Cytotoxic effects of aspartame on human cervical carcinoma cells
Received
4th August 2015
, Accepted 27th September 2015
First published on 29th September 2015
Abstract
Aspartame is used as an artificial sweetener in more than 6000 food varieties. The present study aims to determine the effects of aspartame at various concentrations on the cell viability, morphology, ROS level and DNA of human cervical carcinoma cells over two time periods of exposure. The effects of aspartame on HeLa cell viability were investigated using the sulphorhodamine-B assay (SRB assay) and flow cytometry. Alkaline comet assay was carried out to determine the possible DNA damage induced by aspartame. Mitochondria-derived reactive oxygen species (ROS) were determined using 2′,7′-dichlorodihydrofluorescein diacetate (DCFH-DA). Fluorescence microscopy was used to determine the presence of apoptotic and necrotic cells following aspartame treatment. Cell viability was significantly altered following a higher concentration of aspartame exposure. Mitochondria-derived ROS increased at higher concentrations of aspartame exposure. Exposure to 10 mM and 20 mM of aspartame induced DNA fragmentation. Apoptotic and necrotic bodies were found in the range of 1–20 mM aspartame exposure. Exposure to high concentrations of aspartame may alter cell viability and morphology, and it may induce ROS generation and DNA damage in cervical carcinoma cells.
Introduction
Aspartame is widely used as an artificial sweetener in several foods1 and is found in low-calorie beverages and desserts.2 It is a dipeptide of aspartyl–phenylalanine methyl ester, and facilitates intestinal hydrolysis and absorption of amino acids together with methanol.3 Aspartic acid and phenylalanine are commonly present in natural proteins.4 Consumption of aspartame has been associated with several behavioral and neurological disturbances.5
Consumption of small amounts of aspartame can increase methanol levels in animals.6 Severe metabolic acidosis and clinical disturbances including blindness, neurological sequelae and death may occur upon accidental and suicidal intake of aspartame.7 Methanol is a substance that can potentially damage liver and kidney cells. Methanol is converted into formaldehyde and formate.8 This process accompanies elevation of NADH level and formation of superoxide anions, which may participate in the process of lipid peroxidation.9 Mitochondrial damage, increased microsomal proliferation and production of oxygen radicals are commonly associated with methanol intoxication.10 Oxidative stress is known as a state in which oxidative force overloads the cellular antioxidant systems due to loss of redox balance. The possibility of increased oxidative stress occurs when the level of production of reactive oxygen species (ROS) exceeds the body's ability to neutralize and eliminate them. Thus, the creation of an imbalance and overabundance of free radicals is reported during these conditions.11 Therefore these factors, found on exposure to excess amounts of formaldehyde formed during acute methanol intoxication, may alter cell viability and cause membrane damage due to increased lipid peroxidation.9
Several studies have evidenced the possible risk of cancer with use of aspartame. The use of high concentrations of artificial sweeteners indicated a potential association with bladder cancer in rodents.12 However, these findings do not correlate with findings in humans.13 There were no neoplasms observed in saccharin-treated rats.14 Andreatta et al.,15 have reported that the prolonged use of artificial sweeteners in combined form results in the formation of urinary tract tumors in Argentina. Furthermore, Sturgeon et al.,16 have reported that heavy artificial sweeteners are known to cause an elevated risk of high-grade tumors. Brain and breast cancers have been associated with the occurrence of these tumors at different sites in rats.13 Higher concentrations of artificial sweeteners have been known to induce DNA damage in rats.14 The selection of HeLa cells used for this study based on the monocytic lineage could mimic local immune responses, and cervical carcinoma is one of the most common neoplastic diseases affecting women worldwide. Therefore, elucidating the mechanism of aspartame in tumor development in humans could provide information on the possible effect on mammalian cells.
