DOI:
10.1039/C7RA00596B
(Paper)
RSC Adv., 2017,
7, 14015-14020
1,3-Bis(cyanomethoxy)calix[4]arene capped CdSe quantum dots for the fluorogenic sensing of fluorene†
Received
14th January 2017
, Accepted 22nd February 2017
First published on 2nd March 2017
Abstract
Capping of 1,3-bis(cyanomethoxy)-tert-butylcalix[4]arene (CAD) onto CdSe quantum dots (QDs) was characterized by a fluorescence enhancement of the QDs (λem = 580 nm) upon surface interaction with the phenolic moiety of CAD. CAD@QD showed selective and sensitive 1.67 fold fluorescence enhancement in the presence of fluorene among fifteen PAHs. The fluorescence enhancement was characterized by monolayer adsorption of fluorene on to the surface of CAD@QD. The limit of detection for fluorene was observed to be 0.8 nM. This method was used and compared with detection of fluorene in spiked respirable dust (PM10) samples collected during an open biomass (stubble) burning event.
Introduction
Polynuclear aromatic hydrocarbons (PAHs) are a family of pollutants emitted involuntarily during the combustion processes in our daily lives including cooking, domestic heating, smoking, charbroiling meat, and open fires (including forest fires & open biomass burning, incense and candle emissions).1 PAHs are used to make dyes, plastics, and pesticides and some are even used in medicines2 and they find their way into the human body commonly through air, soil, water and food.3 PAHs have been reported to be potential carcinogens and mutagens.4 Thus, the United States Environmental Protection Agency (USEPA) has regulated this class of chemicals in ambient air, water and soil to a trace level.5 The analysis of PAHs in environmental samples are presently being carried out using extraction followed by high performance liquid chromatography (HPLC) with a UV-vis/fluorescence detector6 and Gas Chromatography-Mass Spectrometry/Flame Ionization Detector (GC-MS/FID).2,7 However, these methods vary from matrix to matrix, are costly, time consuming and require skilled manpower. Thus, there is a dire need for simple, cost-effective, real-time field analysis of PAHs with low detection limits.
Fluorogenic sensing is a key approach, for selective and sensitive detection of analyte. Fluorogenic sensing of metal and anion have been widely studied, but few reports exists in the sensing of organic analytes.8 Sensing of aromatic analytes is based on the phenomenon of inclusion, whereby the chemosensor has a receptor moiety comprising of a cavity having potential for formation of inclusion complex. These inclusion based chemosensor receptor moieties exploit weak intermolecular bonding with the analyte of interest. Container molecules which form inclusion complex with small organic molecules include, cyclodextrins,9 calixarenes,10 cucurbiturils and pillararenes.11 Of these, calixarenes are a versatile class of compounds which have significantly been studied for inclusion behaviour.8 Calix[4]arenes have the advantage of easy scalable synthesis, easy derivatization on lower rim, upper rim and bridging methylene moieties, with significant conformational control during derivatization.12 Optical sensors based on functionalized calix[4]arene have widely been studied for the recognition of cations,13 anions14 and various neutral analytes.14 However, there have been limited studies on the fluorogenic recognition behavior of calix[4]arene based receptors for small organic molecules of environmental interest. The limitation is partly accounted to the challenges in designing of suitable fluorogenic derivatives of calix[4]arene for the relay of inclusion complexation.
