DOI:
10.1039/C4AY02568G
(Paper)
Anal. Methods, 2015,
7, 237-243
Utilizing LC-MS/MS to provide adaptable clinical bioanalytical support for an extended half-life bioactive peptide fused to an albumin-binding domain antibody
Received
28th October 2014
, Accepted 18th November 2014
First published on 20th November 2014
Abstract
Bioactive peptides are often unstable in the body leading to short half lives and requiring frequent dosing intervals. Linking these peptides to moieties such as albumin, fatty acids and polyethylene glycol has been shown to extend the half-lives of various therapeutics allowing less frequent dosing regimens. In this study the GLP-1 receptor agonist, therapeutic under investigation (GSK2374697), was a bioactive peptide (exendin-4) that was fused to an albumin-binding domain antibody (AlbudAb™) to increase the half-life of the therapeutic. However, developing selective quantitative methods for these molecules to provide a complete understanding of the pharmacokinetic (PK) properties using immunoassay, has proved to be challenging. Methods utilizing LC-MS/MS for the determination of GSK2374697 in human plasma were based on the selection and quantification of two surrogate peptides after enzymatic digestion using either Lys-C or trypsin. These methods were validated and used for the analysis of clinical samples from a first time in human (FTIH) study. Method validation data for both surrogate peptides indicate that the methods are rugged, accurate, precise and well suited for support of regulated clinical studies. The pharmacokinetic results obtained from the two surrogate peptides indicate that the peptide derived from the bioactive portion of the molecule has a much shorter terminal half-life than the peptide derived from the AlbudAb portion of the molecule. Development of assays for these multiple molecular fragments allowed for the accurate quantification and integrity of the molecule from different binding regions illustrating different AUCs and half lives.
Introduction
The presence of biotherapeutics in the pipelines of pharmaceutical companies has increased dramatically over the last 10 years.1 Recently the FDA has approved a number of peptides as therapies for multiple indications including diabetes mellitus type 2 (exenatide, liraglutide, lixisenatide), osteoporosis (teriparatide), congestive heart failure (nesiritide) and hormone-responsive cancer (triptorelin). However, the limited oral bioavailability and short half-lives typically associated with peptide therapeutics has led to the need to administer these drugs by subcutaneous or intravenous administration at frequent dosing intervals. To overcome these challenges, researchers have used various strategies including modification of the peptides native sequence to resist catabolism or by chemical modification (i.e. pegylation) to increase the half-life of these molecules.
One class of next generation molecules being investigated to increase the half-life of peptides, small proteins and small molecule therapeutics are engineered protein scaffolds such as domain antibodies (dAbs) that have high affinity for human serum albumin (AlbudAbs).2 These AlbudAbs are approximately 110 amino acids in length and have been found to be extremely stable and well expressed in culture. This small size would normally lead to a short half life due to rapid renal clearance but the ability of these molecules to bind to serum albumin increases the half-life to that approximating serum albumin itself.3 In addition, these molecules can be genetically or chemically fused to various peptides and proteins to increase half-life, solubility, or impart bispecific functionality to a molecule, imparting unique therapeutic pharmacokinetic characteristics.2–4
Along with the cost of discovering and developing a new drug of between $1.2 and $1.7 billion dollars,5 drug development processes requires pharmacokinetic (PK) analysis to be performed as part of safety and efficacy studies in both nonclinical species and human subjects. Currently, immunoassay is considered the bioanalytical ‘gold standard’ for the detection and quantification of biopharmaceuticals for the support of PK and toxicokinetic (TK) exposure studies. However, due to the large amount of interfering endogenous immunoglobulins present, dAbs are an analytical challenge to selectively detect using immunoassay. In addition, the bispecific nature of the AlbudAb-fusion therapeutics makes it particularly challenging to fully understand the integrity of the molecule using immunoassay alone. Recently, mass spectral (MS) assays, coupled with liquid chromatography (LC), have been shown to allow development of a robust, sensitive and selective method for a domain antibody therapeutic and expedited method development time compared to traditional immunoassay methodologies.6,7
This manuscript describes the method development and validation of LC-MS/MS methods for the determination of GSK2374697 (GLP-1 receptor agonist, peptide therapeutic genetically fused to an AlbudAb) in human plasma to support a clinical study where GSK2374697 was dosed to healthy volunteers. The developed methods allowed quantification of a Lys-C derived twelve amino acid peptide form the N-terminus of the molecule or a tryptically derived peptide from the complimentary determining region (CDR) of the molecule. This strategy allowed quantification of both active drug (from N-terminus) and drug-related material (from CDR) giving information on the integrity of the molecule that would not have been possible using a single immunoassay approach. This clinical study was approved by the GSK institutional review board and informed consent was obtained from all participants.
