DOI:
10.1039/C4RA13352H
(Paper)
RSC Adv., 2015,
5, 12613-12623
Fractionation, physicochemical properties, nutritional value, antioxidant activity and ACE inhibition of palm kernel expeller protein
Received
29th October 2014
, Accepted 12th January 2015
First published on 12th January 2015
Abstract
Five protein fractions (albumin, globulin, prolamines, glutelin-1 and glutelin-2) from palm kernel expeller were fractionated and characterized. Their nutritional value, physicochemical properties, antioxidant activity and angiotensin I-converting enzyme (ACE) inhibition were also investigated. Results showed that the globulin and glutelin-2 were the predominant fractions. The albumin and globulin were rich in arginine, and the glutelin-2 had a high content of sulfur amino acids and showed good nutritional quality. In both the native and reduced states, albumin and glutelin-1 consisted of three polypeptides (18.8, 22.1 and 51.5 kDa) and five peptides (20.7 to 51.5 kDa), respectively. The globulin and glutelin-2 were composed of polypeptides linked via one or more disulfide bonds. Albumin, prolamines and the total palm kernel expeller protein (TPKP) had endothermic peaks at 53.8, 126.5 and 102.3 °C respectively. All the fractions except globulin exhibited a good emulsifying capacity (137.87–269.54 m2 g−1) and emulsion stability (75.13–89.71%) but poor foaming capacity (≤47.56 g/100 g). Among the fractions, prolamines showed the highest inhibiting ability of lipid autoxidation, ACE inhibition (13.63 ± 2.18%) and free radical-scavenging activity on DPPH (79.45 ± 6.80%), hydroxyl (93.40 ± 1.46%) and ABTS (79.35 ± 1.99%). Overall, these palm kernel expeller protein fractions could be used in the food or other industries.
1. Introduction
Oil palm (Elaeis guineensis Jacq) is an important cash crop in the tropics. Palm kernel expeller (PKE), a byproduct of the palm milling industry, is rich in carbohydrate and protein but is just used as a fertilizer or ruminant, poultry and swine feed ingredient.1 Palm kernel expeller protein (PKP) is a good potential edible protein for its abundance of essential amino acids and relatively well-balanced amino acid profile.1,2 However, PKP is not used extensively for human consumption in the form of processed food, which may be due to its lower digestibility and poor foaming properties.2 Moreover, the lack of a commercially feasible extraction method and a systematic study on its physicochemical properties and bioactivity also limits its application in the food industry.
The relative proportion of each solubility fraction in a seed protein affects the nutritional quality and the functional properties of the total protein.3 Such as the 7S globulin-rich soybean proteins had better emulsifying properties and the glycinin played an important role in the gel property of soybean proteins.4 Some protein fractions (such as the soybean globulin, egg albumin, zein, etc.) are widely used in food industry for their special physicochemical properties. The beneficial effects of some protein fractions (like ribonuclease A-albumin, immunoglobulin, zein, and so on) for human health have been widely reported, too.5 Thus, the fractionation and characterization of an unconventional protein is very important for its application in food and other industries. Though the 2S and 7S fractions of oil palm embryos had been extracted and classified,6 there is no information about fractionation and characterization of PKP.
The successful acceptance and adaptation of an unconventional protein by the food industry and consumers depend on its nutritional value, safety criteria, physicochemical and functional properties.7 Recently, it has been recognized that apart from their basic nutritional role, some dietary proteins exhibit different activities (e.g., antimicrobial, antioxidant, antithrombotic, antihypertensive, immunomodulatory, etc.) and exert beneficial effects upon human health.8 Many proteins and related peptides like wheat protein,9 carp muscle protein10 and silver carp protein11 have been demonstrated to possess antioxidant activity and ACE inhibition. Though the solubility and emulsifying capacity of PKP extracted with trypsin was studied,2 there is no available data on the nutritional value, functional properties and bioactivity of palm kernel protein fractions. This also limits the usage of PKP in food industry.
Over the years, protein fractions (albumin, globulin, prolamines, etc.) had been successfully separated from materials such as soybean, grain and coconut by the sequential extraction method and widely used in food and other industries.4,8,12,13 In Malaysia alone, nearly 2.31 million tons of PKE is produced per year,14 which means that a large amount of protein fractions could be produced from it. If these protein fractions have good nutritional quality, functional properties or some beneficial effects for human health, the usage of PKP will be expanded and remarkable economic benefits will be achieved.
Therefore, the objects of this paper are: (1) to study the fractionation and purification of palm kernel albumin, globulin, prolamines, glutelin-1 and glutelin-2; (2) to investigate their nutritional value, physicochemical properties, antioxidant activity and ACE inhibitory activity for better understanding their potential application in various food systems.
2. Materials and methods
2.1 Materials
Palm kernel expeller (PKE) powder was obtained from the Coconut Grand View Garden, Wenchang, Hainan province, China. Low molecular weight protein markers were purchased from Shanghai DINGUO Biotech. Co., Ltd. (China). Sephadex G-100 was purchased from Pharmacia (Uppsala, Sweden). Angiotensin I-converting enzyme from rabbit lung and N-Hippuryl-His-Leu hydrate (HHL) were purchased from Sigma Co., China. Other chemicals and reagents were of analytical grade.
2.2 Protein preparation and fractionation
Five PKP fractions (albumin, globulin, prolamines, glutelin-1 and glutelin-2) were separated and purified according to the sequential extraction method.12 Different solvents, including distilled water, 0.4 M NaCl, 70% (v/v) 2-propanol (IPA), 50% (v/v) glacial acetic acid and 0.1 M NaOH, were in sequence to extract the five fractions. The protocol of this sequential extraction was shown in Fig. 1. Each fraction was extracted by simply stirring, with a meal to solvent ratio of 1
:
10 (g mL−1), at 4 °C for 8 h. Extraction with each solvent was in triplicates, and all supernatants for each solvent were pooled to obtain a representative of each solubility fraction. Each fraction then was dialyzed against deionized water. Correspondingly, water-, NaCl-, IPA-, acetic acid-, and NaOH-soluble proteins were designated as albumin, globulin, prolamines, glutelin-1 and glutelin-2, respectively.
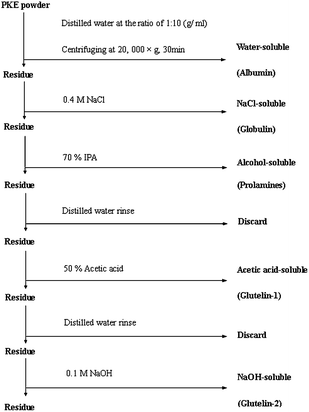 |
| Fig. 1 Flow sheet of the protocol used for fractionation of palm kernel expeller protein by different solubility. | |
The total palm kernel expeller protein (TPKP) was extracted by the modified method of Angelia et al.13 Ten grams of PKE were suspended in 100 mL of phosphate buffer (0.1 M, pH 9.0, with 0.4 M NaCl). After being stirred at 4 °C for 1 h, the mixture was filtered and centrifuged at 10
000×g for 30 min. The supernatant was collected and precipitated by adjusting to pH 4.5 with 0.1 M HCl, and then centrifuged again. The precipitate was collected, dialyzed against distilled water at 4 °C for 48 h and lyophilized in a dryer (LABCONCO 2.5, USA). Then TPKP was obtained and stored at −20 °C.