Recently with the understanding of the optical properties of quantum dots (QDs), there have been surge in the fluorescent sensors based on QDs.8,15 Quantum dots have various advantages over organic fluorogenic moieties like broad excitation, narrow and size tuneable emission spectra and high quantum yield with reduced susceptibility to photobleaching and high photochemical stability.16 Apart from the optical features, QDs provide the flexibility in designing of the receptor QD association, whereby the receptor can be held on the surface of QD by covalent or non-covalent interaction. Lower rim carboxylic acid substituted and upper rim sulphonate functionalized calix[n]arene (n = 4, 6, 8)17 have extensively been studied for capping of CdSe QDs. Few quantum dot capped calixarene derivatives have been studied for inclusion complex based fluorogenic relay of organic analytes.8,11 Li et al. reported a similar sol–gel derived silica nanospheres coated with calix[4]arene and calix[7]arene CdTe QDs as luminescent probe for methomyl.18 Recently Carrillo-Carrión et al. (2011) studied CdSe/ZnS non-covalently coated with calix[8]arene as a fullerene (C60) nanosensor and demonstrated its use in the liquid–liquid extraction based analysis of the analyte in river water sample.19 These studies suggest use of calixarene, its lower rim carboxylate or upper rim sulphonate functionalized derivatives as suitable substrates for sensing of aromatic analytes based on inclusion of analyte in the π-basic cavity of the calixarene. However, with the recent advancement in the influence of the photophysical behaviour of quantum dot by various organic functional moieties20 like thioether,21 carboxylates,22 sulphonates,23 amine,24 pyridine,25 phenols26 and phosphine oxides,27 and the unfavourable electrostatic interactions in sulphonated/carboxylated calix[4]arene for inclusion of neutral molecules, the non-polar calix[4]arene derivatives are suitable receptors for inclusion based sensing. Thus, the present work investigates the CdSe QD surface interaction with lower rim functionalized 1,3-bis(cyanomethoxy)-tert-butylcalix[4]arene (CAD) and its PAH sensing behavior. The sensing behavior of the CAD@QD were further evaluated for PAH sensing in ambient air PM10 samples.
Materials and methods
Details provided in ESI.†
Synthesis of CdSe QDs
CdSe QDs were synthesized by the method described by Peng and coworkers using CdO as precursor28 (details provided in ESI†).
Synthesis of CAD@CdSe QDs
The CAD capped QD was prepared by addition of CAD solution to a solution of CdSe QDs in CHCl3. To a 1 mL solution of TOPO-capped QD (120 nM), was added 1–20 μL of CAD solution (1 mM) in CHCl3. The mixture was placed for vortex mixing at room temperature for about 30 min. Upon dispersion, the mixture was sonicated for 30 min using an ultrasonic bath at room temperature. The resulting mixture (CAD@QD) were treated with concentration of 20 μM CAD and 120 nM w.r.t. CdSe QDs and used for further spectroscopic studies and characterization.
Fluorescence titration
The fluorescence titration of CAD and PAH with QD and CAD@QD, respectively were carried out. To a 1 mL solution of 120 nM solution of CdSe QD in CHCl3, was added 1–20 μL of 1 mM CAD solution in CHCl3.
The CAD@QD stock solution was prepared by addition of 20 μL of CAD to 120 nM QD solution in CHCl3. The solution w.r.t. CAD is 10 μM against the QD concentration of 120 nM. The stock solution was diluted to 10 nM w.r.t. CdSe QD and used for fluorescence titration. To a 10 nM solution of CAD@QD w.r.t. the QD, was added 1–10 μL of 1 μM PAH solution.
Sampling and monitoring of ambient air
Sampling was performed during the October 2015 at Naruwana, Bathinda, Punjab, India (latitude 30.163806° and longitude 74.896031°) as shown in ESI Fig. S1.† The respirable fraction of ambient air particulate matter (PM10) was collected on USEPA glass fibre (GFA) filter paper of dimension 20.3 × 25.4 cm2. The samples were collected during biomass burning at a flow rate >1.0 m3 min−1 for a continuous period of 8 h using respirable dust sampler APM 460BL, Envirotech India Pvt Ltd. The particles were collected with glass fibre filters, desiccated and weighed till constant weight, before and after sample collection. Analysis of the filter paper containing PM10 was performed according to USEPA TO 13A norm.7
Extraction & cleanup and GC-MS analysis of PAHs
Extraction & cleanup and GC-MS analysis of the PAHs extract was carried out as provided in ESI.†
Fluorescence study of PM10 extract and spiked extract
The PM10 extract was diluted in CHCl3 to 10, 100 and 1000 times dilutions. The solution thus prepared were spiked with fluorene upto 20 nM and were studied for fluorescence sensing of fluorene. The samples were then subjected to fluorescence analysis at an excitation wavelength of 350 nm and the fluorescence emission were recorded with wavelength range between 400–700 nm.