Results and discussion
Assay design and surrogate peptide selection
GSK2374697 consists of a bioactive GLP-1 receptor agonist peptide genetically linked to an AlbudAb moiety with a total molecular weight of ∼17 kDa (Fig. 1). Ideally, a bioanalytical method that measures the intact molecule is preferred as this eliminates concerns about catabolized or transformed forms of the molecule. However, for larger molecules (>10 kDa), it becomes increasingly difficult to use this strategy due to difficulties with sample preparation and reduction in sensitivity.4 Thus, current practice relies on enzymatic digestion of the intact molecule to produce peptide fragments (surrogate peptides) that then are quantified and used to represent a portion of or the entire molecule. Proper surrogate peptide selection is extremely important when designing the assay as modifications or truncations to that particular peptide may cause inaccurate representation of the molecule. In this case, the surrogate peptides selected were the Lys-C derived twelve amino acid peptide from the N-terminus of the molecule as the N-terminus of the peptide is necessary for bioactivity of the molecule, and the Trypsin derived six amino acid peptide from one of AlbudAbs three complimentary determining region (CDR) which is responsible for the molecules albumin binding specificity and contains drug-related material.
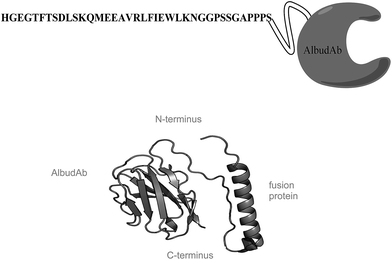 |
| Fig. 1 Graphical representation of GSK2374697 and the AlbudAb complex. | |
Internal standard selection
Even with extensive method optimization, the ability to accurately account for differences due to enzymatic digestion, sample extraction, LC injection volume, and variability needs to be addressed with internal standard selection for mass spectrometer methods. In the last several years there have been a variety of internal standard molecules used to develop quantitative assays utilizing an enzymatic digestion step for large molecule proteins including: analog proteins,4 stable isotopically labelled (SIL) peptides, SIL peptides with a N- and/or C-terminal extendion (extended sequence SIL peptides) or fully labeled peptides.8 Just as with small molecule IS selection, a closely matched internal standard will correct for assay variability.9 In addition, a fully labeled molecule allows selection of any surrogate peptide without the need for synthesis of a new labeled peptide. Unlike monoclonal antibodies, domain antibodies can be efficiently expressed in E. coli.10 This allowed the use of ISOGRO® 15N to make fully-uniformly labeled (13C15N or 15N) internal standard molecule. Initially, GSK2374697 was grown in medium with either 13C15N containing 99 atom percent 13C and 98 atom percent 15N or 15N containing 98 atom percent 15N. As the selected surrogate peptide contained 54 carbon atoms and 15 nitrogen atoms the 13C15N labeled peptide would increase in mass by 69 and the 15N labeled peptide would increase in mass by 15.
The 13C15N or 15N labeled GSK2374697 molecule was digested with Lys-C and injected onto the LC-MS/MS system monitoring for both the unlabeled peptide and the labeled version of the peptide. In both cases there was no unlabeled peptide detected, indicating at least partial incorporation of either 13C and/or 15N. However, a ten-fold difference in signal was noted between the 13C15N and the 15N labeled peptide. Likely, this was due to incomplete incorporation of the label into the protein. With this information the 15N labeled peptide was selected as the internal standard for the assay.
