DOI:
10.1039/C5SM02438B
(Paper)
Soft Matter, 2016,
12, 876-887
Oil-in-oil emulsions stabilised solely by solid particles†
Received
30th September 2015
, Accepted 4th November 2015
First published on 9th November 2015
Abstract
A brief review of the stabilisation of emulsions of two immiscible oils is given. We then describe the use of fumed silica particles coated with either hydrocarbon or fluorocarbon groups in acting as sole stabilisers of emulsions of various vegetable oils with linear silicone oils (PDMS) of different viscosity. Transitional phase inversion of emulsions, containing equal volumes of the two oils, from silicone-in-vegetable (S/V) to vegetable-in-silicone (V/S) occurs upon increasing the hydrophobicity of the particles. Close to inversion, emulsions are stable to coalescence and gravity-induced separation for at least one year. Increasing the viscosity of the silicone oil enables stable S/V emulsions to be prepared even with relatively hydrophilic particles. Predictions of emulsion type from calculated contact angles of a silica particle at the oil–oil interface are in agreement with experiment provided a small polar contribution to the surface energy of the oils is included. We also show that stable multiple emulsions of V/S/V can be prepared in a two-step procedure using two particle types of different hydrophobicity. At fixed particle concentration, catastrophic phase inversion of emulsions from V/S to S/V can be effected by increasing the volume fraction of vegetable oil. Finally, in the case of sunflower oil + 20 cS PDMS, the study is extended to particles other than silica which differ in chemical type, particle size and particle shape. Consistent with the above findings, we find that only sufficiently hydrophobic particles (clay, zinc oxide, silicone, calcium carbonate) can act as efficient V/S emulsion stabilisers.
Introduction
It has been well documented that a variety of solid particles can adsorb at fluid interfaces1 and hence stabilise emulsions and foams as well as enabling the preparation of novel materials like dry water,2–4 dry oil,5–7 liquid marbles8–11 and powdered emulsions.12 The fluid pairs include oil and water (Pickering emulsions),13 air and water (particle-stabilised aqueous foams),14 air and oil (particle-stabilised oil foams)15 and water and water (Pickering emulsions).16 This paper is concerned with mixtures of immiscible oils stabilised by particles, so-called oil-in-oil, o/o, emulsions. We refer specifically to immiscible liquids of low dielectric constant ε (both ≤3.2 at 25 °C), like vegetable oil + silicone oil. Although in the literature water-free emulsions have been termed non-aqueous or even oil-in-oil emulsions,17–20 in which the water component (ε = 80.1) has been replaced by other polar solvents like ethylene glycol (ε = 37.0), dimethyl formamide (ε = 36.7) or dimethyl sulphoxide (ε = 46.7), we will not be concerned with these here. However, we acknowledge the earlier studies on alcohol (ε = 24.5–32.7)–alkane (ε = 2.0) mixtures stabilised by particles yielding bijels or conventional droplet emulsions depending on the processing route in changing the temperature of the system.21 In addition, literature exists on the use of both hydrophobic and hydrophilic particles in stabilising immiscible polymer blends,22–25e.g. poly(isobutylene)-in-poly(dimethylsiloxane) blends which are very stable to coalescence have been prepared in the presence of fumed silica particles.22
There are very few reports in the literature on oil-in-oil emulsions, where both oils have low dielectric constants, and the majority of these are patents. Table 1 contains a summary of those we could find in year order with an indication of which oil constitutes the dispersed/continuous phase, the type of emulsifier and the kind of application.26–40 The mutual solubilities of the oil pairs are not reported but from our own work we have established that certain combinations exhibit non-trivial mutual miscibility. It can be seen that o/o emulsions are of use in a wide variety of industries including cosmetics,31–33 personal care,26,28,31,34 electronics29,30,39 and pharmaceuticals.35,40 It is also worth adding that these emulsions are sometimes used as metalworking fluids which must exclude water and as chemical reaction vehicles involving reactants sensitive (even explosive) to the presence of traces of water.37 Nearly all of the examples given involve a silicone oil (low intermolecular forces) as either the droplet or continuous phase in combination with either mineral/paraffin oil or vegetable oil. Ten of the fifteen compositions contain molecular surfactant28,35,37,40 or polymer27,30,31,34,36,39 as the emulsifier, whilst in four of them the emulsions are stabilised by solid particles alone. The latter are all hydrophobic in nature (organo-clay,26 fluoro-silicone,32 fluoro-lauroyl taurate33 and wax38). In a couple of papers, Nonomura et al. determined the phase diagram of ternary mixtures of a silicone oil, perfluoropolymethylisopropyl ether (a fluorinated oil) and either spherical fluorinated silicone resin particles (diameter 4.5 μm)32 or plate-like fluorinated calcium lauroyl taurate particles (diameter 8 μm).33 Seven or eight different regions were identified in which, inter alia, powders, granules, dispersions and emulsions were formed. The emulsions were only of the kind silicone oil-in-fluorinated oil with droplets being shown to be coated by a dense layer of particles. No description of their sizes or bulk emulsion stability was given however. In the patent authored by Cauvin et al.,38 silicone oil-in-rapeseed oil emulsions were stabilised by wax crystals formed in situ by cooling the vegetable oil phase prior to emulsification. A triggered release of small antifoam particles of silica dispersed in the silicone oil droplets, achieved by heating the emulsion above the melting temperature of the wax particles coating the drops, allowed effective defoaming of surfactant-stabilised aqueous foams. Since the other reports are patents without scientific insight, it is thus clear that a detailed understanding of oil-in-oil emulsions is lacking and this is what we set out to achieve here.
Table 1 Summary of the published literature on emulsions of two immiscible oils
Dispersed phase |
Continuous phase |
Emulsifier |
Application |
Ref. |
PDMS – poly(dimethylsiloxane).
PMPS – poly(methylphenylsiloxane).
Although its dielectric constant is >3 it is included as an interesting oil.
PFPMIE – perfluoropolymethylisopropyl ether.
BA – butyl acrylate.
O/NPE – octyl/nonylpolyoxyethylene ether.