Results and discussion
Characterization of QD and CAD@QD
Distally substituted 1,3-bis(cyanomethoxy)-tert-butylcalix[4]arene (CAD)29 and CdSe QD28 were synthesized and characterized using the method reported in literature. The UV-vis absorption spectrum of the QD in the dispersed CHCl3 media was characterized by an absorption peak at 580 nm (ESI Fig. S2†). Based on the UV-vis characteristic and using plasmonic equation,30 the size of the quantum dot was calculated to be 3.8 nm and extinction co-efficient 2.04 × 105 per mol. Based on the extinction co-efficient per mol QD, the observed concentration of the solution was 120 nM. The capping of CAD derivative on CdSe QD was carried out in CHCl3 as dispersion medium with stirring followed by sonication.
Fig. 1(A and B) shows the Transmission Electron Microscope (TEM) images and histograms of QD and CAD@QD. All diameters are a measure of the major axis. A histogram showing the results of 100 individual measurements of the diameters of QDs from the TEM micrograph is depicted in Fig. 1(C and D). The measured mean diameter of QD and CAD@QD obtained with an average of 2.5 ± 0.5 and 2.8 ± 0.5 nm, respectively. From the TEM images it is quite evident that the QD and CAD@QD are both well dispersed and there is a coating of CAD on the surface of QD.
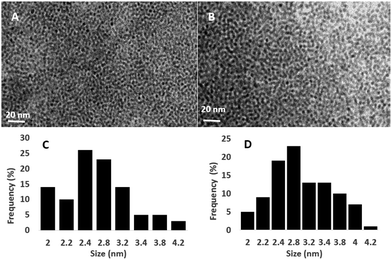 |
| Fig. 1 TEM images of (A) QD and (B) CAD@QD at 20 nm scale. Particle size histograms of (C) QD and (D) CAD@QD. | |
The binding behaviour of the CAD on the surface of QD were also studied using fluorescence spectroscopy. The QD (120 nM) was characterized by a fluorescence emission at λem = 580 nm when excited at 350 nm in CHCl3 as given in Fig. 2. The full-width-at-half-maximum (FWHM) of 25 nm was observed for QD, is below 30 nm, which indicates narrow size range distribution of these QDs.30 The change in the fluorescence spectra of QD upon addition of increasing aliquot of CAD in CHCl3 is depicted in Fig. 2. Upon addition of CAD (1–20 μM) fluorescence enhancement was observed in the emission due to QD at λmax = 580 nm. This behaviour could be accounted to the interaction of CAD with the surface of the nanocolloid QD upon adsorption of CAD.31 Similar fluorescence enhancement have been observed in case of pyridine and amine nitrogen upon interaction with QD surface.32 To investigate the binding of CAD to the surface of QD FTIR spectroscopy and 1H NMR of CAD and CAD@QD were investigated as given in ESI Fig. S3 and S4.† However, no significant changes were observed in both the studies, except for the presence of an interaction of CAD phenolic hydroxyl group with the QD surface leading to broadening of the phenolic proton singlet in 1H NMR. Further, the di-propoxy derivative of CAD (1–20 μM) (PCAD)14b was used for fluorescence titration with QD (ESI Fig. S5†). An insignificant fluorescence quenching of the QD was observed upon addition of PCAD (1–10 nM) to QD. This suggests that interaction between the CAD and the QD surface occurs through proton exchange.33
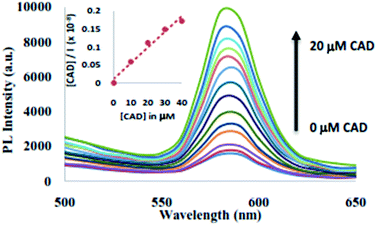 |
| Fig. 2 Fluorescence emission spectra of QD (10 nM) upon addition of CAD (0–20 μM). Inset: linear fitting for Langmuir monolayer adsorption of CAD on to the surface of QD. | |
The fluorescence enhancement of QD upon addition of CAD in CHCl3 solution was studied for the Langmuir monolayer adsorption isotherm.34 The linear expression for Langmuir monolayer adsorption was studied using eqn (7) (ESI†). The plot of the Langmuir isotherm is shown in inset of Fig. 2 was obtained using [CAD]/I vs. [CAD]. The equilibrium binding constant, B was found to be 3.77 × 106 M−1. The calculated equilibrium binding constant is much greater than those reported for TOPO binding on CdSe quantum dot,35 thus it seems that CAD displaces the TOPO competetively from the QD surface.
