DOI:
10.1039/C5RA07987J
(Paper)
RSC Adv., 2015,
5, 58600-58608
Click synthesis of a polyamidoamine dendrimer-based camptothecin prodrug†
Received
30th April 2015
, Accepted 29th June 2015
First published on 29th June 2015
Abstract
In the present work we report on the click synthesis of a new camptothecin (CPT) prodrug based on an anionic polyamidoamine (PAMAM) dendrimer intended for cancer therapy. We applied ‘click’ chemistry to improve the polymer-drug coupling reaction efficiency. Specifically, CPT was functionalized with a spacer, 1-azido-3,6,9,12,15-pentaoxaoctadecan-18-oic acid (APO), via a EDC/DMAP coupling reaction. In parallel, propargylamine (PPA) and methoxypoly(ethylene glycol) amine were conjugated to PAMAM dendrimer G4.5 in sequence using an effective coupling agent 4-(4,6-dimethoxy-(1,3,5)triazin-2-yl)-4-methyl-morpholinium chloride (DMTMM). CPT–APO was then coupled to the PEGylated PAMAM dendrimer G4.5–PPA via a click reaction using copper bromide/2,2′-bipyridine/dimethyl sulfoxide (catalyst/ligand/solvent). Human glioma U1242 cells were exposed to the CPT prodrug to determine the toxicity and cell cycle effects using a WST-1 assay and flow cytometry. The CPT prodrug displayed a dose-dependent toxicity with an IC50 of 5 µM, a 185-fold increase relative to free CPT, as a result of slow release. Furthermore, conjugated CPT resulted in G2/M arrest and cell death while the PEGylated dendrimer had little to no toxicity. Altogether, highly efficient click chemistry allows for the synthesis of multifunctional dendrimers for sustained drug delivery.
Introduction
Dendrimers have attracted increasing attention as drug carriers in that they possess a high degree of molecular uniformity, high drug loading capacity, and the ability to accommodate various functional entities.1–3 Thus, various dendrimer-based drug delivery platforms have been developed,4–7 and their utility for delivering anticancer drugs has yielded promising results.8–14 Regardless, a commonly encountered problem is the heterogeneity of the ligand and drug on the dendrimer surface during chemical synthesis.15 It is essential to obtain a uniform distribution of the ligand and drug for standardizing therapeutic effects and for the successful translation of dendrimer-based nanomedicines to clinical application. Robust and efficient coupling methods must be applied to overcome such issues.
Camptothecin is a plant alkaloid isolated from Camptotheca acuminata of the Nyssaceae family with remarkable anticancer activity by inhibiting both DNA and RNA synthesis.16 Camptothecin has shown potency against many types of cancer despite its low water solubility and poor stability of the lactone form, a required form for therapeutic activity.17 In particular, it is of advantage to use CPT to treat brain cancer as it selectively kills proliferating (S-phase) cells, thus exerting little to no toxicity to non-dividing normal cells resident of the brain.18,19 Polymer–CPT conjugates have been made by various methods.20–22 Although several dendrimer–CPT derivatives have been made in the past, the efficiency using high capacity of dendrimer to deliver CPT was low. For instance, Thiagarajan et al. applied EDC/NHS chemistry to conjugate CPT to the dendrimer via a glycine spacer.23 Less than 20% of CPT used in the reaction was successfully attached to the dendrimer. Copper-catalyzed azide-alkyne cycloaddition (CuAAC), the best known example of a “click” reaction, is a highly efficient and selective synthetic method. It has proven to be a powerful strategy for precisely loading drugs to various polymeric carriers including dendrimers.22,24,25 The current study reports on the use of click chemistry for the synthesis of water soluble polyamidoamine (PAMAM) dendrimer–camptothecin (CPT) prodrug conjugates towards development of an enabling modular dendrimer-based delivery system for cancer therapy. In particular, we applied CuAAC for improving coupling reaction efficiency in the synthesis of CPT–dendrimer conjugates. Anionic PAMAM dendrimer G4.5 was used as the carrier because of low toxicity and low non-specific cellular uptake. It was modified with polyethylene glycol (PEG) for improved water solubility and cytocompatibility. CPT was click coupled to the dendrimer carrier via a short heterobifunctional spacer. The click synthesis and characterization of the resulting dendrimer-based CPT prodrug and its therapeutic activity are reported herein.
Experimental section
Materials
EDA core PAMAM dendrimer G4.5 carboxylate sodium salt was purchased from Dendritech (Midland, MI). 2,2′-Bipyridine, 4-dimethylaminopyridine (DMAP), copper(II) sulfate (CuSO4), (+)-sodium L-ascorbate, 4-(4,6-dimethoxy-(1,3,5)triazin-2-yl)-4-methyl-morpholinium chloride (DMTMM), deuterated solvents, dichloromethane (DCM), dimethyl sulfoxide (DMSO), and other organic solvents were purchased from Acros (Morris Plains, NJ). (S)-(+)-Camptothecin (CPT), propargylamine (PPA), copper bromide (CuBr), N-(3-dimethylaminopropyl)-N′-ethylcarbodiimide hydrochloride (EDC), sodium bicarbonate (NaHCO3), trifluoroacetic acid (TFA) and silica gel 60 (40–63 µm, 230–400 mesh) were purchased from Sigma-Aldrich (St. Louis, MO). 1-Azido-3,6,9,12,15-pentaoxaoctadecan-18-oic acid (APO) and methoxypoly(ethylene glycol)amine (mPEG-NH2, 2000 g mol−1) were purchased from Biomatrik (Jiaxing, Zhejiang, China) and JenKem Technology USA (Plano, TX), respectively. SnakeSkin dialysis tubing 3.5 kDa and 7 kDa MWCO, acetonitrile (ACN), HPLC grade water, phosphate-buffered saline (PBS), and magnesium sulfate (MgSO4) were purchased from Thermo Fisher Scientific (Pittsburg, PA).