2.3 Nutritional value and physicochemical properties
2.3.1 Amino acid analysis. Protein content of each soluble fraction was determined by the Bradford method,15 and protein content (N × 6.25) of PKE was determined by the micro-Kjeldahl method.16 Amino acid compositions of each sample were determined according to the method of Wang et al.17 Amino acid composition was expressed as g/100 g protein, and the data were used for calculating Bigelow parameters, including NPS (the frequency of nonpolar side chains) and P (ratio of polar to nonpolar side chains). Where, NPS was calculated by counting the Trp, Ile, Tyr, Phe, Pro, Leu and Val residues and expressing the sum as a fraction of the total number of residues.18
2.3.2 Evaluation of nutritional parameters. The in vitro protein digestibility (IVPD) was evaluated with the method of Saunders et al.19 The digestible protein was analyzed with the Bradford method13 and expressed as a percent of the total protein (g/100 g). The amino acid compositions of samples were also used to calculate their nutritional parameters, including the proportion of essential amino acids to the total amino acids (E/T) and biological value (BV). |
E/T, g/100 g = [(Val + Thr + Ile + Leu + Lys + Phe + Trp + Tyr + Cys + Met) × 100]/(Ala + Asp + Arg + Gly + Glu + Val + Thr + Ile + Leu + Lys + Cys + Phe + His + Trp + Tyr + Met + Pro + Ser)
| (1) |
BV was calculated using the method of Morrup and Olesen20 as follows:
|
BV = 102.15 × qLys0.41 × qPhe+Tyr0.60 × qMet+Cys0.77 × qThr2.4 × qTrp0.21
| (2) |
where,
qi = (a
i sample)/(a
i reference), if a
i sample ≤ a
i reference,
qi = (a
i reference)/ (a
i sample), if a
i sample ≥ a
i reference, a
i = mg amino acid per g of total essential amino acid.
Essential amino acid (g amino acid/100 g protein) pattern of the FAO/WHO (Food and Agriculture Organization/World Health Organization) standard protein is Ile = 4.00, Leu = 7.04, Lys = 5.44, Met + Cys = 3.52, Phe + Tyr = 6.08, Thr = 4.00, Trp = 0.96 and Val = 4.96.21
2.3.3 Sodium dodecyl sulfate polyacrylamide gel electrophoresis (SDS-PAGE). Electrophoresis was done according to the method described by Laemmli.22 SDS-PAGE was performed on 5% stacking gel and 12% separating gel using gel electrophoreses apparatus DYCZ-30 (Beijing LIUYI Instrument Factory, China). The samples were directly dissolved in the sample buffer, i.e., 0.125 M Tris–HCl buffer, pH 6.8, containing 1% (w/v) SDS, 0.05% (v/v) bromophenol blue and 30% (v/v) glycerol. The electrophoresis was run at 100 V in stacking gel and 120 V in separating gel until the tracking dye reached the bottom of the gel. The gels were stained with Coomassie Brilliant Blue R-250 (1 mg mL−1) for 3 h and destained in a 10% (v/v) methanol and 10% (v/v) acetic acid solution. To analyze the reduced proteins, β-mercaptoethanol (ME) was added to the sample buffer to a final concentration of 5% (v/v). The protein electrophoretic patterns were analyzed by a gel imaging system (Synene BOXF3, UK). Molecular weight of the protein bands were estimated using the following protein makers:
phosphorylase b (97.4 kDa), BSA (66.2 kDa), ovalbumin (43.0 kDa), carbonic anhydrase (31.0 kDa), trypsin inhibitor (20.1 kDa) and lysozyme (14.4 kDa).
2.3.4 Gel filtration. All the PKP fractions except prolamines (for its little amount) were further fractionated by gel filtration chromatography with Sephadex G-100 (sigma, USA) column. After being swelled in the extraction solution for 48 h, the gel was degassed and packed in a column (1.6 × 60 cm) and then equilibrated with extraction solution for 48 h. The bed volume (Vt) of the column was 125 mL, and the void volume (Vo) was 23.5 mL, which were determined with blue dextran 2000. Each fraction (10 to 20 mg) was dissolved in its own extraction solvent and loaded onto the Sephadex G-100 column which had been equilibrated with the same solvent. Then the fraction was eluted with the extraction solvent at a flow rate of 0.6 mL min−1. Fractions of 3.0 mL were collected and the absorbance was monitored at 280 nm. The column was calibrated with aldolase (158.0 kDa), albumin (67.0 kDa), ovalbumin (43.0 kDa), chymotrypsinogen A (25.0 kDa) and lysozyme (14.4 kDa).
2.3.5 Differential scanning calorimetry (DSC). The thermal behavior of samples was analyzed using a differential scanning calorimeter (STAR SW-11, METTLER Co., China). Each sample (0.7 to 1.1 mg) was directly weighed onto the aluminum pan, and a sealed empty sample pan was used as the reference. The heating rate was 5 °C min−1 over the range of 30–130 °C.23
2.3.6 Surface hydrophobicity. A range of protein concentrations (0.01–0.6 mg mL−1) were obtained by serially dilution with phosphate buffer (0.01 M, pH 8.0). For 2 mL of each sample, a 20 μL of 1-anilino-8-naphthalene-sulphonate (ANS, 8 mM) was added. The fluorescence intensity (FI) was measured with a spectrofluorophotometer (970CRT) at an excitation wavelength of 390 nm and an emission wavelength of 470 nm, with a slit width of 5 nm. The FI values were plotted against protein concentrations and the initial slope of the regression curve was designated as S0.23
2.3.7 Emulsifying properties. The emulsifying activity index (EAI) and emulsion stability index (ESI) of albumin, globulin, glutelin-1 and glutelin-2 at different ionic strengths adjusted by NaCl (ionic strengths 0.1, 0.2, 0.4 and 0.6 M) were determined by the method of Soares et al.24 with a slight modification. Aliquot (3 mL) of sample solutions (1 g mL−1) was mixed with 1 mL of soybean oil, and then homogenized at a speed of 10
000 rpm for 90 s. A 100 μL reaction mixture was immediately pipetted from the bottom of the tubes and diluted with 4.9 mL of SDS solution (1 mg mL−1). The absorbance of the diluted emulsion was measured after emulsion formation (Ao) and 10 min emulsion formation (At) at 500 nm. EAI (m2 g−1) and ESI (%) were calculated by the following equation: |
EAI = (2.303 × 2 × A0 × dilution factor)/(C × Φ × 10 000)
| (3) |
where, C = 0.001 g protein per mL; Φ = oil volume fraction for emulsion (0.25); dilution factor = 50 (100 μL emulsion diluted with 5 mL 0.1 g/100 mL SDS solution).where, At was the absorbance at 500 nm, 10 min after emulsion formation.