PAH sensing behavior of CAD@QD
Fluorescence is a sensitive technique for detection of analyte, as it is influenced by the immediate environment of the fluorophore. The fluorescence sensing behaviour of CAD@QD (10 nM w.r.t. QD) was carried out in CHCl3 as solvent with various PAH namely, fluorene (FLU), napthalene (NAP), phenanthrene (PHE), pyrene (PYN), fluoranthene (FLA), anthracene (ANT), benzo[a]anthracene (BAN), benzo[b]fluoranthene (BFL), benzo[a]pyrene (BAL), benzo[ghi]perylene (BGP), benzo[k]fluoranthene (BKF), chrysene (CHR), dibenzo[a,h]anthracene (DAN), indenol[1,2,3-cd]pyrene (ICP) and acenaphthene (ACT). Influence of background fluorescence of PAHs on the fluorescence spectra of QD and CAD@QD, was ascertained by performing fluorescence titration of all the PAHs to either solvent or CdSe QD as given in ESI Fig. S6 and S7.† Studies indicate no significant emission of all the 15 PAHs was observed in the emission range of the CdSe QD. Further, upon addition of 10 nM of each PAH to CdSe QD, no change in the fluorescence of the quantum dot was observed. Similarly, fluorescence titration of TOPO capped QD (10 nM) were also carried out with various PAHs. The results of these study indicate no significant change in the fluorescence behaviour of the TOPO capped QD (10 nM) upon addition of various PAH (10 nM) as analytes in CHCl3. Further, the fluorescence emission of CAD@QD (10 nM) in presence of 10 nM PAH solution are as given in Fig. 3 (ESI Fig. S8†). A significant fluorescence enhancement was observed upon addition of 10 nM FLU to CAD@QD in CHCl3. However in presence of other PAHs, CAD@QD did not show any significant change in fluorescence emission (ESI Fig. S9†). Thus confirming the selective sensing behaviour of CAD@QD for fluorene to be characteristic of the calix[4]arene on the QD surface. The CAD@QD showed varied response to the PAH sensing, which could be due to the improper orientation of calix[4]arene inclusion cavity of CAD upon surface binding on to QD.
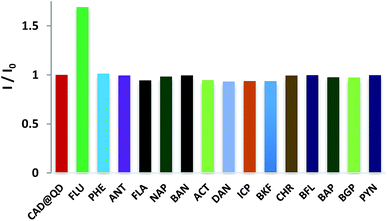 |
| Fig. 3 Fluorescence intensity change (I/I0) of CAD@QD (10 nM) upon addition of 10 nM of different PAHs (FLU, PHE, ANT, FLA, NAP, BAN, ACT, DAN, ICP, BKF, CHR, BFL, BAP, BGP, PYN) in CHCl3. | |
Further, fluorescence titrations of CAD@QD was carried out with fluorene (10 nM). Upon addition of increasing amount of fluorene to the CAD@QD (10 nM), a fluorescence enhancement was observed with the emission band centred at λem = 580 nm. The fluorescence intensity of CAD@QD increased linearly with increase in concentration of fluorene and maximum enhancement was observed at 10 nM fluorene concentration (Fig. 4). The significant fluorescence enhancement upto 1.67 folds was observed upon binding of fluorene (10 nM) to CAD@QD (10 nM). Thus, from these results, it is envisaged that the resulting fluorescence enhancement of CAD@QD in the presence of fluorene, could be accounted to the interaction of fluorene with CAD in CHCl3 solution.
