 5.51 |
5.64 |
1000 |
951.31 ± 24.32 |
95.13 ± 2.43 |
2.56 |
938.41 ± 47.09 |
93.84 ± 4.71 |
5.02 |
5 |
4.85 ± 0.31 |
97.00 ± 6.22 |
6.41 |
4.80 ± 0.28 |
95.97 ± 5.78 |
5.92 |
Emodin |
100 |
100.88 ± 2.78 |
100.88 ± 2.78 |
2.76 |
102.42 ± 3.28 |
102.42 ± 3.28 |
3.20 |
1000 |
995.81 ± 31.88 |
99.58 ± 3.19 |
3.20 |
1018.35 ± 32.86 |
101.83 ± 3.29 |
3.23 |
3.2.4. Extraction recovery and matrix effect.
The absolute extraction recoveries and matrix effects (n = 6) of P-hydroxybenzaldehyde, 2,3,5,4′-tetrahydroxystilbene-2-O-β-glucoside, emodin-8-O-β-D-glucopyranoside, emodin, and I.S. are shown in Table 4. No matrix components in plasma led to significant changes in the MS/MS responses to P-hydroxybenzaldehyde, 2,3,5,4′-tetrahydroxystilbene-2-O-β-glucoside, emodin-8-O-β-D-glucopyranoside, emodin, or the I.S. Overall, these results indicate that no endogenous substances significantly influence recovery or ionization of the I.S. and analytes.
Table 4 Recoveries and matrix effects of P-hydroxybenzaldehyde, 2,3,5,4′-tetrahydroxystilbene-2-O-β-glucoside, emodin-8-O-β-D-glucopyranoside, emodin, and the I.S. The data are represented as the mean ± SD, n = 6
Compound |
Compound concentration (ng mL−1) |
Recovery (%) |
Matrix effects (%) |
P-hydroxybenzaldehyde |
5 |
88.02 ± 3.97 |
99.25 ± 3.94 |
100 |
77.00 ± 5.54 |
103.98 ± 8.71 |
1000 |
91.30 ± 2.96 |
98.45 ± 2.92 |
5 |
83.45 ± 4.64 |
93.70 ± 4.60 |
2,3,5,4′-tetrahydroxystilbene-2-O-β-glucoside |
100 |
89.08 ± 4.72 |
96.48 ± 3.64 |
1000 |
89.61 ± 2.92 |
98.43 ± 4.62 |
5 |
85.69 ± 5.33 |
101.37 ± 6.96 |
Emodin-8-O-β-D-glucoPyranoside |
100 |
78.75 ± 4.72 |
99.23 ± 4.86 |
1000 |
85.00 ± 2.57 |
98.13 ± 3.58 |
5 |
85.78 ± 5.00 |
101.45 ± 1.97 |
Emodin |
100 |
88.91 ± 4.56 |
98.72 ± 4.69 |
1000 |
84.71 ± 4.62 |
96.31 ± 2.89 |
Hyperin |
500 |
85.82 ± 3.58 |
92.14 ± 3.63 |
3.2.5. Stability.
Stability studies showed that the concentrations of P-hydroxybenzaldehyde, 2,3,5,4′-tetrahydroxystilbene-2-O-β-glucoside, emodin-8-O-β-D-glucopyranoside, and emodin did not significantly change in plasma stored at −20 °C for one month, at 20 °C for 24 h, plasma that underwent three freeze–thaw cycles from −20 °C to 20 °C, and in the post-preparation samples stored at 20 °C for 24 h. All of the values are shown in Table 5.
