DOI:
10.1039/C5QO00407A
(Research Article)
Org. Chem. Front., 2016,
3, 298-303
Organocatalytic enantioselective Mukaiyama–Mannich reaction of fluorinated enol silyl ethers and cyclic N-sulfonyl ketimines†
Received
2nd December 2015
, Accepted 26th December 2015
First published on 28th December 2015
Abstract
The first catalytic asymmetric Mukaiyama–Mannich reaction of fluorinated silyl enol ethers and ketimines is developed. Under the catalysis of hydroquinine derived bifunctional urea, cyclic N-sulfonyl ketimines readily react with fluorinated enol silyl ethers to afford benzosultam based Cα-tetrasubstituted α-amino acid derivatives featuring a fluoroalkyl group in high to excellent yields and stereoselectivities.
Introduction
C
α-tetrasubstituted α-amino acid derivatives are widely present in natural products, drugs and pharmaceutically active substances.1 For example, altemicidin is an acaricidal and antitumor agent,1c and methyldopa is an orally active antihypertensive drug.1d It is also known that the introduction of conformationally constrained Cα-tetrasubstituted α-amino acids into peptides may bring about enhanced properties.1e–h Therefore, catalytic asymmetric synthesis of this privileged scaffold in sufficient diversity is an important task in asymmetric catalysis.2 In this context, benzosultam based α-amino acid derivatives are attractive targets, not only due to their synthetic value as chiral auxiliaries and reagents,3 but also due to their presence in bioactive compounds,4 as exemplified by HIV-1 inhibitors4a and aldose reductase inhibitors4b (Fig. 1). On the other hand, the decoration of amino acids with a fluoroalkyl group is also effective in modulating their properties,5 as illustrated by the rational design of eflornithine, a potent drug for treating sleeping sickness, by introducing an α-CF2H group to the α position of ornithine.5b Therefore, benzosultam based Cα-tetrasubstituted α-amino acid derivatives bearing a fluoroalkyl group are interesting targets for medicinal research. However, despite the advances in the enantioselective synthesis of optically active benzosultams,6 catalytic asymmetric synthesis of benzosultam based fluorinated α-amino acid derivatives is unprecedented.
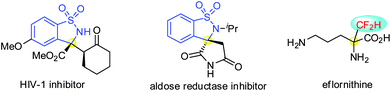 |
| Fig. 1 Selected bioactive compounds. | |
The catalytic asymmetric addition of nucleophiles to cyclic N-sulfonyl ketimines 1 provides a facile access to enantiopure benzosultam based Cα-tetrasubstituted α-amino acid derivatives.7 In 2013, Xu et al. exploited a highly enantioselective arylation of ketimines 1 using arylboronic acids catalyzed by a novel sulphur–olefin ligand derived Rh complex.7a Most recently, Nakamura et al. utilized their bifunctional cinchona alkaloid derived sulfamide to develop a nice decarboxylative Mannich addition of malonic acid half thioesters to ketimines 1.7b Wang and Loh developed a novel bifunctional amino sulfonohydrazide to accomplish a direct Mannich reaction of 1 with methyl ketones or cyclic ketones via asymmetric enamine catalysis.4a Ma et al. reported a saccharide-derived tertiary amino-thiourea enabled highly enantioselective reaction of ketimine 1 and allylic ketones.7c Herein, we wish to report our results on the enantioselective addition of fluorinated enol ethers to ketimine 1 for an unprecedented synthesis of benzosultam based β-fluorinated Cα-tetrasubstituted α-amino acid esters 3 or 5.
Recently, there has been ever-increasing interest in developing catalytic asymmetric synthesis of carbon stereocenters featuring a fluoroalkyl group, as selective fluoroalkylation is very useful in drug design to modulate the properties of bioactive compounds.8 However, while much progress has been made in asymmetric trifluoromethylation, limited success is achieved in the catalytic asymmetric creation of carbon stereocenters bearing a mono- or gem-difluoroalkyl group.8h–l In this scenario, catalytic asymmetric functionalization of fluorinated silyl enol ethers has recently emerged as a fruitful methodology for enantioselective nucleophilic mono- or gem-difluoroalkylation.9 First, the deprotonative activation of α-fluorinated ketones is unexpectedly difficult under mild conditions,10a,b so fluorinated enol silyl ethers serve as their active equivalents for reaction design. Second, the resulting optically active adducts bearing an α-fluorinated ketone moiety are of high synthetic value and can undergo various transformations.11 In addition, both mono- and difluoro enol silyl ethers can be readily prepared from inexpensive starting materials.12 To date, they have found utility in catalytic enantioselective allylic substitution,13 Mannich,14 aldol10 and Michael reactions.15 Despite the achievements, the catalytic asymmetric Mannich reaction using ketimines remains unexplored, although the corresponding reaction of aldehyde derived hydrazones or aldimines using difluoroenoxysilanes has been pioneered by Shi14a,b and Akiyama14c using a chiral Lewis acid and phosphoric acid, respectively. Recently, with our interest in selective fluoroalkylation, we have identified chiral bifunctional Lewis base catalysis16 as an effective tool to activate fluorinated silyl enol ethers for enantioselective reactions,10,15 and we are pleased to find that this catalysis pattern can be extended to the asymmetric Mukaiyama–Mannich reaction of cyclic N-sulfonyl ketimines 1 with fluorinated silyl enol ethers.
