DOI:
10.1039/C5FO00928F
(Paper)
Food Funct., 2016,
7, 540-548
Hydroxytyrosol decreases the oxidative and nitrosative stress levels and promotes angiogenesis through HIF-1 independent mechanisms in renal hypoxic cells
Received
29th July 2015
, Accepted 5th November 2015
First published on 10th November 2015
Abstract
In the kidney, tissue oxygen tension is comparatively low and this renders this organ more prone to hypoxic injury. In fact, hypoxia has a central role in the development and progression of renal disease. The recovery from this situation is dependent on the degree to which sublethally damaged cells restore normal function. The master regulator of the hypoxic response is hypoxia-inducible factor-1 (HIF-1). HIF-1 activity depends on the HIF-1α subunit level which is regulated by oxygen, nitric oxide (NO), reactive oxygen species and mTOR. Given the antioxidant and antinitrosative properties ascribed to hydroxytyrosol (HT), this study evaluates the impact of this olive oil polyphenol on the response to hypoxia in kidney cells. For this purpose, the human embryonic kidney HEK293T cell line was treated with HT and cultured under sublethal hypoxic conditions. Our results demonstrate that HT treatment decreases both, post-hypoxic reactive oxygen species and NO levels and, consequently, HIF-1α accumulation. However, HT does not affect mTOR activation or the factor inhibiting HIF level but promotes the expression of angiogenic proteins, suggesting that HT activates an adaptive response to hypoxia in a HIF-1α-independent pathway. In fact, this effect could be ascribed to the up-regulation of estrogen-related receptor α. In conclusion, our results suggest that in renal hypoxia, HT treatment might act as an effective preventive therapeutic approach to decrease stress and to improve the adaptive response to this pathological situation.
1. Introduction
The reduction of oxygen tension (hypoxia) is associated with a wide range of highly prevalent pathologies such as pulmonary and cardiovascular diseases, diabetic complications, stroke, cancer or inflammation. Particularly in the kidney, tissue oxygen tension is comparatively low and this renders this organ prone to hypoxic injury. This hypoxia susceptibility has a central role in the development and progression of renal vascular disease, acute kidney injury or chronic renal disease and can negatively influence the short- and long-term outcomes of kidney transplantation.1 Among the complex metabolic reactions occurring during hypoxia, many are related to the formation of reactive oxygen (ROS) and nitrogen (RNS) species. Uncontrolled increases in the concentration of these oxidants play a significant role in the genesis of kidney hypoxic injury. According to the severity of the insult, hypoxic injury can be lethal or sublethal to kidney cells. However, the nature of the recovery response is dependent on the degree to which sublethally damaged cells can restore normal function and promote regeneration.2
The master regulator of the hypoxic response is the hypoxia-inducible factor (HIF). Three different HIF factors have been identified, HIF-1 being the key mediator of hypoxic signalling in renal epithelial cells.3 HIF-1 is a heterodimeric DNA-binding complex composed of α and β subunits. Because HIF-1β is constitutively expressed, HIF-1 activity depends on the HIF-1α subunit level. Although transcriptional, post-transcriptional and translational mechanisms of regulation have been described, there is no doubt that HIF-1α levels are mainly regulated by post-translational mechanisms, particularly by hydroxylation. Under normoxic conditions, HIF-1α is hydroxylated in Pro-402 and/or Pro-564 by HIF prolyl hydroxylases (PHDs). This post-translational modification triggers protein degradation via the ubiquitin–proteasome system.4 Because PHDs require oxygen, the reduced oxygen availability under hypoxic conditions inhibits their activity, increasing the HIF-1α half-life. PHD activity can also be affected by ROS and nitric oxide (NO), which promote PHD inactivation and HIF-1α accumulation.5 Moreover, HIF-1α can be regulated independently of PHDs by the phosphatidylinositol-3-kinase/AKT/mammalian target of rapamycin (PI3K/AKT/mTOR) pathway.6 mTOR is a serine/threonine protein kinase that phosphorylates p70 ribosomal protein S6 kinase 1 and eIF-4E binding protein 1, thereby stimulating HIF-1α protein synthesis. Once stabilized, HIF-1α translocates into the nucleus, dimerizes with HIF-1β and forms the functional HIF-1 transcription factor which binds to hypoxia-response elements (HRE), inducing the expression of over a hundred genes involved in the adaptive response to hypoxia. HIF-1 transcriptional activity is also regulated in an oxygen dependent way by the hydroxylation of Asn-803 in the C-terminal domain. This reaction, catalyzed by the factor inhibiting HIF (FIH), avoids its interaction with the CBP/p300 coactivator and inhibits HIF-1 transcriptional activity.7
The Mediterranean diet has been traditionally associated with an improved cardiovascular risk profile. Cardiovascular risk factors impair renal function8 inducing tissue hypoxia. In this sense, recent reports demonstrate that adherence to a Mediterranean diet slows kidney function decline9,10 and, in fact, this diet has been proposed as a viable alternative to a low-protein diet in nephropathic patients.11 Olive oil is the main source of fat in the Mediterranean diet. Although the beneficial effects of olive oil had been traditionally attributed to its high content of monounsaturated fatty acids, much attention has recently been paid to olive oil minor components. The most representative of those components are phenolic compounds, particularly, hydroxytyrosol (3,4-dihydroxyphenylethanol; HT). HT penetrates easily into cells by a passive diffusion mechanism12 and has been proven to be a potent antioxidant able to scavenge oxygen and nitrogen free radicals.13,14
Given the importance of hypoxia and oxidative/nitrosative stress in renal diseases, the key role of both types of stress in HIF-1 regulation, and the antioxidant effect of HT, we propose to evaluate whether this polyphenol is able to improve the adaptive response to a sublethal hypoxic injury in kidney cells. The results obtained will help to develop new therapeutic interventions against hypoxia in order to decrease the morbidity associated with renal pathologies.