Instrumentation
1H and 13C NMR spectra were recorded on a Bruker AVANCEIII 600 MHz spectrometer. Mass spectra were obtained on AB Sciex 5800 MALDI TOF-TOF using α-cyano-4-hydroxycinnamic acid (4-HCCA) as matrix. UV-Vis spectra were acquired on an Agilent 8453 spectrophotometer. The dendrimer conjugates in solutions were characterized with Malvern Zetasizer Nano ZS90 (Malvern Instruments, Worcestershire, U.K.).
Synthesis of CPT–APO
To a suspension of CPT (200 mg, 0.57 mmol) in 60 mL of DCM were added EDC (330 mg, 1.73 mmol) and DMAP (140 mg, 1.14 mmol) followed by APO (290 mg, 0.86 mmol) pre-dissolved in 5 mL of DCM. After having been stirred for 24 h at room temperature, the reaction mixture was poured into 50 mL of water. The organic phase was collected. The aqueous phase was extracted with DCM two times. The DCM fractions were combined and dried over MgSO4. Upon the removal of DCM by rotary evaporation, the obtained CPT–APO was further purified by column chromatography on silica gel using a DCM/methanol mixture (97%/3%, v/v), yield 67%. 1H NMR (d6-DMSO, 600 MHz): δ (ppm) 8.68 (s, 1H), 8.15 (d, J = 8.5 Hz, 1H), 8.12 (d, J = 8.2 Hz, 1H), 7.86 (t, J = 7.9 Hz, 1H), 7.71 (t, J = 7.5 Hz, 1H), 7.16 (s, 1H), 5.50 (s, 2H), 5.28 (q, 2H), 3.83–3.33 (m, 22H), 2.81 (m, 1H), 2.66 (m, 1H), 2.14 (m, 2H), 0.94 (t, J = 7.4 Hz, 3H). 13C NMR (CDCl3, 600 MHz): δ (ppm) 170. 81, 167.63, 157.57, 152.61, 149.04, 146.36, 146.26, 131.33, 130.83, 129.80, 128.66, 128.39, 128.34, 128.20, 120.25, 96.54, 76.25, 70.86, 70.79, 70.70, 70.19, 67.19, 66.54, 50.86, 50.10, 35.12, 31.92, 7.79. MALDI-TOF MS (m/z): [M + H]+ calculated for C33H40N5O10: 666.27, found: 666.22.
Synthesis of G4.5–PPA
To a solution of PAMAM dendrimer G4.5 in carboxyl form (50 mg, 2.1 µmol) in 3 mL of 0.1 M NaHCO3 was added DMTMM (58 mg, 0.21 mmol) followed by addition of 0.5 mL of DMF containing 0.13 mmol PPA. The reaction mixture was stirred overnight. Upon the removal of the solvents under reduced pressure, the remaining residue was dialyzed against water using SnakeSkin dialysis tube with MWCO 3.5 kDa and freeze-dried to yield 53 mg of G4.5–PPA. 1H NMR (D2O, 600 MHz): δ (ppm) 3.98 (m, 2H, CH2C
CH), 2.43–3.69 (m, methylene protons of G4.5).
Synthesis of PPA–G4.5–PEG
To a solution of G4.5–PPA (40 mg, 1.7 µmol) and DMTMM (17 mg, 61 μmol) in 4 mL of 0.1 M NaHCO3 was added mPEG-NH2 (102 mg, 51 µmol). The mixture was stirred overnight at room temperature, dialyzed against water using SnakeSkin dialysis tubing with 7.0 kDa MWCO for 48 h, and then freeze-dried to obtain 86 mg of PPA–G4.5–PEG. 1H NMR (D2O, 600 MHz): δ (ppm) 3.99 (m, 2H), 3.73 (br.s, methylene protons in PEG repeat units), 3.41 (s, 3H), 3.40–2.42 (m, methylene protons of G4.5).