Results and discussion
We embarked on condition optimization by using the reaction of ketimine 1a and difluoroenoxysilane 2a as the model reaction, which was carried out in THF at room temperature. Some typical results are shown in Table 1.
Table 1 Condition optimization
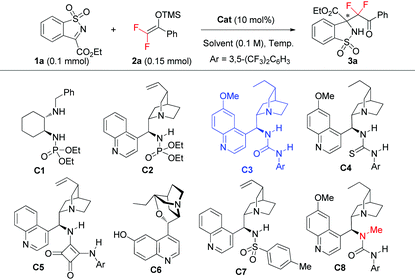
|
Entry |
Cat. |
Solvent |
Temp. (°C) |
Time (d) |
Yielda (%) |
eeb (%) |
Isolated yield.
Determined by chiral HPLC.
On a 0.25 mmol scale, with a concentration of 1a at 0.2 M.
2.0 equiv. of 2a were used.
|
1 |
C1
|
THF |
25 |
3 |
58 |
11 |
2 |
C2
|
THF |
25 |
0.5 |
81 |
17 |
3 |
C3
|
THF |
25 |
1 |
63 |
87 |
4 |
C4
|
THF |
25 |
4 |
40 |
43 |
5 |
C5
|
THF |
25 |
4 |
7 |
67 |
6 |
C6
|
THF |
25 |
3 |
70 |
56 |
7 |
C7
|
THF |
25 |
2 |
75 |
50 |
8 |
C3
|
CH3CN |
25 |
4 |
35 |
30 |
9 |
C3
|
Toluene |
25 |
4 |
68 |
94 |
10c |
C3
|
Toluene |
45 |
4 |
86 |
93 |
11c,d |
C3
|
Toluene |
45 |
4 |
90 |
93 |
12c,d |
C8
|
Toluene |
45 |
4 |
82 |
71 |
Bifunctional secondary amine-phosphoramide C115 that we developed for the corresponding Michael addition gave product 3a in 58% yield with only 11% ee (entry 1). Our bifunctional phosphoramide-tertiary amine C217 showed much better activity, but the ee value of 3a was still low (entry 2). Then we turned to a cinchona alkaloid derived urea catalyst C3,18 which enabled an efficient aldol reaction using fluorinated enol silyl ether.10 To our delight, it catalyzed the reaction to afford product 3a in 63% yield with 87% ee after a day (entry 3). The related thiourea analogue C4 proved to be inferior (entry 4). Other catalysts C5–C7,19 with different types of H-bond donors, were also examined, but no improvement was obtained in terms of both reactivity and enantioselectivity (entries 5–7). To further improve the reaction outcome, solvent effects were examined using C3 as the catalyst, which was found to play an important role. Almost no reaction occurred when ethyl acetate was used as the solvent, and less than 8% yield was obtained for product 3a when running the reaction in CH2Cl2. MeCN as the solvent delivered poor result as well (entry 8). Helpfully, the ee value of 3a was improved to 94% when toluene was used as the solvent, albeit with low reactivity (entry 9). We further conducted the reaction at a higher concentration and temperature (0.2 M at 45 °C), which enhanced the yield of 3a to 86% (entry 10), and even 90% yield if 2.0 equivalents of 2a was used (entry 11), with still excellent enantioselectivity.
It also turned out that both N–H bonds of the urea of catalyst C3 mattered in achieving high enantiofacial control, as catalyst C8, with one N–H bond of the urea moiety masked by a methyl group, gave product 3a in obviously lower enantioselectivity, but similar reactivity (entry 11 vs. 12).
Based on the above reaction optimization, we determined to evaluate the substrate scope by running the reaction in toluene (0.2 M) at 45 °C, in the presence of 10 mol% bifunctional catalyst C3 and 2.0 equiv. of difluoroenoxysilane 2a, as shown in Table 2. First, ketimine 1b with a methyl ester group afforded the desired product 3b in 93% yield and 94% ee (entry 3). Second, cyclic ketimines 1c–i all worked well with difluoroenoxysilane 2a to afford the desired benzosultam based fluorinated α-amino acid derivatives 3c–i in high to excellent yields and enantioselectivities, whatever the nature and position of the substituent on the aromatic ring (entries 4–10). Difluorinated enol silyl ethers 2b–c with either an electron-rich or electron-withdrawing aromatic substituent all gave the corresponding products 3j–k in good yield with excellent ee values (entries 11 and 12). Unfortunately, aliphatic substituted difluoroenoxysilane 2d was not active enough to react with 1a to give the desired product in a substantial amount. To show the practicability of our protocol, the reaction of 1a and 2a on a 3.0 mmol scale was tried in the presence of 5 mol% C3, affording product 3a in 0.73 g, 61% yield and 92% ee (entry 2).