2. Materials and methods
2.1. Cell culture and treatments
Human embryonic kidney HEK293T cells were grown in 10% foetal bovine serum supplemented Dulbecco's modified Eagle's medium (FBS-DMEM, BiochromAG, Berlin, Germany) at 37 °C in 5% CO2 and 21% O2. Cells were pre-treated or not with HT (Extrasynthese, Lyon, France), dissolved in ethanol immediately before use, for 12 h under normoxic conditions (21% O2). At the end of the incubation time, cells were further cultured for 4 h either under normoxic (21% O2) or hypoxic conditions (1% O2). Controls were treated with an equal concentration of ethanol.
2.2. Cytotoxicity assay
The cytotoxic effect of HT was evaluated using the sulforhodamine B (SRB) assay as previously described.15 Briefly, 4 × 104 cells per well were plated into 24-well tissue culture plates (Nunc, Rosbilde, Denmark). The plates were incubated for 24 h to allow the cells to adhere. HT was then added to the corresponding wells at a range of concentrations (100, 200, 400, 600 and 800 μM), each concentration being used in at least four replicate wells. After 16 h of treatment, the medium was removed and the cultures were washed with PBS. Cells were fixed at 4 °C with 10% trichloroacetic acid (TCA) for 30 min and then washed with tap water to remove TCA. Plates were air dried and stored until use. TCA-fixed cells were stained for 20 min with 0.4% (w/v) SRB (Sigma, St Louis, MO, USA) dissolved in 1% acetic acid. After staining, SRB was removed and cultures were rinsed with 1% acetic acid to eliminate the unbound dye. The cultures were air dried and the bound dye was solubilized with 10 mM Tris base (pH 10.5). Optical density was read on a plate reader at 492 nm. Cell survival was measured as the percentage of absorbance compared with that obtained in non-HT-treated cells.
In addition, the effect of HT and hypoxia on cell viability was also assessed by staining with propidium iodide (PI) and by flow cytometric analysis.
2.3. Measurement of intracellular generation of ROS
Intracellular generation of ROS was analyzed using 2′,7′-dichlorofluorescein diacetate (DCFH-DA; Sigma, St Louis, MO, USA) as a probe.16 ROS in the cells oxidize DCFH, yielding highly fluorescent 2′,7′-dichlorofluorescein (DCF). Briefly, cells were cultured in 24-well plates (5 × 105 cells per well) and treated for 16 h with HT (100 or 200 μM), being cultured during the last 4 h either under normoxic or hypoxic conditions. 30 min before the end of the experiment, cells were washed with Krebs buffer (pH 7.3) and incubated with 10 μM DCFH-DA. Once the incubation was complete, cells were washed three times and DCF fluorescence was measured on a microplate reader (ThermoLabSystem Multiscan Ascent) at an excitation wavelength of 488 nm and an emission wavelength of 535 nm.
2.4. NO measurement
Nitric oxide (NO) production was indirectly quantified by determining nitrate/nitrite and S-nitroso compounds (NOx), using an ozone chemiluminescence-based method. To estimate the NOx level, cells of each experimental condition were collected and lysed by 3 freeze–thaw cycles. After centrifugation at 14
000g for 30 min, the supernatants were collected and protein was quantified.17 Samples were deproteinized in a deproteinization solution (0.8 N NaOH and 16% ZnSO4). The total amount of NOx in the deproteinized samples was determined by a modification of the procedure described by Braman and Hendrix using the purge system of Sievers Instruments, model NOA 280i.18 NOx concentrations were calculated by comparison with standard solutions of sodium nitrate. Final NOx values were referred to the total protein concentration in the initial extracts.
2.5. Western blotting
For western blot analysis, equal amounts of denatured total-protein extracts (60 μg for HIF-1α and p-mTOR; 30 μg for nitrotyrosine and FIH) were loaded and separated in 7.5% (HIF-1α and p-mTOR), 4–12% (nitrotyrosine) or 10% (FIH) SDS-polyacrylamide gel. Proteins in the gel were transferred to a PVDF membrane (Amersham Pharmacia Biotech., NJ, USA) and then blocked. Polyclonal antibodies to HIF-1α (1/1000, Bethyl Lab, Inc., Montgomery, USA), nitrotyrosine (1/2000, produced by our group), FIH (1/1000; Santa Cruz Biotechnology Inc., Santa Cruz, USA) and p-mTOR (1/1000; Cell Signalling, Massachusetts, USA) and a monoclonal antibody to α-tubulin (Sigma, St Louis, MO, USA), as the internal control, were used for the detection of the respective proteins. Antibody reaction was revealed by chemiluminescence detection procedures according to the manufacturer's recommendations (ECL kit, Amersham Corp., Buckinghamshire, UK).