Synthesis of CPT–G4.5–PEG
PPA–G4.5–PEG (20 mg, 0.26 µmol), CPT–APO (2.9 mg, 4.4 µmol), and 2,2′-bipyridine (0.6 mg, 3.8 µmol) were mixed in 1 mL of DMSO. The molar feed ratio of CPT to dendrimer in this reaction was 17
:
1, which was determined based on availability assessment for the alkyne groups on the dendrimer (see ESI†). The obtained mixture underwent 3 times of freeze–pump–thaw cycling for degassing. Afterwards, CuBr (0.3 mg, 2.1 µmol) dissolved in 40 µL of DMSO was added to the mixture solution. The reaction mixture under nitrogen was stirred in dark overnight at room temperature and then poured into 5 mL of water. After 1 h-stirring, the solvents were removed under reduced pressure. Extraction of unreacted CPT–APO was conducted by vortexing the obtained solid residue with ether (1 mL each time) followed by centrifugation for liquid–solid separation. The extraction procedure was repeated until CPT–APO became undetectable in ether by UV-Vis spectrophotometer. The remaining solid was dissolved in 1 mL of water followed by centrifugation. The liquid phase was collected and freeze-dried to yield CPT–G4.5–PEG. The synthesis steps are illustrated in Scheme 1. UV-Vis spectroscopy analysis confirmed that conjugated CPT accounted for 6.4 wt% of the product. 1H NMR (d6-DMSO, 600 MHz): δ (ppm) 8.64 (br.s, 1H), 8.12 (br.s, 2H), 7.85 (br.s, 2H), 7.68 (br.s, 1H), 7.14 (br.s, 1H), 5.50 (s, 2H), 5.26 (br.s, 2H), 4.44 (br.s, 2H), 4.26 (br.s, 2H), 3.50 (br.s, methylene protons in the repeat unit of PEG), 3.23 (s, 3H), 2.06–2.95 (m, methylene protons of G4.5), 0.94 (s, 3H).
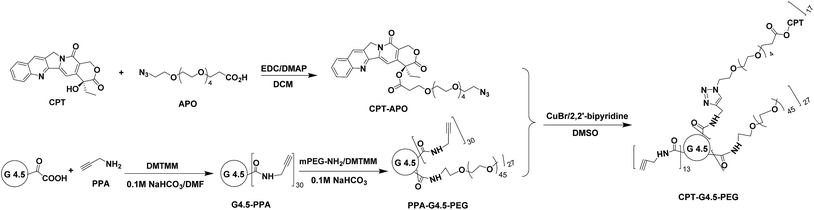 |
| Scheme 1 Synthesis of CPT–G4.5–PEG conjugates. | |
In vitro drug release
The CPT-containing polymers (0.5 mg mL−1) were incubated in pH 7.4 PBS at 37 °C. Aliquots (20 µL) of drug release medium were taken at different time points and analyzed with reverse-phase high-performance liquid chromatography (RP-HPLC) (Waters, Milford, MA). The RP-HPLC system was equipped with Waters 717plus autosampler, XTerra RP18 column (5 µm, 4.6 × 150 mm), Waters 1515 isocratic HPLC pump, and Waters 2487 dual absorbance detector. The mobile phase was comprised of 74.97 v% H2O, 24.99 v% ACN and 0.04 v% TFA. The eluent with a flow rate at 1.0 mL min−1 was monitored by the UV detector at a wavelength of 370 nm for CPT.
Cell culture
Human glioma U1242 cells were cultured in Dulbecco's modified Eagle's medium (DMEM) supplemented with 10% Cosmic calf serum at 37 °C in 95% air/5% CO2.26
Cytotoxicity assay
Human glioma U1242 cells were seeded at a density of 1 × 104 cells per well in a 96-well cell culture plate and cultured for 1 day to allow cell attachment. The cells were then treated with various concentrations (0–50 µM) of CPT in either free or conjugated form for 2 days. For comparison, toxicity of PPA–G4.5–PEG conjugates at the same molar concentrations as CPT–G4.5–PEG conjugates was examined for comparison. Cell viability relative to untreated cells was then determined by WST-1 proliferation assay. GraphPad Prism 5 was used to perform the curve fitting and then determine the 50% maximal inhibitory concentrations of free CPT (IC50free) and conjugated CPT (IC50conjugated).
Cell cycle analysis
Human glioma U1242 cells (1 × 106) were seeded in a 100 mm cell culture dish and cultured for 1 day to allow cell attachment. The cells were then treated with CPT at the concentration of 2 × IC50free, conjugated CPT at the concentration of 2 × IC50conjugated and PPA–G4.5–PEG conjugates at the equivalent molar concentration of CPT–G4.5–PEG conjugates for various lengths of time (6, 12, and 24 h). The cells treated with PBS were used as a control. At the end of each treatment, the cells were washed with PBS, trypsinized, and re-suspended in fresh cell culture medium. Following the centrifugal removal of the medium, the cells were fixed with cold 70% ethanol and maintained at 4 °C for 1 h. Finally, the cells were washed with PBS three times and incubated with RNase at a final concentration of 1 µg mL−1 and propidium iodide at a final concentration of 50 µg mL−1 at 37 °C for 30 min. The cells were then analyzed by flow cytometry using a Guava EasyCyte mini flow cytometry system (Millipore, Billerica, MA).27
Results and discussion
Characterization
We synthesized clickable PAMAM dendrimer G4.5 and used it as a carrier to deliver CPT as half generation PAMAM dendrimers are generally less toxic and have lower nonspecific cellular uptake as opposed to full generation dendrimers.28,29 Given the hydrophobicity of CPT, the dendrimer drug loading degree should be carefully controlled to avoid generating water insoluble entities. The incorporation of hydrophilic molecules such as PEG onto the dendrimer surface has proven effective in improving water solubility and enhancing cytocompatibility.29,30 Thus, this step was applied in the synthesis of our dendrimer–CPT conjugates. A short hetero-bifunctional spacer, i.e., APO bearing azide and carboxyl groups, was used to modify CPT via EDC/DMAP chemistry. The reaction proceeded successfully in DCM. The purity of CPT–APO and CPT–G4.5–PEG conjugates was verified based on the RP-HPLC analysis. As shown in Fig. S-1 (ESI†), unreacted CPT (retention time 1.90 minutes) was successfully removed from CPT–APO (retention time 2.02 minutes) and CPT–G4.5–PEG conjugates (retention time 1.02 minutes). PAMAM dendrimer G4.5 bearing carboxylic acid terminals (0.5 mg mL−1) was found to possess a zeta potential of −18.5 mV. The zeta potential of the resulting conjugates (0.5 mg mL−1) was −8.3 mV. The less negative zeta potential indicated dendrimer surface property change as a result of PEGylation and CPT coupling.