Table 2 Substrate scopes

|
Entry |
1: R1, R2 |
2: R |
3
|
Yielda (%) |
eeb (%) |
Isolated yield.
Determined by chiral HPLC.
Run on 3.0 mmol using 5 mol% of catalyst C3.
|
1 |
1a: H, Et |
2a: Ph |
3a
|
90 |
93 |
2c |
1a: H, Et |
2a: Ph |
3a
|
61 |
92 |
3 |
1b: H, Me |
2a: Ph |
3b
|
93 |
94 |
4 |
1c: 5-Me, Et |
2a: Ph |
3c
|
89 |
92 |
5 |
1d: 5-MeO, Et |
2a: Ph |
3d
|
87 |
94 |
6 |
1e: 5-CF3O, Et |
2a: Ph |
3e
|
95 |
87 |
7 |
1f: 5-F, Et |
2a: Ph |
3f
|
79 |
92 |
8 |
1g: 5-Cl, Et |
2a: Ph |
3g
|
74 |
92 |
9 |
1h: 5-CF3, Et |
2a: Ph |
3h
|
94 |
84 |
10 |
1i: 7-Cl, Et |
2a: Ph |
3i
|
98 |
90 |
11 |
1a: H, Et |
2b: 4-MeOC6H4 |
3j
|
75 |
92 |
12 |
1a: H, Et |
2c: 4-ClC6H4 |
3k
|
74 |
93 |
13 |
1a: H, Et |
2d: CH2Bn |
3l
|
Trace |
— |
Gratifyingly, monofluorinated enol silyl ethers 4 were also viable substrates under the established conditions, as evidenced by synthesis of monofluorinated benzosultam derivatives 5a–c with two adjacent fully substituted carbon stereocenters in high yield with excellent dr and ee values (Scheme 1). Noticeably, the high reactivity of 4 allowed the reaction to run at 35 °C, which in turn improved the stereoselectivity. The absolute configuration of 5a was assigned to be S, S configuration by X-ray analysis of single crystal 5a. All the other products 5 were tentatively assigned.
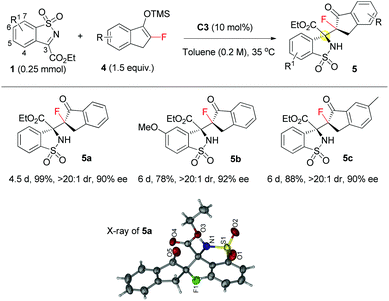 |
| Scheme 1 Scope of monofluorinated enol silyl ethers 4. | |
The resulting adducts could be further elaborated to other fluorinated benzosultam derivatives. Starting from 1.0 mmol of ketimine 1a and difluoroenoxysilane 2b, product 3j was obtained in 83% yield and 92% ee, which readily underwent a further Baeyer–Villiger oxidation20 to afford the corresponding diester 6 in 97% yield without loss in the ee value (Scheme 2). Remarkably, via an aminolysis and following cyclization at room temperature, spirocyclic benzosultam derivative 7 featuring an α gem-difluoro aminal moiety was readily obtained in 63% yield and 92% ee as a single isomer from 3a and CH3NH2. Such a type of spirocyclic compound might be an interesting target in medicinal research, as the modern probe- and drug-discovery programmes have a vast demand for optically active spirocyclic compounds with a novel structure.21 The relative and absolute configuration of compound 7 was assigned by single-crystal X-ray analysis. Based on the stereochemical information of compound 7, the absolute configuration of the Mannich adduct 3a was determined to be S, and those of other products 3b–l were tentatively assigned by analogy.