Assay development and validation
Following method development and assay validation, Assay A (50 to 10
000 ng mL−1) was used to quantify initial plasma samples following administration of GSK2374697 in a FTIH clinical study. After several runs it was observed that there was a large variation in IS response when comparing the standards and QCs to the samples with a greater than 10-fold difference in IS response in certain samples was observed. In addition, following clinical review of the first few subjects at the lowest dose, it was deemed necessary to lower the analytical range of the assay. To improve the IS response and develop a more sensitive method, various modifications to the method were made including increasing the sample volume from 50 to 200 μL as well as including a denaturation step by incubating the sample with 6 M guanidine and heating to 65 °C. As a result, the IS response consistency was vastly improved with no bias associated with standards, QC or study samples (Fig. 2). Assay B then was fully validated (sample chromatograms in Fig. 3, and validation statistics in Table 2) and used to quantify over 3000 samples from 10 dosing regimens in support of the FTIH study.
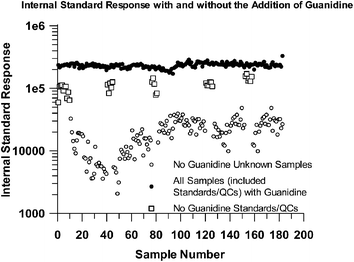 |
| Fig. 2 Internal standard variation with and without guanidine in the digestion procedure. | |
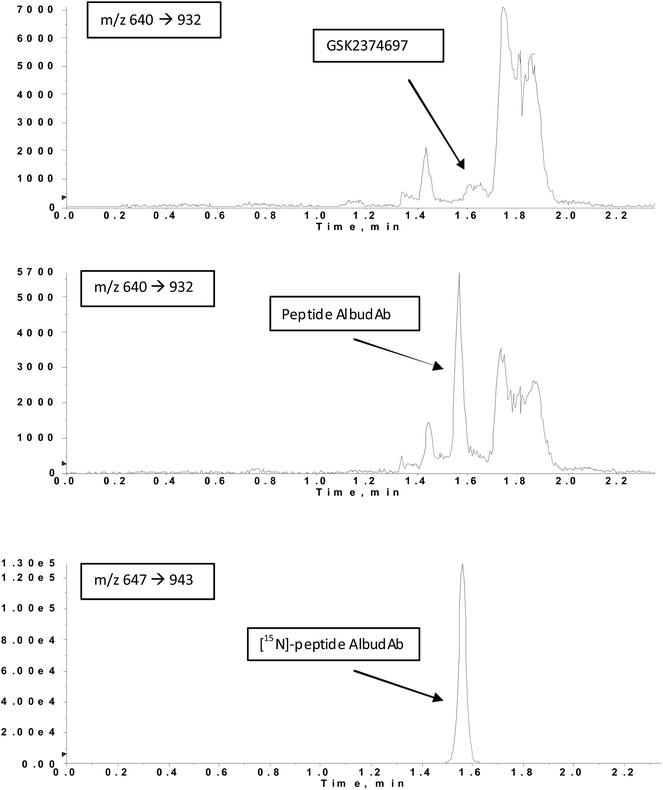 |
| Fig. 3 Example chromatograms of blank (top), LLQ of 10 ng mL−1 (middle) and internal standard (bottom). | |
Pharmacokinetic analysis
The PK parameters obtained for the half-life of GSK2374697 in human were not as expected based upon data obtained from prior TK studies in preclinical species where the N-terminal peptide was quantified using LC-MS/MS. A bioanalytical investigation using the FTIH study samples was undertaken to determine if the stability of the molecule attributed to the observed reduction in half-life. The specific peptide fragment being monitored in the original analysis (Assays A and B) correlated to the active portion of the molecule (N-terminus) conjugated to the AlbudAb. As a result, an additional assay (Assay C) was rapidly developed to monitor for a specific peptide from the complimentary determining region (CDR) of the dAb portion of the molecule. As shown in Fig. 4, the results from the reanalysis of the dAb portion of the molecule resulted in a much longer half-life compared to the active peptide. These results suggest that the active portion of the molecule is being catabolized in vivo with the AlbudAb portion of the drug at least partially intact. For additional information on the PK results and discussion regarding the clinical study please see ref. 11.