1H NMR spectrum clearly shows proton signals from both CPT and APO (Fig. 1). In particular, multiple proton signals between 3.33 and 3.83 ppm are from methylene protons in APO. The signal at 5.28 ppm is assigned to the two methylene protons in the CPT lactone ring, indicating that therapeutically active lactone form remained. Two doublets for the methylene protons in the lactone ring was also clearly seen in the 1H NMR spectrum based on CDCl3 (Fig. S-3, ESI†). 13C NMR spectra (Fig. S-4 and S-5, ESI†) provide complementary information for CPT–APO conjugates.
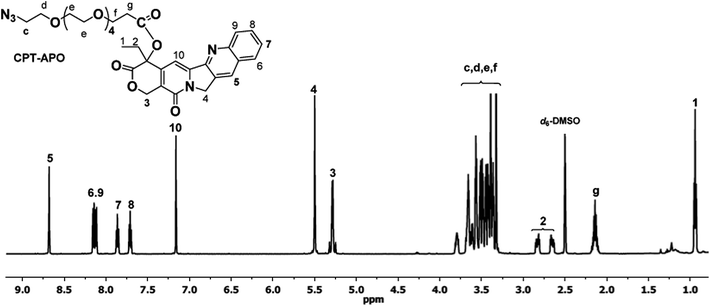 |
| Fig. 1 1H NMR spectrum of CPT–APO in d6-DMSO. | |
To make clickable dendrimers, alkyne groups were introduced onto the PAMAM dendrimer surface via reaction with PPA using coupling reagent DMTMM. 1H NMR spectrum of G4.5–PPA (Fig. 2) shows multiple peaks of dendrimer methylene protons in the range 2.43–3.69 ppm along with multiplet at 3.98 ppm assigned to methylene protons (a) adjacent to the alkyne group of PPA, confirming the successful amide bond formation. Based on the 1H NMR integration analysis, the synthesized G4.5–PPA conjugates carried 30 alkyne groups per dendrimer.
 |
| Fig. 2 1H NMR spectrum of G4.5–PPA in D2O. | |
G4.5–PPA was further PEGylated by coupling mPEG-NH2 (2000 Da) to the dendrimer surface in the presence of DMTMM. The 1H NMR spectrum shown in Fig. 3 confirms successful covalent attachment of mPEG to G4.5–PPA. In addition to the identification of the proton signals from PPA and dendrimer moieties, a broad singlet centered at 3.73 ppm is assigned to the methylene protons of PEG repeat units while a singlet at 3.41 ppm is due to the methyl protons. Based on the 1H NMR spectrum, the degree of PEGylation was calculated to be 27.
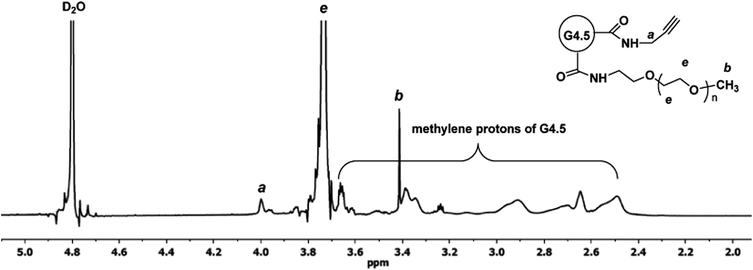 |
| Fig. 3 1H NMR spectrum of PPA–G4.5–PEG in D2O. | |
The final step of the synthesis involves a click reaction between alkyne-carrying PEGylated PAMAM dendrimer G4.5 (i.e., PPA–G4.5–PEG) and azide-carrying CPT (i.e., CPT–APO) in the presence of CuBr/2,2′-bipyridine (catalyst/ligand) system in DMSO. Using the alkyne accessibility analysis result as a guide (see ESI Fig. S-6†), the feed molar ratio (17
:
1) of CPT to dendrimer was used in the click reaction. The resulting CPT–G4.5–PEG conjugates were characterized by 1H NMR spectroscopy. The proton signals of CPT and spacer APO in the conjugates are identified in Fig. 4. A further downfield shift of the signal of methylene protons (a′, 4.26 ppm) adjacent to the ring opposed to proton signal (a, 3.99 ppm) resulted from the triazole linkage formation. In addition, methine proton (b′) in the triazole ring appears as a singlet at 7.85 ppm, overlapping the benzene proton signal of CPT. The proton signal at 3.99 ppm (a) indicates the presence of remaining alkyne groups. Because of proton signal interference by the deuterated solvent d6-DMSO in the spectrum, CPT coupled to the conjugates was quantified by using UV-Vis spectroscopy. Based on the UV absorbance at 370 nm (Fig. S-7, ESI†), the final polymer–drug conjugates carried approximately 17 CPT molecules per dendrimer. The estimated drug loading degree matches the feed molar ratio used in the click reaction, indicating a 100% conversion rate for the utility of CPT in the click reaction. The overall molecular weight of CPT–G4.5–PEG was estimated to be 90
657 g mol−1.