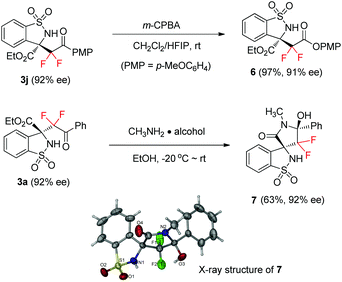 |
| Scheme 2 Synthetic elaborations. | |
Interestingly, difluoroenoxysilane 1a proved to be much more active than the non-fluorinated analogue 8 under the same conditions, as the latter gave the corresponding product 9 in only 24% yield and 32% ee (Scheme 3). This observation was similar to our previous finding that under the catalysis of C3, both di- and mono-fluorinated enol silyl ethers 2a and 4a reacted with isatin in a much faster rate than their non-fluorinated analogue,10b and Akiyama's report that in a phosphoric acid catalyzed Mannich reaction of aldimines, difluoroenoxysilane 1a was more reactive than 8.14c However, the reactivity difference between fluorinated and non-fluorinated enol silyl ethers 2a and 8 was reversed under metal catalyzed conditions. Shreeve et al. found in Pd-catalyzed arylation that 2a was less reactive than nonfluorinated 8,22 and we also recently noticed that in a Au(I)-catalyzed Mannich reaction using isatin derived N-Boc ketimine,23 fluorinated silyl ether 2a was much less active than nonfluorinated analogue 8. On the other hand, dichloroenoxysilane 12 failed to react with ketimine 1a to give the desired product under the same conditions. The reason why fluorinated enol silyl ether 2a is much more active than non-fluorinated analogue 8 under organocatalytic conditions or in the presence of a stoichiometric amount of cesium fluoride24 is unclear, which is now under investigation.
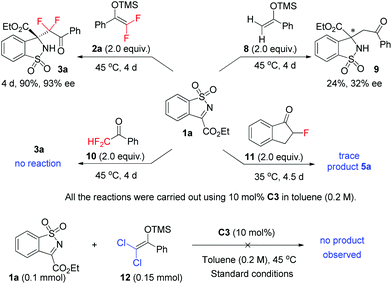 |
| Scheme 3 Fluorine effect. | |
Furthermore, while α-difluoromethyl ketone 10 failed to react with ketimine 1a under this condition, the reaction of α-monofluorinated indanone 11 with 1a worked very poorly to give only a trace amount of product 5a, unable to isolate. These control experiments unambiguously demonstrated the importance of using fluorinated enol silyl ethers to develop enantioselective reaction for selective incorporation of fluoroalkyl groups.
Based on control experiments (entry 11 vs. 12, Table 1) and the absolute configuration of products 3 and 5, the possible transition state was proposed and exemplified using difluorinated enol silyl ethers 2 (Fig. 2). The quinuclidine moiety of catalyst C3 activated difluoroenoxysilane 2via the n–σ* interaction between the nitrogen and silicon atom, whilst the N-sulfonyl ketimines were activated by the urea moiety of C3 through the H-bonding interaction. The attack of ketimines 1 by enol silyl ethers 2 was favored from the Re-face, as the steric repulsion between the aromatic ring of ketimine and enolate was avoided, affording the S-enantiomer as the major product.
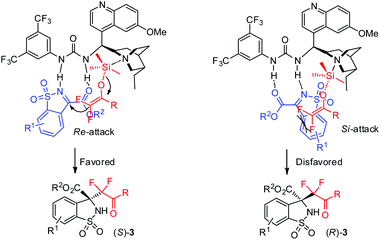 |
| Fig. 2 Proposed transition state. | |
Conclusions
In summary, we have developed the first organocatalyzed asymmetric Mukaiyama–Mannich reaction25 of fluorinated enol silyl ethers and cyclic N-sulfonyl ketimines by employing an easily available hydroquinine-derived urea catalyst. These chiral fluorinated benzosultam based Cα-tetrasubstituted α-amino acid derivatives are interesting targets for medicinal research. The development of powerful chiral bifunctional Lewis base catalysts to develop an enantioselective Mukaiyama–Mannich reaction of fluorinated enol silyl ethers with other types of ketimines is now in progress in our laboratory.
The financial support from the 973 program (2015CB856600) and NSFC (21472049) is appreciated.
Notes and references
- For leading reviews:
(a) C. Cativiela and M. D. Díaz-de-Villegas, Tetrahedron: Asymmetry, 1998, 9, 3517 CrossRef CAS;
(b) C. Cativiela and M. D. Díaz-de-Villegas, Tetrahedron: Asymmetry, 2000, 11, 645 CrossRef CAS. For selected examples:
(c) A. Takahashi, S. Kurasawa, D. Ikeda, Y. Okami and T. Takeuchi, J. Antibiot., 1989, 42, 1556 CrossRef PubMed;
(d) W. S. Saari, W. Halczenko, D. W. Cochran, M. R. Dobrinska, W. C. Vincek, D. C. Titus, S. L. Gaul and C. S. Sweet, J. Med. Chem., 1984, 27, 713 CrossRef CAS. For selected examples of peptides with enhanced properties:
(e) V. J. Hruby, Acc. Chem. Res., 2001, 34, 389 CrossRef CAS PubMed;
(f) R. Kaul and P. Balaram, Bioorg. Med. Chem., 1999, 7, 105 CrossRef CAS PubMed;
(g) W. M. Kazmierski, Z. Urbanczyk-Lipkowska and V. J. Hruby, J. Org. Chem., 1994, 59, 1789 CrossRef CAS;
(h) A. Giannis and T. Kolter, Angew. Chem., Int. Ed. Engl., 1993, 32, 1244 CrossRef;
(i) Y.-H. Wang, Z.-Y. Cao, Y.-F. Niu, X.-L. Zhao and J. Zhou, Acta Chim. Sin., 2014, 72, 867 CrossRef CAS;
(j) X.-P. Yin, P.-W. Xu, K. Dong, K. Liao, F. Zhou and J. Zhou, Acta Chim. Sin., 2015, 73, 685 CrossRef CAS.