The objective of the present study is to determine the effects of aspartame at various concentrations on the cell viability, morphology, ROS level and DNA of human cervical carcinoma cells over two time periods of exposure. SRB assay and flow cytometry analysis were used to investigate the effect of aspartame on cell viability. Comet assay was used to determine possible DNA fragmentation. ROS level was determined using 2′,7′-dichlorodihydrofluorescein diacetate (DCFH-DA). Fluorescence microscopy was used to confirm the presence of apoptotic and necrotic cells.
Materials and methods
Materials
Aspartame, dimethyl sulphoxide (DMSO) and Sulforhodamine B (SRB) were purchased from Sigma. Dulbecco's Modified Eagle's Medium (DMEM), fetal bovine serum (FBS), penicillin–streptomycin and trypsin-EDTA were obtained from Welgene (Daegu, South Korea). Fluorescein diacetate (FDA), propidium iodide (PI) and DCFH-DA were purchased from Santa Cruz Biotechnology, Inc. (Delaware Avenue, California, USA).
Cell culture
Cervical carcinoma cells (HeLa) were purchased from the Korean Cell Line Bank (South Korea). Cells were maintained in growth medium supplemented with 10% FBS and 1% antibiotics (penicillin–streptomycin). The cells were grown in a CO2 incubator at 37 °C and 5% CO2.
Preparation of aspartame
Aspartame stock (1 M concentration) was initially prepared by dissolving in DMEM. The stock solution was filtered using a 0.22 μm filter prior to utilization in the experiment. The stock solution of aspartame was diluted with complete medium to prepare working concentrations and pH was adjusted to 7.4.
SRB assay for cell viability
Cells were seeded at a density of 2 × 104 cells per well and allowed to adhere. Cells were treated with aspartame at different concentrations (100 nM, 1 μM, 10 μM, 100 μM, 1 mM, 10 mM & 20 mM) for 24 and 48 h. At the end of the treatments, the cytotoxic effect of aspartame on HeLa cells was measured by SRB assay.17
Flow cytometry assays for cell viability
HeLa cells were seeded at a density of 2.2 × 105 cells per well in 6-well plates. After 24 h adherence, the cells were treated with aspartame of different concentrations (10 μM, 100 μM, 1 mM, 10 mM & 20 mM) for 24 and 48 h. At the end of treatments, the cells were washed once with PBS and re-suspended in PBS at an initial cell density of 1 × 105 cells ml−1. FDA was added to each tube to attain a final concentration of 0.5 μg ml−1, and the tubes were incubated in the dark for 30 minutes. Then, PI was added to each tube to achieve a final concentration of 50 μg ml−1. All the tubes were kept on ice immediately up to flow cytometry analysis.18
HeLa cell viability was assessed by determining the intracellular green and red fluorescence using a BD FACS Calibur Flow Cytometry System (Becton Dickinson, Franklin Lakes, NJ 07417). A 570 nm beam splitter was used for dual color fluorescence analysis, with a 625 nm filter used to collect red (PI) fluorescence, and a 530 nm filter used for green (FDA) fluorescence. Logarithmic amplification of green and red fluorescence signals was used. Viable cells were identified as those with high green fluorescence.
Alkaline comet assay
The possible DNA damage induced by aspartame at different concentrations (100 μM, 1 mM, 10 mM & 20 mM) was studied after 24 and 48 h time periods by following standard protocols. This assay is a very sensitive and rapid method for the quantification of DNA lesions in animal cells. The assay detects single strand breaks, DNA crosslinking, alkali labile sites, and incomplete excision repair sites.19 The presence of “comets” was observed with a light microscope, and DNA damage was quantitated.