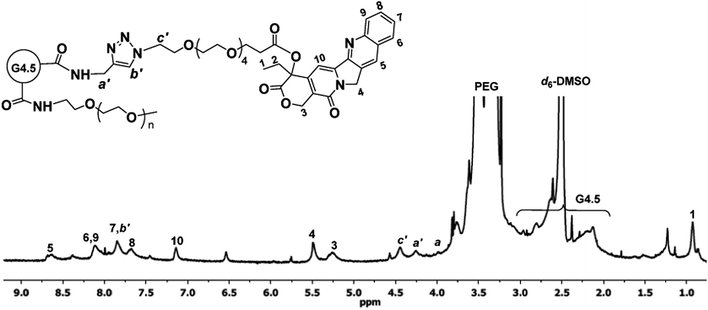 |
| Fig. 4 1H NMR spectrum of CPT–G4.5–PEG in d6-DMSO. | |
In vitro drug release studies were performed on CPT–G4.5–PEG conjugates to assess CPT release rate and potential utility of the resulting conjugates in delivery applications. RP-HPLC was used to measure CPT release kinetics. CPT was slowly released via hydrolysis of the ester bond between CPT and APO. About 10.6% CPT was released from the conjugates, following zero order kinetics over the course of 7 days (Fig. 5). CPT release kinetics may be modulated to achieve a desirable rate and duration of drug action in future work. Efforts may include utility of more labile chemical bonds (e.g., pH sensitive bonds, enzymatic sensitive bonds) and more hydrophilic spacer for drug-spacer coupling for faster release, increasing drug loading, and so on.
 |
| Fig. 5 (A) Representative RP-HPLC chromatograms of CPT–G4.5–PEG incubated in pH7.4 PBS for various lengths of time (i.e., 0, 3, and 7 days). (B) In vitro release profile of CPT conjugated to PAMAM dendrimer G4.5 based on RP-HPLC measurements. | |
Drug activity evaluation
CPT interferes with the breakage–reunion reaction of DNA topoisomerase I (Top1) often referred to as the cleavable complex necessary to relieve DNA stress during DNA replication.16 In doing so, a ternary complex of Top1–CPT–DNA forms, leading to generation of double-strand breaks during DNA replication. The cytotoxicity of the synthesized conjugates to human glioma U1242 cells was then assessed. CPT–G4.5–PEG cytotoxicity was found to be dose-dependent with an IC50 of 5 µM (Fig. 6A). In comparison, the IC50 of free CPT was 27 nM. Conjugated CPT is expected to become therapeutically active following hydrolysis of the ester linkage between CPT and the dendrimer. As expected, the conjugated CPT exhibited much lower potency than free CPT at equivalent concentrations because of slow release as presented in Fig. 5. Thus, the conjugates are capable of sustaining therapeutic activity for a longer period of time. PEGylated PAMAM dendrimer carrier without CPT is cytocompatible and does not cause toxicity to cells at concentrations up to 50 µM (Fig. 6B).
Prolonged exposure to CPT is expected to bring about DNA double-strand breaks during replication hence resulting in an arrest in G2/M of the cell cycle if not repaired.18,31 We performed cell cycle analyses following treatment with PBS, free CPT, CPT–G4.5–PEG or PEGylated dendrimer carrier. As shown in Fig. 7, cells treated with free CPT or CPT–G4.5–PEG conjugate exhibited a remarkable decrease in the G0/G1 cell population and an increase in G2/M going from a little more than 20% to more than 40% over a period of 24 h. Untreated cells (PBS group) showed a normal cell cycle distribution with a larger fraction in the G0/G1 phase. The cells treated with PPA–G4.5–PEG conjugate showed a similar cell cycle distribution as untreated cells, indicating that the PPA–G4.5–PEG conjugate had little to no effect on cell cycle progression. Consistent with cytotoxicity assessment, cell cycle analyses further demonstrated that CPT–G4.5–PEG induced an arrest in G2/M and lead to apoptosis as indicated by a temporal increase in the sub-G1 population.