- For reviews:
(a) A. E. Metz and M. C. Kozlowski, J. Org. Chem., 2015, 80, 1 CrossRef CAS PubMed;
(b) Y.-L. Liu and J. Zhou, Synthesis, 2015, 1210 CAS;
(c) J. Zhou, Org. Chem.: Curr. Res., 2014, 3, e136, DOI:10.4172/2161-0401.1000e136;
(d) F. Zhou, F.-M. Liao, J.-S. Yu and J. Zhou, Synthesis, 2014, 2983 CrossRef CAS;
(e) J. Wang, X. Liu and X. Feng, Chem. Rev., 2011, 111, 6947 CrossRef CAS PubMed;
(f) P. Merino, E. Marqués-López, T. Tejero and R. P. Herrera, Tetrahedron, 2009, 65, 1219 CrossRef CAS;
(g) H. Vogt and S. Bräse, Org. Biomol. Chem., 2007, 5, 406 RSC.
- For chiral auxiliaries:
(a)
O. Reiser, in Organic Synthesis Highlights IV, ed. H.-G. Schmalz, Wiley-VCH, Weinheim, Germany, 2000, p. 11 Search PubMed;
(b) W. Oppolzer, M. Wills, M. J. Kelly, M. Signer and J. Blagg, Tetrahedron Lett., 1990, 31, 5015 CrossRef CAS;
(c) Z. Liu and Y. Takeuchi, Heterocycles, 2002, 56, 693 CrossRef CAS. For synthetic reagents, see:
(d) F. A. Davis and B.-C. Chen, Chem. Rev., 1992, 92, 919 CrossRef CAS;
(e) E. Differding, G. M. Rüegg and R. W. Lang, Tetrahedron Lett., 1991, 32, 1779 CrossRef CAS;
(f) K. H. Ahn, S. Kim and C. Ham, Tetrahedron Lett., 1998, 39, 6321 CrossRef CAS;
(g) Y. Takeuchi, T. Suzuki, A. Satoh, T. Shiragami and N. Shibata, J. Org. Chem., 1999, 64, 5708 CrossRef CAS PubMed;
(h) H. Sun, Z. Liu and L. Tang, Chin. Chem. Lett., 2008, 19, 907 CrossRef CAS.
-
(a) S. Zhang, L. Li, Y. Hu, Z. Zha, Z. Wang and T.-P. Loh, Org. Lett., 2015, 17, 1050 CrossRef CAS PubMed;
(b) J. Wrobel, A. Dietrich, S. A. Woolson, J. Millen, M. McCaleb, M. C. Harrison, T. C. Hohman, J. Sredy and D. Sullivan, J. Med. Chem., 1992, 35, 4613 CrossRef CAS PubMed;
(c)
J. Mao and D. C. Baker, US Patent, 6458962B1, 2003 Search PubMed;
(d)
C. Yoakim, J. O'Meara, B. Simoneau, W. W. Ogilvie and R. Deziel, Patent Appl, WO2004026875A1, 2004 Search PubMed;
(e)
D. C. Baker and B. Jiang, US Patent, 6353112B1, 2002 Search PubMed.
- For a review, see:
(a) X.-L. Qiu and F.-L. Qing, Eur. J. Org. Chem., 2011, 3261 CrossRef CAS. For selected examples:
(b) P. Bey, F. Gerhart, V. V. Dorsselaer and C. Danzin, J. Med. Chem., 1983, 26, 1551 CrossRef CAS PubMed;
(c) C. Pesenti and F. Viani, ChemBioChem, 2004, 5, 590 CrossRef CAS PubMed;
(d) K. Uoto, S. Ohsuki, H. Takenoshita, T. Ishiyama, S. Iimura, Y. Hirota, I. Mitsui, H. Terasawa and T. Soga, Chem. Pharm. Bull., 1997, 45, 1793 CrossRef CAS PubMed.
