DOI:
10.1039/C5QO00204D
(Research Article)
Org. Chem. Front., 2015,
2, 1313-1317
The development of carbene-stabilized N–O radical coupling strategy in metal-free regioselective C–H azidation of quinoline N-oxides†
Received
26th June 2015
, Accepted 19th July 2015
First published on 20th July 2015
Abstract
Although radical oxidative coupling provides a powerful C–H functionalization tool, the selective coupling of an electrophilic radical with a heterocyclic electron-deficient C2 site is still a seemingly impossible challenge. In this work, an efficient oxidative C2–H azidation of quinoline N-oxides with TMSN3 has been developed, proceeding at room temperature within 10 min. Based on control experiments and detailed EPR studies, the reaction appears to involve a novel carbene-stabilized N–O radical intermediate, which undergoes a single electron transfer to TMSN3 to give the C2-azidation product. Moreover, this procedure could be smoothly scaled up to gram-synthesis and the products could be converted into other functional heterocycles.
Introduction
Radicals involved in oxidative cross-couplings have played important roles in organic synthesis; in particular, the direct coupling of two different radicals represents a powerful approach to building new chemical bonds.1 The persistent radical effect (PRE) is widely used in radical coupling reactions; therefore, prolonging the radical lifetime would be a challenging goal.2
Recently, carbene-stabilized radicals and radical ions have been intensively developed3 because carbenes are more efficient for stabilizing radicals through better delocalization of the unpaired electron.4 The N–O radicals have found wide applications in chemistry and biology.5 Heterocyclic N-oxides might undergo a single electron oxidation to form an N–O radical cation6 which could possibly transform into a neighboring carbene-stabilized N–O radical via deprotonation. If so, this transformation could convert the electron-deficient C2-site into an electron-rich carbene nucleophile which would possibly undergo coupling with an electrophilic azide radical or a single electron transfer to TMSN3 to give the C2-azidation product (Scheme 1). This approach would possibly establish a new route for the C2-functionalization of heterocyclic N-oxides, which is currently dominated by the transition metals, especially Pd-catalyzed C2-functionalizations of heterocyclic N-oxides. These reactions normally need prolonged heating and may cause safety concerns regarding thermal stability as well as the possibility of exothermic degration.7
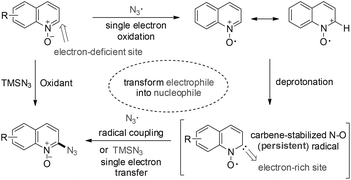 |
| Scheme 1 Initial proposal for the C2-azidation of quinoline N-oxides. | |
Aryl azides have found numerous applications in the synthesis of nitrogen-containing motifs in drug discovery, photoaffinity probes in structural proteomics, and cross-linkers for advanced functional materials.8 Beyond the conventional methods for constructing aryl azides that start from functional aryl compounds,9 two elegant advances in direct C(sp2)–H bond azidations were successively accomplished by Jiao and Li with their coworkers through the development of Cu- and Rh-catalyzed direct C–N bond formations using amine and pyridine as the site-selectivity directing groups, respectively (Scheme 2, a).10 In addition, the electrophilic azidations11a of electron-rich arenes through a hypervalent iodine species have been reported, with and without a metal catalyst (Scheme 2, b).11b–d Although radical oxidative coupling provides a powerful strategy for heterocyclic C–H bond functionalization,12 the selective coupling of heterocycles at an electron-deficient site with an electrophilic azide radical is still a seemingly impossible challenge. Inspired by the C2 site polarity reversal during the formation of carbene-stabilized radicals (Scheme 1), herein, we wish to develop a novel and selective radical oxidative coupling strategy to realize C2-azidation of heterocycles using N-oxide as a non-chelating and removable directing group.