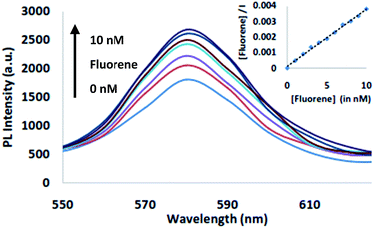 |
| Fig. 4 Change in fluorescence of CAD@QD upon addition of 10 nM of fluorene in CHCl3 (λex = 350 nm). Inset: linear fitting for Langmuir monolayer adsorption of fluorene on to the surface of CAD@QD. | |
To establish the mode of binding between the CAD@QD and fluorene, 1H NMR titration were carried out in CDCl3, which indicated no shift in the fluorene or the CAD protons. Further, fluorescence titration of CAD (1 μM) upon addition of fluorene (1–10 μM) and fluorene (1 μM) upon addition of CAD (1–10 μM) was carried out (ESI Fig. S10 and S11†). The later addition showed a significant change in the fluorescence spectra of fluorene. A significant fluorescence quenching of the fluorene fluorescence at λem = 420 nm was observed upon excitation at 350 nm with the addition of CAD solution (ESI Fig. S11†). Thus, CAD interacts with the fluorene in CHCl3 by weak supramolecular interactions which results in the sensing of fluorene by CAD.
The fluorescence enhancement of the CAD@QD in presence of fluorene was studied by Langmuir isotherm for monolayer adsorption of fluorene in the inclusion cavity of CAD@QD in dispersed phase in CHCl3.34 The plot of concentration of fluorene ([fluorene]) versus [fluorene]/I was found to be a linear as shown in inset Fig. 4. The coefficient of the linear fit is 0.996, which indicates a monolayer adsorption of fluorene on CAD@QD. The plot of the Langmuir isotherm is shown in inset of Fig. 4 was obtained using [fluorene]/I vs. [fluorene]. The equilibrium binding constant, B was found to be 4 × 109 M−1. The limit of detection of PAH fluorene using CAD@QD was found to be 0.8 nM. Earlier Sehatnia et al. studied PAH sensing behavior of calix[4]arene moieties functionalized on upper rim, which showed a detection limit of upto 25 μM with a binding based fluorescence quenching as the sensor relay phenomenon.36 Thus, the present method has a good sensitivity for detection of fluorene using CAD@QD, which would be useful in developing a quantitative methods for environmental and food sample analysis.
Ambient air PM10 PAH analysis
In order to check the feasibility of the CAD@QD for fluorene analysis and monitoring, ambient air samples of respirable suspended particulate matter (PM10) were collected during the open biomass burning in the agricultural fields near Bathinda city. PM10 were collected using a respirable dust sampler on a glass fibre filter. The filter was then Soxhlet extracted and precleaned for PAH.37 The GC-MS analysis of the extracted samples showed the presence of various trace residues of organics during the open biomass burning process (ESI Fig. S12†). The GC-MS spectrograph of the PM10 extracted samples showed the presence of trace residues namely isopropyl-5-methylphenol, 1H-isoindole-1,3(2H)-dione, 2,4-di-tert-butylphenol, 5-phenylpentanoic acid, 1-nitro-β-D-arabinofuranose tetraacetate, 3-[2-tetrahydropyranyloxy]-1-butyne, acetosyrignone, 1-amino-2-hydroxy-3,5-nitrobenzene, 5,6-dihydro-2-methoxy-2H-pyran, 5-butyldihydro-4-methylfuranone, 2-methoxy-1,3,4-trimethyl-benzene and ergost-3-en-3-ol. These are characteristic fragmented oxidized products of the lignocellulosic material of biomass during the open burning process.38 The GC-MS analysis showed no traces of any PAHs. The matrix solution was diluted 10, 100 and 1000 times. This organic loaded matrix with various dilutions were spiked with fluorene upto a concentration of 20 nM and subjected to the analysis of fluorene upon 10 times dilution with 10 nM CAD@QD in CHCl3. The concentration of fluorene as determined in the triplicate analysis of the sample and were found to be 0.56 (±0.21), 1.21 (±0.30), 1.68 (±0.22) and 1.78 (±0.25) nM with the extract 10, 100 and 1000 times diluted extract, respectively. A significantly high organic loading of the sample showed a fluorescence quenching indicating reduced/competitive binding of fluorene under a higher organic loaded ambient air particulate matter matrix.