Determination of intracellular ROS level
HeLa cells were seeded in 96-well plates in growth medium at a cell density of 4500 cells per well. After adherence, cells were treated with aspartame of different concentrations (100 μM, 1 mM, 10 mM & 20 mM). At the end of the treatment the medium was removed and the cells were incubated with 5 μM of DCFH-DA in the growth medium for 30 min at 37 °C and 5% CO2. Fluorescence was measured using a fluorescence plate reader at excitation/emission wavelengths of 490 and 525 nm, respectively, and images were taken using a fluorescence microscope (Axiovert 2000, Carl Zeiss, Germany). ROS level was determined using a fluorescent probe, DCFH-DA.20
Fluorescence microscope
Cells were seeded at a density of 2 × 104 cells per well in 6-well plates. After 24 h adherence, the cells were treated with aspartame of different concentrations (1 mM, 10 mM & 20 mM). After 24 and 48 h, cells were removed from the wells and centrifuged at 500g for 3 minutes. The supernatant was withdrawn from the tubes and phosphate buffered saline (PBS) was added to the tubes. Cell volume was adjusted to 105–106 cells ml−1. 95 μl of cell suspension was added to the Microtube, and then 5 μl of AO and EB dye mixture was added to the same tube. The tubes were incubated at 37 °C for 15–30 minutes with protection from light. 10 μl of the cell staining solution was added to a glass slide and covered with a cover glass. Cells were examined with a fluorescence microscope (Axiovert 2000, Carl Zeiss, Germany).20
Statistical analysis
Values are expressed as mean ± SD. The difference between control and aspartame treatment was evaluated using a Student's t-test. A p value less than 0.05 was considered statistically significant.
Results
Effect of aspartame on cell viability measured by SRB assay
Aspartame showed a statistically insignificant cytotoxic effect on HeLa cells and a slight concentration-dependent response relationship was observed. Exposure to high concentrations of aspartame induced cell death up to 12%, whereas lower concentrations of 100 nM, 1 μM, 10 μM, and 100 μM of aspartame induced less than 6% irrespective of exposure period (Fig. 1).
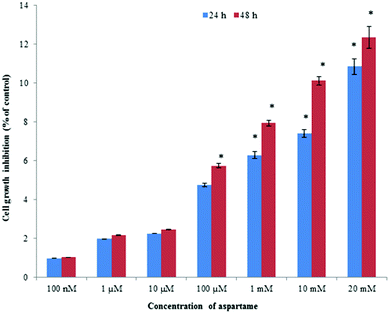 |
| Fig. 1 Cytotoxic effect of aspartame on HeLa cells by SRB assay at 24 and 48 h. Results are presented as percentage of growth inhibition compared with the control. The percentage of growth inhibition was calculated as follows: Growth inhibition (%): (Control − Sample/Control) × 100. Values are expressed as mean ± SD. | |
Effect of aspartame on cell viability measured by flow cytometry
We utilized FDA and PI to determine the numbers of viable and nonviable cells. FDA is a non-polar and non-fluorescent compound. In viable cells, FDA can enter freely; there it is converted by intracellular esterase into a fluorescent compound (fluorescein). Fluorescein is an extremely polar compound, which is trapped within viable cells that possess membrane integrity. On the other hand, fluorescein diffuses out of cells that lack membrane integrity. Viable cells with FDA have bright green fluorescence, whereas nonviable cells are non-fluorescent in flow cytometry analysis. PI is a highly polar and fluorescent substance that can freely enter the nonviable cells where membrane integrity is absent. Therefore, nonviable cells have bright red fluorescence during flow cytometry analysis.
Flow cytometry results are presented in a dot plot on the log scale of green versus red fluorescence. Nonviable cells and debris are separated from the viable cells in the dot plots, which confirms that nonviable cells and debris were not counted in the viable cell window. HeLa cells were incubated with FDA and PI for 30 minutes before measurement. A large population of cells showed FDA fluorescence under controlled conditions (area R1 of Fig. 2 and 3), indicating they were intact active living cells.