|
Silicone oil (PDMS,a PMPSb) |
Mineral oil |
Hydrophobic bentonite particles |
Lubricants on fibers |
26
|
Silicone oil, >300 cS (polydialkylsiloxane) |
Mineral oil |
Ethylene–vinyl acetate copolymer |
Foam inhibitor for lubricating oils |
27
|
Triglyceride oil (soybean, rapeseed…) |
Silicone oil, <500 cS (PDMS) |
Silicone surfactant |
Lubricant on fibers |
28
|
Silicone oil, 2500 cS (PDMS) |
Chlorinated paraffin oil, 340 cS |
None (drops had short lifetimes) |
Electro-rheological fluid |
29
|
Chlorinated paraffin oil, 340 cS |
Silicone oil, 2500 cS (PDMS) |
None (drops had short lifetimes) |
Silicone oil, 1000 cS (PDMS) |
Thermotropic liquid crystalc |
Cyanobiphenyl-PDMS oligomer |
Electro-optical display devices |
30
|
Thermotropic liquid crystal |
Silicone oil, 1000 cS (PDMS) |
Cyanobiphenyl-PDMS polymer |
Mineral oil or vegetable oil |
Silicone oil, <1000 cS |
Elastomeric silicone polyether |
Personal care and cosmetics |
31
|
Silicone oil (PDMS) |
PFPMIEd |
Fluorinated silicone resin particles (sphere) |
Cosmetics |
32
|
Silicone oil (PDMS) |
PFPMIE |
Fluorinated Ca lauroyl taurate particles (plate) |
Cosmetics |
33
|
Mineral oil or animal or vegetable oil |
Silicone oil (PDMS) |
Block copolymer, e.g. p(BAe)-PDMS-p(BA) |
Personal care |
34
|
Castor oil |
Silicone oil, <100 cS (PDMS) |
Nonionic surfactant (O/NPEf) |
Pharmaceutical formulations |
35
|
Organic phosphate |
Hydrocarbon |
Diblock or triblock copolymer |
Liquid toning systems |
36
|
Hydrocarbon |
Fluorocarbon |
Fluorocarbon surfactant |
Vehicles for chemical reactions |
37
|
(Silica in) PDMS |
Vegetable oil or PEO–PPO copolymer |
Wax crystals |
Antifoam |
38
|
Organic phosphate or silicone oil |
Paraffin oil or white mineral oil or cyclic silicone |
Hydrophobic fumed silica particles + polymer co-stabiliser |
Electro-optical modulating display devices |
39
|
Castor oil |
Silicone oil, 20 cS |
Silicone surfactant |
Pharmaceutical formulations |
40
|
Silicone oil, 20 cS (PDMS) |
Castor oil |
Silicone surfactant |
In this paper, we explore systematically the behaviour of immiscible mixtures of vegetable oil and silicone oil in the presence of fumed silica particles, coated with either hydrocarbon groups or fluorocarbon chains. We modify a previously derived theory to calculate the contact angle a spherical particle adopts at the relevant oil–oil interface and compare the experimental findings of emulsion type with these calculations. Simple emulsions of both types and multiple oil-in-oil-in-oil emulsions can be prepared which are ultra-stable to coalescence. Evidence is given for both transitional and catastrophic phase inversion of emulsions. We also explore the possibility of stabilising such emulsions with other types of particle.
Experimental
Materials
Two types of fumed silica particles were used, dichlorodimethylsilane Si(CH3)2Cl2, DCDMS-coated particles and fluoro-coated particles both from Wacker Chemie, Burghausen. The silica particles have varying percentages of residual surface SiOH groups. Both are composed of aggregates of particles of primary diameter 20–30 nm and were obtained by surface chemical modification of hydrophilic fumed silica (100% SiOH, surface area 200 m2 g−1). The silanol content of hydrocarbon-coated particles is varied from 100 to 14% and that of fluoro-coated particles was either 75%, 59% or 50%. They were obtained by reacting hydrophilic silica particles with varying amounts of either DCDMS41 or tridecafluoro-1,1,2,2-tetrahydrooctyltrimethoxysilane, CF3(CF2)5(CH2)2Si(OCH3)3, TDF42 respectively as described earlier. Details of the other particle types used are given in Table S1 of the ESI.† Three triglyceride vegetable oils were selected whose main fatty acid chains derive from oleic (C18, one double bond), linoleic (C18, two double bonds) or erucic (C22, one double bond) acids. They are, respectively, olive oil (Sigma, density ρ = 0.91 g cm−3, ε = 3.1 at 25 °C), sunflower oil (Tesco, ρ = 0.92 g cm−3, ε = 3.2) and rapeseed oil (Fluka, ρ = 0.92 g cm−3, ε = 3.1) and were passed twice through a basic alumina column to remove polar impurities before use. The polydimethylsiloxane, PDMS, silicone oils of viscosity 20 (ρ = 0.95 g cm−3, ε = 2.7), 50 (ρ = 0.96 g cm−3, ε = 2.7) and 100 cS (ρ = 0.97 g cm−3, ε = 2.7) were purchased from Dow Corning and used as received.
Methods
(i) Surface and interfacial tensions.
The oil–air surface tension γo was measured using a Krüss K12 digital tensiometer and the du Noüy ring (Pt–Ir) method at 25 °C. The results of 3 separate measurements were averaged. Measured values above 25 mN m−1 were corrected with the Huh and Mason43 correction factor, knowing the oil density (Anton Paar DMA 35N digital densitometer). The cleaning procedure involved rinsing the vessels in alcoholic KOH solution, Milli-Q water and drying. The ring was heated to glowing in a blue Bunsen flame prior to all measurements. The vegetable oil (o1)–PDMS oil (o2) interfacial tension γo1o2 was measured using the Krüss Site 04 spinning drop tensiometer at 25 °C, calibrated using the heptane–water interface. A small drop of vegetable oil (less dense phase) was spun in a PDMS oil at between 5000 and 8000 rpm to obtain a cylindrical drop. The diameter and length were measured, as was the refractive index of the silicone oil (Abbé Refractometer, Hilger). The various tensions are given in Tables 2 and 3.
Table 2 Measured oil–air surface tensions of vegetable and silicone oils, γo, at 25 °C and their polar γpo and dispersion γdo components
Oil |
Tension/mN m−1 |
γ
o
|
γ
po
|
γ
do
|
Taken from ref. 53 at 20 °C.
Taken from ref. 51 at 25 °C.
|
Olive |
33.1, 33.0a |
0.1a |
32.9a |
Sunflower |
32.2, 33.3a |
0.4a |
32.9a |
Rapeseed |
33.8 |
|
|
20 cS PDMS |
20.8 |
0.9 |
19.9 |
50 cS PDMS |
21.2, 21.0b |
0.9b |
20.1b |
100 cS PDMS |
21.4 |
0.9 |
20.5 |
Table 3 Measured vegetable oil–silicone oil interfacial tensions at 25 °C
Oil–oil interface |
γ
o1o2/±0.2 mN m−1 |
Olive–20 cS PDMS |
1.0 |
Olive–50 cS PDMS |
2.0 |
Olive–100 cS PDMS |
2.8 |
Sunflower–20 cS PDMS |
1.8 |
Sunflower–50 cS PDMS |
2.7 |
Sunflower–100 cS PDMS |
1.5 |
Rapeseed–20 cS PDMS |
1.0 |
Rapeseed–50 cS PDMS |
2.3 |
Rapeseed–100 cS PDMS |
2.7 |
(ii) Miscibility of oil pairs.
Equal volumes (2.5 cm3) of a vegetable oil and a PDMS oil were placed in a screw-cap glass vial (inner diameter of 1.8 cm and height of 7.2 cm). The mixture was sheared vigorously for 2 min using an IKA RCT basic magnetic stirrer operating at 1500 rpm at 25 °C, and the volume of the two oil phases separated after one day was measured.
(iii) Contact angles at oil–oil–solid interface.
We prepared compressed discs of the fumed silica particles and attempted to measure the contact angle of a silicone oil drop under vegetable oil on their surfaces, θoo. This was not possible however as they were porous and oils entered the disks during measurement. Instead, hydrophilic microscope glass slides (Academy Science, UK) were used either bare or after maximum hydrophobisation with DCDMS (purity ≥99.5%, Fluka) after cutting them into suitable sizes (2.5 cm × 2.5 cm). The aim is to mimic the surfaces of the 100 and 14% SiOH fumed silica particles. The contact angle an oil drop makes with the glass surfaces (in air θo or in one of the oils θoo) was measured using the sessile drop technique using a Krüss DSA Mk 10 instrument. Ten (five) μL of oil was used for the advancing (receding) angles. For advancing θo, a drop of oil was formed in air on a glass slide using an Eppendorf micropipette. For advancing θoo, a transparent glass cuvette (inner dimensions 3 cm × 3 cm × 3 cm, OG Hëllma) containing a glass slide was partially filled with a vegetable oil. A PDMS oil drop (10 μL) was then formed on the glass slide. The receding angles were measured after withdrawing 5 μL of the PDMS oil from the drop. The profile of these droplets was obtained separately and analysed and an average of 4 separate contact angle measurements is reported. Similarly, the advancing and receding contact angles of water on the glass slides in air and in the vegetable oils were measured for comparison. The glass slides were hydrophobised by placing them vertically on a Teflon rack in a sealed glass vessel containing 0.5 mL of DCDMS. The glass slides were removed after 1 h, stored in petri dishes and used within 24 h.