Table 5 Stability of P-hydroxybenzaldehyde, 2,3,5,4′-tetrahydroxystilbene-2-O-β-glucoside, emodin-8-O-β-D-glucopyranoside, and emodin under various storage conditions. The data are represented as the mean ± SD, n = 6
Compound |
Concentration (ng mL−1) |
Long-term (−20 °C) |
Short-term (20 °C) |
Freeze–thaw |
Post-preparation (20 °C) |
Found concentration (ng mL−1) |
Accuracy (%) |
Found concentration (ng mL−1) |
Accuracy (%) |
Found concentration (ng mL−1) |
Accuracy (%) |
Found concentration (ng mL−1) |
Accuracy (%) |
P-hydroxybenzaldehyde |
5 |
5.23 ± 0.36 |
104.61 ± 7.15 |
5.03 ± 0.27 |
100.53 ± 5.41 |
5.11 ± 0.28 |
102.16 ± 5.66 |
5.13 ± 0.32 |
102.63 ± 6.47 |
100 |
103.22 ± 4.66 |
103.22 ± 4.66 |
105.87 ± 3.99 |
105.87 ± 3.99 |
106.84 ± 6.91 |
106.84 ± 6.91 |
104.70 ± 3.87 |
104.70 ± 3.87 |
1000 |
1017.89 ± 26.65 |
101.79 ± 2.67 |
1009.70 ± 42.75 |
100.97 ± 4.27 |
1038.16 ± 17.32 |
103.82 ± 1.73 |
1013.98 ± 16.90 |
101.40 ± 1.69 |
2,3,5,4′- |
5 |
4.84 ± 0.26 |
96.79 ± 5.11 |
4.91 ± 0.27 |
98.10 ± 4.54 |
4.77 ± 0.25 |
95.46 ± 5.00 |
4.83 ± 0.29 |
96.79 ± 5.79 |
Tetrahydroxystilbene- |
100 |
98.70 ± 4.21 |
98.70 ± 4.21 |
101.36 ± 4.79 |
101.36 ± 4.79 |
97.87 ± 3.53 |
97.87 ± 3.53 |
97.56 ± 3.77 |
97.56 ± 3.77 |
2-O-β-glucoside |
1000 |
967.24 ± 31.76 |
96.72 ± 3.18 |
986.41 ± 18.65 |
98.64 ± 1.86 |
982.24 ± 33.72 |
98.22 ± 3.37 |
964.38 ± 22.71 |
96.44 ± 2.27 |
Emodin-8-O-β-D- |
5 |
4.79 ± 0.27 |
95.83 ± 5.34 |
5.16 ± 0.15 |
103.29 ± 3.04 |
5.05 ± 0.20 |
101.01 ± 3.97 |
4.96 ± 0.21 |
99.19 ± 4.21 |
glucopyranoside |
100 |
94.66 ± 3.54 |
94.66 ± 3.54 |
98.91 ± 3.31 |
98.91 ± 3.31 |
98.06 ± 2.65 |
98.06 ± 2.65 |
101.45 ± 2.95 |
101.45 ± 2.95 |
1000 |
959.37 ± 20.73 |
95.94 ± 2.07 |
992.99 ± 33.17 |
99.30 ± 3.31 |
973.48 ± 10.41 |
97.35 ± 1.04 |
1002.80 ± 37.74 |
100.28 ± 3.77 |
5 |
4.94 ± 0.23 |
98.77 ± 4.54 |
5.17 ± 0.11 |
103.51 ± 2.16 |
5.15 ± 0.22 |
102.99 ± 4.47 |
4.87 ± 0.17 |
97.42 ± 3.42 |
Emodin |
100 |
96.03 ± 4.35 |
96.03 ± 4.35 |
102.32 ± 3.99 |
102.32 ± 3.99 |
99.92 ± 4.28 |
99.92 ± 4.28 |
101.52 ± 2.39 |
101.52 ± 2.39 |
1000 |
958.06 ± 20.20 |
95.81 ± 2.02 |
983.04 ± 29.47 |
98.30 ± 2.95 |
1019.06 ± 53.54 |
101.91 ± 5.35 |
985.12 ± 21.06 |
98.51 ± 2.11 |
3.3. Pharmacokinetics study
In the present study, the method described above was applied to the analysis of plasma samples obtained from six rats that were given an orally administered preparation of Polygonum multiflorum extract. The mean concentration time is shown in Fig. 3, and the main pharmacokinetic parameters, as derived from these data, are summarized in Table 6.