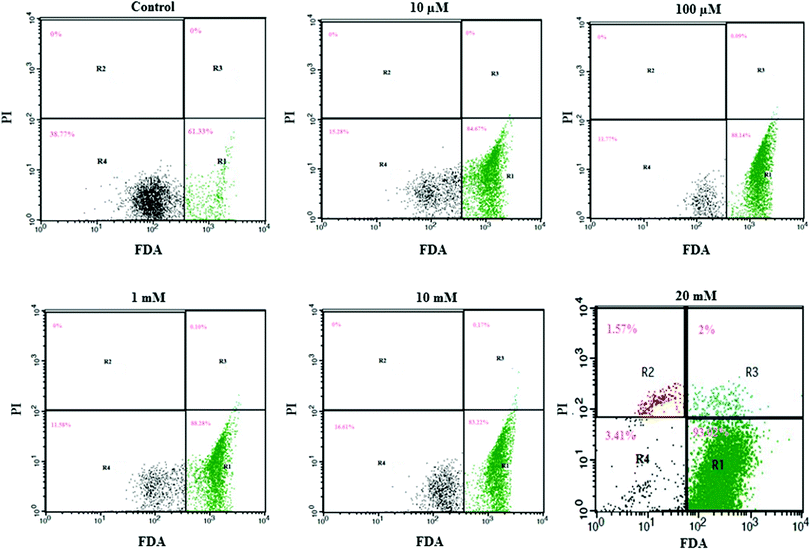 |
| Fig. 2 Effect of aspartame on the HeLa cells discriminated by FDA and PI. Area R1 is FDA positive, R2 is PI positive, R3 is both FDA and PI positive, and R4 is both FDA and PI negative. The effect of aspartame (10 μM, 100 μM, 1 mM, 10 mM & 20 mM) on HeLa cell viability was examined at 24 h. | |
 |
| Fig. 3 Effect of aspartame on the HeLa cells discriminated by FDA and PI. Area R1 is FDA positive, R2 is PI positive, R3 is both FDA and PI positive, and R4 is both FDA and PI negative. The effect of aspartame (10 μM, 100 μM, 1 mM, 10 mM & 20 mM) on HeLa cell viability was examined at 48 h. | |
Exposure to different concentrations of aspartame at 24 h (10 μM, 100 μM, 1 mM, 10 mM & 20 mM) did not produce significant changes in the cell viability. FDA fluorescence in viable cells was more than 90% (area R1 of Fig. 1), and PI fluorescence in nonviable cells was less than 4% (areas R2 & R3 of 20 mM, Fig. 2). After 48h of aspartame exposure, FDA and PI fluorescence were significantly changed in the MDCK cells. The percentages of nonviable (PI) cells were 0.04 ± 0.001, 0.07 ± 0.001, 0.19 ± 0.001, 3.25 ± 0.01 and 3.65 ± 0.01 following 10 μM, 100 μM, 1 mM, 10 mM & 20 mM of aspartame exposure respectively (areas R2 + R3 of Fig. 3). The percentages of viable (FDA) cells were 93.6 ± 2.2, 83.20 ± 1.1, 79.27 ± 1.4, 93.80 ± 1.7 and 45.87 ± 1.5 following 10 μM, 100 μM, 1 mM, 10 mM & 20 mM of aspartame exposure respectively (area R1 of Fig. 3).
Effect of aspartame on DNA damage
The results obtained for the alkaline comet assays for the HeLa cells are shown in Fig. 4. No comet tails were observed in the control and at lower concentrations of aspartame treatment, indicating marginal or no damage to the DNA of the HeLa cells. However after 10 and 20 mM aspartame treatment, long comet tails and tiny head sections could be observed, which indicates severe DNA fragmentation. Cells treated with a low concentration of aspartame yielded no or little DNA fragmentation at 24 and 48 h. Elongated tail formation after cells were treated with aspartame indicated severe DNA fragmentation after 24 and 48 h of incubation (Fig. 4).