(iv) Preparation and characterisation of simple and multiple oil–oil emulsions.
Batch emulsions comprising equal volumes of either olive, sunflower or rapeseed oils with either 20, 50 or 100 cS PDMS oil in the presence of either DCDMS-coated particles of between 100% and 14% SiOH or 75%, 59% or 50% SiOH fluorinated fumed silica particles were investigated. The emulsions were prepared using the powdered particle method in screw cap glass vials (inner diameter of 2.4 cm and height of 7.2 cm). The required volume (5 cm3) of PDMS oil was placed in the vial followed by addition of the required mass of particles. The required volume (5 cm3) of a vegetable oil was then added. All of the fumed silica particle types were spontaneously wetted by the PDMS oils and entered into them. The mixture was homogenised using an IKA T25 digital Ultra-Turrax homogeniser with an operating head of inner diameter 1.3 cm at 12
000 rpm for 2 min. In other experiments to determine the influence of initial particle location, particles were wetted first by the vegetable oil after which PDMS oil was added and the mixture homogenised as before. Multiple oil-in-oil-in-oil emulsions were prepared in two stages. A simple emulsion of vegetable oil-in-silicone oil was prepared as above stabilised by 14% SiOH particles. This emulsion was then either stirred gently at 500 rpm with a magnetic stirrer or mixed with high shear at 6000 rpm with the Ultra-Turrax homogeniser into a vegetable oil dispersion of 51% SiOH particles. The converse was also attempted; silicone oil-in-vegetable oil emulsion with 51% SiOH particles mixed into silicone oil dispersion containing 14% SiOH particles.
The drop test was used to confirm the continuous phase of the emulsions. Vegetable oil (PDMS oil) continuous emulsions disperse easily in vegetable oil (PDMS oil), but did not disperse easily in PDMS oil (vegetable oil). The stability of the emulsions to creaming or sedimentation and coalescence was assessed by noting the fraction of PDMS oil fPDMS or vegetable oil fv and the necessary oil phase, respectively, released in a month. The fv represents the fraction of sunflower oil (fsuno), olive oil (foo) or rapeseed oil (fro) released within a given period. The parameter fv (fPDMS) refers to the ratio of the volume of vegetable oil (PDMS oil) released to the initial volume of vegetable oil (PDMS oil) used. For optical microscopy, a drop of the silica particle-stabilised emulsions was diluted with 1–5 drops of its continuous phase and viewed on a dimple glass slide (Fisher Scientific). The emulsions were viewed using an Olympus BX-51 optical microscope fitted with a DP50 digital camera using Image-Pro Plus 5.1 software (Media Cybernetics). The optical micrographs were used to determine the droplet size of the emulsions by averaging the diameter of ≈300 emulsion droplets.
A small volume of emulsion was pipetted on to a cryo-SEM stub (diameter ∼10 mm) and plunged into liquid nitrogen slush at −210 °C. The frozen sample was transferred to the cryo-SEM preparation chamber (PP3010T, Quorum Technologies Ltd) where it was fractured and sputter-coated with platinum to a thickness of ∼2 nm. The sample was then transferred to a Zeiss EVO 60 scanning electron microscope chamber for viewing and imaging. Micrographs were taken at a voltage of 20 kV and a probe current of 70 pA.
(v) Preparation of oil-particle dispersions.
PDMS and vegetable oil dispersions were prepared containing 2 wt% and 5 wt% of the DCDMS-coated fumed silica particles. The required mass of 100 and 14% SiOH silica was placed on the surface of the PDMS or vegetable oils (5 cm3) in screw cap glass vials (i.d. of 1.8 cm and height of 7.2 cm). The glass vials were hand shaken vigorously for 10 s. after the particles had completely entered the oils at rest.
All experiments were carried out at room temperature (22 ± 2 °C) unless stated otherwise.
Results and discussion
We first describe the stabilisation of emulsions of vegetable oil and silicone oil by DCDMS-coated fumed silica particles and compare the findings with those expected from a consideration of the calculated oil–oil-particle contact angle. This is followed by a description of the behaviour of emulsions stabilised by TDF-coated silica particles. Finally, the study is extended to the use of a variety of other particle types differing in size, shape and chemical composition.
1. Simple and multiple emulsions stabilised by DCDMS-coated silica particles
(a) Experimental findings.
Equal volumes of two oils were stirred vigorously and left to separate in order to assess their mutual solubility. A photograph of glass vials containing these oil–oil mixtures is shown in Fig. 1. All the vegetable oils were completely immiscible with the PDMS oils (<0.5 vol%). By contrast, castor oil and mineral oil were reasonably miscible with the PDMS oils and we did not pursue these mixtures further. We prepared emulsions of equal volumes of two oils in the presence of 1 wt% of DCDMS-coated silica particles (not pre-dispersed in either oil) which vary in the % SiOH on their surfaces. The appearance of the vessels one month after preparation is given in Fig. 2 for sunflower oil (60 cS) or olive oil (80 cS) in combination with 20 cS PDMS oil. For the most hydrophilic particles (100% SiOH) and relatively hydrophilic and relatively hydrophobic particles (88–25% SiOH), emulsions were of the silicone oil (S)-in-vegetable oil (V) type, S/V. However they were extremely unstable to coalescence and exhibited complete phase separation within several days, Fig. S1 (ESI†). For particles possessing 23% SiOH on their surfaces, stable simple S/V emulsions formed with sunflower oil (and for 20% SiOH) and stable multiple vegetable-in-silicone-in-vegetable, V/S/V, emulsions formed with olive oil. Transitional phase inversion then occurs such that for very hydrophobic particles possessing 14% SiOH (sunflower oil) or either 20 or 14% SiOH (olive oil), stable vegetable oil-in-silicone oil, V/S, emulsions form. The most stable emulsions to both creaming and coalescence were those stabilised by the most hydrophobic particles (14% SiOH). Optical micrographs of a selection of these emulsions are shown in Fig. 3 for both vegetable oils. The formation of multiple emulsions in a single emulsification step in the presence of only one type of fumed silica particle has been observed previously.44
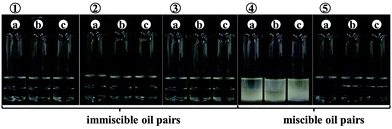 |
| Fig. 1 Photo' of vessels 1 day after stirring equal volumes of (1) sunflower oil, (2) rapeseed oil, (3) olive oil, (4) castor oil and (5) mineral oil and PDMS of (a) 20, (b) 50 and (c) 100 cS at 25 °C. Apart from the castor oil–20 and 50 cS PDMS systems, the upper phase is vegetable oil whilst the lower phase is PDMS oil. | |
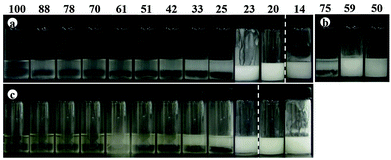 |
| Fig. 2 Photos after 1 month of glass vials containing (a and b) sunflower oil–20 cS PDMS (1 : 1) emulsions stabilised by 1 wt% of (a) DCDMS-coated and (b) TDF-coated fumed silica particles and (c) olive oil–20 cS PDMS (1 : 1) emulsions stabilised by 1 wt% DCDMS-coated fumed silica particles. The fumed silica particles have different % SiOH on their surfaces (given). Dashed lines indicate transitional phase inversion. | |
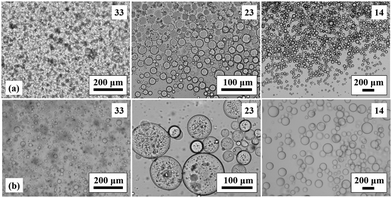 |
| Fig. 3 Corresponding optical micrographs of the emulsions shown in Fig. 2 at selected % SiOH (given) for (a) sunflower oil and (b) olive oil with DCDMS-coated particles. | |
Transitional phase inversion is also observed for emulsions of sunflower or olive oil and 50 cS PDMS seen in Fig. 4. Phase inversion from S/V to V/S emulsions occurs for particles possessing between 23 and 20% SiOH. For both vegetable oils in this case, S/V emulsions stable to coalescence were obtained with particles containing between 88 and 23% SiOH on their surfaces. They released a small fraction of vegetable oil within a month through sedimentation however, with stability increasing towards phase inversion, Fig. S2 (ESI†). We note that an S/V emulsion stable to coalescence is formed with sunflower oil and particles containing 100% SiOH groups, i.e. even for inherently hydrophilic particles, although the corresponding emulsion is unstable to coalescence in the case of olive oil. Similarly, V/S emulsions stabilised by particles possessing 20% SiOH groups were stable to both coalescence and creaming. Also, a V/S emulsion stable to coalescence forms with the most hydrophobic particles (14% SiOH) and sunflower oil, although the corresponding emulsion with olive oil is an unstable multiple one (V/S/V). A similar trend was observed for the more viscous 100 cS PDMS oil systems, with emulsion phase inversion again occurring for particles possessing between 23 and 20% SiOH on their surfaces, Fig. 5 and Fig. S2 (ESI†). The relationship between the average droplet diameter of the S/V emulsions and the % SiOH on particle surfaces is shown in Fig. 6. Although there are a few exceptions, the droplet size decreases as the % SiOH decreases approaching phase inversion in line with the increase in emulsion stability. Silicone oil droplets are in general smaller for the higher viscosity PDMS oil.