2.6. Q RT-PCR
Gene expression of AM, VEGF and estrogen-related receptor α (ERRα) were quantitatively assessed by real-time PCR using peptidylprolylisomerase A (PPIA) as the normalizing gene. RNA was isolated using the RNeasyPlus Mini kit (Qiagen, Hilden, Germany) and cDNA was prepared using a Maxima First Strand cDNA Synthesis kit for RT-qPCR (Fermentas Intl., Vilnius, Lithuania). Real-time PCR was performed on a MxPro thermal cycler (Stratagene, California, USA) using SYBR Fast Master Mix (2×) Universal (KAPABiosystems, Massachusetts, USA) with the following gene-specific primers: AM, CTCTGAGTCGTGGGAAGAGG (F) and CCCTGGAAGTTGTTCATGCT (R); VEGF, TTGTACAAGATCCGCAGACG (F) and TCACATCTGCAAGTACGTTCG (R); ERRα, GCTGCCCTGCTGCAACTA (F), GCCTCGTGCAGAGCTTCTC (R); PPIA, TTCATCTGCACTGCCAAGAC (F), TCGAGTTGTCCACAGTCAGC (R). Experiments were performed in triplicate, and the relative quantities of target genes, corrected with the normalizing gene PPIA, were calculated using the Stratagene MxProTM QPCR software.
2.7. Statistical analysis
Data are expressed as means ± SD. Statistical comparisons between the different experimental groups and their corresponding controls were made using Student's t-test, considering p < 0.05 as the level of significance.
3. Results
3.1. HT toxicity in HEK293T cells
The toxicity of HT in HEK293T cells after a 16 h incubation period is shown in Fig. 1. The results obtained indicate that treatment with up to 600 μM HT does not affect cell growth. However, the highest concentration tested (800 μM) exerts a significant toxic effect (p < 0.05). Hence, we decided to use 100 and 200 μM HT, concentrations devoid of any negative effect on cell viability.
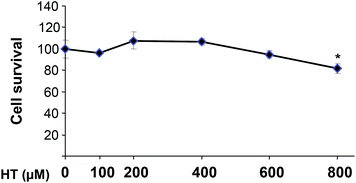 |
| Fig. 1 Effect of HT on HEK293T cell survival. Values represent the mean ± SD from three independent experiments. Statistically significant differences with the corresponding non-treated cells: *p < 0.05. | |
3.2. Hypoxic treatment induces HIF-1α without affecting cell viability
Sublethal injury is crucial in restoring renal function after a hypoxic episode. Therefore, we next evaluated whether a 4 h hypoxia insult was able to induce a molecular response without affecting cell viability. As shown in Fig. 2, the assayed hypoxic conditions induced the HIF-1α response without affecting cell viability. Moreover, the absence of toxicity of the treatment with 100 and 200 μM HT in hypoxia was also corroborated by PI staining (Fig. 2B), in both normoxia and hypoxia.
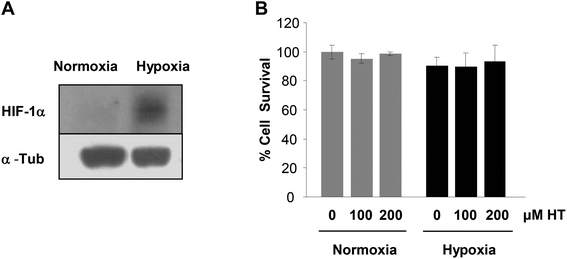 |
| Fig. 2 Development of a hypoxic model of sublethal damage. (A) Effect of the experimental hypoxic model on HIF-α induction. Representative immunoblot of HIF-1α expression in normoxic and hypoxic cells. α-Tubulin (α-Tub) immunodetection was included as a protein-loading control. (B) Effect of the experimental hypoxic model and HT treatment (100 and 200 μM) on cell viability measured by PI staining. Values represent the mean ± SD from three independent experiments. | |
3.3. Effect of HT on post-hypoxic NO production and ROS levels
Most of the HT effects are usually attributed to an antioxidant effect. The analysis of ROS production by DCFH (Fig. 3A) indicates that HT treatment did not affect the oxidative stress level in normoxia, whereas it decreased hypoxic ROS burst.
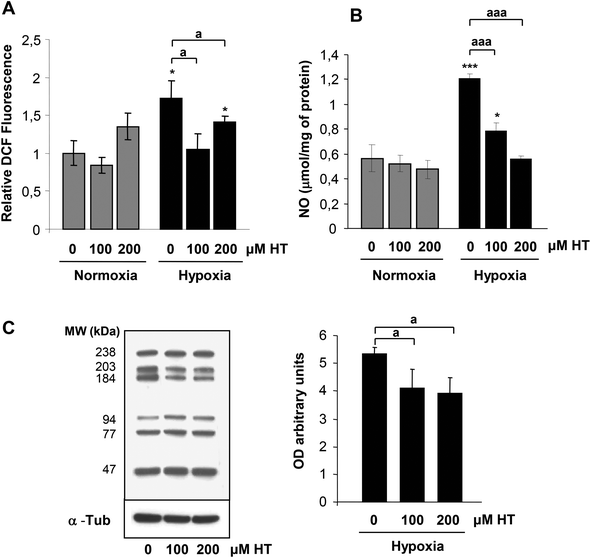 |
| Fig. 3 (A) Effect of HT treatment and hypoxia on the oxidative stress level measured by DCF fluorescence. (B) Effect of HT treatment and hypoxia on NO levels (μmol per mg of protein). (C) Effect of HT treatment on the hypoxic nitrotyrosine level. Values represent the mean ± SD from three independent experiments. Statistically significant differences with the corresponding non-treated normoxic cells: *p < 0.05, ***p < 0.001. Statistically significant differences with the corresponding non-treated hypoxic cells: ap < 0.05, aaap < 0.001. | |
We then evaluated whether HT treatment (100 and 200 μM) affected NO levels (Fig. 3B). HT did not modify NO levels in normoxia. However, the increase in NO observed in hypoxic cells was significantly reduced by 100 μM HT and completely abolished by 200 μM HT.