 |
| Fig. 3 The mean (± SD) plasma concentration–time profiles of P-hydroxybenzaldehyde (A), 2,3,5,4′-tetrahydroxystilbene-2-O-β-glucoside (B), emodin-8-O-β-D-glucopyranoside (C), and emodin (D) after oral administration of Polygonum multiflorum. | |
Table 6 Pharmacokinetic parameters of P-hydroxybenzaldehyde, 2,3,5,4′-tetrahydroxystilbene-2-O-β-glucoside, emodin-8-O-β-D-glucopyranoside and emodin after oral administration of PM. The data are represented as the mean ± SD, n = 6
Parameters |
Unit |
P-Hydroxybenzaldehyde |
2,3,5,4′- Tetrahydroxystilbene-2-O-β-glucoside |
Emodin-8-O-β-D- glucopyranoside |
Emodin |
T
max
|
h |
1.67 ± 0.52 |
0.70 ± 0.11 |
1.00 ± 0.59 |
0.5 ± 0.33 |
C
max
|
ng mL−1 |
36.95 ± 11.54 |
240.19 ± 114.01 |
204.48 ± 85.88 |
76.71 ± 13.19 |
AUC0–24 |
ng h mL−1 |
225.54 ± 50.16 |
368.58 ± 141.31 |
488.75 ± 130.26 |
381.21 ± 186.83 |
AUC0–∞ |
ng h mL−1 |
257.76 ± 69.16 |
373.62 ± 142.73 |
489.67 ± 129.72 |
395.21 ± 208.27 |
T1/2 |
h |
7.80 ± 2.61 |
1.59 ± 0.58 |
1.67 ± 0.51 |
6.53 ± 1.43 |
MRT |
h |
10.71 ± 3.75 |
2.32 ± 0.51 |
2.52 ± 0.23 |
9.35 ± 2.50 |
4. Conclusion
Polygonum multiflorum is one of the most important and frequently used traditional Chinese herbal medicines that possesses anti-aging, immunologic, anti-hyperlipidemia, neuroprotective, anti-cancer, and anti-inflammatory effects. P-hydroxybenzaldehyde, 2,3,5,4′-tetrahydroxystilbene-2-O-β-glucoside, emodin-8-O-β-D-glucopyranoside, and emodin are the representative compounds in it, but there is no method for simultaneous determination of them in plasma by LC-MS/MS. In this study, a novel, rapid, and specific LC-MS/MS method with high sensitivity, accuracy, and recovery was developed for the simultaneous analysis of P-hydroxybenzaldehyde, 2,3,5,4′-tetrahydroxystilbene-2-O-β-glucoside, emodin-8-O-β-D-glucopyranoside, and emodin in rat plasma. This method can provide reference values for the determinations of P-hydroxybenzaldehyde, 2,3,5,4′-tetrahydroxystilbene-2-O-β-glucoside, emodin-8-O-β-D-glucopyranoside, and emodin in other bio-matrices in future studies. Moreover, the pharmacokinetic parameters of P-hydroxybenzaldehyde, 2,3,5,4′-tetrahydroxystilbene-2-O-β-glucoside, emodin-8-O-β-D-glucopyranoside, and emodin following oral dosing would be a valuable reference for clinical applications.
References
- L. S. Lv, X. H. Gu, J. Tang and C. T. Ho, Food Chem., 2007, 104, 1678–1681 CrossRef CAS.
-
C. Y. Wang, Studies on antihyperlipidemic effects and pharmacokinetics of stilbene glycoside from radix polygoni multiflori, Hebei Medical University, Shijiazhuang, 2005 Search PubMed.
- C. Y. Wang, J. M. Gu, W. N. Liu, W. Yang and L. T. Zhang, Chin. J. Pharm. Anal., 2009, 29, 1073–1078 CAS.
- X. M. Wang, L. B. Zhao, T. Z. Han, S. F. Chen and J. L. Wang, Eur. J. Pharmacol., 2008, 578, 339–348 CrossRef CAS.
- C. O. Long and J. Dougherty, Home Healthc. Nurse, 2003, 21, 8–14 CrossRef.
- H. Q. Lin, M. T. Ho, L. S. Lau, K. K. Wong, P. C. Shaw and D. C. Wan, Chem.-Biol. Interact., 2008, 175, 352–354 CrossRef CAS.
- W. S. Chen, J. P. Xu, L. Li and C. Z. Qiao, Chin. Tradit. Herb. Drugs, 2001, 32, 39–41 CAS.
- Y. C. Chan, F. C. Cheng and M. F. Wang, J. Nutr. Sci. Vitaminol., 2002, 48, 491–497 CrossRef CAS.
- F. W. Cheung, A. W. Leung, W. K. Liu and C. T. Che, J. Nat. Prod., 2014, 77, 1270–1274 CrossRef CAS.