2.3.8 Foaming properties. The foaming capacity (FC) and foam stability (FS) at different ionic strengths adjusted by NaCl (ionic strengths 0.1, 0.2, 0.4 and 0.6 M) were measured by the method of Shahidi et al.7 Aliquots (30 mL) of samples suspension (0.5 g L−1) were homogenized for 1 min with a DX-8 homogenizer (Nihonseiki Kaisha Ltd., Tokyo, Japan) at 10
000 rpm. The whipped suspension was immediately transferred into a cylinder, and the volume of the foam was recorded as V0, the volume of foam after 30 min was recorded as Vt. FC and FS were expressed by the following equations: |
Foaming capacity = V0/30 mL × 100%
| (5) |
|
Foam stability = Vt/V0 × 100%
| (6) |
2.3.9 Viscosity. The viscosity of samples at different ionic strengths adjusted by NaCl (ionic strengths 0.1, 0.2, 0.4 and 0.6 M) and different protein concentration (0.02, 0.04, 0.06, 0.08 and 0.1 g mL−1) were determined by the method of Tsumura et al.,4 using a viscometer (RVA-Tecmaster Co., China).
2.4 Determination of antioxidant activity and ACE inhibition
2.4.1 Superoxide radical-scavenging activity. Aliquot of protein fractions (100 μg mL−1, dissolved in 0.1 M Tris–HCl–EDTA buffer with pH 8.0) and 3 mL of pyrogallol solution (3 mM) were added into a tube, the absorbance at 320 nm were recorded at 30 s intervals for up to 10 min using a spectrophotometer. The antioxidant activity was determined as the percentage of inhibiting pyrogallol autoxidation, which was calculated from the absorbance at 320 nm in the presence or absence of samples.25 BHT (butylated hydroxytoluene, 100 μg mL−1) was used as the comparison.
2.4.2 DPPH radical-scavenging activity. The scavenging activity of DPPH (1,1-diphenyl-2-picrylhydrazyl) radical was tested according to the method of Zhang et al.26 Two milliliters of sample solution (100 μg mL−1) and 2 mL of 0.1 mM DPPH were reacted in the dark for 30 min at room temperature. The absorbance at 517 nm was read. BHT (100 μg mL−1) was used as the comparison. The scavenging activity was expressed as follows: |
 | (7) |
where AB was the absorbance of the blank (distilled water instead of protein samples), AC was the absorbance of control (distilled water instead of DPPH solution), and AS was the absorbance of mixture contained protein samples.
2.4.3 ABTS radical scavenging activity. Two millimeters of ABTS (2,2′-azino-bis (3-ethylbenzothiazoline-6-sulphonic acid)) radical solution and 20 μL of the sample solutions (100 μg mL−1) were reacted at 30 °C for 6 min. Then the absorbance at 734 nm was recorded.27 BHT (100 μg mL−1) was used as the comparison. The activity was calculated as follows: |
 | (8) |
where AB was the absorbance of the blank (distilled water instead of protein samples), AC was the absorbance of control (distilled water instead of ABTS solution), and AS was the absorbance of mixture contained protein samples.
2.4.4 Scavenging activity of hydroxyl radical (˙OH). The hydroxyl radical-scavenging activity was assayed using the 2-deoxyribose oxidation method.28 Sample solution (100 μg mL−1), FeSO4–EDTA (10 mM) and 2-deoxyribose (10 mM) were dissolved in phosphate buffer (pH 7.4, 0.1 M) and mixed thoroughly. Then hydrogen peroxide (H2O2, 10 mM) was added into the mixture to start the reaction. After incubated at 37 °C for 1 h, the reaction solution was mixed with trichloroacetic acid (TCA, 2.8 g/100 mL) and thiobarbituric acid (TBA, 1 g/100 mL) and incubated at 100 °C for 20 min. The absorbance was measured at 532 nm. BHT (100 μg mL−1) was used as the comparison. The activity was determined as follows: |
 | (9) |
where AB was the absorbance of the blank (distilled water instead of samples), AC was the absorbance of control (without the addition of 2-deoxyribose oxidation), and AS was the absorbance of mixture contained samples.
2.4.5 Metal chelating activity. According to the method of Kong and Xiong,29 450 μL of sample solution (100 μg mL−1), 45 μL of 2 mM FeCl2 and 1815 μL of distilled water were mixed. The mixture was reacted with 90 μL of 5 mM 3-(2-pyridya)-5,6-bis (4-phenyl-sulfonic acid)-1,2,4-triazine (ferrozine) for 30 min at room temperature. Then the absorbance was read at 562 nm. BHT (100 μg mL−1) was used as the comparison. Chelating activity was calculated as follows: |
 | (10) |
where AB was the absorbance of the blank (distilled water instead of samples), AC was the absorbance of control (without the addition of ferrozine), and AS was the absorbance of mixture contained samples.
2.4.6 Reducing power. Reducing power was determined by the method of Benzie and Strain.30 Aliquot of sample solution (100 μg mL−1) was mixed with 0.2 M phosphate buffer (pH 6.6) and potassium ferricyanide (0.01 g mL−1). The mixture was first incubated at 50 °C for 20 min. An aliquot (2.5 mL) of TCA (0.1 g mL−1) was added to the mixture, followed by centrifugation at 3000 rpm for 10 min. The supernatant (2.5 mL) was mixed with 2.5 mL of distilled water and 2.5 mL of ferric chloride (1 g/100 mL), and the absorbance at 700 nm was read after 10 min. The control was prepared in the same manner but without the sample, the blank was obtained in the absence of ferric chloride. Increased absorbance of the reaction mixture indicated increased reducing power. BHT (100 μg mL−1) was also used as the comparison.
2.4.7 Inhibition of linoleic acid autoxidation. Following the modified method of Wu et al.,31 aliquot of sample solutions (100 μg mL−1, dissolved in 0.1 M phosphate buffer, pH 7.0) was added to 1 mL of linoleic acid (50 mM) in a tube which was sealed tightly and kept at 60 °C in the dark for 8 days. The degree of oxidation was evaluated by measuring the ferric thiocyanate values.32 Then 0.1 mL of the reaction mixture described above was mixed with 4.7 mL of 75% ethanol, 0.1 mL of ammonium thiocyanate (0.3 g mL−1), and 0.1 mL of 0.02 M FeCl2. The absorbance at 500 nm representing the degree of linoleic acid oxidation was recorded after 3 min. The antioxidant activity of propyl gallate (PG, 100 μg mL−1) was assayed for comparison purposes.
2.4.8 ACE inhibition. ACE inhibitory activity was determined using the method of Jimsheena and Gowda33 with a slight modification. Aliquot (50 μL) of PKP fractions (1.0 mg mL−1), 50 μL of ACE (25 mill units per mL) and 150 μL of 8.3 mM HHL (dissolved in 50 mM sodium borate buffer containing 0.5 M NaCl at pH 8.3) were mixed and incubated at 37 °C for 60 min, followed by termination of the reaction by adding 250 μL of 1 M HCl. Then 1.4 mL of ethyl acetate was added, and the mixture was vortexed for 5 s and centrifuged at 14
100×g for 5 min. 1 mL of the upper organic phase was transferred into a test tube, and placed in a vacuum oven at room temperature for 1.5 h. Then 2 mL of distilled water was added into the tube, and the absorbance was determined with a spectrophotometer. The inhibition (%) was calculated as below: |
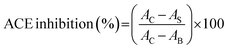 | (11) |
where, AC was the absorbance of the ACE solution without an inhibitor (the fractions), AS was the absorbance of mixture contained samples, and AB was the absorbance of mixture without ACE.
2.5 Statistical analysis
All the results were the means of triplicates. Data were subjected to analysis of variance, and Duncan value with a confidence interval of 95% was calculated to compare means.