As a whole, these results support that this polyphenol protects from oxidative and nitrosative stress under hypoxic conditions. In fact, the decreased levels of bulk-nitrated proteins observed in hypoxic HT-treated cells corroborate this hypothesis (Fig. 3C).
3.4. Effect of HT treatment on HIF-1α expression
NO and ROS have been previously implicated in HIF-1α stabilization. The difference in the oxidative and nitrosative stress levels observed in HT treated cells prompted us to determine whether HIF-1α expression was influenced by HT. As shown in Fig. 4, hypoxic HIF-1α induction is abolished by HT treatment.
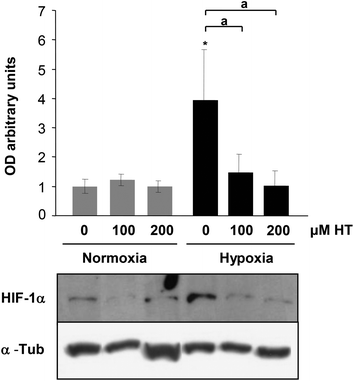 |
| Fig. 4 Effect of HT on HIF-1α stabilization. Representative immunoblot of HIF-1α expression in normoxic and hypoxic cells. α-Tubulin (α-Tub) immunodetection was included as a protein-loading control. Values represent the mean ± SD relative to α-Tub from three independent experiments. Statistically significant differences with the corresponding non-treated normoxic cells: *p < 0.05. Statistically significant differences with the corresponding non-treated hypoxic cells: ap < 0.05. | |
3.5. Effect of HT treatment on mTOR activation
The activation of the PI3K/AKT/mTOR pathway plays an important role in kidney disease progression and is also involved in HIF-1α expression. We then determined whether the phosphorylation of mTOR was modified by HT. Our results (Fig. 5) did not indicate any effect of HT on mTOR activation, suggesting that the PI3K/mTOR pathway is not responsible for the differences observed in HIF-1 expression.
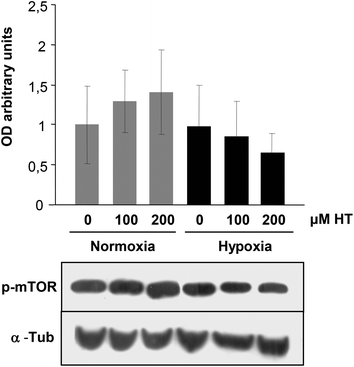 |
| Fig. 5 Western-blot analysis of the effect of HT on p-mTOR levels. Representative immunoblot of p-mTOR expression in normoxic and hypoxic cells. α-Tubulin (α-Tub) immunodetection was included as a protein-loading control. Values represent the mean ± SD relative to α-Tub from three independent experiments. | |
3.6. Effect of HT treatment on angiogenic HIF-1 alpha target genes
We next assessed the expression of two angiogenic HIF-1α target genes (AM and VEGF; Fig. 6). Strikingly, both under normoxic and hypoxic conditions, HT treatment significantly increased the mRNA levels of these two genes.
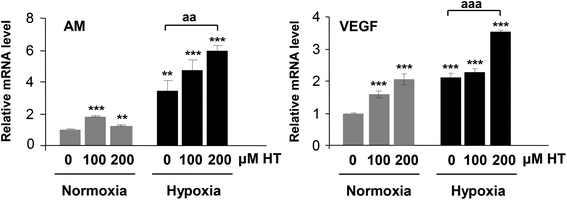 |
| Fig. 6 Effect of HT treatment on the mRNA expression of AM and VEGF. The results are expressed as mRNA expression relative to normoxic non-HT-treated cells after normalization against PPIA. Values represent the mean ± SD from three independent experiments. Statistically significant differences with the corresponding non-treated normoxic cells: **p < 0.01, ***p < 0.001. Statistically significant differences with the corresponding non-treated hypoxic cells: aap < 0.01, aaap < 0.001. | |
3.7. Effect of HT treatment on FIH expression
HIF-1 transcriptional activity is inhibited by FIH. We therefore analyzed the effect of HT treatment on FIH levels. The western-blot results (Fig. 7) demonstrate that the expression of this protein remained invariable in all the experimental groups. The fact that FIH levels are not affected by HT suggests the existence of HIF-1 independent mechanisms of regulation involved in the up-regulation of these angiogenic genes.
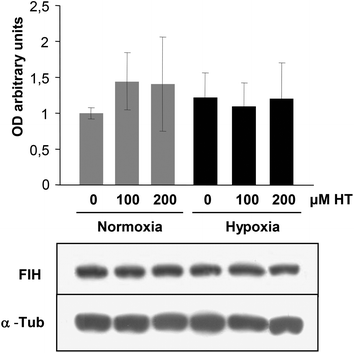 |
| Fig. 7 Western-blot analysis of the effect of HT on FIH levels. Representative immunoblot of FIH expression in normoxic and hypoxic cells. α-Tubulin (α-Tub) immunodetection was included as a protein-loading control. Values represent the mean ± SD relative to α-Tub from three independent experiments. | |
3.8. Effect of HT treatment on ERRα expression
Angiogenic genes also respond to ERRα. In an attempt to explain the HIF-1 independent increase in these genes, we next analyzed the mRNA level of this protein. As shown in Fig. 8, HT treatment but not hypoxia induced the expression of ERRα similar to the reported effect of this polyphenol on the mRNA expression of angiogenic genes.