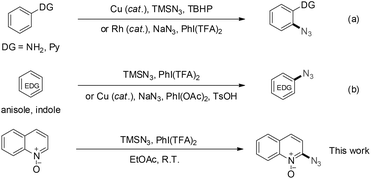 |
| Scheme 2 Azidation of aryl C–H bonds via different routes. | |
Results and discussion
With this assumption in mind, the reaction of quinoline N-oxide 1a with the azide radical,13 generated in situ by a hypervalent iodine reagent,14 was selected as the model azidation to screen the optimized reaction conditions (Table S1 in the ESI†). Gratifyingly, the desired 2-azidoquinoline N-oxide 2a was isolated in 43% yield in acetonitrile using a combination of NaN3 and iodobenzene bis(trifluoroacetate) (PIFA, entry 1). The yield of 2a was improved to 71% when using TMSN3 instead of NaN3 (entry 2). The less reactive iodobenzene diacetate (PIDA) only afforded 2a in 34% yield (entry 3). Among the used solvents, ethyl acetate and dichloromethane gave the best (86%) yield of 2a (entries 2 and 4–7). By altering the ratio of TMSN3 and PIFA, the yield was raised to 90% (entries 8 and 9). The oxidant (PIFA or PIDA) was found to be indispensable for the present azidation (entry 10). When 1,1-diphenylethylene was used as a substrate instead of 1a in the present azidation system, benzophenone generated from a peroxide radical intermediate was separated in 43% yield (Scheme S1 in the ESI†).15 In addition, mixing TMSN3 with PIFA in ethyl acetate produced a large amount of N2 gas.16 These control experiments appear to indicate that an azide radical was involved in the transformation. Surprisingly, the azidation reaction could reach completion in just ten minutes (entry 11). In order to prove the practicality of this approach, a gram-scale synthesis of 2a (1.49 g, 80% yield) was successfully performed (Scheme S2 in the ESI†). Furthermore, 2a could be synthesized from quinoline in one pot in 70% yield (Scheme S3 in the ESI†).
To determine the reaction mechanism, we aimed to observe some intermediates by electron paramagnetic resonance (EPR). Only one signal was clearly observed in the mixture of 1a, TMSN3, and PIFA in ethyl acetate, and these three Lorentzian lines (g = 2.0064, a(14N) = 12.73 G) display a resonance characteristic of a N–O radical (Fig. 1a).17 The N–O radical could be a quinolin-2-one N–O radical that was generated from a carbene-stabilized N–O radical.18 The carbene has been reported to be highly efficient for stabilizing the neighboring radical through delocalization of its spin density; in this way, the interaction between the N–O radical and carbene also played a C2-directing role, and thus the present C–H azidation exhibited excellent regioselectivity. However, this N–O radical signal was not observed for the mixture of 1a and PIFA in ethyl acetate; in addition, no signal was detected in either the combination of 1a and TMSN3 or the mixture of TMSN3 and PIFA, indicating that the azide radical rather than PIFA can oxidate quinoline N-oxide to form the carbene-stabilized N–O radical.
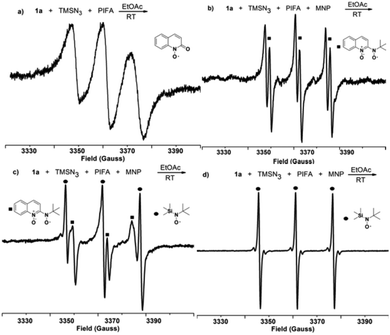 |
| Fig. 1 EPR studies of the interaction of 1a, TMSN3, PIFA, and MNP in EtOAc at room temperature. | |
Considering that the spin-trapping technique would afford more radical information, we then studied the present reaction using 2-methyl-2-nitrosopropane (MNP) as a spin trapping reagent (Fig. 1). Initially, a new N–O radical signal (g = 2.0062, a(14N) = 13.37 G, a(1H) = 1.94 G),19 which might be the adduct of the carbene-stabilized N–O radical with MNP, appeared in the mixture of 1a, TMSN3, PIFA, and MNP (Fig. 1b).20 Several minutes later, this signal gradually died away and another signal (g = 2.0061, a(14N) = 15.52 G, a(33Si) = 4.36 G), generated from the coupling of the trimethylsilyl radical with MNP, appeared (Fig. 1c and d). It is worth noting that no signal was observed in the mixture of TMSN3, PIFA and MNP. These two differences might imply that the trimethylsilyl radical was generated from the carbene-stabilized N–O radical and TMSN3, not from the TMSN3 and PIFA. Therefore, the direct coupling of the carbene-stabilized N–O radical with the azide radical could be excluded.