3. Results and discussion
3.1 Distribution of protein fractions
The crude protein content of PKE was 24.34 g/100 g (dry matter). The contents of albumin, globulin, prolamines, glutelin-1 and glutelin-2 in the total extractable proteins of PKE were 13.36 ± 0.34, 26.08 ± 0.45, 1.79 ± 0.24, 16.19 ± 0.16 and 33.32 ± 1.56 g/100 g, respectively. It meant that the globulin and glutelin-2 were the predominant fraction in PKE, which was different from that of palm embryos proteins (globulin is the major).6 This may be caused by the difference in protein extraction conditions. After all, fractionation of proteins depending on solubility is only an approximation of the actual protein composition.34 Moreover, it was pointed out that the sequential fractionation may impact results obtain for other fractions, and an alternative way of studying protein fraction was the step-by-step way (i.e., each fraction is extracted separately from a determine amount of untreated sample). However, to get a pure protein fraction and study its physicochemical properties, the sequential fractionation strategy may be the better choice. For it may be more complex to get a pure protein fraction by the second way (e.g., you have to remove albumin and globulin during the extraction of glutelin-2).
3.2 Nutritional quality and physicochemical properties
3.2.1 Amino acid composition and nutritional quality. As shown in Table 1, each palm kernel protein fraction owned individual amino acid composition. The albumin and globulin showed a similar amino acid profile with a great amount of arginine, whereas glutelin-1, glutelin-2 and TPKP were rich in leucine, aspartic acid and glutamic acid. The glutelin-2 owned a high content of methionine and cysteine. Moreover, significant difference (P < 0.05) in the nutritional quality and Bigelow parameters of the fractions was also obtained. Though with higher IVPD value, albumin and globulin showed low nutritive value, resulting from their unbalanced amino acid composition. Compared with the requirement of child as recommended by FAO/WHO,21 the glutelin-2 and TPKP exhibited a relative balanced amino acid composition and high nutritive quality. However, any single fraction was not the optimum choice for human consumption. Though with a high NPS value, glutelin-2 showed the lowest surface hydrophobicity (S0). This meant that NPS of a protein was not always consistent with its S0.
Table 1 Amino acid composition (g/100 g protein), Bigelow parameters and nutritional quality of the five palm kernel protein fractions and the total palm kernel expeller protein (TPKP)a
Amino acid |
Albumin |
Globulin |
Prolamines |
Glutelin-1 |
Glutelin-2 |
TPKP |
FAO/WHO, 1990 |
0–12 months |
2–5 year old |
Different small letters in the same row meant significant difference (P < 0.05). The frequency of nonpolar side chains. Ratio of polar to nonpolar side chains. Ratio of essential amino acid to total amino acids. In vitro digestibility. Not measured. |
Essential amino acid |
Threonine |
2.90 ± 0.17a |
2.2 ± 0.174a |
1.72 ± 0.08a |
6.39 ± 0.29a |
5.99 ± 0.11a |
5.18 ± 0.09a |
4.3 |
3.4 |
Valine |
2.04 ± 0.02a |
2.32 ± 0.25a |
2.84 ± 0.06a |
6.12 ± 0.09a |
5.54 ± 0.21a |
5.93 ± 0.05a |
5.5 |
3.5 |
Lysine |
3.47 ± 0.08a |
4.70 ± 0.31a |
1.45 ± 0.16a |
5.01 ± 0.17a |
4.05 ± 0.14a |
5.23 ± 0.34a |
6.6 |
5.8 |
Methionine |
0.05 ± 0.01a |
0.06 ± 0.01a |
0.15 ± 0.01a |
0.21 ± 0.01a |
2.41 ± 0.26a |
2.98 ± 0.16a |
|
|
Cysteine |
0.05 ± 0.01a |
0.06 ± 0.01a |
0.02 ± 0.00a |
0.05 ± 0.01a |
7.86 ± 0.58a |
0.30 ± 0.02a |
|
|
Met + Cys |
0.10 ± 0.02a |
0.12 ± 0.02a |
0.17 ± 0.02a |
0.26 ± 0.02a |
10.85 ± 0.27a |
3.28 ± 0.19a |
4.2 |
2.5 |
Isoleucine |
1.48 ± 0.14a |
1.50 ± 0.17a |
1.93 ± 0.14a |
5.24 ± 0.24a |
5.13 ± 0.45a |
4.40 ± 0.30a |
4.6 |
2.8 |
Leucine |
2.98 ± 0.02a |
4.00 ± 0.08a |
5.14 ± 0.08a |
10.38 ± 0.38a |
9.85 ± 0.45a |
9.61 ± 0.49a |
9.3 |
6.6 |
Phenylalanine |
0.33 ± 0.01a |
0.35 ± 0.02a |
2.35 ± 0.01a |
3.13 ± 0.16a |
4.45 ± 0.28a |
5.19 ± 0.69a |
|
|
Tyrosine |
2.39 ± 0.12a |
3.12 ± 0.21a |
6.18 ± 0.07a |
5.81 ± 0.21a |
0.08 ± 0.01a |
4.41 ± 0.17a |
|
|
Histidine |
1.41 ± 0.15a |
1.98 ± 0.20a |
1.43 ± 0.15a |
3.29 ± 0.03a |
2.67 ± 0.17a |
4.32 ± 0.20a |
2.6 |
1.9 |
Tryptophan |
0.22 ± 0.00a |
0.21 ± 0.02a |
0.22 ± 0.01a |
0.22 ± 0.02a |
0.93 ± 0.08a |
1.04 ± 0.03a |
1.1 |
0.5 |
TEAA |
14.72 ± 0.25a |
17.22 ± 0.10a |
17.04 ± 0.08a |
39.83 ± 0.34a |
48.65 ± 1.10a |
43.15 ± 1.77a |
33.9 |
12.7 |
![[thin space (1/6-em)]](https://www.rsc.org/images/entities/char_2009.gif) |
Nonessential amino acid |
Arginine |
56.39 ± 2.23a |
59.99 ± 1.18a |
21.31 ± 1.45a |
9.64 ± 0.1a |
7.56 ± 0.2a |
3.70 ± 0.02a |
|
|
Aspartic acid |
1.70 ± 0.07a |
1.32 ± 0.11a |
5.24 ± 0.19a |
7.43 ± 0.18a |
12.85 ± 0.60a |
10.19 ± 0.44a |
|
|
Serine |
3.92 ± 0.02a |
2.76 ± 0.19a |
3.24 ± 0.10a |
9.12 ± 0.04a |
8.29 ± 0.31a |
7.41 ± 0.04a |
|
|
Glutamic acid |
2.26 ± 0.35a |
2.47 ± 0.21a |
7.08 ± 0.05a |
10.61 ± 0.13a |
7.15 ± 0.27a |
14.67 ± 0.82a |
|
|
Glycine |
2.76 ± 0.15a |
2.80 ± 0.24a |
3.77 ± 0.14a |
4.19 ± 0.19a |
4.09 ± 0.15a |