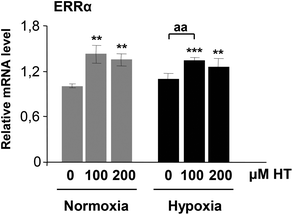 |
| Fig. 8 Effect of HT on the transcription of ERRα. The results are expressed as mRNA expression relative to normoxic non-HT-treated cells after normalization against PPIA. Values represent the mean ± SD from three independent experiments. Statistically significant differences with the corresponding non-treated normoxic cells: **p < 0.01, ***p < 0.001. Statistically significant differences with the corresponding non-treated hypoxic cells: aap < 0.01. | |
4. Discussion
Numerous studies have established the relationship between oxidative/nitrosative stress, hypoxia and renal dysfunction.2,19 Therefore, in recent years much effort has been dedicated to design treatments to reduce the stress level associated with hypoxia-related renal pathologies while improving recovery. HT, one of the main simple phenolic compounds in olive oil, has been described as a potent scavenger of oxygen and nitrogen free radicals;13,14 however, its effect in renal hypoxia has not been tested before, and let alone in a sublethal hypoxic model in which viable cells are able to trigger molecular pathways leading to an adaptive response. The present study demonstrates that HT treatment decreases hypoxic NO and ROS production also in renal cells. NO can be synthesized by the NO synthases (NOS). Although NO from endothelial NOS plays a protective role by maintaining endothelial cells in a quiescent state, high levels of NO, similar to those observed under our hypoxic conditions, are highly deleterious and promote an inflammatory status. High levels of NO are usually ascribed to the inducible NOS isoform (iNOS) and HT has been proposed to decrease the expression of this isoform through the impairment of NF-κB activation.20 Thus, the reduced NO level observed in hypoxic HT-treated cells may reflect a lower iNOS induction, and, consequently, our results support the protective role of HT treatment against nitrosative stress. HT has also been described to decrease hypoxic oxidative stress in liver and brain ischemic models.21–23 According to the literature, HT exerts its antioxidant effect by directly scavenging ROS or by inducing the activity of antioxidant enzymes such as those from the GSH system.24,25 In this sense, our results corroborate this effect in hypoxic renal cells, although further experiments should be performed to assess the particular mechanisms involved.
We next assessed the incidence of this decreased hypoxia-induced stress associated with HT treatment on the expression of HIF-1α, one of the master regulators of the adaptive response to hypoxia. It has been previously published that polyphenols from green tea26 or red propolis27 decrease HIF-1 accumulation. Our results are in agreement with those reports as, under a similar hypoxic stimulus, there is a lower HIF-1α induction in HT-treated cells. Both NO and ROS have been described to promote HIF-1α accumulation, although we and others have reported the predominant role of NO rather than ROS in HIF-1α increase.28 Consequently, it is plausible to assume that the decrease in both species associated with HT treatment could be responsible for the lower HIF-1α accumulation observed.
Apart from oxygen, NO and ROS, HIF-1α expression is also regulated by the PI3K/AKT/mTOR pathway.6 Moreover, it has been described that mTOR activity can be either activated or inhibited by ROS in a context-dependent manner. Previous data about the effect of HT on this pathway are controversial. In hepatic carcinoma cells this polyphenol has been described to inhibit Akt phosphorylation and mTOR activation.29 However, HT has also been shown to promote Akt phosphorylation.30,31 Our present findings in HEK293T provide evidence that HT does not affect mTOR activation either under normoxic or hypoxic conditions, suggesting that the PI3K/AKT/mTOR pathway is not responsible for the changes in HIF-1α expression and that the effect of HT may vary according to the experimental model studied.
Taking into account the fact that HIF-1 is the key mediator of hypoxic HIF signalling in renal epithelial cells, we have also analyzed the effect of HT on the transcriptional activity of HIF-1 by evaluating the expression of two of its typical target genes, AM and VEGF. In our experimental model, HT treatment induced greater mRNA levels of these genes under normoxic and hypoxic conditions. AM and VEGF are key angiogenic factors that induce the formation of new blood vessels from the pre-existing ones favouring local blood supply. Therefore, angiogenic factors are cytoprotective in hypoxic renal injury. Moreover, AM suppresses inflammatory cytokines and apoptosis, and has been proposed to ameliorate renal injury via the suppression of NADPH oxidase and the renin–angiotensin system.32 In fact, AM levels are highly sensitive markers of kidney disease and could be used as indicators for its prognosis.33 VEGF is also a pluripotent peptide with cytoprotective and antiapoptotic effects which has been proven to be beneficial in ischemia-induced renal failure.34 Therefore, the induction of these proteins by HT treatment should be considered a beneficial effect to counteract hypoxia. Previous literature about the effect of HT on angiogenesis is scarce and point to an antiangiogenic effect of this polyphenol.29,35 As none of those studies were performed in renal cells, it should be inferred that the effect of HT is greatly dependent on the cell type and experimental conditions. The only hypoxic model in which this effect has been studied was carried out in retina cells using the hypoxia-mimicking agent cobalt chloride (CoCl2) with a subsequent HT-treatment.35 Apart from inducing HIF-1, CoCl2 affects other important enzymes and pathways, hence its effects do not strictly reproduce those of a hypoxic situation. On the contrary, in our study, we have used a hypoxic model of sublethal damage in renal cells with a previous treatment of HT. As a result, we have been able to evaluate the effect of a preventive intervention in cells that can trigger an adaptive response, which could explain the disagreement between the results.