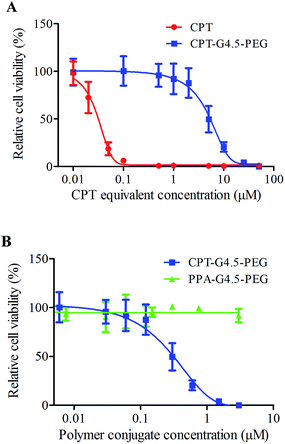 |
| Fig. 6 Cytotoxicity of free CPT, CPT–G4.5–PEG and PPA–G4.5–PEG conjugates in U1242 cells. The data points are mean ± SD. | |
 |
| Fig. 7 Cell cycle analysis. U1242 cells were left untreated (control), CPT at the concentration of 2 × IC50free, conjugated CPT at the concentration of 2 × IC50conjugated, and PPA–G4.5–PEG conjugates at the equivalent concentration of CPT–G4.5–PEG conjugates for various lengths of time (6, 12, and 24 h). | |
Overall, the current work illustrated the therapeutic activity of dendrimer-based CPT prodrug synthesized via click chemistry and it may exert long-lasting effect through slow release. The presence of a targeting moiety on the surface of a dendrimer molecule whose receptors are overexpressed on cancer cell membrane surface will enable the delivery of PAMAM dendrimer–drug conjugates to the cell of the interest and improve drug uptake. The synthesized dendrimer-based CPT prodrug can be further functionalized for targeted therapy of cancer such as glioblastoma multiforme (GBM). GBM is an aggressive form of brain cancer with poor prognosis and a median survival of only 12–15 months. In general, GBMs are resistant to standard treatment consisting of surgery followed by concurrent chemo- and radiotherapy.32 New therapeutic approaches such as the use of small molecule radiosensitizers and gene therapy are under investigation for the treatment of GBM.33,34 However, treatment outcomes still depend largely on whether or not sufficient levels of therapeutic agent can be delivered to the brain tumor mass.35 Considering that the blood-brain barrier (BBB), the blood cerebral spinal fluid, and the blood-tumor barrier hamper the administration of the therapeutic to the brain, efficient delivery still remains a challenge, and new technologies and delivery systems need to be developed.36,37 Despite that a number of carriers have been developed to facilitate drug entry into the brain,32,35,37–39 dendrimers appear to be a suitable platform to deliver drugs along with BBB-specific ligands because of their multivalency. In future studies, coupling tumor- and BBB-specific ligands to the synthesized dendrimer–CPT conjugates via click chemistry will be explored for GBM.
Conclusions
CPT was coupled to PEGylated PAMAM dendrimer G4.5 via copper-catalyzed click reaction. Nearly 100% of CPT molecules used in the reaction were covalently conjugated to the dendrimer. The resulting conjugates carried an average of 17 CPT molecules per dendrimer and demonstrated dose-dependent toxicity against human glioma U1242 cells by causing an arrest in G2/M and inducing cell death while the carrier itself had no toxicity. Conjugated CPT was found to have an IC50 of 5 µM, a 185-fold increase in comparison to the IC50 of free CPT as a result of slow release. The further utility of click chemistry based coupling strategy would make it possible to make large-scale high quality polymer–drug–ligand conjugates possessing a uniform loading of ligand and drug on the dendrimer surface, a critical factor for achieving reproducible antitumor efficacy.
Acknowledgements
This work was supported, in part, by the National Science Foundation (CAREER award CBET0954957), National Institutes of Health (R01EY024072, R01NS064593, and R21CA156995), and Virginia Commonwealth University Massey Cancer Center (multi-investigator award 2013-MIP-01).
References
- D. A. Tomalia, H. Baker, J. Dewald, M. Hall, G. Kallos, S. Martin, J. Roeck, J. Ryder and P. Smith, A new class of polymers: starburst-dendritic macromolecules, Polym. J., 1985, 17(1), 117–132 CrossRef CAS.
- D. A. Tomalia, Birth of a new macromolecular architecture: dendrimers as quantized building blocks for nanoscale synthetic polymer chemistry, Prog. Polym. Sci., 2005, 30(3–4), 294–324 CrossRef CAS PubMed.
- A. R. Menjoge, R. M. Kannan and D. A. Tomalia, Dendrimer-based drug and imaging conjugates: design considerations for nanomedical applications, Drug Discovery Today, 2010, 15(5–6), 171–185 CrossRef CAS PubMed.
- H. Yang and J. W. Kao, Synthesis and characterization of nanoscale dendritic RGD clusters for potential applications in tissue engineering and drug delivery, Int. J. Nanomed., 2007, 2(1), 89–99 CrossRef CAS.
- H. Yang and S. T. Lopina, Penicillin V-conjugated PEG-PAMAM star polymers, J. Biomater. Sci., Polym. Ed., 2003, 14(10), 1043–1056 CrossRef CAS PubMed.
- H. Yang and S. T. Lopina, Extended release of a novel antidepressant, venlafaxine, based on anionic polyamidoamine dendrimers and poly(ethylene glycol)-containing semi-interpenetrating networks, J. Biomed. Mater. Res., Part A, 2005, 72(1), 107–114 CrossRef PubMed.
- H. Yang, J. J. Morris and S. T. Lopina, Polyethylene glycol-polyamidoamine dendritic micelle as solubility enhancer and the effect of the length of polyethylene glycol arms on the solubility of pyrene in water, J. Colloid Interface Sci., 2004, 273(1), 148–154 CrossRef CAS PubMed.