4.48 ± 0.21a |
|
|
Alanine |
3.42 ± 0.17a |
2.89 ± 0.24a |
3.48 ± 0.21a |
4.72 ± 0.14a |
4.05 ± 0.14a |
4.43 ± 0.28a |
|
|
Proline |
2.13 ± 0.07a |
2.41 ± 0.28a |
2.60 ± 0.20a |
5.81 ± 0.17a |
5.49 ± 0.31a |
5.14 ± 0.21a |
|
|
![[thin space (1/6-em)]](https://www.rsc.org/images/entities/char_2009.gif) |
Bigelow parameters |
NPSb |
0.13 ± 0.01a |
0.15 ± 0.01a |
0.30 ± 0.01a |
0.38 ± 0.01a |
0.32 ± 0.01a |
0.36 ± 0.02a |
|
|
Pc |
6.76 ± 0.02a |
5.84 ± 0.20a |
2.30 ± 0.08a |
1.65 ± 0.01a |
2.18 ± 0.13a |
1.76 ± 0.13a |
|
|
Surface hydrophobicity |
50.91 ± 0.32a |
40.61 ± 0.23a |
51.15 ± 0.21a |
52.77 ± 0.66a |
37.29 ± 0.12a |
203.55 ± 3.95a |
|
|
![[thin space (1/6-em)]](https://www.rsc.org/images/entities/char_2009.gif) |
Nutritional quality |
E/Td (%) |
16.41a |
18.11a |
24.30a |
40.88a |
44.49a |
42.77a |
|
|
First limiting amino acid |
Met + Cys |
Met + Cys |
Met + Cys |
Met + Cys |
Lysine |
Lysine |
|
|
IVPDe (g/100g) |
80.56 ± 1.62a |
70.9 5 ± 2.25a |
NDa |
69.15 ± 2.13a |
65.87 ± 2.46a |
68.20 ± 1.89a |
|
|
BV (biological values) |
2.92a |
7.57a |
6.83a |
5.74a |
63.03a |
74.47a |
|
|
3.2.2 SDS-PAGE. As shown in Fig. 2, size heterogeneity among the fractions was revealed by the electrophoretic separations. At both native and reduced state, the albumin and glutelin-1 consisted of three polypeptides (18.8, 22.1 and 51.5 kDa) and five peptides (20.7 to 51.5 kDa), respectively. This indicated that there was no disulfide bond among the peptides of albumin and glutelin-1, which was in accordance with their low content of sulfur amino acids (Table 1). On the other hand, significant difference between the unreduced glutelin-2 and reduced glutelin-2 was observed; the same was to the globulin. The two fractions may consist of polypeptides linked via one or more disulfide bonds. The components of prolamines were too faint to detective in this study.
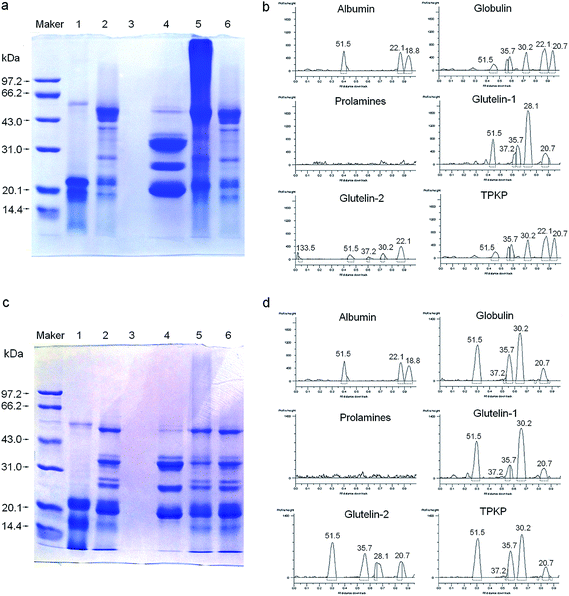 |
| Fig. 2 SDS-PAGE patterns and molecular weight distribution profiles of palm kernel expeller protein fractions in the absence (a and b) and presence (c and d) of β-mercaptoethanol; maker: molecular weight standards; track (1) albumin, (2) globulin, (3) prolamines, (4) glutelin-1 and (5) glutelins-2; TPKP, the total palm kernel expeller protein. | |
3.2.3 Gel chromatography. Compared with Fig. 2, the gel chromatography of each solubility fraction showed different result from that of the electrophoresis separation. This may be caused by the difference on the separation conditions between the electrophoresis and gel chromatography. All the albumin, globulin and glutelin-1 separated into three major peaks with molecular weight (Mw) ranging from 17.6 to 51.2 kDa (Fig. 3). The glutelin-2 exhibited two major peaks with a large amount of cross-contamination, and the major subunit (133.5 kDa) observed in SDS-PAGE was not detected by gel filtration. Similar result was found during the separation of coconut albumin.34
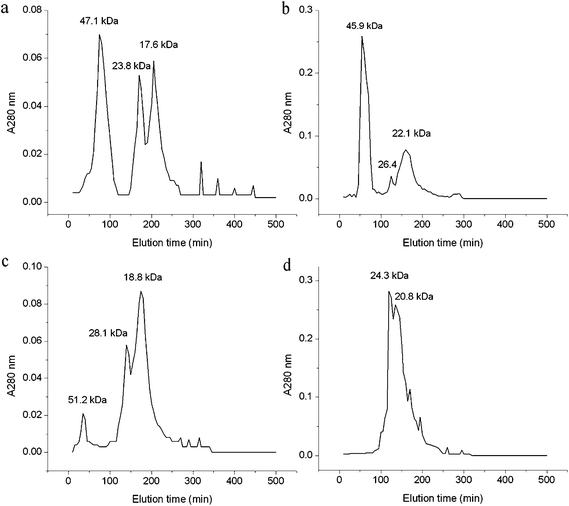 |
| Fig. 3 Gel filtration patterns of albumin (a), globulin (b), glutelin-1 (c) and glutelin-2 (d) on Sephadex G-100 in each extraction buffer. | |
3.2.4 Differential scanning calorimetry (DSC). As shown in Fig. 4, the globulin and prolamines separately showed an endothermic peak with a denaturation (Td) of approximately 53.8 °C and 126.5 °C. TPKP exhibited a broad endothermic peak with a denaturation (Td) of approximately 102.3 °C. Moreover, the albumin, glutelin-1 and glutelin-2 did not showed any remarkable thermal response, which suggested that protein denaturation probably had happened during the preparation of these fractions.13
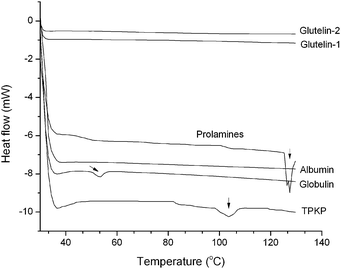 |
| Fig. 4 Differential scanning calorimetry thermograms of palm kernel expeller albumin, globulin, prolamines, glutelin-1, glutelin-2 and TPKP (the total palm kernel expeller protein); vertical arrows indicate the appearance of minor endothermic peak. | |
3.2.5 Emulsifying, foaming properties and viscosity. In general, all the fractions showed excellent emulsifying property but poor foaming property (Fig. 5). The glutelin-1 exhibited the highest emulsifying properties, which was probably due to its high S0 (Table 1). With larger Mw, the glutelin-2 exhibited higher FC than other samples. The globulin showed the highest foam stability and viscosity. Previous study also pointed out that emulsifying property of proteins was correlated with S0, and the peptides with low Mw had low viscosity and had no enough strength to form stable foams.7 Moreover, samples exhibited higher ESI and FC but lower EAI at lower ionic strength (≤0.4 M) than at high ionic strength. The viscosity was nearly linearly correlated to sample concentration, especially when the protein concentration was above 0.08 g mL−1. Furthermore, TPKP also showed a far superior emulsifying property than soy protein isolate and sesame seed protein isolate (Table 2).2,35 These results meant that the fractions and TPKP could be used as emulsifier or ingredients in products like meat replacers and soups.4
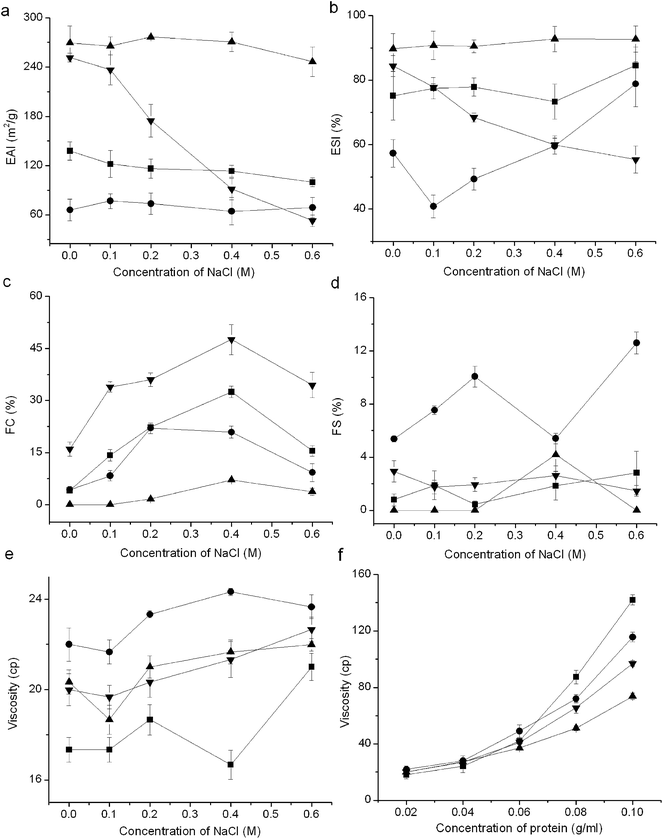 |