However, the increase in VEGF and AM could not be attributed to a higher HIF-1α accumulation, because the levels of this protein were lower in hypoxic HT-treated cells than in hypoxic non-treated cells. HIF-1 transcriptional activity is regulated by FIH.7 Therefore, we speculated that a lower expression of FIH could account for the higher transcriptional activity of HIF-1 observed in HT-treated cells. However, no effect of HT on the FIH level was detected, suggesting that HT induces the expression of AM and VEGF through HIF-1 independent mechanisms.
A HIF-1 independent mechanism of inducing angiogenesis involves the estrogen-related receptor (ERR) pathway.36,37 ERRs are a family of constitutively active orphan nuclear hormone receptors that dot not require activation with any agonist.38 Particularly, ERRα, one of the first identified orphan nuclear receptors, is expressed in most tissues at moderate to high levels and, particularly, in organs with high metabolic needs such as kidneys.39 ERRα recognizes the consensus DNA sequence AAGGTCA. This ERRα response element was initially described in several genes regulating cellular energy metabolism.40 Moreover, Arany et al. also described the existence of these ERRα-sites both in the promoter and in a conserved enhancer in the first intron of the VEGF gene.36 Since then, a number of studies have demonstrated the pro-angiogenic role of ERRα through the direct transcriptional regulation of angiogenic genes.41–43 Our results demonstrate that ERRα expression is not affected by hypoxia. Nevertheless, HT-treatment induces a significant increase in ERRα mRNA both under normoxic and hypoxic conditions, which could explain the higher level of angiogenic genes described above. The potential estrogenic effect of extra virgin olive oil polyphenols has been recently suggested in the literature43,44 but, to our knowledge, this is the first report that supports the induction of ERRα in response to HT.
5. Conclusion
HT treatment significantly decreased the nitrosative and oxidative stress levels associated with renal hypoxia. This effect resulted in a lower HIF-1α accumulation although, unexpectedly, an increase in the response of the angiogenic genes was observed. We propose that this pro-angiogenic action of HT is mediated by a HIF-1 independent mechanism that involves the up-regulation of ERRα (Fig. 9). Although further in vivo experiments should be performed, these findings suggest that HT may be a plausible preventive therapeutic approach to improve the adaptive response to renal hypoxia.
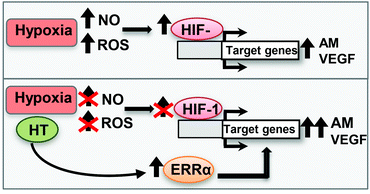 |
| Fig. 9 Proposed model of action of HT-treatment in the hypoxic response of sublethally damaged renal cells. In the absence of HT, hypoxia increases both NO and ROS levels, promoting HIF-1α accumulation and HIF-1 transcriptional activity. HT-treatment decreases hypoxia-associated stress increase, inhibiting HIF-1α post-hypoxic accumulation. However, its effect on ERRα expression further enhances an angiogenic response. | |
Acknowledgements
This study was supported by the Junta de Andalucía (BIO-184). Technical and human (Ana Jiménez and Ricardo Oya) support provided by CICT of Universidad de Jaén (UJA, MINECO, Junta de Andalucía, FEDER) is gratefully acknowledged.
References
- K. U. Eckardt, C. Rosenberger, J. S. Jürgensen and M. S. Wiesener, Role of hypoxia in the pathogenesis of renal disease, Blood Purif., 2003, 21, 253–257 CrossRef PubMed.
- D. P. Basile, M. D. Anderson and T. A. Sutton, Pathophysiology of acute kidney injury, Compr. Physiol., 2012, 2, 1303–1353 Search PubMed.
- V. H. Haase, Hypoxia-inducible factors in the kidney, Am. J. Physiol. Renal Physiol., 2006, 291, F271–F281 CrossRef CAS PubMed.
- P. Jaakkola, D. R. Mole, Y. M. Tian, M. I. Wilson and J. Gielbert,
et al., Targeting of HIF-alpha to the von Hippel-Lindau ubiquitylation complex by O2-regulated prolyl hydroxylation, Science, 2001, 292, 468–472 CrossRef CAS PubMed.
- N. S. Chandel, D. S. McClintock, C. E. Feliciano, T. M. Wood and J. A. Melendez,
et al., Reactive oxygen species generated at mitochondrial complex III stabilize hypoxia-inducible factor-1alpha during hypoxia: a mechanism of O2 sensing, Biol. Chem., 2000, 275, 25130–25138 CrossRef CAS PubMed.
-
B. H. Jiang, G. Jiang, J. Z. Zheng, Z. Lu and T. Hunter, et al., Phosphatidylinositol 3-kinase signaling controls levels of hypoxia-inducible factor 1, Cell Growth Differ, 2001, 12, 363–369 Search PubMed.
- D. Lando, D. J. Peet, J. J. Gorman, D. A. Whelan and M. L. Whitelaw,
et al., FIH-1 is an asparaginyl hydroxylase enzyme that regulates the transcriptional activity of hypoxia-inducible factor, Genes Dev., 2002, 16, 1466–1471 CrossRef CAS PubMed.
- A. R. Chade, A. Lerman and L. O. Lerman, Kidney in early atherosclerosis, Hypertension, 2005, 45, 1042–1049 CrossRef CAS PubMed.
