DOI:
10.1039/C7RA11305F
(Paper)
RSC Adv., 2017,
7, 51062-51068
A multicomponent reaction of 2-aminoimidazoles: microwave-assisted synthesis of novel 5-aza-7-deaza-adenines†‡
Received
13th October 2017
, Accepted 30th October 2017
First published on 2nd November 2017
Abstract
An efficient and highly selective multicomponent synthesis of 4-aminoimidazo[1,2-a][1,3,5]triazines, which are 5-aza-7-deaza-isosteres of adenine, was developed. The reaction of 2-aminoimidazoles, triethyl orthoformate and cyanamide under microwave irradiation proceeded regioselectively to provide a library of novel 5-aza-7-deaza-adenines in good yields and purity. The developed method was demonstrated to be scalable and highly reproducible in three different monomode microwave reactors. A rearrangement was proposed to explain the high selectivity of the reaction.
Introduction
Purine is a heterocyclic scaffold of primary importance in nature. Purine bases adenine and guanine encode half of the genetic information in DNA molecules.2 Being the most ubiquitous nitrogen-containing heterocycle in nature, purine serves as a scaffold for molecules involved in the functioning of thousands of proteins in the human body.3 Thus, purines have become a privileged scaffold in drug discovery and development, inspiring also research in the area of structurally related heterocyclic systems i.e. purine isosteres. Among 1,3,5-triazine based purine isosteres, 5-azapurines (1,2,4-triazolo[1,5-a][1,3,5]triazines)4 and 5-aza-9-deazapurines (pyrazolo[1,5-a][1,3,5]triazines)5 have been widely investigated and considerable success has been achieved in the development of new bioactive compounds based on these scaffolds.6 Due to limited methods for their preparation, biological activity of imidazo-fused 1,3,5-triazines has been less explored. However, the 5-aza-7-deaza-isostere of the purine system (imidazo[1,2-a][1,3,5]triazine) has been employed as a scaffold for the construction of inhibitors of therapeutically valuable enzymes: activated Cdc42-associated tyrosine kinase 1,7 focal adhesion kinase,8 and dipeptidyl peptidase IV.9 Some 5-aza-7-deazapurines were claimed to be agonists of opioid μ-receptors,10 ligands of adenosine receptors,11 and potential antiviral agents.12
The search for bioactive molecules among aza-/deaza-isosteres of adenine has been an efficient research strategy in medicinal chemistry. For example, the 8-aza-7-deaza-adenine analogue 1 (Chart 1) was developed as an isosteric modification of erythro-9-(2-hydroxy-3-nonyl)adenine (EHNA), the earliest selective inhibitor of phosphodiesterase 2 (PDE2).13 Compound 1 was found to be much more potent inhibitor of PDE2, inhibiting this enzyme with Ki value of 0.053 nM (compared with Ki = 7 nM for EHNA).14 Another 8-aza-7-deaza-adenine based drug ibrutinib (2) has been recently approved for the treatment of mantle cell lymphoma and chronic lymphocytic leukemia.15 7-Deaza-adenine derivative CGP62464 (3) was found to be a potent inhibitor of Src family kinases.16 An antiviral agent inhibiting S-adenosyl homocysteinase was developed on the 5-aza-9-deaza-adenine scaffold (compound 4).17
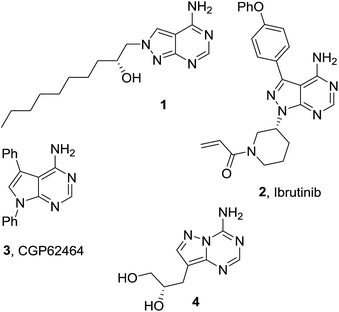 |
| Chart 1 Biologically active aza-/deaza-isosteres of adenine. | |
The discovery of bioactive aza/deaza isosteres of adenine has encouraged us to develop new and effective methods for the synthesis of compounds within this general framework. As part of our ongoing research program on practical approaches for preparation of 1,3,5-triazine based purine-like compounds, in this contribution we report a new method for the synthesis of 4-aminoimidazo[1,2-a][1,3,5]triazines (6), which are 5-aza-7-deaza-isosteres of adenine.