- Selected reaction of cyclic N-sulfonyl ketimines:
(a) M. Rommel, T. Fukuzumi and J. W. Bode, J. Am. Chem. Soc., 2008, 130, 17266 CrossRef CAS PubMed;
(b) B. M. Trost and S. M. Silverman, J. Am. Chem. Soc., 2012, 134, 4941 CrossRef CAS PubMed;
(c) Y. Luo, H. B. Hepburn, N. Chotsaeng and H. W. Lam, Angew. Chem., Int. Ed., 2012, 51, 8309 CrossRef CAS PubMed;
(d) T. Nishimura, A. Noishiki, G. C. Tsui and T. Hayashi, J. Am. Chem. Soc., 2012, 134, 5056 CrossRef CAS PubMed;
(e) G. Yang and W. Zhang, Angew. Chem., Int. Ed., 2013, 52, 7540 CrossRef CAS PubMed;
(f) Y. Yao, J.-L. Li, Q.-Q. Zhou, L. Dong and Y.-C. Chen, Chem. – Eur. J., 2013, 19, 9447 CrossRef CAS PubMed;
(g) S. Takizawa, F. A. Arteaga, Y. Yoshida, M. Suzuki and H. Sasai, Asian J. Org. Chem., 2014, 3, 412 CrossRef CAS;
(h) X. Yin, Y. Zheng, X. Feng, K. Jiang, X. Wei, N. Gao and Y. Chen, Angew. Chem., Int. Ed., 2014, 53, 6245 CrossRef CAS PubMed;
(i) C. Jiang, Y. Lu and T. Hayashi, Angew. Chem., Int. Ed., 2014, 53, 9936 CrossRef CAS PubMed;
(j) J. L. Y. Chen and V. K. Aggarwal, Angew. Chem., Int. Ed., 2014, 53, 10992 CrossRef CAS PubMed;
(k) H. B. Hepburn and H. W. Lam, Angew. Chem., Int. Ed., 2014, 53, 11605 CrossRef CAS PubMed;
(l) S. Zhang, L. Li, Y. Hu, Y. Li, Y. Yang, Z. Zha and Z. Wang, Org. Lett., 2015, 17, 5036 CrossRef CAS PubMed;
(m) Y. Álvarez-Casao, D. Monge, E. Álvarez, R. Fernández and J. M. Lassaletta, Org. Lett., 2015, 17, 5104 CrossRef PubMed;
(n) C. A. Osborne, T. B. D. Endean and E. R. Jarvo, Org. Lett., 2015, 17, 5340 CrossRef CAS PubMed;
(o) X. Chen, J.-Q. Zhang, S.-J. Yin, H.-Y. Li, W.-Q. Zhou and X.-W. Wang, Org. Lett., 2015, 17, 4188 CrossRef CAS PubMed;
(p) Y. Luo, A. J. Carnell and H. W. Lam, Angew. Chem., Int. Ed., 2012, 51, 6762 CrossRef CAS PubMed;
(q) C. Schrapel and R. Peters, Angew. Chem., Int. Ed., 2015, 54, 10289 CrossRef CAS PubMed;
(r) L. Wu, R.-R. Liu, G. Zhang, D.-J. Wang, H. Wu, J. Gao and Y.-X. Jia, Adv. Synth. Catal., 2015, 357, 709 CrossRef CAS;
(s) S. Takizawa, K. Kishi, M. A. Abozeid, K. Murai, H. Fujioka and H. Sasai, Org. Biomol. Chem., 2016, 14, 761 RSC.
-
(a) H. Wang, T. Jiang and M.-H. Xu, J. Am. Chem. Soc., 2013, 135, 971 CrossRef CAS PubMed;
(b) S. Nakamura, M. Sano, A. Toda, D. Nakane and H. Masuda, Chem. – Eur. J., 2015, 21, 3929 CrossRef CAS PubMed;
(c) B. Qiao, Y.-J. Huang, J. Nie and J.-A. Ma, Org. Lett., 2015, 17, 4608 CrossRef CAS PubMed . Also see ref. 4a.
-
(a) K. Mikami, Y. Itoh and M. Yamanaka, Chem. Rev., 2004, 104, 1 CrossRef CAS PubMed;
(b) J.-A. Ma and D. Cahard, Chem. Rev., 2004, 104, 6119 CrossRef CAS PubMed;
(c) D. O'Hagan, Chem. Soc. Rev., 2008, 37, 308 RSC;
(d) L. Hunter, Beilstein J. Org. Chem., 2010, 6 DOI:10.3762/bjoc.6.38;
(e) C.-P. Zhang, Q.-Y. Chen, Y. Guo, J.-C. Xiao and Y.-C. Gu, Chem. Soc. Rev., 2012, 41, 4536 RSC;
(f) J. Wang, M. Sánchez-Roselló, J. L. Aceña, C. del Pozo, A. E. Sorochinsky, S. Fustero, V. A. Soloshonok and H. Liu, Chem. Rev., 2014, 114, 2432 CrossRef CAS PubMed;
(g) J.-A. Ma and S. Li, Org. Chem. Front., 2014, 1, 712 RSC;
(h) Y.-L. Liu, J.-S. Yu and J. Zhou, Asian J. Org. Chem., 2013, 2, 194 CrossRef CAS;
(i) J.-H. Lin and J.-C. Xiao, Tetrahedron Lett., 2014, 55, 6147 CrossRef CAS;
(j) C. Ni, L. Zhu and J. Hu, Acta Chim. Sin., 2015, 73, 90 CrossRef CAS;
(k) X. Yang, T. Wu, R. J. Phipps and F. D. Toste, Chem. Rev., 2015, 115, 826 CrossRef CAS PubMed;
(l) P. A. Champagne, J. Desroches, J.-D. Hamel, M. Vandamme and J.-F. Paquin, Chem. Rev., 2015, 115, 9073 CrossRef CAS PubMed.