Based on the above investigations and reported knowledge, a putative mechanism is drawn as shown in Path A of Scheme 3. First, a double ligand exchange between PIFA and TMSN3 would afford PhI(N3)2, which undergoes thermal homolytic cleavage to generate two azide radicals.13 With the help of the azide radical, the quinoline N-oxide would undergo single electron oxidation to generate the radical cation A or A′, which could transform into the carbene-stabilized N–O radical Bvia deprotonation. Eventually, the single electron transfer (SET) from the radical B to TMSN3 gives the product and the trimethylsilyl radical, which could be trapped by MNP. Alternatively, another possibility is Path B, which proceeds via the electrophilic azide radical attacking the quinoline N-oxide to produce the N–O radical C or C′, followed by a further single electron oxidation to afford the desired product.21 Based on the C2-regioselectivity issue and the EPR studies, we were inclined towards Path A.
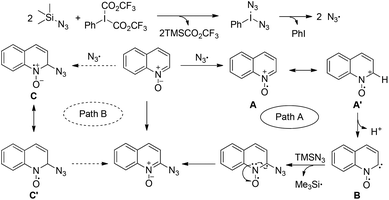 |
| Scheme 3 Plausible reaction mechanism. | |
After attaining a deep understanding of this novel azidation strategy, we turned to examining its application (Scheme 4). The quinoline N-oxide derivatives with electron-donating groups (OMe, Me, Ph, and OCOPh) and withdrawing groups (Br, Cl, COOMe, and CCPh) all smoothly underwent this oxidative azidation, only at the C2-position and within 10 min. The substituted groups at the 4, 5, 6, 7 and 8 positions afforded the corresponding products in 40% to 89% yields (2b–2p). Notably, the present azidation of 3-substituted quinoline N-oxides still exhibited good to excellent yields (2q–2t), possibly because the steric hindrance could stabilize the carbene neighboring N–O radical intermediate, wherein the quinoline N-oxide with a more effectively delocalizing Ph even gave a quantitative yield of 2q.22 The product structure was unambiguously confirmed by the X-ray diffraction (XRD) analysis of a representative product, 2s (CCDC 1031243). In addition, phenanthridine N-oxide was also found to be suitable for this transformation (2u). No azidation occurred for the 2-substituted (2-Me, 2-Cl) quinoline N-oxides, further proving the high C2-regioselectivity of this strategy. Besides, isoquinoline N-oxide and pyridine N-oxide didn't work under the present conditions, indicating the importance of radical stabilization from the π-neighboring Ph group besides that from carbene on the other side.3
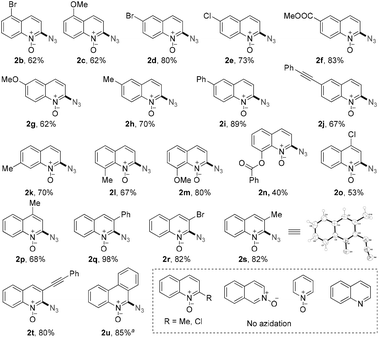 |
| Scheme 4 Scope of the quinoline N-oxides. Reaction conditions: 1 (0.3 mmol), TMSN3 (0.75 mmol), PIFA (0.45 mmol) in EtOAc at R.T. for 10 min. a PIFA (0.3 mmol) was used in EtOAc at −20 °C to R.T. for 30 min. | |
Furthermore, the 2-azidoquinoline N-oxides could be further transformed into a wide variety of functional heterocycles through the azide group (Scheme 5). For instance, 2a could be hydrogenated to 2-aminequinoline 3a in 78% yield using Pd2@NAC-80023 catalyst under 1 atm H2 at R.T., and 3a was subsequently converted into another interesting compound 4 in 65% yield.24 In addition, 2a could be selectively reduced using PCl3 to give the corresponding 2-azidoquinoline 3b, which could be readily transformed into tetrazolo[1,5-a]quinoline.25 According to previous reports, 2-aminoquinoline N-oxide and 2-cyano-3-oxo-3H-indole 1-oxide can also be facilely obtained from 2a.26
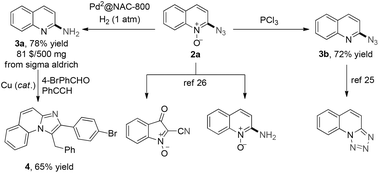 |
| Scheme 5 The transformation of 2-azidoquinoline N-oxide. | |
Conclusions
In summary, we have developed a novel and efficient azidation approach via a polarity reversal strategy, involving the transformation of quinoline N-oxide with an electron-deficient C2 site into a nucleophilic carbene-stabilized N–O radical, and a SET process from the carbene site to TMSN3 that occurred to form the C–N bond with release of a trimethylsilyl radical, as witnessed by EPR trapping. This is the first example that reports the generation of a carbene-stabilized radical using the N–O group as a non-chelating and removable directing group. Compared with reported azidations, the present metal-free procedure is convenient and regioselective under mild conditions, and could be scaled up to gram-synthesis. It is believed that the success of this azidation strategy will initiate other oxidative radical couplings of heterocyclic C–H bonds via carbene-stabilized N–O radicals.