| Fig. 5 Emulsifying, foaming properties and viscosity of PKP fractions: (a) emulsifying activity index (EAI), (b) emulsion stability index (ESI), (c) foaming capacity (FC), (d) foaming stability (FS), (e) viscosity at different ionic strength, (f) viscosity in different protein concentration. (■) albumin, (●) globulin, (▲) glutelin-1, (▼) glutelin-2; vertical bars indicate the standard deviation. | |
Table 2 Functional properties of the palm kernel expeller protein fractions and the total palm kernel expeller protein (TPKP) with the comparison of soy protein isolate (SPI) and sesame seed protein isolate (SSPI)
Fractions |
Emulsifying capacity index (m2 g−1) |
Emulsion stability index (%) |
Foaming capacity (%) |
Foam stability (%) |
Viscosity (cp) |
References |
Different small letters in the same row meant significant difference (P < 0.05). Not measured. |
Albumin |
137.87 ± 5.27a |
75.13 ± 5.99a |
4.11 ± 0.51a |
0.78 ± 0.46a |
17.33 ± 0.55a |
|
Globulin |
65.89 ± 6.44a |
57.33 ± 4.26a |
4.33 ± 0.88a |
5.36 ± 0.22a |
22.00 ± 0.73a |
|
Glutelin-1 |
269.54 ± 10.58a |
89.71 ± 2.78a |
0.78 ± 0.12a |
0.20 ± 0.10a |
20.33 ± 0.52a |
|
Glutelin-2 |
251.58 ± 5.28a |
84.40 ± 3.27a |
16.00 ± 2.00a |
2.94 ± 0.19a |
20.00 ± 0.70a |
|
TPKP |
112.54 ± 8.39a |
24.44 ± 1.93a |
35.02 ± 3.12a |
17.61 ± 1.57a |
21.33 ± 0.58a |
|
SPI |
32.57 ± 0.76a |
43.08 ± 0.08a |
100.00 ± 2.50a |
19.2 ± 0.29a |
NDb |
2 |
SSPI |
70.00 ± 0.24a |
70.02 ± 0.02a |
4.20 ± 0.58a |
7.50 ± 0.10a |
53.10 ± 1.20a |
35 |
3.3 Antioxidant activities
3.3.1 Superoxide radical scavenging activity. Scavenging activity of superoxide radical is a major criterion to assess potential antioxidants.36 As shown in Table 3, all the samples showed considerable superoxide radical scavenging activity. And the glutelin-1 exhibited the highest ability, which was resulted from its high NPS, S0 and relatively high content of aromatic amino acids (Table 1), for aromatic amino acids (Tyr and Phe) could make active oxygen stable through direct electron transfer.37 This indicated that the glutelin-1 could be used as ingredients in cosmetic industry like skin care products.36
Table 3 Radical scavenging ability, antioxidant activity and ACE (angiotensin I-converting enzyme) inhibition of the five palm kernel protein fractions with the comparison of BHTa
Fractions |
Activity |
Free radical scavenging ability (%) |
Antioxidant activity |
ACE inhibition (%) |
Superoxide radical |
DPPH radical |
Hydroxyl radical |
ABTS radical |
Chelating activity |
Reducing power |
The concentration of all the palm kernel expeller protein fractions and BHT used to test free radical scavenging and antioxidant capacity was 100 μg mL−1, whereas the concentration of all the fractions used to test ACE inhibition was 1 mg mL−1. Significantly different (P < 0.05) from their respective controls in BHT. Not measured. |
Albumin |
35.04 ± 0.52b |
12.48 ± 0.87b |
55.61 ± 2.06b |
56.92 ± 2.86b |
17.08 ± 0.37b |
0.236 ± 0.017b |
4.31 ± 1.18 |
Globulin |
29.14 ± 1.91 |
45.20 ± 0.69b |
7.80 ± 1.51b |
42.56 ± 1.25b |
13.20 ± 1.38b |
0.253 ± 0.022b |
2.67 ± 1.04 |
Prolamines |
27.98 ± 3.77 |
79.45 ± 6.80 |
93.40 ± 1.46b |
79.35 ± 1.99b |
40.81 ± 0.98b |
0.455 ± 0.039b |
13.63 ± 2.18 |
Glutelin-1 |
49.54 ± 1.65b |
77.69 ± 5.51 |
34.41 ± 1.62b |
19.38 ± 1.51b |
9.23 ± 0.98b |
0.211 ± 0.009b |
0.22 ± 0.09 |
Glutelin-2 |
28.78 ± 2.37 |
50.98 ± 4.13b |
55.79 ± 5.37b |
64.19 ± 1.70b |
20.24 ± 0.37b |
0.319 ± 0.005 |
1.11 ± 0.80 |
BHT |
30.04 ± 0.76 |
73.5 ± 1.31 |
77.31 ± 0.58 |
8.84 ± 1.40 |
45.42 ± 1.40 |
0.343 ± 0.017 |
NDc |
3.3.2 DPPH radical scavenging activity. Results in Table 3 showed that both the prolamines and glutelin-1 exhibited higher DPPH radical scavenging activity than BHT and chickpea protein hydrolysates (44.31–71.26%), which was probably attributed to their high S0.38,39 However, the albumin showed the lowest scavenging activity. This may be due to its low S0 and low content of aromatic amino acid (Table 1).40
3.3.3 Hydroxyl radical scavenging activity. The fractions showed hydroxyl radical scavenging activity in the order of prolamines > glutelin-2 > albumin > glutelin-1 > globulin. Especially, prolamines exhibited a significantly higher scavenging ability than BHT (P < 0.05). Though owned similar amino acid composition and NPS values, the globulin exhibited much lower scavenging ability on hydroxyl and superoxide radical than albumin, which may result from its higher Mw and lower S0. Previous studies also shown that the antioxidative of proteins is dependent on their Mw.38,41,42
3.3.4 ABTS radical scavenging activity. The ABTS radical-scavenging activity of potential antioxidants was widely employed to estimate their total antioxidant activity. All the fractions exhibited much higher ABTS radical-scavenging activity than BHT, indicating that these fractions had considerable total antioxidant activity. The glutelin-1 showed the lowest activity among the samples, which may attribute to its special solubility (just soluble in dilute acidic solution) and molecular structure.