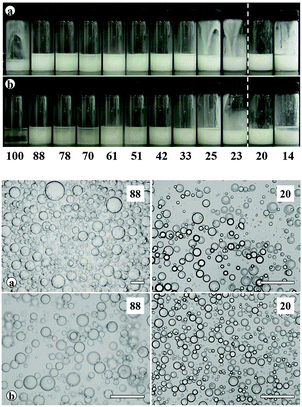 |
| Fig. 4 (upper) Photographs of glass vials containing (a) sunflower oil–50 cS PDMS and (b) olive oil–50 cS PDMS (both 1 : 1) emulsions after 1 month stabilised by 1 wt% of DCDMS-coated fumed silica particles with different % SiOH on their surfaces (given). Emulsions invert from S/V to V/S around dashed line. (lower) Corresponding optical micrographs of the emulsions at selected % SiOH (given). Scale bars = 200 μm. | |
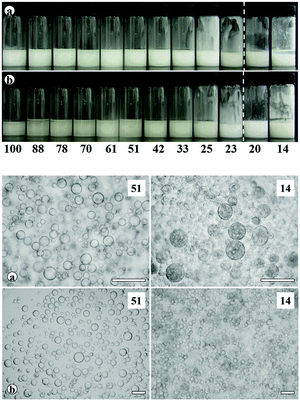 |
| Fig. 5 (upper) Photographs of glass vials containing (a) sunflower oil–100 cS PDMS and (b) olive oil–100 cS PDMS emulsions (both 1 : 1) after 1 month stabilised by 1 wt% of DCDMS-coated fumed silica particles with different % SiOH on their surfaces (given). Dashed line indicates transitional phase inversion. (lower) Corresponding optical micrographs of the emulsions at selected % SiOH (given). Scale bars = 200 μm. | |
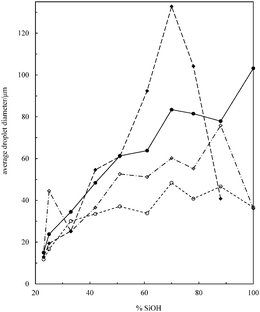 |
| Fig. 6 Plot of average droplet diameter of 50 cS PDMS-in-sunflower oil (●), 100 cS PDMS-in-sunflower oil (○), 50 cS PDMS-in-olive oil (◆) and 100 cS PDMS-in-olive oil (⋄) emulsions after 1 month versus % SiOH on DCDMS-coated fumed silica particle surfaces. Standard deviation is in the range of 30 μm at relatively high % SiOH and 10 μm at relatively low % SiOH. | |
The formation of stable emulsions with fumed silica particles having a relatively high percentage of SiOH groups, e.g. 88–70%, was not anticipated based on existing literature (Table 1). The stabilisation of emulsions in the presence of these particles might be due to the tendency of the particles to behave more hydrophobically in the oils due to a reduction in the effective SiOH content on the particles surfaces as a result of gel formation via hydrogen bonds between silanol groups.45 In order to verify this, oil dispersions were prepared in both types of oil for completely hydrophilic (100% SiOH) and the most hydrophobic (14% SiOH) fumed silica particles. Photographs of the glass vials containing the oil dispersions are shown in Fig. S3 (ESI†). The PDMS oil dispersions containing 2 wt% of 100% SiOH fumed silica were fluid, but those containing 5 wt% of the particles gelled (a). The viscosity of the gels was seen visually to increase with the viscosity of the PDMS oil. On the contrary, the vegetable oil dispersions were fluid at both particle concentrations (b). For the 14% SiOH fumed silica particles, no gel was obtained with either the PDMS oils or the vegetable oils up to 5 wt% of the particles (c). These findings indicate that the silica particles are interacting more (via hydrogen bonding) in the PDMS oils than in the vegetable oils. Microscopic oil droplets can be trapped in an oil-particle gel contributing to the overall stability the emulsions. This is different in water where solvation of particle surfaces prevents the formation of hydrogen bonds between particles such that particles behave hydrophilically.45
For some particle-stabilised emulsions incorporating water, the initial location of the particles in oil or water dictates the type of the final emulsion, argued in terms of the hysteresis of contact angle at the three-phase line.46,47 As such, the effect of the initial location of the fumed silica particles in the oil phases was also investigated here for selected particles. DCDMS-coated fumed silica particles possessing either 51% or 14% SiOH were used with the oils. Because the oils wet these particles spontaneously, the required amount (0.2 g) of particles was placed on the surface of either vegetable oil or PDMS oil (5 cm3). The particles were wetted completely by the oils and entered in them before the second oil phase was added. Photographs and the corresponding optical micrographs of the simple oil-in-oil emulsions prepared are given in Fig. S4 (ESI†). For all the vegetable oil–PDMS oil combinations studied, the emulsion type is independent of the initial location of the particles. Their ensuing stability and average drop size are also very similar and not affected by which oil contacts the particles first.