Acknowledgements
This work was supported by the Chinese Academy of Sciences, the National Natural Science Foundation of China (21133011 and 21373246). We also thank Prof. Longmin Wu of Lanzhou University for his generous help in EPR experiments.
Notes and references
- For recent reviews on radical oxidative cross-couplings, see:
(a) C. Liu, D. Liu and A. Lei, Acc. Chem. Res., 2014, 47, 3459 CrossRef CAS PubMed;
(b) A. Studer and D. P. Curran, Nat. Chem., 2014, 6, 765 CrossRef CAS PubMed.
- For selected reviews on the persistent radical effect, see:
(a) A. Studer, Chem. Soc. Rev., 2004, 33, 267 RSC;
(b) H. Fischer, Chem. Rev., 2001, 101, 3581 CrossRef CAS PubMed;
(c) A. Studer, Chem. – Eur. J., 2001, 7, 1159 CrossRef CAS.
- For selected examples of carbene-stabilized radicals, see:
(a) C. D. Martin, M. Soleilhavoup and G. Bertrand, Chem. Sci., 2013, 4, 3020 RSC;
(b) O. Back, B. Donnadieu, P. Parameswaran, G. Frenking and G. Bertrand, Nat. Chem., 2010, 2, 369 CrossRef CAS PubMed.
- For selected reviews on stable radical chemistry, see:
(a) R. G. Hicks, Org. Biomol. Chem., 2007, 5, 1321 RSC;
(b) P. P. Power, Chem. Rev., 2003, 103, 789 CrossRef CAS PubMed.
- For selected reviews on N–O radicals, see:
(a) E. G. Bagryanskaya and S. R. A. Marque, Chem. Rev., 2014, 114, 5011 CrossRef CAS PubMed;
(b) V. Sciannamea, R. Jerome and C. Detrembleur, Chem. Rev., 2008, 108, 1104 CrossRef CAS PubMed;
(c) C. J. Hawker, A. W. Bosman and E. Harth, Chem. Rev., 2001, 101, 3661 CrossRef CAS PubMed.
- For selected examples of quinoline N–O radicals, see:
(a) M. Colonna, L. Greci and M. Poloni, J. Heterocycl. Chem., 1980, 17, 293 CrossRef CAS PubMed;
(b) C. Berti, M. Colonna and L. Greci, Tetrahedron, 1976, 32, 2147 CrossRef CAS.
- For a recent review on functionalization of heterocyclic N-oxides via C–H activation, see: G. Yan, A. J. Borah and M. Yang, Adv. Synth. Catal., 2014, 356, 2375 CrossRef CAS PubMed.
-
(a) W. Song, S. I. Kozhushkov and L. Ackermann, Angew. Chem., Int. Ed., 2013, 52, 6576 CrossRef CAS PubMed;
(b) S. Chiba, Synlett, 2012, 21 CrossRef CAS;
(c)
S. Brase and K. Banert, Organic Azides, Wiley-VCH, Weinheim, 2010 Search PubMed;
(d) M. Minozzi, D. Nanni and P. Spagnolo, Chem. – Eur. J., 2009, 15, 7830 CrossRef CAS PubMed;
(e) S. Brase, C. Gil, K. Knepper and V. Zimmermann, Angew. Chem., Int. Ed., 2005, 44, 5188 CrossRef CAS PubMed;
(f) E. F. V. Scriven and K. Turnbull, Chem. Rev., 1988, 88, 297 CrossRef CAS;
(g) D. M. Huryn and M. Okabe, Chem. Rev., 1992, 92, 1745 CrossRef CAS.