The annulation of imidazole onto the 1,3,5-triazine ring was the first and has been the most common approach reported to date for the synthesis of imidazo[1,2-a][1,3,5]triazines.18–20 We decided to investigate the less explored annulation of the 1,3,5-triazine ring onto substituted 2-aminoimidazoles. Our group has previously achieved successful annulation of 1,3,5-triazine ring onto aminoazoles viz. 5-aminopyrazoles and 5-aminotriazoles via their three-component, microwave-assisted reaction with orthoformates and cyanamide.21 Herein we extend this methodology to a similar heterocyclic system preparing hitherto unknown 5-aza-7-deaza-adenines via our multicomponent, microwave-assisted reaction using 2-aminoimidazoles. These substrates are different from those previously used by their significantly greater basicity. Moreover, regioselectivity of the ring closure could be a potential challenge for non-symmetrically substituted 2-aminoimidazoles due to the similarity of their reactive endocyclic nitrogen atoms.
Results and discussion
Synthesis
The optimisation of conditions for the multicomponent reaction was performed with 2-amino-4-phenylimidazole (5a) as a model substrate (1 mmol in 2 mL of solvent) using the Discover SP (CEM) microwave synthesizer (Table 1). Initially, we attempted a one-pot, three-component reaction of 5a with triethyl orthoformate and cyanamide in methanol under microwave irradiation at 150 °C for 25 min (Entry 1). To our satisfaction, the product 6a was obtained in high purity after simple filtration, but the yield was rather low (26%). The analysis of the filtrate revealed the presence of a substantial quantity of unreacted 5a together with the product. In the screening of solvents for this reaction, we found that the yield of isolated product could be improved to 61% when the reaction was carried out in ethyl acetate (Entry 6). Continuing optimisation of the reaction conditions by altering the time of reaction, we found that shortening the reaction time to 20 min gave further improvement in the yield (Entry 7). Varying reagent ratio revealed that 6a could be obtained in a better yield using the 1
:
2.5
:
2.5 ratio of 5a, triethyl orthoformate and cyanamide, respectively (Entry 10). The further increase of this ratio to 1
:
3
:
3 as well as changes of the reaction temperature did not improve the outcome of reaction. It should be noted that increase of the reaction temperature to 160 °C resulted in the substantial loss of the product purity. For comparison purposes, we also performed the reaction using conventional heating under reflux in ethyl acetate. The reflux of the reagents in the optimised ratio for 24 h enabled us to isolate the desired product 6a in 13% yield.
Table 1 Optimization of conditions for the synthesis of 8-phenyl-5-aza-7-deaza-adenine (6a)a
The developed method was validated using three different models of microwave synthesizers: Discover SP (CEM), Monowave 450 (Anton Paar), and Initiator+ (Biotage). The three-component reaction of 5a, triethyl orthoformate and cyanamide under optimised conditions was conducted with all three systems in triplicates. Similar results were obtained for all three reactors: 83%, 80%, and 75% for Discover SP, Monowave 450, and Initiator+, respectively. We also attempted to scale up our microwave-assisted reaction by a factor of ten. To our satisfaction, conducting the reaction with 10 mmol of 5a under identical conditions gave an even better yield (92%).
To test the scope of our developed method for the synthesis of 5-aza-7-deaza-adenines, we prepared a series of substituted 2-aminoimidazoles (5) using the method developed by Van der Eiken's group22 and then used them as substrates in our multicomponent reaction with triethyl orthoformate and cyanamide. The reaction of different 5 proceeded with the formation of the desired products 6. Various aromatic substituents with electron-withdrawing and electron-donating groups in different positions were tolerated on 5 in the reaction (Table 2).