3.3.5 Chelating activity. Results in Table 3 demonstrated that all the fractions could exhibit antioxidant ability by capturing ferrous ion or other ions. The gluteins-2 showed the highest activity, which was probably attributed to the disulfide bonds in its molecular structure (Fig. 2). Previous study had demonstrated that disulfide bonds or sulfhydryl was consisted with the ionic chelating activity of proteins.37 Furthermore, the prolamines also exhibited good chelating activity.
3.3.6 Reducing power. The reducing power, denoted by monitoring the Fe2+ concentration was also included in Table 3. The highest reducing powder was found in prolamines, followed by glutelin-2, globulin, albumin and glutelin-1. Moreover, the reducing power of samples was corresponding to their ABTS radical-scavenging activity, which indicated that reducing power and antioxidant activity were correlated. Similar trend was observed by previous studies.38,39
3.3.7 Inhibition of linoleic acid autoxidation. As shown in Fig. 6, the prolamines showed the highest inhibition ability on linoleic acid autoxidation, which was probably due to its high aromatic acids content and good solubility in liquid. The higher inhibition ability of glutelin-1 and glutelin-2 may be contributed to their high content of sulfur amino acid and aromatic amino acids (Table 1). Qian et al.37 reported that Cys residue could protect lipid and other biomolecules by donating protons to peroxyl radicals and other free radicals, and Tyr or Phe could make active oxygen stable through direct electron transfer. It indicated that these fractions could be used as natural antioxidant.
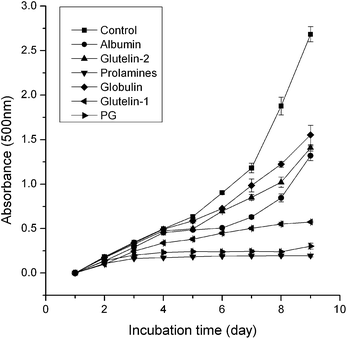 |
| Fig. 6 Lipid peroxidation measured in linoleic acid model system for 8 days in the presence of samples. higher absorbance at 500 nm represents higher linoleic acid peroxidation. control: the autoxidation of linoleic acid. | |
3.3.8 ACE inhibition. As shown in Table 3, all the fractions showed poor ACE inhibitory activity. This may be due to their relatively large molecular mass, for the majority of ACE-inhibitory peptides were short sequences containing from 2 to 12 amino acids.8 Nevertheless, the prolamines exhibited the highest ACE inhibition among the samples, which was probably because of its low molecular weight (Fig. 2) and rather high content of aromatic amino acids (Table 1).
4. Conclusions
Five solubility fractions were successfully fractionated from palm kernel expeller by the sequential extraction method. The yield of all the fractions was nearly 90.74% of the total PKP, which was higher than that of the trypsin-assisted extraction method (61.99%).2 The cost of the sequential extraction method was also lower compared to the trypsin-assisted extraction method. The fractions showed obvious heterogeneity in amino acid composition, peptides distribution and nutritional quality. Both the glutelin-2 and TPKP showed a relatively balanced amino acid composition and good nutritional quality, indicating that they were new source of edible protein. All the fractions exhibited excellent emulsifying properties and considerable antioxidant activity, meaning their potential usage as emulsifier, natural antioxidant, ingredients in products like meat replacers, soups, skin care products and so on.
Moreover, the emulsifying properties, foaming properties and viscosity of the fractions seemed to be correlated with their S0 and molecular size, and these fractions exhibited better functional properties at low ionic strength (≤0.4 M). The antioxidative activity was probably influenced by amino acid composition, S0 and molecular size. In addition, the results of ACE inhibition assay in this study indicated that peptides with high ACE inhibitory activity could be obtained from the albumin and prolamines, and further investigations should be carried out.
Acknowledgements
This work was supported by program of Science and Technology of 12th Five-year Plan (no. 2012BAD31B03).
References
- A. R. Alimon, The nutritive value of palm kernel cake for animal feed, Palm Oil Development, 2010, 40, 12–14 Search PubMed.
- K. L. Chee, H. K. Ling and M. K. Ayob, Optimization of trypsin-assisted extraction, physicochemical characterization nutritional qualities and functionalities of palm kernel cake protein, LWT–Food Sci. Technol., 2012, 46, 419–427 CrossRef CAS.
- V. A. Johnson and C. L. Lay, Genetic improvement of plant proteins, J. Agric. Food Chem., 1974, 22, 497–566 CrossRef.
- K. Tsumura, T. Satio, K. Tsuge, H. Ashida, W. Kugimiya and K. Inouye, Functional properties of soy protein hydrolysates obtained by selective proteolysis, LWT–Food Sci. Technol., 2005, 38, 255–261 CrossRef CAS.
- B. Hernandez-Ledesma, I. Recio and L. Amigo, β-lactoglobulin as source of bioactive peptides, Amino Acids, 2008, 35, 257 CrossRef CAS PubMed.
- F. Morcillo, F. Aberlenc-Bertossi, P. Trouslot, S. Hamon and Y. Duval, Characterization of 2S and 7S storage proteins in embryos of oil palm, Plant Sci., 1997, 122, 141–151 CrossRef CAS.
- F. Shahidi, X. Q. Han and J. Synowiecki, Production and characteristics of protein hydrolysates from capelin (Mallotus villosus), Food Chem., 1995, 53, 285–293 CrossRef CAS.
- B. Hernandez-Ledesma, M. D. Mar and I. Recio, antihypertensive peptides: Production, bioavailability and incorporation into foods, Adv. Colloid Interface Sci., 2011, 165, 23–35 CrossRef CAS PubMed.
- C. M. Liyana-Pathiranaa and F. Shahidi, Antioxidant and free radical scavenging activities of whole wheat and milling fractions, Food Chem., 2007, 101, 1151–1157 CrossRef.
- J. Borawska, M. Darewicz, G. E. Vegarud, A. Iwaniak and P. Minkiewicz, Ex vivo digestion of carp muscle tissue- ACE inhibitory and antioxidant activities of obtained hydrolysates, Food Funct., 2014, 1, 10.1039/c4fo00621f.
- S. Y. Zhong, C. W. Ma, Y. C. Lin and Y. K. Luo, Antioxidant properties of peptide fractions from silver carp (hypophthalmichthys molitrix) processing by-product protein hydrolysates evaluated by electron spin resonance spectrometry, Food Chem., 2011, 126, 16–1642 CrossRef PubMed.