-
(a) L. Niu, H. Yang, D. Yang and H. Fu, Adv. Synth. Catal., 2012, 354, 2211 Search PubMed;
(b) Y. Li, L.-X. Gao and F.-S. Han, Chem. – Eur. J., 2010, 16, 7969 Search PubMed;
(c) C. Tao, X. Cui, J. Li, A. Liu, L. Liu and Q. Guo, Tetrahedron Lett., 2007, 48, 3525 Search PubMed;
(d) W. Zhu and D. Ma, Chem. Commun., 2004, 888 Search PubMed;
(e) C. D. Ritchie and D. J. Wright, J. Am. Chem. Soc., 1971, 93, 2429 Search PubMed;
(f) P. A. S. Smith, C. D. Rowe and L. B. Bruner, J. Org. Chem., 1969, 34, 3430 Search PubMed;
(g) J. C. Kauer and R. A. Carboni, J. Am. Chem. Soc., 1967, 89, 2633 Search PubMed.
-
(a) Y. Fan, W. Wan, G. Ma, W. Gao, H. Jiang, S. Zhu and J. Hao, Chem. Commun., 2014, 50, 5733 Search PubMed;
(b) F. Xie, Z. Qi and X. Li, Angew. Chem., Int. Ed., 2013, 52, 11862 Search PubMed;
(c) C. Tang and N. Jiao, J. Am. Chem. Soc., 2012, 134, 18924 Search PubMed.
-
(a) D. Lubriks, I. Sokolovs and E. Suna, J. Am. Chem. Soc., 2012, 134, 15436 Search PubMed;
(b) M. S. Workentin, B. D. Wagner, J. Lusztyk and D. D. M. Wayner, J. Am. Chem. Soc., 1995, 117, 119 Search PubMed;
(c) Y. Kita, H. Tohma, K. Hatanaka, T. Takada, S. Fujita, S. Mitoh, H. Sakurai and S. Oka, J. Am. Chem. Soc., 1994, 116, 3684 Search PubMed;
(d) Y. Kita, H. Tohma, M. Inagaki, K. Hatanaka and T. Yakura, Tetrahedron Lett., 1991, 32, 4321 Search PubMed.
- For selected examples of innate C–H bond functionalization of heterocycles via radical coupling strategy, see:
(a) A. G. O'Brien, A. Maruyama, Y. Inokuma, M. Fujita, P. S. Baran and D. G. Blackmond, Angew. Chem., Int. Ed., 2014, 53, 11868 Search PubMed;
(b) K. Foo, E. Sella, I. Thome, M. D. Eastgate and P. S. Baran, J. Am. Chem. Soc., 2014, 136, 5279 Search PubMed;
(c) T. Bruckl, R. D. Baxter, Y. Ishihara and P. S. Baran, Acc. Chem. Res., 2012, 45, 826 Search PubMed;
(d) Y. Fujiwara, J. A. Dixon, F. O'Hara, E. D. Funder, D. D. Dixon, R. A. Rodriguz, R. D. Baxter, B. Herle, N. Sach, M. R. Collins, Y. Ishihara and P. S. Baran, Nature, 2012, 492, 95 Search PubMed;
(e) Y. Ji, T. Brueckl, R. D. Baxter, Y. Fujiwara, I. B. Seiple, S. Su, D. G. Blackmond and P. S. Baran, Proc. Natl. Acad. Sci. U. S. A., 2011, 108, 14411 Search PubMed;
(f) I. B. Seiple, S. Su, R. A. Rodriguez, R. Gianatassio, Y. Fujiwara, A. L. Sobel and P. S. Baran, J. Am. Chem. Soc., 2010, 132, 13194 Search PubMed.