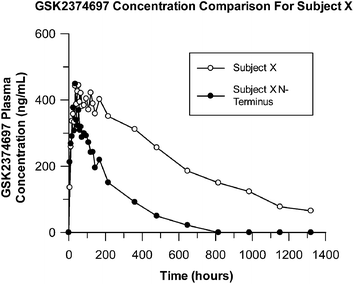 |
| Fig. 4 Concentration and half-life comparison for dAb and N-terminus cleavage and monitoring. | |
Experimental
Materials and methods
The therapeutic AlbudAb molecule, GSK2374697, was developed and manufactured by GlaxoSmithKline (GSK) and supplied at 10 mg mL−1 in sodium acetate buffer and stored at 4 °C. A 15N and a 15N/13C uniformly labeled version of GSK2374697 was prepared using ISOGRO® complex growth media (Sigma) by GlaxoSmithKline and used as the internal standard (IS) for method development, validation and sample analysis. The IS was supplied at 1 mg mL−1 in buffer and stored at −20 °C. Control whole human blood was collected from in-house GSK volunteers while control human plasma was purchased from Bioreclaimation. All study participants were presumed healthy and provided written informed consent forms. Chemicals such as sodium bicarbonate, sodium hydroxide, methanol, formic acid, isopropanol, guanidine HCL, and acetic acid were purchased from Fisher Scientific. Endoproteinase Lys-C was purchased from Roche Diagnostics. Trypsin gold was purchased from Promega. Strata XC-L 30 mg solid phase extraction (SPE) plates were purchased from Phenomenex.
Assay details
Over the course of method development and sample analysis for this clinical study, three separate assays were used. The specific assay details can be found below and are summarized in Table 1.
Table 1 Assay comparison
|
Assay |
A |
B |
C |
According to FDA guidance.
|
Assay range (ng mL−1) |
50 to 10 000 |
10 to 2500 |
10 to 2500 |
Aliquot volume |
50 |
200 |
25 |
LC run time (min) |
3 |
4 |
3.5 |
Fully validateda |
YES |
YES |
NO |
Denature reagent |
NONE |
Guanidine |
Guanidine |
Digestion reagent |
Lys-C |
Lys-C |
Trypsin gold |
Divert valve |
NO |
YES |
YES |
Monitored fragment |
N-terminus |
N-terminus |
CDR region |
Assay A sample preparation.
The initial validated assay had an analytical range from 50 to 10
000 ng mL−1 (Assay A). A 50 μL aliquot of plasma (standard, quality control, blank, or subject sample) was placed into a 1.4 mL polypropylene tube (Micronic, Aston PA), followed by 50 μL of IS. After mixing, a 75 μL aliquot of Lys-C solution (1 μg mL−1 in 100 mM sodium bicarbonate, pH 8.5) was added to all tubes for sample protein digestion. Tubes were capped, vortexed and allowed to mix gently for approximately 24 hours under ambient conditions. The following day, the Strata XL-C SPE plates were conditioned with methanol followed by 2% (v/v) formic acid in water. After SPE conditioning, formic acid was added to all samples to halt the digestion, followed by loading of the samples onto the SPE plate. The SPE plate was washed with 2% formic acid followed by methanol. After drying with vacuum, the samples were eluted using 5% ammonium hydroxide in methanol. The samples then were dried under nitrogen and reconstituted in a mixture of 80/20 0.1% formic acid/acetonitrile (v/v). LC-MS/MS conditions stated below were used to quantify the Lys-C derived N-terminal peptide.
Assay B sample preparation.
To improve the sensitivity of the assay and limit internal standard variability, Assay B was developed. Most extraction and digestion steps remained identical to Assay A with the exception of increasing the plasma volume to 200 μL and including a denaturation step by adding 0.2 mL of 6 M guanidine hydrochloride and heating at 65 °C for 30 minutes, to denature the proteins and increase digestion efficiency, prior to the addition of the Lys-C solution. LC-MS/MS conditions stated below were used to quantify the Lys-C derived N-terminal peptide.