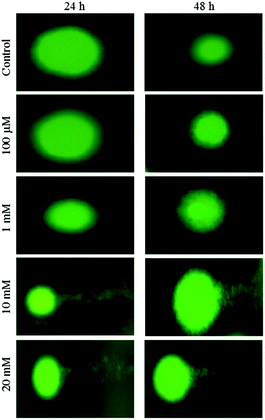 |
| Fig. 4 Effect of aspartame on DNA fragmentation. HeLa cells were grown in 6-well plates and allowed to adhere for 24 h. Cells were treated with aspartame (100 μM, 1 mM, 10 mM & 20 mM) for 24 and 48 h. | |
Effect of aspartame on ROS level
Intracellular ROS levels were determined using the fluorescent probe DCFH-DA following exposure to aspartame at 24 and 48 h. Green fluorescence intensity of DCF gradually increased in the aspartame-treated cells compared with the control cells. A clear dose- and time-dependent increase of ROS level was observed in the aspartame-treated cells (Fig. 5 and 6).
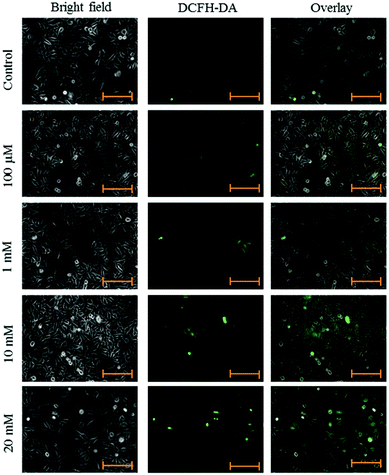 |
| Fig. 5 Effect of aspartame on ROS production. HeLa cells were grown in 6-well plates and allowed to adhere for 24 h. Cells were treated with aspartame (100 μM, 1 mM, 10 mM & 20 mM) for 24 h. Scale bar is 200 μm. Representative images are from three independent experiments (20×). | |
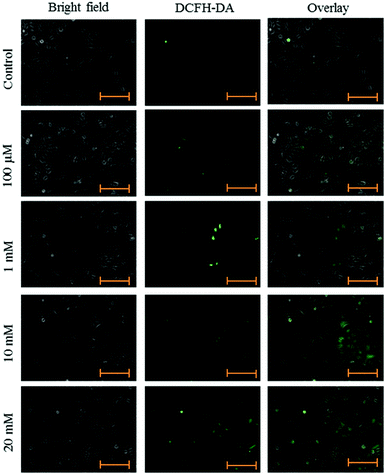 |
| Fig. 6 Effect of aspartame on ROS production. HeLa cells were grown in 6-well plates and allowed to adhere for 24 h. Cells were treated with aspartame (100 μM, 1 mM, 10 mM & 20 mM) for 48 h. Scale bar is 200 μm. Representative images are from three independent experiments (20×). | |
Effect of aspartame on apoptosis
Fluorescence microscopy was carried out to determine whether the cytotoxic effect of apoptosis was related to the induction of apoptosis and morphological features of cell death. This method combines the dual uptake of fluorescent DNA binding dyes AO and EB. The chromatin condenses in the stained nucleus, allowing one to distinguish viable, apoptotic, and necrotic cells. Control cells have normal morphology. Viable cells possess a uniform bright green nucleus. Early apoptotic cells show bright green areas of fragmented chromatin in the nucleus, and necrotic cells show a uniform bright orange nucleus. However, HeLa cells exposed to aspartame for 24 and 48 h exhibited fragmented and condensed chromatin, fragmented nuclei and the appearance of apoptotic bodies (Fig. 7 and 8).