Table 2 One pot, multicomponent synthesis of 7-aryl substituted 4-aminoimidazo[1,2-a][1,3,5]triazines (6)a
Microwave-assisted step performed on a 1 mmol scale in EtOAc (2 mL) using a Discover SP CEM microwave synthesiser. Microwave-assisted step performed on a 10 mmol scale in EtOAc (20 mL) using a Discover SP CEM microwave synthesiser. |
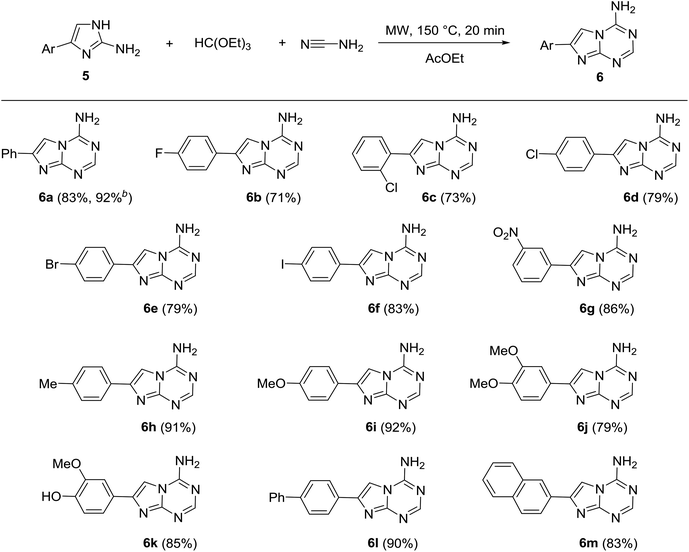 |
In principle, the formation of the aminotriazine ring in the three-component reaction of 2-aminoimidazoles 5 with triethyl orthoformate and cyanamide may proceed in four different ways (Scheme 1). Two regioisomeric pairs of isosteres of adenine (compounds 6 and 7) and isoadenine (compounds 8 and 9) could be theoretically obtained. However, only one product was isolated from the multicomponent reaction. The same product was obtained in the independent two-step synthesis using the general method reported earlier.23 Treatment of 5a with N,N-dimethylformamide dimethylacetal produced formamidine 11, which was found to exist in DMSO solution in the equilibrium with its tautomer 11′ (Scheme 2). The formamidine 11 further reacted with cyanamide in the presence of sodium methoxide affording the triazine ring formation. This approach excluded formation of 8 and 9, but the triazine ring closure might happen at any of the two imidazole nitrogen atoms resulting in 6 or 7. The unambiguous structure assignment for the products was done using X-ray crystallography of 6i (Fig. 1).
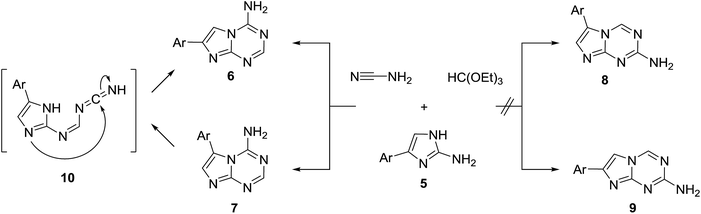 |
| Scheme 1 Possible annulations of the 1,3,5-triazine ring onto 2-amino-1H-imidazoles. | |
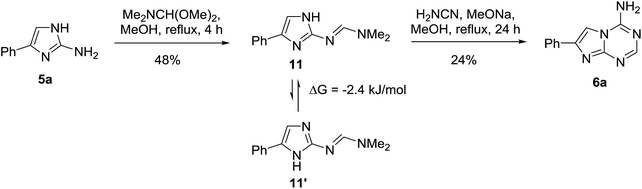 |
| Scheme 2 Step-wise synthesis of 6a. | |
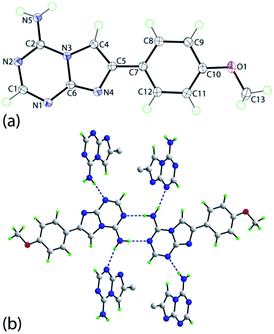 |
| Fig. 1 (a) Molecular structure of 6i showing atom labelling scheme and 70% anisotropic displacement parameters, and (b) a view of the N–H⋯N hydrogen bonding (blue dashed lines) leading to double-chains (see ESI Fig. S1‡). The outer four molecules are represented by only the ispo-C atom of the methoxyphenyl groups for reasons of clarity. | |
It was confirmed that our multicomponent reaction exclusively produced 4-amino-7-arylimidazo[1,2-a][1,3,5]triazines (6). Their regioisomers 7 are probably less stable due to steric hindrance between the amino group and the aryl; even if formed, they immediately rearrange at the reaction conditions to 6 (Scheme 1). The proposed rearrangement pathway involves formation of intermediate 10 resembling to the mechanism suggested for the amino-1,3,5-triazine ring rearrangement in a similar heterocyclic system.24 The reaction was found to be highly chemo- and regioselective. Compounds 6 were produced exclusively for all aminoimidazoles 5 involved in the reaction and no traces of other isomers were detected.
X-ray crystallography
The molecular structure of 6i is shown in Fig. 1a and comprises two planar fragments with the r.m.s. deviation of the nine atoms of the fused ring system being 0.015 Å. The maximum deviation from the least-squares plane is 0.022(1) Å for the N3 atom, and the amino-N5 atom lies 0.046(1) Å out of the plane in the opposite direction to N3. A twist in the molecule is indicated by the dihedral angle of 14.20(3)° between the imidazo[1,2-a][1,3,5]triazine and phenyl planes. Finally, the methoxy group is twisted out of the plane of the ring to which it is connected with the C13–O1–C10–C9 being 172.03(8)°.