As in the case of surfactant-stabilised emulsions where two surfactants of different hydrophile–lipophile balance number are required for the stabilisation of multiple emulsions,48 two particle types of different wettability are normally required for the stabilisation of multiple Pickering emulsions.49,50 Kinetically stable multiple emulsions of both types (oil-in-water-in-oil and water-in-oil-in-water) have been prepared using a mixture of hydrophilic and hydrophobic silica particles (for inner drops and outer globules) in a two stage emulsification method.49 In this study, we have seen that particles possessing relatively high percentages of SiOH groups prefer to stabilise vegetable oil-continuous emulsions whilst those with 20 or 14% SiOH prefer to stabilise silicone oil-continuous emulsions. Using a two stage emulsification method exploiting this variation of emulsion type with particle type, oil-in-oil-in-oil multiple emulsions were prepared stabilised by a mixture of 51 and 14% SiOH particles. The concentration of these particles in the multiple emulsions was approx. 1 wt%. A V/S emulsion stabilised by 14% SiOH particles was stirred at 500 rpm with a vegetable oil dispersion of 51% SiOH particles resulting in a V/S/V multiple emulsion stable to coalescence for up to one year. If however high shear was used (6000 rpm via Ultra-Turrax homogeniser) only a simple S/V emulsion formed as a result of catastrophic phase inversion of the precursor V/S emulsion. A photo' of the glass vials containing the V/S/V emulsions prepared using low shear and their corresponding optical micrographs are shown in Fig. 7. The V/S/V multiple emulsions contain different volume fractions of the initial V/S emulsions. On the contrary, in attempts to prepare S/V/S emulsions, gently stirring S/V emulsions stabilised by 2 wt% of 51% SiOH silica into a silicone oil dispersion of 14% SiOH silica only produced unstable V/S emulsions (Fig. 7).
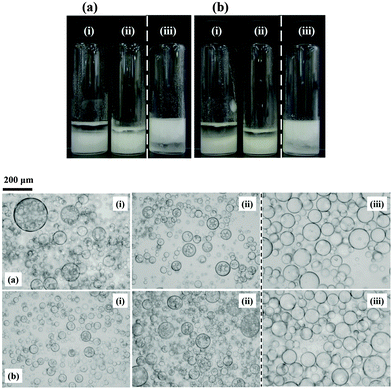 |
| Fig. 7 (upper) Photographs after 1 month of (a) sunflower oil-in-50 cS PDMS-in-sunflower oil and (b) olive oil-in-50 cS PDMS-in-olive oil multiple emulsions containing a volume fraction of initial V/S emulsion of (i) 0.3 and (ii) 0.7 prepared using low shear; (iii) simple V/S emulsions containing the vegetable oils and 50 cS PDMS after attempting to prepare S/V/S multiple emulsions with low shear. (lower) Corresponding optical micrographs of the emulsions. | |
(b) Calculated oil–oil-particle contact angles and link to emulsion type.
It is very difficult to measure the contact angle small solid particles make with fluid interfaces especially if the particles are agglomerated and polydisperse like the fumed silica particles used in this work. We thus use a previously derived theory51 for estimating the vegetable oil–silicone oil–silica particle contact angle θo1o2 through the PDMS phase starting with Young's equation | 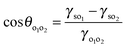 | (1) |
The oil–oil interfacial tension γo1o2 is obtained from experiment and is quite low (Table 3). The symbols γso1 and γso2 represent the silica–vegetable oil and silica–PDMS oil interfacial energies respectively given in eqn (2) and (3).52 |  | (2) |
|  | (3) |
Here, γsa, γpsa and γdsa represent the total surface energy and the polar and the dispersion components of the surface energy of the solid surface in air respectively. Likewise, γo, γPo and γdo represent the surface tension of the oil and its polar and dispersion components respectively (Table 2). It is anticipated that in a system without water, the magnitude of any polar component will be crucial. In order to calculate θo1o2 as a function of the % SiOH on silica surfaces, we choose γsa of 100% SiOH to be that of a clean silica glass surface and γsa of 0% SiOH (unobtainable experimentally) to be that of a high molecular weight PDMS itself as discussed earlier.51 We further assume that both dispersion and polar components of γsa are linearly related to the percentage of silanol groups on the surfaces. Using this approach, we calculate the oil–oil-particle contact angle for the system sunflower oil–20 cS PDMS–DCDMS-coated silica relating to Fig. 2(a). However, since values of the polar and dispersion components of the surface tensions of the oils are not known precisely, we decided to explore how the contact angle depended on their exact magnitude around the values quoted in the literature. In Fig. 8(a), the contact angle was calculated for fixed values of γpo and γdo for PDMS taken from literature51 but where those for sunflower oil vary. As seen, the contact angle increases in general with an increase in the value of % SiOH on particle surfaces (contrast for oil–water through water where it decreases44) and is very sensitive to the magnitude of the polar contribution of the vegetable oil. Likewise, in Fig. 8(b) fixed values of γpo and γdo were used for sunflower oil whilst those for PDMS vary. The calculations reveal that θo1o2 increases with increasing γpo of sunflower oil but decreases with increasing γpo of PDMS oil. Although these contact angles predict V/S emulsions (θo1o2 < 90°) at low % SiOH and S/V emulsions (θo1o2 > 90°) at high % SiOH as we find experimentally, the % SiOH predicted for phase inversion at θo1o2 = 90° clearly depends on the choice of the polar and dispersion contributions to the surface tension of the two oils. However, the combination γpo = 1.0 mN m−1 and γdo = 32.3 mN m−1 for sunflower oil yields θ < 90° for 14% SiOH and θ > 90° for 20% SiOH in-line with the respective emulsions found experimentally. These values are not too distant from those in the literature given in Table 2, bearing in mind the doubtful accuracy of the latter.
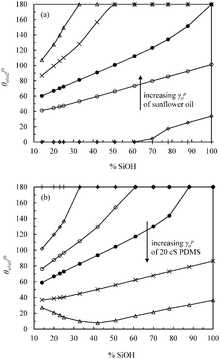 |
| Fig. 8 Plot of calculated θo1o2 into PDMS versus % SiOH on DCDMS-coated fumed silica particle surfaces for the interface sunflower oil–20 cS PDMS with (a) γpo; γdo = 0; 33.3 (+), 0.2; 33.1 (⋄), 0.4; 32.9 (○), 0.6; 32.7 (●), 1.0; 32.3 (×) and 1.4; 31.9 (△) mN m−1 for sunflower oil and γpo; γdo = 0.9; 19.9 mN m−1 for PDMS and (b) γpo; γdo = 0; 20.8 (+), 0.2; 20.6 (⋄), 0.4; 20.4 (○), 0.6; 20.2 (●), 1.0; 19.8 (×) and 1.4; 19.4 (△) mN m−1 for PDMS and γpo; γdo = 0.4; 32.9 mN m−1 for sunflower oil. | |
In order to mimic the surface of the most hydrophilic (100% SiOH) and most hydrophobic (14% SiOH) particles, we prepared completely hydrophilic glass slides and glass slides coated with the highest density of DCDMS groups respectively, and measured the contact angles of the individual oils in air and of a drop of PDMS oil under vegetable oil (θo1o2) on these substrates, Table 4. All oils wet the hydrophilic substrate in air as expected (θo < 5°). For hydrophilic slides, θo1o2 is around 150° implying the substrate is more wet by vegetable oil. For hydrophobic slides, the advancing θo1o2 is around 70° and the more wetting oil is now PDMS. In line with these measurements for every oil–oil combination investigated, the preferred emulsion type (1
:
1) is S/V for relatively hydrophilic particles and V/S or S/V/S for more hydrophobic ones (last column in Table 4). The findings are consistent with the principle put forward by Finkle et al.54 for oil–water-particle systems which stated that the liquid wetting the particles less becomes the dispersed phase. This is the first time this principle has been verified in oil–oil-particle systems.