Conclusions
1,3-Bis(cyanomethoxy)-tert-butylcalix[4]arene (CAD) capped on CdSe QD shows selective fluorogenic response for the PAH fluorene with a detection limit well below the environmental permissible limits. The PAH sensing was selectively observed in CAD@QD (λem = 580 nm) which is accounted to the orientation of calix[4]arene on the quantum dot surface. Further this sensor was subjected to fluorogenic sensing of fluorene in ambient air monitoring in the sensing event, however upon dilution upto 100 and 1000 times there is significant lowering of the interference and actual determined fluorene is close to the expected value. Thus, a reliable analysis of fluorene could be carried out.
Acknowledgements
This work was supported in terms of funds, infrastructure, fellowship and Central Instrumentation Facility by Central University of Punjab, Bathinda, India for M. Phil-Ph. D work of Rabindra Kumar. J. N. Babu is thankful to DST, New Delhi, India for providing funds through DST Fast Track Young Scientist as Project Ref. No. 240/2010 for research support. The authors' acknowledge Electron Microscope Division, AIIMS, New Delhi for TEM facility and Rajinder Singh for ambient air sampling in his farm.
Notes and references
-
(a) S. Baek, R. Field, M. Goldstone, P. Kirk, J. Lester and R. Perry, Water, Air, Soil Pollut., 1991, 60, 279–300 CrossRef CAS;
(b) L. Zhu, Y. Takahashi, T. Amagai and H. Matsushita, Talanta, 1997, 45, 113–118 CrossRef CAS PubMed;
(c) H. Fromme, A. Oddoy, M. Piloty, M. Krause and T. Lahrz, Sci. Total Environ., 1998, 217, 165–173 CrossRef CAS PubMed;
(d) C.-S. Li and Y.-S. Ro, Atmos. Environ., 2000, 34, 611–620 CrossRef CAS;
(e) S. C. Lung, M. C. Kao and S. C. Hu, Indoor Air, 2003, 13, 194–199 CrossRef CAS PubMed.
- Y. Liu, M. L. Lee, K. J. Hageman, Y. Yang and S. B. Hawthorne, Anal. Chem., 1997, 69, 5001–5005 CrossRef CAS.
- S. K. Samanta, O. V. Singh and R. K. Jain, Trends Biotechnol., 2002, 20, 243–248 CrossRef CAS PubMed.
-
(a) M. Müller, C. Kübel and K. Müllen, Chem.–Eur. J., 1998, 4, 2099–2109 CrossRef;
(b) H. E. van Gijssel, L. J. Schild, D. L. Watt, M. J. Roth, G.-Q. Wang, S. M. Dawsey, P. S. Albert, Y.-L. Qiao, P. R. Taylor and Z.-W. Dong, Mutat. Res. Fund. Mol. Mech. Mutagen., 2004, 547, 55–62 CrossRef CAS PubMed;
(c) G. Falcó, J. L. Domingo, J. M. Llobet, A. Teixidó, C. Casas and L. Müller, J. Food Prot., 2003, 66, 2325–2331 CrossRef;
(d) H. Guo, S. Lee, L. Chan and W. Li, Environ. Res., 2004, 94, 57–66 CrossRef CAS PubMed.
- K. C. Jones and P. De Voogt, Environ. Pollut., 1999, 100, 209–221 CrossRef CAS PubMed.
- T. Cserháti and E. Forgács, Chromatography in Environmental Protection, CRC Press, 2000 Search PubMed.