- For selected examples of the generation of azide radicals using PIFA and TMSN3 or NaN3, see:
(a) R. Narayan and A. P. Antonchick, Chem. – Eur. J., 2014, 20, 4568 Search PubMed;
(b) K. Matcha, R. Narayan and A. P. Antonchick, Angew. Chem., Int. Ed., 2013, 52, 7985 Search PubMed;
(c) K. Matcha and A. P. Antonchick, Angew. Chem., Int. Ed., 2013, 52, 2082 Search PubMed;
(d) A. P. Antonchick and L. Burgmann, Angew. Chem., Int. Ed., 2013, 52, 3267 Search PubMed.
- For selected reviews on hypervalent iodine, see:
(a) M. S. Yusubov and V. V. Zhdankin, Curr. Org. Synth., 2012, 9, 247 Search PubMed;
(b) E. A. Merritt and B. Olofsson, Synthesis, 2011, 517 Search PubMed;
(c) J. P. Brand, D. F. Gonzalez, S. Nicolai and J. Waser, Chem. Commun., 2011, 47, 102 Search PubMed;
(d) T. Dohi, M. Ito, N. Yamaoka, K. Morimoto, H. Fujioka and Y. Kita, Tetrahedron, 2009, 65, 10797 Search PubMed;
(e) M. Uyanik and K. Ishihara, Chem. Commun., 2009, 2086 Search PubMed;
(f) V. V. Zhdankin and P. J. Stang, Chem. Rev., 2008, 108, 5299 Search PubMed;
(g) R. M. Moriarty and O. Prakash, Acc. Chem. Res., 1986, 19, 244 Search PubMed.
- For a recent example of C
C double-bond cleavage using an azide radical, see: T. Wang and N. Jiao, J. Am. Chem. Soc., 2013, 135, 11692 Search PubMed . For the detailed process, see Scheme S1† in the ESI.†.
- For selected examples of the generation of nitrogen from azide radicals, see:
(a) P. Magnus and J. Lacour, J. Am. Chem. Soc., 1992, 114, 767 Search PubMed;
(b) W. S. Trahanovsky and M. D. Robbins, J. Am. Chem. Soc., 1971, 93, 5256 Search PubMed.
- The broad spectral line unresolved hyperfine splitting is attributed to other protons on the aromatic ring.
- For selected reviews on the generation of carbonyl oxides from carbenes, see:
(a) W. H. Bunnelle, Chem. Rev., 1991, 102, 335 Search PubMed;
(b) W. Sander, Angew. Chem., Int. Ed. Engl., 1990, 29, 344 Search PubMed . A possible pathway from a carbene-stabilized N–O radical to a quinolin-2-one N–O radical is:
.
- Owing to the C3–H of quinoline N-oxide, the N–O radical displayed six Lorentzian lines.
- For recent examples of Cu-catalyzed C2-acetoxylation and Pd-catalyzed C2-alkylation of quinoline N-oxide via a radical process, see:
(a) X. Chen, C. Zhu, X. Cui and Y. Wu, Chem. Commun., 2013, 49, 6900 Search PubMed;
(b) Z. Wu, C. Pi, X. Cui, J. Bai and Y. Wu, Adv. Synth. Catal., 2013, 355, 1971 Search PubMed.
- The electrophilic azide radicals may easily attack the 3-position (relatively electron-rich) of quinoline N-oxides; see: J. Zhao, P. Li, C. Xia and F. Li, RSC Adv., 2015, 5, 32835 Search PubMed.
- The steric hindrance is able to stabilize the carbene, which could further steady the N–O radical.
- For the synthesis of Pd2@NAC-800, see: Z. Li, J. Li, J. Liu, Z. Zhao, C. Xia and F. Li, ChemCatChem, 2014, 6, 1333 Search PubMed.
- N. Chernyak and V. Gevorgyan, Angew. Chem., Int. Ed., 2010, 49, 2743 Search PubMed.
- C. Wentrup and H. W. Winter, J. Am. Chem. Soc., 1980, 102, 6159 Search PubMed.
- R. A. Abramovitch and B. W. Cue Jr., J. Org. Chem., 1980, 45, 5316 Search PubMed.
Footnote |
† Electronic supplementary information (ESI) available. CCDC 1031243. For ESI and crystallographic data in CIF or other electronic format see DOI: 10.1039/c5qo00204d |
|
This journal is © the Partner Organisations 2015 |
Click here to see how this site uses Cookies. View our privacy policy here.