- X. Huang, J. J. Jiménez-Moleón, B. Lindholm, T. Cederholm and J. Arnlöv,
et al., Mediterranean diet, kidney function, and mortality in men with CKD, Clin. J. Am. Soc. Nephrol., 2013, 8, 1548–1555 CrossRef CAS PubMed.
- M. Khatri, Y. P. Moon, N. Scarmeas, Y. Gu and H. Gardener,
et al., The association between a Mediterranean-style diet and kidney function in the Northern Manhattan Study cohort, Clin. J. Am. Soc. Nephrol., 2014, 9, 1868–1875 CrossRef PubMed.
- N. Di Daniele, L. Di Renzo, A. Noce, L. Iacopino and P. M. Ferraro,
et al., Effects of Italian Mediterranean organic diet vs. low-protein diet in nephropathic patients according to MTHFR genotypes, J. Nephrol., 2014, 27, 529–536 CrossRef PubMed.
- C. Manna, P. Galletti, G. Maisto, V. Cucciolla and S. D'Angelo,
et al., Transport mechanism and metabolism of olive oil hydroxytyrosol in Caco-2 cells, FEBS Lett., 2000, 470, 341–344 CrossRef CAS PubMed.
- F. Visioli, G. Bellomo and C. Galli, Free radical-scavenging properties of olive oil polyphenols, Biochem. Biophys. Res. Commun., 1998, 247, 60–64 CrossRef CAS PubMed.
- M. Deiana, O. I. Aruoma, M. L. Bianchi, J. P. Spencer and H. Kaur,
et al., Inhibition of peroxynitrite dependent DNA base modification and tyrosine nitration by the extra virgin olive oil-derived antioxidant hydroxytyrosol, Free Radicals Biol. Med., 1999, 26, 762–769 CrossRef CAS PubMed.
- M. T. Valenzuela, M. I. Núñez, M. Villalobos, E. Siles and N. Olea,
et al., Relationship between doxorubicin cell sensitivity, drug-induced DNA double-strand breaks, glutathione content and P-glycoprotein in mammalian tumor cells, Anti-Cancer Drugs, 1995, 6, 749–757 CrossRef CAS PubMed.
- H. Wang and J. A. Joseph, Quantifying cellular oxidative stress by dichlorofluorescein assay using microplate reader, Free Radicals Biol. Med., 1999, 27, 612–616 CrossRef CAS PubMed.
- M. M. Bradford and A. rapid, sensitive method for the quantifitation of microgram quantities of protein utilizing the principle of protein–dye binding, Anal. Biochem., 1976, 72, 248–254 CrossRef CAS PubMed.
- J. C. López-Ramos, R. Martínez-Romero, F. Molina, A. Cañuelo and E. Martínez-Lara,
et al., Evidence of a decrease in nitric oxide storage molecules following acute hypoxia
and/or hypobaria, by means of chemiluminescence analysis, Nitric Oxide, 2005, 13, 62–67 CrossRef PubMed.
- S. R. Meenakshi and R. Agarwal, Nitric oxide levels in patients with chronic renal disease, J. Clin. Diagn. Res., 2013, 7, 1288–1290 CAS.
- M. C. Maiuri, D. De Stefano, P. Di Meglio, C. Irace and M. Savarese,
et al., Hydroxytyrosol, a phenolic compound from virgin olive oil, prevents macrophage activation, Naunyn-Schmiedebergs Arch. Pharmacol., 2005, 371, 457–465 CrossRef CAS PubMed.
- S. Cabrerizo, J. P. De La Cruz, J. A. López-Villodres, J. Muñoz-Marín and A. Guerrero,
et al., Role of the inhibition of oxidative stress and inflammatory mediators in the neuroprotective effects of hydroxytyrosol in rat brain slices subjected to hypoxia reoxygenation, J. Nutr. Biochem., 2013, 24, 2152–2157 CrossRef CAS PubMed.
- S. Pan, L. Liu, H. Pan, Y. Ma and D. Wang,
et al., Protective effects of hydroxytyrosol on liver ischemia/reperfusion injury in mice, Mol. Nutr. Food Res., 2013, 57, 1218–1227 CAS.
- J. P. De La Cruz, M. I. Ruiz-Moreno, A. Guerrero, J. A. López-Villodres and J. J. Reyes,
et al., Role of the catechol group in the antioxidant and neuroprotective effects of virgin olive oil components in rat brain, J. Nutr. Biochem., 2015, 26, 549–555 CrossRef CAS PubMed.
- S. J. Rietjens, A. Balt and G. R. Haenen, New insights into controversies on the antioxidant potential of the olive oil antioxidant hydroxytyrosol, J. Agric. Food Chem., 2007, 55, 7609–7614 CrossRef CAS PubMed.
- M. L. Hu Dietary, polyphenols as antioxidants and anticancer agents: more questions than answers, Chang Gung Med. J., 2011, 34, 449–460 Search PubMed.
- S. J. Hsu, S. S. Wang, I. F. Hsin, F. Y. Lee and H. C. Huang,
et al., Green tea polyphenol decreases the severity of portosystemic collaterals and mesenteric angiogenesis in rats with liver cirrhosis, Clin. Sci., 2014, 126, 633–644 CrossRef CAS PubMed.
- J. B. Daleprane, T. Schmid, N. Dehne, M. Rudnicki and H. Menrad,
et al., Suppression of hypoxia-inducible factor-1α contributes to the antiangiogenic activity of red propolis polyphenols in human endothelial cells, J. Nutr., 2012, 142, 441–447 CrossRef CAS PubMed.