- M. Y. Um, W. H. Choi, J. Y. Aan, S. R. Kim and T. Y. Ha, J. Ethnopharmacol., 2006, 104, 144–148 CrossRef.
- C. H. Huang, L. Y. Homg, C. F. Chen and R. T. Wu, J. Ethnopharmacol., 2007, 114, 199–206 CrossRef.
- C. J. Liu, Q. H. Zhang and J. Lin, China J. Chin. Mater. Med., 1992, 17, 595–596 CAS.
- Y. S. Ma, S. W. Weng, M. W. Lin, C. C. Lu, J. H. Chiang, J. S. Yang, K. C. Lai, J. P. Lin, N. Y. Tang, J. G. Lin and J. G. Chung, Food Chem. Toxicol., 2012, 50, 1271–1278 CrossRef CAS.
- W. Wang, Y. Sun, X. Li, H. Li, Y. Chen, Y. Tian, J. Yi and J. Wang, Oncol. Rep., 2011, 26, 1143–1148 CAS.
- T. D. Way, J. T. Huang, C. H. Chou, C. H. Huang, M. H. Yang and C. T. Ho, Eur. J. Cancer, 2014, 50, 366–378 CrossRef CAS.
- J. Q. Yu, W. Bao and J. C. Lei, Phytother. Res., 2013, 27, 251–257 CrossRef CAS.
- M. H. Liu, X. B. Jing, X. B. Cai, S. Z. Chen and J. Y. Cai, Journal of Shantou University Medical College, 2009, 22, 12–14 Search PubMed.
- J. G. Lin, G. W. Chen, T. M. Li, S. T. Chouh, T. W. Tan and J. G. Chung, J. Urol.,, 2006, 175, 343–347 CrossRef CAS.
- C. Tabolacci, A. Lentini, P. Mattioli, B. Provenzano, S. Oliverio, F. Carlomosti and S. Beninati, Life Sci., 2010, 87, 316–324 CrossRef CAS.
- J. M. Yon, C. Lin, K. W. Oh, H. S. Baek, B. J. Lee, Y. W. Yun and S. Y. Nam, Birth Defects Res., Part B, 2013, 98, 268–275 CrossRef CAS.
- K. M. Lim, J. H. Kwon, K. Kim, J. Y. Noh, S. Kang, J. M. Park, M. Y. Lee, O. N. Bae and J. H. Chung, Br. J. Pharmacol., 2014, 171, 4300–4310 CrossRef CAS PubMed.
-
NTP technical report, NIH Publication, 2001, pp. 1–278, no. 01–3952 Search PubMed.
- X. Q. Hu, X. Q. Yang, Y. R. Xing, Y. X. Miao and H. L. Fang, J. Shaanxi Collage Tradition Chin. Med., 2006, 27, 625–626 Search PubMed.
- X. Q. Hu, H. L. Fang, Z. B. Quan and Z. Y. Geng, J. Shaanxi Collage Tradition Chin. Med., 2007, 30, 63–64 Search PubMed.
- W. Huang, Y. N. Zhang and R. Sun, Chinese Journal of Pharmacovigilance, 2011, 8, 193–197 Search PubMed.
- W. X. Li, Q. Zhang, G. R. Wang, X. L. Lin and Y. T. Peng, J. Chin. Med., 2012, 27, 595–597 Search PubMed.
- X. Q. Wu, X. Z. Chen, Q. C. Huang, D. M. Fang, G. Y. Li and G. L. Zhang, Fitoterapia, 2012, 83, 469–475 CrossRef CAS PubMed.
- L. Zhang, W. F. Ma, J. Li, J. He, P. Zhang, F. Zheng, B. L. Zhang, X. M. Gao and Y. X. Chang, J. Ethnopharmacol., 2013, 148, 246–253 CrossRef CAS PubMed.
- X. H. Qiu, J. Zhang, Z. H. Huang, D. Y. Zhu and W. Xu, J. Chromatogr. A, 2013, 1292, 121–131 CrossRef CAS PubMed.
- H. S. Lin, C. Tringali, C. Spatafora, C. Wu and P. C. Ho, J. Pharm. Biomed. Anal., 2010, 51, 679–684 CrossRef CAS PubMed.
Footnote |
† These authors contributed equally to this study and share first authorship. |
|
This journal is © The Royal Society of Chemistry 2015 |
Click here to see how this site uses Cookies. View our privacy policy here.