Assay C sample preparation.
A third method (Assay C) was developed by modifying Assay B to enable different peptide fragments to be quantified. To achieve this, Lys-C was replaced with trypsin gold. Digestion with trypsin was used to obtain the specific peptide of interest from the CDR of the AlbudAb. An evaluation of the assay precision and accuracy was performed. LC-MS/MS conditions stated below were used to quantify the trypsin derived peptide.
LC configuration
Similar LC equipment was used for the three assays detailed above. The UHPLC system was an Acquity system from Waters, consisting of a pump, autosampler, sample organizer and column compartment. To minimize or eliminate any carryover, the autosampler washes consisted of 40/40/20 acetonitrile/isopropanol/0.1% formic acid in water (v/v/v) and 0.1% formic acid in water. The analytical column was a Waters Acquity 135 Å BEH C18, 1.7 μm (2.1 × 50 mm) held at 65 °C. The mobile phase consisted of linear, gradient conditions of 0.025% formic acid (mobile phase A) and 50/50 isopropanol/acetonitrile (mobile phase B) with a flow rate of 700 μL min−1. As the assay range was lowered, the LC conditions had to be modified slightly to separate various endogenous interferences. An LC-diverter valve was also incorporated with the assays that included guanidine (Assay B and C). Without the use of the divert valve, IS response dropped rapidly and significantly following the initiation of the run. With the 4 minute run time, flow was diverted to waste for the first minute, followed by flow to the MS from 1–2.5 minutes, followed by flow to waste. A make-up pump was incorporated that pumped 50/50 acetonitrile/0.1% formic acid into the MS when the LC line consisting of the analytical column was diverted to waste.
Mass spectrometry and quantification
An API5500 mass spectrometer (Applied Biosystems) with a TurboIonSpray® Electrospray interface running Analyst software version 1.5 was used for method development and validation. The following source conditions were used: ion spray voltage 5500 V, nebulizing gas 70 psi, turbo gas 70 psi, curtain gas 40, collision gas 10, declustering potential 95, collision energy 36, and temperature 750 °C. For Assay A and B, MRM transitions were monitored for the Lys-C derived doubly charged native and IS peptide, respectively: 640 double charged parent ion to 932 single charged daughter ion, and 647 double charged parent ion to 943 single charged daughter ion (daughter ions correspond to the b9 product ion fragment). The dwell time was 150 ms and Q1 and Q3 were operated in low resolution. For Assay C, MRM transitions were monitored for the tryptically derived doubly charged native and IS peptide, respectively: 416 double charged parent ion to 606 single charged daughter ion, and 421 double charged parent ion to 612 single charged daughter ion. The dwell time was 50 ms and Q1 and Q3 were operated in low resolution. The precursor ions were selected for monitoring based on the in silico analysis of GSK2374697 using either the endoproteinase enzyme Lys-C or trypsin and the daughter ions were selected to ensure high selectivity.
Linear responses in the analyte/internal standard peak area ratio(s) were observed over the range 50–10
000 (Assay A) and 10 to 2500 ng mL−1 (Assay B and C). The correlation coefficients obtained using 1/x2 weighted linear regression were better than 0.9991, 0.9968 or 0.9963, for Assay A, B, and C respectively. UHPLC MS/MS data were acquired and processed (integrated) using the proprietary software application Analyst (Version 1.5, Applied Biosystems/MDS Sciex). Concentrations of GSK2374697 in QC samples were determined from the appropriate calibration line, and used to calculate the bias and precision of the method within the Study Management System, SMS2000 (Version 2.3, GlaxoSmithKline).