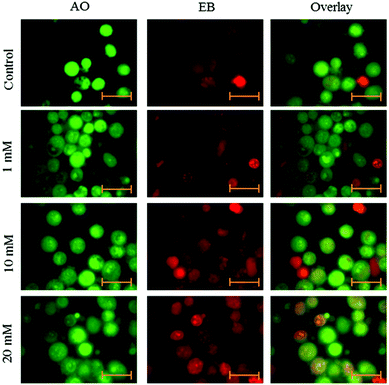 |
| Fig. 7 Morphological observation with AO/EB double staining by fluorescence microscope (100×). HeLa cells were seeded in 6-well plates and left for 24h for adherence; cells were treated with aspartame (1 mM, 10 mM & 20 mM) for 24 h. Scale bar is 20 μm. Representative images are from three independent experiments. | |
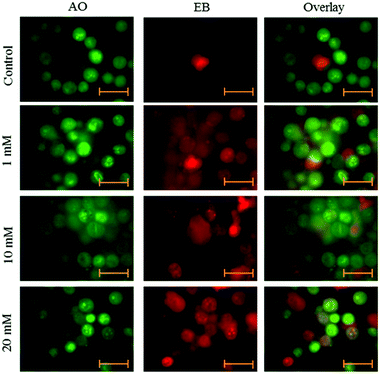 |
| Fig. 8 Morphological observation with AO/EB double staining by fluorescence microscope (100×). HeLa cells were seeded in 6-well plates and left for 24h for adherence; cells were treated with aspartame (1 mM, 10 mM & 20 mM) for 48 h. Scale bar is 20 μm. Representative images are from three independent experiments. | |
Discussion
The association of aspartame usage and increased risk of developing cancer is still highly controversial. Recent studies have reported that there is an association between the usage of aspartame and an increased danger of developing tumors, especially when used at higher consumption levels for extended periods of time. Morphological alterations were observed in cervical carcinoma cells exposed to high concentrations of aspartame (10 and 20 mM). The cells were observed to be flattened, granular and enlarged with multiple nuclei as compared to untreated controls. The Food and Drug Administration (FDA) approved aspartame use in food.21,22 Visual impairments, high aspartate transaminase levels, ear buzzing, loss of equilibrium, muscle aches, pancreatitis, depression and hypertension have been associated with intake of aspartame.4
Aspartic acid, phenylalanine, and methanol are produced as metabolites of aspartame.23 Aspartic acid is usually removed in the form of CO2 through the lungs. Stegink24 has reported that the plasma level of aspartic acid did not increase significantly following the oral administration of aspartame at a dose of 34 mg kg−1 in humans. Most of the phenylalanine was transported into the amino acid pool for protein synthesis, whereas a small amount of phenylalanine was removed in the form of CO2 through the lungs.25 Parthasarathy et al.,9 have reported that the methanol was primarily metabolized into formaldehyde and formate by oxidation. Hydrogen peroxide (H2O2) and superoxide anions were produced during these oxidation processes.
Flow cytometry provides a highly sensitive and accurate means of quantifying viable/nonviable MDCK cells following exposure to aspartame. Results obtained with the flow cytometry assay at 24 and 48 h after aspartame exposure approximated those obtained from the SRB assay. Moreover, we found a significant increase of nonviable (PI) estimates with the FDA/PI method following exposure to aspartame. Aspartame caused minor changes in the cell morphology in a dose- and time-dependent manner. Rounding, loss of adherence and sporadic distribution of cells were observed at higher concentrations of aspartame exposure. Changes in cell morphology are commonly associated with general cellular functions including locomotion, mitosis, cytokinesis, phagocytosis and secretion of macromolecules.26 Cell-to-cell and cell-to-substrate attachments are mediated via actin filaments.27 Aspartame may loosen this attachment as the actin filaments move for cell contraction. Aspartame may have a greater effect on the peripheral region of the culture compared to the confluent region due to cell crowding and adhesion of the cells to the substrate. The peripheral cells are younger and adhere more loosely than confluent cells, which have greater adhesion to the substrate and cell–cell.
Oxidative stress is an imbalance between the elevated level of ROS and impaired function of the antioxidant. Overproduction of ROS can induce death of immature cultured cortical neurons28 and DNA damage.29 Chandra et al.,30 have reported that increased ROS can trigger cell damage. The increase in ROS level may be due to methanol that is released during aspartame metabolism in the GI tract, as the pathway leads to formaldehyde and formate production by the catalase enzyme. Cell death could occur in response to high oxidative stress.31 In agreement with this, ROS level was increased in our study due to oxidative stress in a time- and dose-dependent manner.