- For a recent review: M. Decostanzi, J.-M. Campagne and E. Leclerc, Org. Biomol. Chem., 2015, 13, 7351 CAS.
-
(a) Y.-L. Liu and J. Zhou, Chem. Commun., 2012, 48, 1919 RSC;
(b) Y.-L. Liu, F.-M. Liao, Y.-F. Niu, X.-L. Zhao and J. Zhou, Org. Chem. Front., 2014, 1, 742 RSC;
(c) Y.-L. Liu and J. Zhou, Acta Chim. Sin., 2012, 70, 1451 CrossRef CAS. For other reactions using fluorinated enol silyl ethers developed by our group, see:
(d) J.-S. Yu, Y.-L. Liu, J. Tang, X. Wang and J. Zhou, Angew. Chem., Int. Ed., 2014, 53, 9512 CrossRef CAS PubMed;
(e) F.-M. Liao, Y.-L. Liu, J.-S. Yu, F. Zhou and J. Zhou, Org. Biomol. Chem., 2015, 13, 8906 RSC;
(f) Y.-L. Liu, X.-P. Zeng and J. Zhou, Chem. – Asian J., 2012, 7, 1759 CrossRef CAS PubMed.
-
(a) D. Schirlin, S. Baltzer, J. M. Altenburger, C. Tarnus and J. M. Remy, Tetrahedron, 1996, 52, 305 CrossRef CAS;
(b) A. M. Silva, R. E. Cachau, H. L. Sham and J. W. Erickson, J. Mol. Biol., 1996, 255, 321 CrossRef CAS PubMed;
(c) S. Thalsrivongs, D. T. Pals, W. M. Kati, S. R. Turner, L. M. Tharnasco and W. Watt, J. Med. Chem., 1986, 29, 2080 CrossRef;
(d) M. H. Gelb, J. Am. Chem. Soc., 1986, 108, 3146 CrossRef CAS.
-
(a) H. Amii, T. Kobayashi, Y. Hatamoto and K. Uneyama, Chem. Commun., 1999, 1323 RSC;
(b) G. K. S. Prakash, J. Hu and G. A. Olah, J. Fluorine Chem., 2001, 112, 357 CrossRef;
(c) F. Huguenot, A. Billac, T. Brigaud and C. Portella, J. Org. Chem., 2008, 73, 2564 CrossRef CAS PubMed.
- É. Bélanger, K. Cantin, O. Messe, M. Tremblay and J.-F. Paquin, J. Am. Chem. Soc., 2007, 129, 1034 CrossRef PubMed.
-
(a) Z. Yuan, Y. Wei and M. Shi, Chin. J. Chem., 2010, 28, 1709 CrossRef CAS;
(b) Z. Yuan, L. Mei, Y. Wei, M. Shi, P. V. Kattamuri, P. McDowell and G. Li, Org. Biomol. Chem., 2012, 10, 2509 RSC;
(c) W. Kashikura, K. Mori and T. Akiyama, Org. Lett., 2011, 13, 1860 CrossRef CAS PubMed.
- J.-S. Yu, F.-M. Liao, W.-M. Gao, K. Liao, R.-L. Zuo and J. Zhou, Angew. Chem., Int. Ed., 2015, 54, 7381 CrossRef CAS PubMed.
-
(a) S. E. Denmark and G. L. Beutner, Angew. Chem., Int. Ed., 2008, 47, 1560 CrossRef CAS PubMed;
(b) J. Gawronski, N. Wascinska and J. Gajewy, Chem. Rev., 2008, 108, 5227 CrossRef CAS PubMed.
-
(a) M. Ding, F. Zhou, Y.-L. Liu, C.-H. Wang, X.-L. Zhao and J. Zhou, Chem. Sci., 2011, 2, 2035 RSC;
(b) W.-M. Gao, J.-S. Yu, Y.-L. Zhao, Y.-L. Liu, F. Zhou, H.-H. Wu and J. Zhou, Chem. Commun., 2014, 50, 15179 RSC;
(c) Y.-L. Liu, F. Zhou, J.-J. Cao, C.-B. Ji, M. Ding and J. Zhou, Org. Biomol. Chem., 2010, 8, 3847 RSC.