Table 4 Contact angle (through oil) of the oils in air and advancing (first entry) and receding (second entry) contact angle (through PDMS) of PDMS oil drops under vegetable oil measured on either completely hydrophilic or hydrophobic DCDMS-coated glass slides. Also given is the type of emulsion formed in vegetable oil–PDMS oil 1
:
1 mixtures stabilised by 100% SiOH or 14% SiOH silica particles respectively
Glass surface |
Fluid pair |
Contact angle/° |
Emulsion type |
Contact angle is through water.
|
Hydrophilic |
Water–air |
<5a |
|
Sunflower oil–air |
<5 |
|
Olive oil–air |
<5 |
|
20 cS PDMS–air |
<5 |
|
50 cS PDMS–air |
<5 |
|
100 cS PDMS–air |
<5 |
|
Sunflower oil–water |
52, 32a |
Not studied |
Olive oil–water |
43, 19a |
Not studied |
Sunflower oil–20 cS PDMS |
150, 150 |
Unstable S/V |
Sunflower oil–50 cS PDMS |
148, 160 |
Stable S/V |
Sunflower oil–100 cS PDMS |
152, 158 |
Stable S/V |
Olive oil–20 cS PDMS |
148, 151 |
Unstable S/V |
Olive oil–50 cS PDMS |
148, 150 |
Unstable S/V |
Olive oil–100 cS PDMS |
152, 154 |
Stable S/V |
|
Hydrophobic |
Water–air |
107, 110a |
|
Sunflower oil–air |
52, 35 |
|
Olive oil–air |
58, 34 |
|
20 cS PDMS–air |
<5 |
|
50 cS PDMS–air |
<5 |
|
100 cS PDMS–air |
<5 |
|
Sunflower oil–water |
168, 171a |
Not studied |
Olive oil–water |
167, 169a |
Not studied |
Sunflower oil–20 cS PDMS |
67, 44 |
Stable V/S |
Sunflower oil–50 cS PDMS |
72, 34 |
Stable V/S |
Sunflower oil–100 cS PDMS |
81, 42 |
Unstable S/V/S multiple emulsion |
Olive oil–20 cS PDMS |
70, 36 |
Stable V/S |
Olive oil–50 cS PDMS |
82, 32 |
Unstable S/V/S multiple emulsion |
Olive oil–100 cS PDMS |
71, 45 |
Stable V/S |
2. Emulsions stabilised by TDF-coated silica particles
(a) Effect of particle concentration.
We recently reported the influence on particle hydrophobicity of replacing the DCDMS coating on fumed silica particle surfaces by a perfluoroalkyl coating.42 For the same residual SiOH content, fluoro-coated particles are more hydrophobic than DCDMS-coated ones and so should be more effective as stabilisers of oil–oil emulsions. For fluoro particles with 75% SiOH, the effect of particle concentration on emulsions of equal volumes of various vegetable oils and 50 cS PDMS has been investigated. Fig. 9 shows their appearance one month after preparation. In the absence of particles, all vegetable oil–PDMS emulsions phase separated completely within an hour after homogenisation. With particles, preferred emulsions were S/V throughout, as in the case of DCDMS-coated particle emulsions of similar silanol content (78%). The stability of S/V emulsions to coalescence increases markedly between 0.5 and 1.0 wt% particles, after which emulsions showed no signs of coalescence for months. Their stability to sedimentation (releasing vegetable oil) increases progressively with particle concentration, Fig. 10(a), such that by 2 wt% emulsions were completely stable. As expected, the average emulsion drop size decreases to a more or less constant value with increasing particle concentration, Fig. 10(b). Similar trends in emulsion stability and drop diameters were found for S/V emulsions containing 100 cS PDMS (Fig. S5, ESI†). However, in the case of 20 cS PDMS, emulsions were of the opposite type, V/S, and less stable to coalescence overall compared with those made of higher viscosity PDMS (Fig. S6, ESI†). Nonetheless, their stability to creaming (releasing PDMS) and coalescence (releasing vegetable oil) increases with particle concentration. We also note that emulsions of sunflower oil and 20 cS PDMS stabilised by fluoro particles possessing 59% or 50% SiOH (i.e. less hydrophobic) were also V/S and stability to both creaming and coalescence improved with decreasing silanol content (see Fig. 2(b)). The change in emulsion type with PDMS viscosity is a form of transitional phase inversion. For PDMS oils of increasing viscosity, the average molecular mass of the molecules comprising the oil increases leading to entanglement. Their surface tension with air and their interfacial tension with water both increase slightly with oil viscosity. It may be that through these changes the contact angle of silica particles at the vegetable oil–PDMS interface varies depending on the viscosity of PDMS rendering particles more oleophilic towards vegetable oil as viscosity increases. The only evidence we have relating to this is the advancing contact angle through PDMS of a PDMS oil drop under sunflower oil on a hydrophobic glass substrate (Table 4), which increases from 67° to 81° as the PDMS viscosity increases from 20 to 100 cS. That is, the surface becomes more vegetable oil-liking with PDMS viscosity in line with the tendency to prefer S/V emulsions. In the absence of particles, temporarily stable emulsions are V/S for 20 and 50 cS PDMS and S/V for 100 cS PDMS. This is in agreement with the rule of thumb that the fluid of lowest viscosity tends to become the continuous phase, since the viscosity of sunflower oil at room temperature is 60 cS. It thus appears that the type of particle-stabilised emulsion is also influenced by the relative viscosities of the two oils.
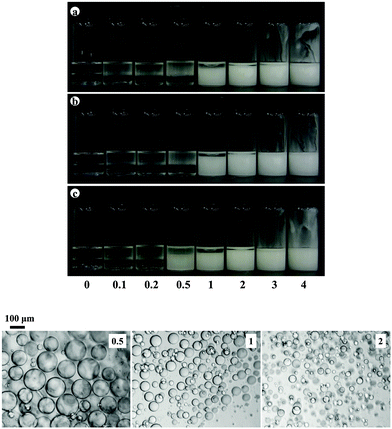 |
| Fig. 9 (upper) Photographs of glass vials containing 50 cS PDMS-in-(a) sunflower oil, (b) olive oil or (c) rapeseed oil emulsions (1 : 1) 1 month after preparation stabilised by different concentrations (given, wt%) of 75% SiOH fluorinated fumed silica particles. (lower) Corresponding optical micrographs of the emulsions in (a) at selected particle concentrations (given, wt%). | |
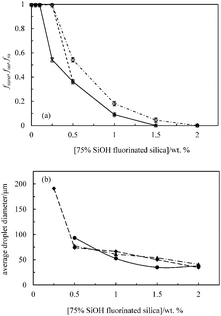 |
| Fig. 10 (a) Fraction of sunflower oil fsuno (○) or olive oil foo (⋄) or rapeseed oil fro (×) released from 50 cS PDMS-in-sunflower oil, 50 cS PDMS-in-olive oil or 50 cS PDMS-in-rapeseed oil emulsions, respectively, shown in Fig. 9versus concentration of 75% SiOH fluorinated silica particles. (b) Average droplet diameter after 1 month of PDMS drops in sunflower oil (●), olive oil (▲) or rapeseed oil (◆) emulsions versus particle concentration. The standard deviation decreases from ∼40 μm at 0.25 wt% to ∼10 μm at 2 wt%. | |
(b) Catastrophic phase inversion.
In the presence of 1 wt% of 75% SiOH fluorosilica particles, we explored whether catastrophic phase inversion was possible in batch emulsions upon varying the volume fraction of vegetable oil ϕsuno in the system sunflower oil–50 cS PDMS. As seen in Fig. 11(a) and (b), at ϕsuno ≤ 0.4 the preferred emulsions are V/S. For ϕsuno = 0.05 and 0.1, all the sunflower oil becomes incorporated into the emulsion which appears below the silicone oil after 1 month, in which the droplets are particularly non-spherical and heavily aggregated. The relatively dense fluorinated particles serve to raise the density of the sunflower oil coated by them. Catastrophic phase inversion of emulsions occurs at ϕsuno > 0.4 yielding S/V emulsions. Importantly, this demonstrates that both emulsion types can be stabilised in oil–oil mixtures using one and the same particles, not yet achievable with surfactants at fixed temperature, salt concentration or pH. The stability of the V/S emulsions to creaming for ϕsuno ≥ 0.1 decreases approaching inversion, whilst that of the S/V emulsions to sedimentation increases (Fig. 11(c)). All emulsions however were stable to coalescence for over a year, as seen earlier in oil–water emulsions stabilised by fumed silica particles.55 We verified that the average drop size passes through a maximum around the conditions for phase inversion; since the particle concentration with respect to the dispersed phase increases progressively either side of inversion, smaller drops can be stabilised.