- S. Gurdag, J. Khandare, S. Stapels, L. H. Matherly and R. M. Kannan, Activity of dendrimer-methotrexate conjugates on methotrexate-sensitive and resistant cell lines, Bioconjugate Chem., 2006, 17(2), 275–283 CrossRef CAS PubMed.
- B. Huang, J. F. Kukowska-Latallo, S. Tang, H. Zong, K. B. Johnson, A. Desai, C. L. Gordon, P. R. Leroueil and J. R. Baker Jr, The facile synthesis of multifunctional PAMAM dendrimer conjugates through copper-free click chemistry, Bioorg. Med. Chem. Lett., 2012, 22(9), 3152–3156 CrossRef CAS PubMed.
- I. J. Majoros, A. Myc, T. Thomas, C. B. Mehta and J. R. Baker Jr, PAMAM dendrimer-based multifunctional conjugate for cancer therapy: synthesis, characterization, and functionality, Biomacromolecules, 2006, 7(2), 572–579 CrossRef CAS PubMed.
- S. Zhu, M. Hong, L. Zhang, G. Tang, Y. Jiang and Y. Pei, PEGylated PAMAM dendrimer-doxorubicin conjugates: in vitro evaluation and in vivo tumor accumulation, Pharm. Res., 2010, 27(1), 161–174 CrossRef CAS PubMed.
- R. Huang, W. Ke, L. Han, Y. Liu, K. Shao, L. Ye, J. Lou, C. Jiang and Y. Pei, Brain-targeting mechanisms of lactoferrin-modified DNA-loaded nanoparticles, J. Cereb. Blood Flow Metab., 2009, 29(12), 1914–1923 CrossRef CAS PubMed.
- S. Kannan, H. Dai, R. S. Navath, B. Balakrishnan, A. Jyoti, J. Janisse, R. Romero and R. M. Kannan, Dendrimer-based postnatal therapy for neuroinflammation and cerebral palsy in a rabbit model, Sci. Transl. Med., 2012, 4(130), 130ra46 Search PubMed.
- Q. Yuan, Y. Fu, W. J. Kao, D. Janigro and H. Yang, Transbuccal Delivery of CNS Therapeutic Nanoparticles: Synthesis, Characterization, and In Vitro Permeation Studies, ACS Chem. Neurosci., 2011, 2(11), 676–683 CrossRef CAS PubMed.
- X. Y. Shi, I. J. Majoros, A. K. Patri, X. D. Bi, M. T. Islam, A. Desai, T. R. Ganser and J. R. Baker, Molecular heterogeneity analysis of poly(amidoamine) dendrimer-based mono- and multifunctional nanodevices by capillary electrophoresis, Analyst, 2006, 131(3), 374–381 RSC.
- L. F. Liu, DNA Topoisomerase Poisons as Antitumor Drugs, Annu. Rev. Biochem., 1989, 58(1), 351–375 CrossRef CAS PubMed.
- V. J. Venditto and E. E. Simanek, Cancer therapies utilizing the camptothecins: a review of the in vivo literature, Mol. Pharmaceutics, 2010, 7, 307–349 CrossRef CAS PubMed.
- F. Cheng, J. Liu, C. Teh, S. W. Chong, V. Korzh, Y. J. Jiang and L. W. Deng, Camptothecin-induced downregulation of MLL5 contributes to the activation of tumor suppressor p53, Oncogene, 2011, 30(33), 3599–3611 CrossRef CAS PubMed.
- L. F. Liu, S. D. Desai, T.-K. Li, Y. Mao, M. E. I. Sun and S.-P. Sim, Mechanism of Action of Camptothecin, Ann. N. Y. Acad. Sci., 2000, 922(1), 1–10 CrossRef CAS PubMed.
- K. M. Schultz, L. Campo-Deano, A. D. Baldwin, K. L. Kiick, C. Clasen and E. M. Furst, Electrospinning covalently cross-linking biocompatible hydrogelators, Polymer, 2013, 54(1), 363–371 CrossRef CAS PubMed.
- A. Dal Pozzo, M. H. Ni, E. Esposito, S. Dallavalle, L. Musso, A. Bargiotti, C. Pisano, L. Vesci, F. Bucci, M. Castorina, R. Fodera, G. Giannini, C. Aulicino and S. Penco, Novel tumor-targeted RGD peptide-camptothecin conjugates: synthesis and biological evaluation, Bioorg. Med. Chem., 2010, 18(1), 64–72 CrossRef CAS PubMed.
- O. Y. Zolotarskaya, A. F. Wagner, J. M. Beckta, K. Valerie, K. J. Wynne and H. Yang, Synthesis of Water-Soluble Camptothecin-Polyoxetane Conjugates via Click Chemistry, Mol. Pharm., 2012, 9(11), 3403–3408 CrossRef CAS PubMed.
- G. Thiagarajan, A. Ray, A. Malugin and H. Ghandehari, PAMAM-Camptothecin Conjugate Inhibits Proliferation and Induces Nuclear Fragmentation in Colorectal Carcinoma Cells, Pharm. Res., 2010, 27(11), 2307–2316 CrossRef CAS PubMed.