Method validation
Both Assay A and B were validated according to the FDA procedures outlined in the Guidance for Industry, Bioanalytical Method Validation, May 2001 (ref. 12) and departmental Standard Operating Procedures. Assay A was used for the initial assessment (10% of study samples) of compound concentrations in the clinical samples. However it was soon discovered that the assay range was not sensitive enough to quantify at all desired timepoints. The more sensitive, Assay B, was then used for the analysis of the remaining FTIH study samples, along with re-analysis of BLQ (below limit of quantification) samples that utilized Assay A. The method validation discussion going forward will focus on Assay B. The three validation batches comprised an eight-point calibration curve extracted in duplicate with GSK2374697 calibrants at 10, 25, 50, 100, 250, 500, 1000 and 2500 ng mL−1; various blanks with and without the addition of IS; and six replicates of the following QC levels: 10, 30, 200, 2000 and 2500 ng mL−1. The precision and accuracy limits were ±15% (±20% at the lower limit of quantification; LLQ), and the statistical analysis of the validation data is presented in Table 2. The selectivity of the method was established by the analysis of blank and double blank samples of control human plasma from six individual volunteers. UHPLC MS/MS chromatograms of the blanks and validation samples were visually examined and compared for chromatographic integrity and potential interferences. Representative chromatograms of a double blank sample, validation sample at the LLQ (10 ng mL−1) and internal standard are shown in Fig. 3. No unacceptable interferences at the retention times of GSK2374697 and its internal standard were observed. Finally, the validation included stability assessment of GSK2374697 in stock solution, human plasma (room temperature, long term frozen stability and freeze thaw), human whole blood, and after processing. In addition, as mandated by various regulatory authorities, the method was investigated for incurred sample reproducibility (ISR). In this case, approximately 10% of the total study samples assayed with both Assay A and B were selected for ISR. The evaluation of bioanalytical methods through the reanalysis of incurred samples can be taken as one additional measure of assay reproducibility. ISR results were within the acceptable limits set forth by regulatory agencies (95% of the selected results were within 20% of the original), indicating assay reproducibility, stability and ruggedness. A further breakdown of the ISR results indicates that 85% of the 48 samples chosen from Assay A, and 98% of the 191 samples chosen from Assay B were within the acceptance limits, indicating the improved robustness of Assay B.
Table 2 Quality control statistics from validation for Assay B
Concentration (ng mL−1) |
10 |
30 |
200 |
2000 |
2500 |
RUN 1, n = 6
|
Mean |
9.7 |
31.1 |
192 |
1837.2 |
2252.2 |
Precision (% CV) |
13.2 |
3.0 |
1.9 |
2.1 |
2.5 |
Bias% |
−2.8 |
3.7 |
−4.0 |
−8.1 |
−9.9 |
![[thin space (1/6-em)]](https://www.rsc.org/images/entities/char_2009.gif) |
RUN 2, n = 6
|
Mean |
9.6 |
32.7 |
204.7 |
1957.2 |
2428.1 |
Precision (% CV) |
7.6 |
7.1 |
1.6 |
1.3 |
2.4 |
Bias% |
−3.7 |
8.9 |
2.4 |
−2.1 |
−2.9 |
![[thin space (1/6-em)]](https://www.rsc.org/images/entities/char_2009.gif) |
RUN 3, n = 6
|
Mean |
8.4 |
30.8 |
200.5 |
1920.8 |
2412 |
Precision (% CV) |
11.2 |
11.0 |
1.5 |
1.9 |
1.4 |
Bias% |
−15.6 |
2.6 |
0.3 |
−4.0 |
−3.5 |
![[thin space (1/6-em)]](https://www.rsc.org/images/entities/char_2009.gif) |
Overall totals, n = 18
|
Mean |
9.3 |
31.5 |
199.1 |
1905 |
2364.1 |
Precision (% CV) |
12.1 |
7.7 |
3.2 |
3.2 |
4.0 |
Bias (%) |
−7.4 |
5.1 |
−0.5 |
−4.7 |
−5.4 |
Between-run precision (%) |
6.3 |
0.7 |
3.2 |
3.1 |
4.0 |
Method qualification of Assay C included a single run consisting of duplicate calibration curves, and 6 replicates of quality control samples at 10, 30, 200, 2000, and 2500 ng mL−1. For Assay C, precision and accuracy limits were extended to ±20%. Selectivity assessment in plasma from six different volunteers was also investigated with no noted interferences.