- For pioneer work on cinchona alkaloid thiourea catalysts, see:
(a) B.-J. Li, L. Jiang, M. Liu, Y.-C. Chen, L.-S. Ding and Y. Wu, Synlett, 2005, 603 CAS;
(b) B. Vakulya, S. Varga, A. Csámpai and T. Soós, Org. Lett., 2005, 7, 1967 CrossRef CAS PubMed;
(c) S. H. McCooey and S. J. Connon, Angew. Chem., Int. Ed., 2005, 44, 6367 CrossRef CAS PubMed;
(d) J. Ye, D. J. Dixon and P. S. Hynes, Chem. Commun., 2005, 4481 RSC.
-
(a) E. M. O. Yeboah, S. O. Yeboah and G. S. Singh, Tetrahedron, 2011, 67, 1725 CrossRef CAS;
(b) T. Marcelli and H. Hiemstra, Synthesis, 2010, 1229 CrossRef CAS;
(c)
J. Zhou, Multicatalyst system in asymmetric catalysis, John Wiley &
Sons, New York, 2014, ch. 3 Search PubMed.
- S. Kobayashi, H. Tanaka, H. Amii and K. Uneyama, Tetrahedron, 2003, 59, 1547 CrossRef CAS.
- For leading reviews:
(a) I. Sharma and D. S. Tan, Nat. Chem., 2013, 5, 157 CrossRef CAS PubMed;
(b) Y. Zheng, C. M. Tice and S. B. Singh, Bioorg. Med. Chem. Lett., 2014, 24, 3673 CrossRef CAS PubMed;
(c) R. Rios, Chem. Soc. Rev., 2012, 41, 1060 RSC;
(d) E. M. Carreira and T. C. Fessard, Chem. Rev., 2014, 114, 8257 CrossRef CAS PubMed;
(e) J.-H. Xie and Q.-L. Zhou, Acta Chim. Sin., 2014, 72, 778 CrossRef CAS;
(f) Z.-Y. Cao and J. Zhou, Org. Chem. Front., 2015, 2, 849 RSC. For our efforts in the synthesis of spirocylic compounds:
(g) Z.-Y. Cao, X. Wang, C. Tan, X.-L. Zhao, J. Zhou and K. Ding, J. Am. Chem. Soc., 2013, 135, 8197 CrossRef CAS PubMed;
(h) Y.-L. Liu, X. Wang, Y.-L. Zhao, F. Zhu, X.-P. Zeng, L. Chen, C.-H. Wang, X.-L. Zhao and J. Zhou, Angew. Chem., Int. Ed., 2013, 52, 13735 CrossRef CAS PubMed;
(i) X.-P. Yin, X.-P. Zeng, Y.-L. Liu, F.-M. Liao, J.-S. Yu, F. Zhou and J. Zhou, Angew. Chem., Int. Ed., 2014, 53, 13740 CrossRef CAS PubMed.
- Y. Guo and J.-M. Shreeve, Chem. Commun., 2007, 3583 RSC.
- J.-S. Yu and J. Zhou, Org. Biomol. Chem., 2015, 13, 10968 CAS.
- During the reviewing process, one of the referees was interested in whether the reactivity difference exists when using CsF to promote the reaction. We found that the reaction of 2a and 1a mediated by 1.0 equivalent CsF could finish within 0.5 h to give the corresponding racemic product 3a in 66% yield, whereas nonfluorinated enol silyl ether 8 failed to react with 1a even when the reaction time was prolonged to 4 h under the same conditions.
.
- For the Mukaiyama–Mannich reaction of ketimines:
(a) S. Saaby, K. Nakama, M. A. Lie, R. G. Hazell and K. A. Jørgensen, Chem. – Eur. J., 2003, 9, 6145 CrossRef CAS PubMed;
(b) Y. Suto, M. Kanai and M. Shibasaki, J. Am. Chem. Soc., 2007, 129, 500 CrossRef CAS PubMed. For reviews on the asymmetric addition of nucleophiles to ketimines:
(c) S. Kobayashi, Y. Mori, J. S. Fossey and M. M. Salter, Chem. Rev., 2011, 111, 2626 CrossRef CAS PubMed;
(d) N. Kumagai and M. Shibasaki, Bull. Chem. Soc. Jpn., 2015, 88, 503 CrossRef CAS.
Footnote |
† Electronic supplementary information (ESI) available. CCDC 1439365 (5a) and 1439366 (7). For ESI and crystallographic data in CIF or other electronic format see DOI: 10.1039/c5qo00407a |
|
This journal is © the Partner Organisations 2016 |
Click here to see how this site uses Cookies. View our privacy policy here.