- B. Hu and A. Esen, Heterogeneity of soybean seed proteins: one-dimensional electrophoretic profiles of six different solubility fractions, J. Agric. Food Chem., 1981, 29, 497–501 CrossRef CAS PubMed.
- M. R. N. Angelia, R. N. Garcia, K. M. P. Caldo, K. Prak, S. Utsumi and E. M. Tecson-Mendoza, Physicochemical and functional characterization of cocosin, the coconut 11S globulin, Food Sci. Technol. Int., 2010, 16(3), 225–232 CrossRef CAS PubMed.
- MPOB, http://econ.mpob.gov.my/stat/web_report1php?val=200907, (accessed December 2010).
- M. M. Bradford, A rapid and sensitive method for the quantitation of microgram quantities of protein utilizing the principle of protein dye binding, Anal. Biochem., 1976, 72, 248–254 CrossRef CAS PubMed.
- Association of Official Analytical Chemists, Official methods of analysis, Arlington, 15th edn, 1990 Search PubMed.
- X. S. Wang, C. H. Tang, X. Q. Yang and W. R. Gao, Characterization, amino acid composition and in vitro digestibility of hemp (Cannbis sativa L.) proteins, Food Chem., 2008, 107, 11–18 CrossRef CAS.
- C. C. Bigelow, On the coverage hydrophobicity of proteins and the relationship between it and protein structure, J. Theor. Biol., 1976, 16, 187–211 CrossRef.
- R. M. Saunders, M. A. Connor, A. N. Booth, M. M. Bickoff and G. O. Kohler, Measurement of digestibility of alfalfa protein concentrate by in vivo and in vitro methods, J. Nutr., 1973, 103, 530–535 CAS.
- L. Morrup and E. S. Olesen, New method for prediction of protein value from essential amino acid pattern, Nutr. Rep. Int., 1976, 13, 355–365 Search PubMed.
- Food and Agricultural Organization/World Health Organization, Protein quality evaluation, FAO/WHO Nutrition Meetings, Report Series 51, Rome, 1990 Search PubMed.
- U. K. Laemmli, Cleavage of structural proteins during the assembly of the head bacteriophage T4, Nature, 1970, 227, 680–685 CrossRef CAS PubMed.
- K. X. Zhu, X. H. Sun, Z. C. Chen, W. Peng, H. F. Qian and H. M. Zhou, Comparison of functional properties and secondary structures of defatted wheat germ proteins separated by reverse micelles and alkaline extraction and isoelectric precipitation, Food Chem., 2010, 123(4), 1163–1169 CrossRef CAS.
- L. H. D. B. Soares, F. Albuquerque, F. Assmann and M. A. Z. Ayub, Physicochemical properties of three food proteins treated with transglutaminase, Cienc. Rural, 2004, 34, 1219–1223 CrossRef.
- S. Marklund and G. Marklund, Involvement of the superoxide anion radical in the autoxidation of pyrogallal and a convenient assay for superoxide dismutase, Eur. J. Biochem., 1974, 47, 469–474 CrossRef CAS PubMed.
- B. Zhang, Y. Luo and Q. Wang, Effect of acid and base treatments on structural, rheological, and antioxidant properties of zein, Food Chem., 2011, 124(1), 210–220 CrossRef CAS.
- J. T. J. Mariken, J. Arts, S. Dallinga, H. P. Voss, G. R. M. M. Haenen and A. Bast, A new approach to assess the total antioxidant capacity using the TEAC assay, Food Chem., 2004, 88, 567–570 CrossRef.
- S. Sakanaka and Y. Tachibana, Active oxygen scavenging activity of egg-yolk protein hydrolysates and their effects on lipid oxidation in beef and tuna homogenates, Food Chem., 2006, 95, 243–249 CrossRef CAS.
- B. H. Kong and Y. L. Xiong, Antioxidant activity of zein hydrolysates in a liposome system and the possible mode of action, J. Agric. Food Chem., 2006, 54, 6059–6068 CrossRef CAS PubMed.
- I. F. F. Benzie and J. J. Strain, The ferric reducing ability of plasma (FRAP) as a measure of “antioxidant power”: The FRAP assay, Anal. Biochem., 1996, 239, 70–76 CrossRef CAS PubMed.
- H.-C. Wu, H.-M. Chen and C.-Y. Shiau, Free amino acids and peptides as related to antioxidant properties in protein hydrolysates of mackerel (Scomber austriasicus), Food Res. Int., 2003, 36, 949–957 CrossRef CAS.
- H. Mitsuda, K. Yasumoto and K. Iwami, Antioxidative action of indole compounds during the autoxidation of linoleic acid, Eiyo to Shokuryo, 1996, 19, 210–214 CrossRef.
- V. K. Jimsheena and L. R. Gowda, Colorimetric, high-throughput assay for screening angiotensin I-converting enzyme inhibitors, Anal. Chem., 2009, 81, 9388–9394 CrossRef CAS PubMed.
- K. Kwon, K. H. Park and K. C. Rhee, Fractionation and characterization of proteins from coconut (cocos nucifera L.), J. Agric. Food Chem., 1996, 44(7), 1741–1745 CrossRef CAS.
- E. K. Khalid, E. E. Babiker and A. H. EL Tinay, Solubility and functional properties of sesame seed proteins as influenced by pH and/or salt concentration, Food Chem., 2003, 82, 361–366 CrossRef CAS.
- R. J. Elias, S. S. Kellerby and E. A. Decker, Antioxidant activity of proteins and peptides, Crit. Rev. Food Sci. Nutr., 2008, 48, 430–441 CrossRef CAS PubMed.
- Z. J. Qian, W. K. Jung, H. G. Byun and K. S. Kim, Protective effect of an antioxidative peptide purified from gastrointestinal digests of oyster, Crassostrea gigas against free radical induced DNA damage, Bioresour. Technol., 2008, 99, 3365–3371 CrossRef CAS PubMed.
- Y. H. Li, B. Jiang, T. Zhang, W. M. Mu and J. Liu, Antioxidant and free radical-scavenging activity of chickpea protein hydrolysate (CPH), Food Chem., 2008, 106, 444–450 CrossRef CAS.
- C. H. Tang, X. S. Wang and X. Q. Yang, Enzymatic hydrolysis of hemp (Cannabis sativa L.) protein isolate by various proteases and antioxidant properties of the resulting hydrolysates, Food Chem., 2009, 114, 1484–1490 CrossRef CAS.
- K. Suetsuna, H. Ukeda and H. Ochi, Isolation and characterization of free radical scavenging activities peptides derived from casein, J. Nutr. Biochem., 2000, 11, 128–131 CrossRef CAS PubMed.
- N. Rajapakse, E. Mendis, W. K. Jung, J. Y. Je and S. K. Kim, Purification of a radical scavenging peptide from fermented mussel sauce and its antioxidant properties, Food Res. Int., 2005, 38, 175–182 CrossRef CAS.
- A. Pihlanto, Review: antioxidative peptides derived from milk proteins, Int. Dairy J., 2006, 16, 1306–1314 CrossRef CAS.
Footnote |
† The two authors contributed equally to this work. |
|
This journal is © The Royal Society of Chemistry 2015 |
Click here to see how this site uses Cookies. View our privacy policy here.