There is a possibility of the production of larger and multinuclear cells during aspartame treatment, and therefore DNA content may increase in these cells. Therefore, the comet assay was carried out to understand the effect of various concentrations of aspartame on the DNA content of cells at 24 and 48 h. The results of this study revealed that aspartame induced DNA strand breaks observed as increased comet tails. The results indicated a time- and concentration-dependent effect on DNA within cells after exposure to aspartame. The effect of incubation time was, however, found to be not statistically significant, while the effects of higher concentrations of aspartame were highly significant. The increased DNA fragmentation observed at concentrations in excess of 1 mM of aspartame could be attributed to osmolarity as well as high concentration-induced apoptosis. Bandyopadhyay et al.32 found that aspartame had the potential to induce DNA damage in mouse bone marrow cells. Aspartame did not have a time- or concentration-dependent effect on the DNA content. The results found in this study are in agreement with results obtained by Sasaki et al.33 The metabolites of aspartame might have the potential to cause severe deleterious effects within cells at high concentrations.2
AO/EB double staining and fluorescence microscopy study showed distinct apoptotic morphological changes including cell shrinkage, chromatin condensation and formation of apoptotic bodies following 24 and 48 h of aspartame treatment. Our results cannot be directly extrapolated to in vivo situation since damage could observed at concentration levels in excess of 1 mM of aspartame that are possible in vivo after ingesting orally. The present study serves to provide further information on the potential effects of aspartame on cervical carcinoma cells.
Conflict of interest
The authors declare no conflict of interest.
Acknowledgements
This work was supported by the KU Research Professor Program of Konkuk University, Seoul, South Korea.
References
- P. Muthuraman, P. Jeongeun and K. Eunjung, In Vitro Cell. Dev. Biol.: Anim., 2014, 50, 851–857 CrossRef PubMed.
- Y. Oyama, H. Sakai, T. Arata, Y. Okano, N. Akaike, K. Sakai and K. Noda, Cell Biol. Toxicol., 2002, 18, 43–50 CrossRef CAS PubMed.
- W. E. Lipton, Y. N. Li, M. K. Younoszai and L. D. Stegink, Metab., Clin. Exp., 1991, 40, 1337–1345 CrossRef CAS PubMed.
- C. Woodrow, J. Appl. Nutr., 1984, 36, 1–15 Search PubMed.
- P. Humphries, E. Pretorius and H. Naude, Eur. J. Clin. Nutr., 2008, 62, 451–462 CrossRef CAS PubMed.
- E. Davoli, Food Chem. Toxicol., 1986, 24, 187–189 CrossRef CAS PubMed.
- J. J. Liu, M. R. Daya, O. Carrasquillo and N. S. Kales, Journal of Toxicology: Clinical Toxicology, 1998, 36, 175–181 CrossRef CAS PubMed.
- C. Trocho, R. Pardo, I. Rafecas, J. Virgili, X. Remesar, J. A. Fernandez-Lopez and M. Alemany, Life Sci., 1998, 63, 337–349 CrossRef CAS PubMed.
- J. N. Parthasarathy, S. K. Ramasundaram, M. Sundaramahalingam and S. D. Pathinasamy, J. Occup. Health, 2006, 48, 20–27 CrossRef PubMed.
- G. D. Castro, M. H. Costantini, A. M. Delgado de layno and A. Castro, Toxicol. Lett., 2002, 129, 227–236 CrossRef CAS PubMed.
- Y. Naito and T. Yoshikawa, JMAJ, 2002, 45, 271–276 Search PubMed.
- S. Fukushima, M. Arai, J. Nakanowatari, T. Hibino, M. Okuda and N. Ito, Gann: Jpn. J. Cancer Res., 1983, 74, 8–20 CAS.