-
(a) A. V. Herrera-Herrera, M. Asensio-Ramos, J. Hernández-Borges and M. Á. Rodríguez-Delgado, Trends Anal. Chem., 2010, 29, 728–751 CrossRef CAS;
(b) C. L. Arthur, K. Pratt, S. Motlagh, J. Pawliszyn and R. P. Belardi, HRC, J. High Resolut. Chromatogr., 1992, 15, 741–744 CrossRef CAS;
(c) M. M. Schantz, J. J. Nichols and S. A. Wise, Anal. Chem., 1997, 69, 4210–4219 CrossRef CAS;
(d) D. L. Poster, M. M. Schantz, L. C. Sander and S. A. Wise, Anal. Bioanal. Chem., 2006, 386, 859–881 CrossRef CAS PubMed.
- H. J. Kim, M. H. Lee, L. Mutihac, J. Vicens and J. S. Kim, Chem. Soc. Rev., 2012, 41, 1173–1190 RSC.
- R. Villalonga, R. Cao and A. Fragoso, Chem. Rev., 2007, 107, 3088–3116 CrossRef CAS PubMed.
- C. D. Gutsche, in Calixarenes 2001, ed. M. Z. Asfari, V. Bohmer, J. Harrowfield and J. Vicens, Springer, 2001, pp. 1–25 Search PubMed.
- K. Kim, N. Selvapalam, Y. H. Ko, K. M. Park, D. Kim and J. Kim, Chem. Soc. Rev., 2007, 36, 267–279 RSC.
- C. D. Gutsche, in Calixarenes Revisited, ed. J. F. Stoddart, RSC, Cambridge, UK, 1998 Search PubMed.
-
(a) R. Joseph and C. P. Rao, Chem. Rev., 2011, 111, 4658–4702 CrossRef CAS PubMed;
(b) R. K. Pathak, K. Tabbasum, A. Rai, D. Panda and C. P. Rao, Anal. Chem., 2012, 84, 5117–5123 CrossRef CAS PubMed;
(c) M. Kumar, J. N. Babu, V. Bhalla and R. Kumar, Sens. Actuators, B, 2010, 144, 183–191 CrossRef CAS;
(d) M. Kumar, V. Bhalla, A. Dhir and J. N. Babu, Dalton Trans., 2010, 39, 10116–10121 RSC;
(e) M. Kumar, J. N. Babu and V. Bhalla, J. Inclusion Phenom. Macrocyclic Chem., 2010, 66, 139–145 CrossRef CAS.
-
(a) V. S. Mummidivarapu, V. K. Hinge and C. P. Rao, Dalton Trans., 2015, 44, 1130–1141 RSC;
(b) J. N. Babu, V. Bhalla, M. Kumar, R. K. Puri and R. K. Mahajan, New J. Chem., 2009, 33, 675–681 RSC;
(c) M. Kumar, J. N. Babu and V. Bhalla, Talanta, 2010, 81, 9–14 CrossRef CAS PubMed;
(d) J. N. Babu, V. Bhalla, M. Kumar and H. Singh, Lett. Org. Chem., 2006, 3, 787–793 CrossRef CAS;
(e) A. Dhir, V. Bhalla and M. Kumar, Org. Lett., 2008, 10, 4891–4894 CrossRef CAS PubMed.
-
(a) R. Freeman and I. Willner, Chem. Soc. Rev., 2012, 41, 4067–4085 RSC;
(b) J. Cheng, X. Zhou and H. Xiang, Analyst, 2015, 140, 7082–7115 RSC.
- U. Banin, M. Bruchez, A. Alivisatos, T. Ha, S. Weiss and D. Chemla, J. Chem. Phys., 1999, 110, 1195–1201 CrossRef CAS.
-
(a) T. Jin, F. Fujii, E. Yamada, Y. Nodasaka and M. Kinjo, J. Am. Chem. Soc., 2006, 128, 9288–9289 CrossRef CAS PubMed;
(b) T. Jin, F. Fujii, H. Sakata, M. Tamura and M. Kinjo, Chem. Commun., 2005, 4300–4302 RSC.
- T. Li, Y. Zhou, J. Sun, D. Tang, S. Guo and X. Ding, Microchim. Acta, 2011, 175, 113–119 CrossRef CAS.
- C. Carrillo-Carrión, B. Lendl, B. M. Simonet and M. Valcárcel, Anal. Chem., 2011, 83, 8093–8100 CrossRef PubMed.