Conclusion
We have illustrated the performance of a novel analytical method for the determination of GSK2374697 (range 10–2500 ng mL−1) in human plasma using UHPLC MS/MS. GSK2374697 was extracted from 200 μL of human plasma, after the addition of an isotopically labeled internal standard by protein digestion followed by solid phase extraction (SPE). Assay throughput, robustness, stability and other performance characteristics were found to be acceptable for clinical sample analysis. The incorporation of the LC-MS/MS based assay provided information on the AlbudAb from the N-terminus and CDR peptide region in a single assay. In addition, we have highlighted one of the key strengths of LC-MS/MS, which is the ability to develop assays in real time providing adaptability in comparison to immunoassay methods.
Contributors
Each author made significant contributions to the scholarly content of this manuscript in the following domains:
CLB: concept & design of the analyses, data analysis and interpretation, manuscript preparation and revisions in terms of important intellectual content.
JK: concept & design of the analyses, data analysis, acquisition and interpretation, manuscript preparation and revisions in terms of important intellectual content.
TM: data analysis, acquisition and interpretation, manuscript preparation.
BO: data analysis; manuscript preparation and revisions in terms of important intellectual content.
MS: data analysis, acquisition and interpretation, manuscript preparation.
All authors were critically involved in revising the manuscript and all reviewed the final manuscript and gave approval for submission.
Conflicts of interest
CLP, JK, TM, BO, MS are employed by and shareholders of GlaxoSmithKline.
Acknowledgements
The authors would like to acknowledge the following people for their scientific input and thorough manuscript review: Molly Karlinsey, Jack Chism, Lee Abberley, Christopher Herring and Christopher Evans as well as Andrew Sanderson for the preparation of the internal standards. Funding for this analysis was provided by GlaxoSmithKline.
References
- B. Hughes, Nat. Rev. Drug Discovery, 2010, 9, 89–92 CrossRef CAS PubMed.
- A. Walker, G. Dunlevy, D. Rycroft, P. Topley, L. J. Holt and T. Herbert,
et al.
, Protein Eng., Des. Sel., 2010, 4, 271–278 CrossRef PubMed.
- L. J. Holt, A. Basran, K. Jones, J. Chorlton, L. S. Jespers, N. D. Brewis and I. M. Tomlison, Protein Eng., Des. Sel., 2007, 21, 283–288 CrossRef PubMed.
- K. J. Fraley, L. Abberley, C. S. Hottenstein, J. J. Ulicne, D. R. Citerone and M. E. Szapacs, Bioanalysis, 2013, 14, 1765–1773 CrossRef PubMed.
- W. R. Strohl and D. M. Knight, Curr. Opin. Biotechnol., 2009, 20, 668–672 CrossRef CAS PubMed.
- M. E. Szapacs, J. J. Urbanski, J. R. Kehler, R. Wilson, S. L. Boram, C. S. Hottenstein and D. R. Citerone, Bioanalysis, 2010, 9, 1597–1608 CrossRef PubMed.
- M. E. Lame, E. E. Chambers and M. Blatnik, Biochemistry, 2011, 419, 133–139 CAS.
- L. Fumin, C. M. Schmerberg and Q. C. Ji, Rapid Commun. Mass Spectrom., 2009, 23, 729–732 CrossRef PubMed.
-
M. Szapacs, T. Mencken, J. Williams and Y. Li, Bioanalysis of large molecules by LC-MS/MS, Future Science Group, in press.
- L. J. Holt, C. Herring, L. S. Jespers, B. P. Woolven and I. M. Tomlinson, Trends Biotechnol., 2003, 21, 484–490 CrossRef CAS PubMed.
- R. L. O'Connor-Semmes, J. Lin, R. J. Hodge, S. Andrews, J. Chism, A. Choudhury and D. J. Nunez, Clin. Pharmacol. Ther. Ser., 2014 Search PubMed , in press.
-
US Department of Health and Human Services, Guidance for industry, bioanalytical method validation, http://www.fda.gov/downloads/Drugs/Guidances/ucm070107.pdf, accessed May 2001 Search PubMed.
|
This journal is © The Royal Society of Chemistry 2015 |
Click here to see how this site uses Cookies. View our privacy policy here.