- S. Gallus, L. Scotti, E. Negri, R. Talamini, S. Franceschi, M. Montella, A. Giacosa, L. Dal Maso and C. La Vecchia, Ann. Oncol., 2006, 18, 40–44 CrossRef PubMed.
- C. R. Whitehouse, J. Boullata and L. A. McCauley, AAOHN Journal, 2008, 56, 251–259 CrossRef PubMed.
- M. M. Andreatta, S. E. Munoz, M. J. Lantieri, A. R. Eynard and A. Navarro, Prev. Med., 2008, 47, 136–139 CrossRef CAS PubMed.
- S. R. Sturgeon, P. Hartge, D. T. Silverman, A. F. Kantor, W. M. Linehan, C. Lynch and R. N. Hoover, Epidemiology, 1994, 5, 218–225 CrossRef CAS PubMed.
- P. Muthuraman, G. Enkhtaivan, M. Bhupendra, M. Chandrasekaran, N. Rafi and D. H. Kim, Investigation of the role of aspartame on apoptosis process in HeLa cells, Saudi J. Biol. Sci., 2015 DOI:10.1016/j.sjbs.2015.06.01.
- D. D. Ross, C. C. Joneckis, J. V. Ordóñez, A. M. Sisk, R. K. Wu, A. W. Hamburger, R. E. Nora and R. E. Nora, Cancer Res., 1989, 49, 3776–3782 CAS.
- A. D. Van Eyk, Drug Chem. Toxicol., 2014, 15, 1–10 Search PubMed.
- P. Muthuraman, G. Enkhtaivan, V. Baskar, M. Bhupendra, N. Rafi, J. Bong Yeon and D. H. Kim, Biol. Trace Elem. Res., 2015 DOI:10.1007/s12011-015-0467-4.
- FDA, Fed. Regist., 1981, 46, 38285–38308 Search PubMed.
- FDA, Fed. Regist., 1983, 48, 31376–31382 Search PubMed.
-
A. Karim and T. Burns, Assessment of aspartame, CRC, Boca Raton, New York, London, Tokyo, 1996, pp. 57–66 Search PubMed.
-
L. D. Stegink, Aspartame: Physiology and Biochemistry, Marcel Dekker, New-York, 1984, pp. 509–553 Search PubMed.
-
F. K. Trefz and H. Bickel, The clinical evaluation of a food additive. Assessment of aspartame, 1996, pp. 149–160 Search PubMed.
- R. S. Adelstein, S. P. Scordilis and J. A. Trotter, Methods Achiev. Exp. Pathol., 1979, 8, 1–41 CAS.
- R. R. Weihing, Methods Achiev. Exp. Pathol., 1979, 8, 42–109 CAS.
- R. R. Ratan, T. H. Murphy and J. M. Baraban, J. Neurochem., 1994, 62, 376–379 CrossRef CAS PubMed.
- J. P. Spencer, A. Jenner, O. I. Aruoma, P. J. Evans, H. Kaur, D. T. Dexter, A. J. Lees, D. C. Maraden and B. Halliwell, FEBS Lett., 1994, 352, 246–250 CrossRef.
- J. Chandra, A. Samali and S. Orrenius, Free Radical Biol. Med., 2000, 29, 323–333 CrossRef CAS PubMed.
- S. V. Rana, J. Trace Elem. Med. Biol., 2008, 22, 262–284 CAS.
- A. Bandyopadhyay, S. Ghoshal and A. Mukherjee, Drug Chem. Toxicol., 2008, 31, 447–457 CrossRef CAS PubMed.
- Y. F. Sasaki, S. Kawaguchi, A. Kamaya, M. Ohshita, K. Kabasawa, K. Iwama, K. Taniguchi and S. Tsuda, Mutat. Res., Genet. Toxicol. Environ. Mutagen., 2002, 519, 103–119 CrossRef CAS.
|
This journal is © The Royal Society of Chemistry 2016 |
Click here to see how this site uses Cookies. View our privacy policy here.