- E. Lallana, A. Sousa-Herves, F. Fernandez-Trillo, R. Riguera and E. Fernandez-Megia, Click Chemistry for Drug Delivery Nanosystems, Pharm. Res., 2012, 29(1), 1–34 CrossRef CAS PubMed.
- B. Huang, A. Desai, H. Zong, S. Tang, P. Leroueil and J. R. Baker Jr, Copper-free click conjugation of methotrexate to a PAMAM dendrimer platform, Tetrahedron Lett., 2011, 52(13), 1411–1414 CrossRef CAS PubMed.
- S. E. Golding, E. Rosenberg, B. R. Adams, S. Wignarajah, J. M. Beckta, M. J. O'Connor and K. Valerie, Dynamic inhibition of ATM kinase provides a strategy for glioblastoma multiforme radiosensitization and growth control, Cell Cycle, 2012, 11(6), 1167–1173 CrossRef CAS PubMed.
- Q. Yuan, W. A. Yeudall and H. Yang, PEGylated Polyamidoamine Dendrimers with Bis-Aryl Hydrazone Linkages for Enhanced Gene Delivery, Biomacromolecules, 2010, 11(8), 1940–1947 CrossRef CAS PubMed.
- R. Jevprasesphant, J. Penny, R. Jalal, D. Attwood, N. B. McKeown and A. D'Emanuele, The influence of surface modification on the cytotoxicity of PAMAM dendrimers, Int. J. Pharm., 2003, 252(1–2), 263–266 CrossRef CAS.
- H. Yang, S. T. Lopina, L. P. DiPersio and S. P. Schmidt, Stealth dendrimers for drug delivery: correlation between PEGylation, cytocompatibility, and drug payload, J. Mater. Sci.: Mater. Med., 2008, 19(5), 1991–1997 CrossRef CAS PubMed.
- H. Yang and S. T. Lopina, In vitro enzymatic stability of dendritic peptides, J. Biomed. Mater. Res., Part A, 2006, 76(2), 398–407 CrossRef PubMed.
- B. L. Staker, K. Hjerrild, M. D. Feese, C. A. Behnke, A. B. Burgin and L. Stewart, The mechanism of topoisomerase I poisoning by a camptothecin analog, Proc. Natl. Acad. Sci. U. S. A., 2002, 99(24), 15387–15392 CrossRef CAS PubMed.
- L. Biddlestone-Thorpe, N. Marchi, K. Guo, C. Ghosh, D. Janigro, K. Valerie and H. Yang, Nanomaterial-mediated CNS delivery of diagnostic and therapeutic agents, Adv. Drug Delivery Rev., 2012, 64(7), 605–613 CrossRef CAS PubMed.
- L. Biddlestone-Thorpe, M. Sajjad, E. Rosenberg, J. M. Beckta, N. C. K. Valerie, M. Tokarz, B. R. Adams, A. F. Wagner, A. Khalil, D. Gilfor, S. E. Golding, S. Deb, D. G. Temesi, A. Lau, M. J. O'Connor, K. S. Choe, L. F. Parada, S. K. Lim, N. D. Mukhopadhyay and K. Valerie, ATM Kinase Inhibition Preferentially Sensitizes p53-Mutant Glioma to Ionizing Radiation, Clin. Cancer Res., 2013, 19(12), 3189–3200 CrossRef CAS PubMed.
- S. E. Golding, E. Rosenberg, N. Valerie, I. Hussaini, M. Frigerio, X. F. Cockcroft, W. Y. Chong, M. Hummersone, L. Rigoreau, K. A. Menear, M. J. O'Connor, L. F. Povirk, T. van Meter and K. Valerie, Improved ATM kinase inhibitor KU-60019 radiosensitizes glioma cells, compromises insulin, AKT and ERK prosurvival signaling, and inhibits migration and invasion, Mol. Cancer Ther., 2009, 8(10), 2894–2902 CrossRef CAS PubMed.
- H. Yang, Nanoparticle-mediated brain-specific drug delivery, imaging, and diagnosis, Pharm. Res., 2010, 27(9), 1759–1771 CrossRef CAS PubMed.
- M. S. Lesniak and H. Brem, Targeted therapy for brain tumours, Nat. Rev. Drug Discovery, 2004, 3(6), 499–508 CrossRef CAS PubMed.
- G. H. Huynh, D. F. Deen and F. C. Szoka, Barriers to carrier mediated drug and gene delivery to brain tumors, J. Controlled Release, 2006, 110(2), 236–259 CrossRef CAS PubMed.
- J. Kreuter, Drug delivery to the central nervous system by polymeric nanoparticles: What do we know?, Adv. Drug Delivery Rev., 2014, 71, 2–14 CrossRef CAS PubMed.
- W. M. Pardridge, shRNA and siRNA delivery to the brain, Adv. Drug Delivery Rev., 2007, 59(2–3), 141–152 CrossRef CAS PubMed.
Footnotes |
† Electronic supplementary information (ESI) available. See DOI: 10.1039/c5ra07987j |
‡ The authors contributed equally to the work. |
|
This journal is © The Royal Society of Chemistry 2015 |
Click here to see how this site uses Cookies. View our privacy policy here.