-
(a) R. D. Harris, S. B. Homan, M. Kodaimati, C. He, A. B. Nepomnyashchii, N. K. Swenson, S. Lian, R. Calzada and E. A. Weiss, Chem. Rev., 2016, 116, 12865–12919 CrossRef CAS PubMed;
(b) A. J. Morris-Cohen, M. Malicki, M. D. Peterson, J. W. J. Slavin and E. A. Weiss, Chem. Mater., 2013, 25, 1155–1165 CrossRef CAS.
- D. Wan, Q. Fu and J. Huang, J. Appl. Polym. Sci., 2006, 102, 3679–3684 CrossRef CAS.
- K. S. Leschkies, R. Divakar, J. Basu, E. Enache-Pommer, J. E. Boercker, C. B. Carter, U. R. Kortshagen, D. J. Norris and E. S. Aydil, Nano Lett., 2007, 7, 1793–1798 CrossRef CAS PubMed.
- M. T. Fernández-Argüelles, W. J. Jin, J. M. Costa-Fernández, R. Pereiro and A. Sanz-Medel, Anal. Chim. Acta, 2005, 549, 20–25 CrossRef.
- S. T. Selvan, T. T. Tan and J. Y. Ying, Adv. Mater., 2005, 17, 1620–1625 CrossRef CAS.
- C. Zhang, S. O'Brien and L. Balogh, J. Phys. Chem. B, 2002, 106, 10316–10321 CrossRef CAS.
- H. Li and C. Han, Chem. Mater., 2008, 20, 6053–6059 CrossRef CAS.
- H. Skaff, M. F. Ilker, E. B. Coughlin and T. Emrick, J. Am. Chem. Soc., 2002, 124, 5729–5733 CrossRef CAS PubMed.
- W. W. Yu and X. Peng, Angew. Chem., Int. Ed., 2002, 41, 2368–2371 CrossRef CAS PubMed.
- E. M. Collins, M. A. McKervey, E. Madigan, M. B. Moran, M. Owens, G. Ferguson and S. J. Harris, J. Chem. Soc., Perkin Trans. 1, 1991, 3137–3142 RSC.
- W. W. Yu, L. Qu, W. Guo and X. Peng, Chem. Mater., 2003, 15, 2854–2860 CrossRef CAS.
- X. Ji, G. Palui, T. Avellini, H. B. Na, C. Yi, K. L. Knappenberger Jr and H. Mattoussi, J. Am. Chem. Soc., 2012, 134, 6006–6017 CrossRef CAS PubMed.
- V. I. Klimov, A. Mikhailovsky, D. McBranch, C. Leatherdale and M. Bawendi, Phys. Rev. B: Condens. Matter, 2000, 61, R13349 CrossRef CAS.
- R. D. Harris, S. Bettis Homan, M. Kodaimati, C. He, A. B. Nepomnyashchii, N. K. Swenson, S. Lian, R. Calzada and E. A. Weiss, Chem. Rev., 2016, 116, 12865–12919 CrossRef CAS PubMed.
- W. Brey, Physical Chemistry and its Biological Applications, Elsevier, 2012 Search PubMed.
- A. M. Munro, I. Jen-La Plante, M. S. Ng and D. S. Ginger, J. Phys. Chem. C, 2007, 111, 6220–6227 CAS.
- B. Sehatnia, R. E. Sabzi, F. Kheiri and A. Nikoo, J. Appl. Electrochem., 2014, 44, 727–733 CrossRef CAS.
- H. A. Bamford, D. Z. Bezabeh, M. M. Schantz, S. A. Wise and J. E. Baker, Chemosphere, 2003, 50, 575–587 CrossRef CAS PubMed.
- D. Özçimen and A. Ersoy-Meriçboyu, Renewable Energy, 2010, 35, 1319–1324 CrossRef.
Footnote |
† Electronic supplementary information (ESI) available. See DOI: 10.1039/c7ra00596b |
|
This journal is © The Royal Society of Chemistry 2017 |
Click here to see how this site uses Cookies. View our privacy policy here.