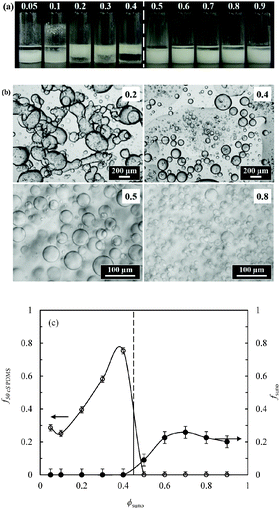 |
| Fig. 11 (a) Photograph after 1 month of glass vials containing sunflower oil–50 cS PDMS emulsions stabilised by 1 wt% of 75% SiOH fluorinated silica particles containing varying volume fraction of sunflower oil ϕsuno (given). Emulsions are V/S (left) and S/V (right) either side of the dashed line. (b) Corresponding optical micrographs of the emulsions at selected ϕsuno (given). (c) Stability of the emulsions in terms of f50 cS PDMS and fsuno released 1 month after preparation. | |
3. Emulsions stabilised by other types of particle
In order to ascertain how general the findings obtained using fumed silica particles are, we have studied emulsions stabilised by a wide range of different particle types, shape and size for the mixture sunflower oil–PDMS (1
:
1). The majority of the particles are hydrophobic imparted by either long hydrocarbon or fluorocarbon chains. The PF-5 Eight Pearl 300S-Al powder comprises partially fluorinated sericite platelet particles ca. a few μm long and several nm thick.7 The influence of particle concentration on the appearance of emulsions containing 20 cS PDMS is shown in Fig. 12(a). Preferred emulsions are V/S and their stability to both creaming and coalescence increases with particle concentration, Fig. 12(b). Similar trends in behaviour are witnessed with the same particles and both olive oil–PDMS emulsions (Fig. S7 and S8, ESI†) and rapeseed oil–PDMS emulsions (Fig. S9 and S10, ESI†), where it can be seen that around 1 wt% of particles is required for emulsions stable to coalescence.
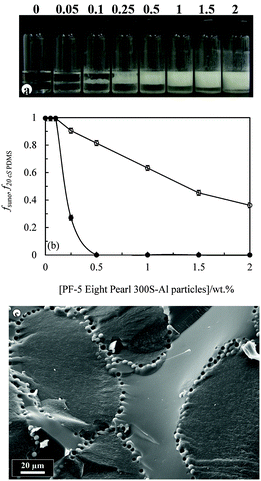 |
| Fig. 12 (a) Photographs of glass vials containing sunflower oil-in-20 cS PDMS emulsions (1 : 1, 1 month after preparation) stabilised by different concentrations (given, wt%) of PF-5 Eight Pearl 300S-Al particles, (b) fraction of sunflower oil fsuno (●) and fraction of PDMS oil f20 cS PDMS (○) released from above emulsions versus particle concentration, (c) cryo-SEM image of a sunflower oil-in-50 cS PDMS emulsion stabilised by 1 wt% of silicone particles of PF-5 Tospearl 145A. | |
Emulsions comprising equal volumes of sunflower oil and 20 cS PDMS oil were also prepared in the presence of 1 wt% of either (i) hydrophilic rod-shaped CaCO3 particles (25 μm, Whiscal A) or platelet bentonite particles (>100 μm, PFX-10 Kunipia F) or (ii) hydrophobic spherical silicone particles (4.5 μm, PF-5 Tospearl 145A), platelet sericite particles (few μm, PF-10 FSE Al), spherical ZnO particles (few μm, PFX-10 ZnO (TP)), platelet bentonite particles (2–30 μm, Bentone 34), spherical CaCO3 particles (0.1 μm, Calofort SV) and irregular shaped PTFE particles (1–10 μm, Zonyl MP1400). As seen in Fig. S11 (ESI†), the hydrophilic particles stabilised S/V emulsions temporarily but these exhibited complete phase separation within several days as expected. Hydrophobic particles however stabilised V/S emulsions which, apart from those containing Bentone 34, were stable to coalescence for at least one year. The cryo-SEM image in Fig. 12(c) is that of a V/S emulsion stabilised by spherical particles of fluorinated silicone resin. The two oil phases are distinguished from each other by their different texture upon freezing; vegetable oil is relatively rough compared with silicone oil which is smooth. Particles are entirely at the interface of the droplets and relatively close-packed. The appearance of spherical voids on interfaces where particles were originally located suggests these particles are dislodged from the interface during either the freezing or fracturing of the emulsion. This brief study extends what we discovered with silica particles to a wider range of systems and reinforces our earlier findings that hydrophobic particles of different size and shape are effective stabilisers of oil-in-oil emulsions.
Conclusions
By systematically varying the inherent hydrophobicity of fumed silica particles in mixtures of immiscible vegetable oil and silicone oil, we find that emulsions are S/V for relatively hydrophilic particles but V/S for very hydrophobic particles. Close to the inversion condition, emulsions are stable to coalescence and gravity-induced separation and of minimum drop size. Predictions of emulsion type based on calculated contact angles of a particle at the oil–oil interface are in good agreement with experiment providing a small polar contribution is included in the surface tension of the oils. By utilising two particle types of different hydrophobicity, we prepare a multiple V/S/V emulsion in which more hydrophobic particles coat inner droplets and more hydrophilic particles coat outer globules. Importantly, catastrophic phase inversion from V/S to S/V emulsions can be effected by increasing the volume fraction of vegetable oil, demonstrating that both emulsion types can be stabilised with one and the same particles. For a range of hydrophobic particles of different chemical type, size and shape, we confirm that they can also be efficient stabilisers of V/S emulsions.
Acknowledgements
We are grateful to the Tertiary Education Trust Fund of Nigeria and the University of Hull for a studentship to ATT and Mr A. Sinclair for carrying out SEM analysis.
References
-
Colloidal Particles at Liquid Interfaces, ed. B. P. Binks and T. S. Horozov, Cambridge University Press, Cambridge, 2006 Search PubMed.
- B. P. Binks and R. Murakami, Nat. Mater., 2006, 5, 865–869 CrossRef CAS PubMed.
- L. Forny, I. Pezron, K. Saleh, P. Guigon and L. Komunjer, Powder Technol., 2007, 171, 15–24 CrossRef CAS.
- B. O. Carter, W. Wang, D. J. Adams and A. I. Cooper, Langmuir, 2010, 26, 3186–3193 CrossRef CAS PubMed.
- R. Murakami and A. Bismarck, Adv. Funct. Mater., 2010, 20, 732–737 CrossRef CAS.
- B. P. Binks, T. Sekine and A. T. Tyowua, Soft Matter, 2014, 10, 578–589 RSC.
- B. P. Binks, S. K. Johnston, T. Sekine and A. T. Tyowua, ACS Appl. Mater. Interfaces, 2015, 7, 14328–14337 CAS.
- E. Bormashenko, Curr. Opin. Colloid Interface Sci., 2011, 16, 266–271 CrossRef CAS.
- A. Hashmi, A. Strauss and J. Xu, Langmuir, 2012, 28, 10324–10328 CrossRef CAS PubMed.
- G. McHale and M. I. Newton, Soft Matter, 2015, 11, 2530–2546 RSC.