- R. Martínez-Romero, A. Cañuelo, E. Martínez-Lara, F. J. Oliver and S. Cárdenas,
et al., Poly(ADP-ribose) polymerase-1 modulation of in vivo response of brain hypoxia-inducible factor-1 to hypoxia/reoxygenation is mediated by nitric oxide and factor inhibiting HIF, J. Neurochem., 2009, 111, 150–159 CrossRef PubMed.
- B. Zhao, Y. Ma, Z. Xu, J. Wang and F. Wang,
et al., Hydroxytyrosol, a natural molecule from olive oil, suppresses the growth of human hepatocellular carcinoma cells via inactivating AKT and nuclear factor-kappa B pathways, Cancer Lett., 2014, 347, 79–87 CrossRef CAS PubMed.
- A. Incani, M. Deiana, G. Corona, K. Vafeiadou and D. Vauzour,
et al., Involvement of ERK, Akt and JNK signalling in H2O2-induced cell injury and protection by hydroxytyrosol and its metabolite homovanillic alcohol, Mol. Nutr. Food Res., 2010, 54, 788–796 CAS.
- L. Sun, C. Luo and J. Liu, Hydroxytyrosol induces apoptosis in human colon cancer cells through ROS generation, Food Funct., 2014, 5, 1909–1914 CAS.
- M. Hayashi, A. Tojo, T. Shimosawa and T. Fujita, The role of adrenomedullin in the renal NADPH oxidase and (pro) renin in diabetic mice, J. Diabetes Res., 2013 DOI:101155/2013/134395.
- F. Kronenberg, Emerging risk factors and markers of chronic kidney disease progression, Nat. Rev. Nephrol., 2009, 5, 677–689 CrossRef CAS PubMed.
- A. Vannay, A. Fekete, V. Müller, J. Strehlau and O. Viklicky,
et al., Effects of histamine and the h2 receptor antagonist ranitidine on ischemia-induced acute renal failure: involvement of IL-6 and vascular endothelial growth factor, Kidney Blood Pressure Res., 2004, 27, 105–113 CrossRef CAS PubMed.
- T. Granner, S. Maloney, E. Antecka, J. A. Correa and M. N. Burnier Jr., 3,4 dihydroxyphenyl ethanol reduces secretion of angiogenin in human retinal pigment epithelial cells, Br. J. Ophthalmol., 2013, 97, 371–374 CrossRef PubMed.
- Z. Arany, S. Y. Foo, Y. Ma, J. L. Ruas and A. Bommi-Reddy,
et al., HIF-independent regulation of VEGF and angiogenesis by the transcriptional coactivator PGC-1alpha, Nature, 2008, 451, 1008–1112 CrossRef CAS PubMed.
- K. Zhang, J. Lu, T. Mori, L. Smith-Powell and T. W. Synold,
et al., Baicalin increases VEGF expression and angiogenesis by activating the ERRα/PGC-1alpha pathway, Cardiovasc. Res., 2011, 89, 426–435 CrossRef CAS PubMed.
- J. Kallen, J. M. Schlaeppi, F. Bitsch, I. Filipuzzi and A. Schilb,
et al., Evidence for ligand-independent transcriptional activation of the human estrogen-related receptor alpha (ERRalpha): crystal structure of ERR alpha ligand binding domain in complex with peroxisome proliferator-activated receptor coactivator-1alpha, J. Biol. Chem., 2004, 279, 49330–49337 CrossRef CAS PubMed.
- A. L. Bookout, Y. Jeong, M. Downes, R. T. Yu and R. M. Evans,
et al., Anatomical profiling of nuclear receptor expression reveals a hierarchical transcriptional network, Cell, 2006, 126, 789–799 CrossRef CAS PubMed.
- R. Sladek, J. A. Bader and V. Giguère, The orphan nuclear receptor estrogen-related receptor alpha is a transcriptional regulator of the human medium-chain acyl coenzyme A dehydrogenase gene, Mol. Cell. Biol., 1997, 17, 5400–5409 CrossRef CAS PubMed.
- R. A. Stein, S. Gaillard and D. P. McDonnell, Estrogen-related receptor alpha induces the expression of vascular endothelial growth factor in breast cancer cells, J. Steroid Biochem. Mol. Biol., 2009, 114, 106–112 CrossRef CAS PubMed.
- A. Fradet, H. Sorel, L. Bouazza, D. Goehrig and B. Dépalle,
et al., Dual function of ERRα in breast cancer and bone metastasis formation: implication of VEGF and osteoprotegerin, Cancer Res., 2011, 71, 5728–5738 CrossRef CAS PubMed.
- J. Z. Hu, H. Long, T. D. Wu, Y. Zhou and H. B. Lu, The effect of estrogen-related receptor α on the regulation of angiogenesis after spinal cord injury, Neuroscience, 2015, 290, 570–580 CrossRef CAS PubMed.
- A. M. Keiler, O. Zierau, R. Bernhardt, D. Scharnweber and N. Lemonakis,
et al., Impact of a functionalized olive oil extract on the uterus and the bone in a model of postmenopausal osteoporosis, Eur. J. Nutr., 2014, 53, 1073–1081 CrossRef CAS PubMed.
Footnote |
† These authors contributed equally to this work. |
|
This journal is © The Royal Society of Chemistry 2016 |
Click here to see how this site uses Cookies. View our privacy policy here.