- N. M. Oliveira, C. R. Correia, R. L. Reis and J. F. Mano, Adv. Healthcare Mater., 2015, 4, 264–270 CrossRef CAS PubMed.
- R. Murakami, H. Moriyama, M. Yamamoto, B. P. Binks and A. Rocher, Adv. Mater., 2012, 24, 767–771 CrossRef CAS.
- J. Tang, P. J. Quinlan and K. C. Tam, Soft Matter, 2015, 11, 3512–3529 RSC.
- M. V. Tzoumaki, D. Karefyllakis, T. Moschakis, C. G. Billaderis and E. Scholten, Soft Matter, 2015, 11, 6245–6253 RSC.
- B. P. Binks, A. Rocher and M. Kirkland, Soft Matter, 2011, 7, 1800–1808 RSC.
- B. T. Nguyen, T. Nicolai and L. Benyahia, Langmuir, 2013, 29, 10658–10664 CrossRef CAS PubMed.
- R. V. Petersen and R. D. Hamill, J. Soc. Cosmet. Chem., 1968, 19, 627–640 CAS.
- A. Imhof and D. J. Pine, J. Colloid Interface Sci., 1997, 192, 368–374 CrossRef CAS.
- A. K. F. Dyab and A. M. Atta, RSC Adv., 2013, 3, 25662–25665 RSC.
- B. P. Binks, P. D. I. Fletcher, M. A. Thompson and R. P. Elliott, Langmuir, 2013, 29, 5723–5733 CrossRef CAS PubMed.
- P. S. Clegg, E. M. Herzig, A. B. Schofield, S. U. Egelhaaf, T. S. Horozov, B. P. Binks, M. E. Cates and W. C. K. Poon, Langmuir, 2007, 23, 5984–5994 CrossRef CAS PubMed.
- J. Vermant, G. Cioccolo, K. Golapan Nair and P. Moldenaers, Rheol. Acta, 2004, 43, 529–538 CrossRef CAS.
- P. Thareja and S. Velankar, Rheol. Acta, 2007, 46, 405–412 CrossRef CAS.
- L. Elias, F. Fenouillot, J. C. Majeste and P. Cassagnau, Polymer, 2007, 48, 6029–6040 CrossRef CAS.
- L. Elias, F. Fenouillot, J. C. Majesté, P. Alcouffe and P. Cassagnau, Polymer, 2008, 49, 4378–4385 CrossRef CAS.
-
R. D. Vartanian, Stable mineral oil-silicone oil compositions, US Pat., 3445385, American Cyanamid Company, 1969 Search PubMed.
-
A. Guillaume and F. Sagi, Homogeneous dispersions of diorganopolysiloxane compositions in mineral oils, US Pat., 4115343, Rhone-Poulenc Industries, 1978 Search PubMed.
-
G. H. Greene, J. C. Phillips and J. F. Stults, Non-aqueous emulsion of silicone oil and stearine, WO1991010771 A1, Karlshamns AB, 1991 Search PubMed.
- X.-D. Pan and G. H. McKinley, J. Colloid Interface Sci., 1997, 195, 101–113 CrossRef CAS.
- J. C. Loudet, H. Richard, G. Sigaud and P. Poulin, Langmuir, 2000, 16, 6724–6730 CrossRef CAS.
-
Z. Lin, W. J. Schulz Jr. and J. M. Smith, Oil-in-oil and three-phase emulsions, US Pat., 6238657 B1, Dow Corning Corporation, 2001 Search PubMed.
- Y. Nonomura, T. Sugawara, A. Kashimoto, K. Fukuda, H. Hotta and K. Tsujii, Langmuir, 2002, 18, 10163–10167 CrossRef CAS.
- Y. Nonomura, K. Fukuda, S. Komura and K. Tsujii, Langmuir, 2003, 19, 10152–10156 CrossRef CAS.
-
H. Lannibois-Drean, J.-M. Ricca, M. Destarac and P. Olier, Oil-in-oil emulsions comprising a silicone, dispersions and use of said emulsions, WO03/000396 A1, Rhodia Chimie, 2003 Search PubMed.
- V. Jaitely, T. Sakthivel, G. Magee and A. T. Florence, J. Drug Delivery Sci. Technol., 2004, 14, 113–117 CrossRef CAS.
-
M. Nair, T. K. Jones and M. C. Brick, Oil-in-oil emulsions, US Pat., 2007/0189998 A1, Eastman Kodak Company, 2007 Search PubMed.
-
C. Holtze, R. E. Guerra, J. Agresti, D. A. Weitz, K. Ahn, J. B. Hutchison, A. Griffiths, A. El Harrak, O. J. Miller, J.-C. Baret, V. Taly, M. Ryckelynck and C. Merten, Fluorocarbon emulsion stabilizing surfactants, WO2008/021123 A1, Harvard College, 2008 Search PubMed.
-
S. Cauvin, A. Colson, T. Dimitrova and J.-P. Lecomte, WO2010072711 A1, Dow Corning Corporation, 2010.
-
T. K. Jones and M. Nair, Oil-in-oil dispersions stabilised by solid particles and methods of making the same, US Pat., 8323392 B2, Eastman Kodak Company, 2012 Search PubMed.
- S. Verma and J. S. Dangi, Ind. J. Novel Drug Del., 2012, 4, 223–226 Search PubMed.
- B. P. Binks and S. O. Lumsdon, Langmuir, 2000, 16, 8622–8631 CrossRef CAS.
- B. P. Binks and A. T. Tyowua, Soft Matter, 2013, 9, 834–845 RSC.
- C. Huh and S. G. Mason, Colloid Polym. Sci., 1975, 253, 566–580 CAS.
- B. P. Binks, P. D. I. Fletcher, B. L. Holt, P. Beaussoubre and K. Wong, Phys. Chem. Chem. Phys., 2010, 12, 11954–11966 RSC.
- B. P. Binks, J. Philip and J. A. Rodrigues, Langmuir, 2005, 21, 3296–3302 CrossRef CAS PubMed.
- B. P. Binks and S. O. Lumsdon, Phys. Chem. Chem. Phys., 2000, 2, 2959–2967 RSC.
- B. P. Binks and J. A. Rodrigues, Langmuir, 2003, 19, 4905–4915 CrossRef CAS.
- M.-F. Ficheux, L. Bonakdar, F. Leal-Calderon and J. Bibette, Langmuir, 1998, 14, 2702–2706 CrossRef CAS.
-
H. Barthel, B. P. Binks, A. Dyab and P. Fletcher, Multiple Emulsions, US Pat., 2003/0175317 A1, Wacker-Chemie GmbH, 2003 Search PubMed.
- S. Zou, C. Wang, Q. Gao and Z. Tong, J. Dispersion Sci. Technol., 2013, 34, 173–181 CrossRef CAS.
- B. P. Binks and J. H. Clint, Langmuir, 2002, 18, 1270–1273 CrossRef CAS.
- D. K. Owens and R. C. Wendt, J. Appl. Polym. Sci., 1969, 13, 1741–1747 CrossRef CAS.
- M.-C. Michalski, S. Desobry, M.-N. Pons and J. Hardy, J. Am. Oil Chem. Soc., 1998, 75, 447–454 CrossRef CAS.
- P. Finkle, H. D. Draper and J. H. Hildebrand, J. Am. Chem. Soc., 1923, 45, 2780–2788 CrossRef CAS.
- B. P. Binks and S. O. Lumsdon, Langmuir, 2000, 16, 2539–2547 CrossRef CAS.
Footnote |
† Electronic supplementary information (ESI) available. See DOI: 10.1039/c5sm02438b |
|
This journal is © The Royal Society of Chemistry 2016 |