The crystal of 6i comprises double-layers of hydrogen bonded molecules with a fragment of the array illustrated in Fig. 1b. Thus, centrosymmetric eight-membered {⋯HNCN}2 supramolecular synthons are formed between the amine-N–H and endocyclic nitrogen atoms of triazine residues with the remaining amine-N–H atom being connected to the second available triazine-N atom of a different molecule. The double-layers are formed parallel to [0 0 1] and are connected into a three-dimensional architecture via methyl-C–H⋯O(methoxy) and methyl-C–H⋯N(imidazo) non-conventional hydrogen bonding. The geometric details for the mentioned intermolecular contacts are given in the ESI Table S1‡ and a view of the unit cell contents is given in the ESI Fig. S1.‡
Conclusions
In summary, we have successfully developed a new, microwave-assisted, multicomponent reaction for the synthesis of 5-aza-7-deaza-adenines (6). The catalyst-free reaction was found to proceed with high selectivity and produce the desired product in good yields and purity. A rearrangement was suggested to explain regioselectivity of the ring closure.
Experimental section
General information
Melting points (uncorrected) were determined on a Stuart™ SMP40 automatic melting point apparatus. 1H and 13C NMR spectra were recorded on a Bruker Fourier 300 spectrometer (300 MHz) using DMSO-d6 as a solvent and TMS as an internal reference. Microwave-assisted reactions were carried out in the closed vessel focused single mode using a Discover SP microwave synthesizer (CEM, USA) monitoring reaction temperature by equipped IR sensor. For the method validation, the model reaction was also carried out using Monowave 450 (Anton Paar, Austria) and Initiator+ (Biotage, Sweden) reactors.
General method for the microwave-assisted synthesis of 5-aza-7-deaza-adenines (4-aminoimidazo[1,2-a][1,3,5]triazines, 6)
The mixture of a 2-amino-1H-imidazole (5, 1 mmol), cyanamide (105 mg, 2.5 mmol) and triethyl orthoformate (0.4 mL, 2.5 mmol) in ethyl acetate (2 mL) were irradiated in a 10 mL seamless pressure vial using microwave system operating at maximal microwave power up to 150 W at 150 °C for 20 min. After cooling, the precipitate was filtered, washed with ethyl acetate and recrystallised from DMF.
4-Amino-7-phenylimidazo[1,2-a][1,3,5]triazine (6a). Brown solid, yield 83%, mp 313–315 °C (DMF). 1H NMR (300 MHz, DMSO-d6): δ 7.37 (1H, t, 3J = 7.3 Hz, H-4′), 7.48 (2H, t, 3J = 7.5 Hz, H-3′ and H-5′), 7.88 (2H, d, 3J = 7.1 Hz, H-2′ and H-6′), 8.15 (1H, s, H-2), 8.23 (1H, s, H-6), 8.50 (2H, brs, NH2). 13C NMR (75 MHz, DMSO-d6): δ 102.1 (C-6), 125.3 (C-2′ and C-6′), 128.1 (C-4′), 128.8 (C-3′ and C-5′), 133.1 (C-1′), 143.5 (C-7), 149.6 (C-8a), 151.3 (C-4), 155.5 (C-2). Anal. calcd for C11H9N5: C, 62.55; H, 4.29; N, 33.16. Found: C, 62.35; H, 4.37; N, 33.02.
4-Amino-7-(4-fluorophenyl)imidazo[1,2-a][1,3,5]triazine (6b). Brown solid, yield 71%, mp 314–316 °C (DMF). 1H NMR (300 MHz, DMSO-d6): δ 7.32 (2H, dd, 3J = 9.0 Hz, 3JHF = 9.0 Hz, H-3′ and H-5′), 7.90 (2H, dd, 3J = 8.9 Hz, 4JHF = 5.5 Hz, H-2′ and H-6′), 8.14 (1H, s, H-2), 8.18 (1H, s, H-6), 8.47 (2H, brs, NH2). 13C NMR (75 MHz, DMSO-d6): δ 101.9 (C-6), 115.7 (d, 2JCF = 21.7 Hz, C-3′ and C-5′), 127.3 (d, 3JCF = 8.3 Hz, C-2′ and C-6′), 129.6 (d, 4JCF = 3.0 Hz, C-1′), 142.6 (C-7), 149.6 (C-8a), 151.3 (C-4), 155.5 (C-2), 162.0 (d, 1JCF = 244.8 Hz, C-4′). Anal. calcd for C11H8FN5: C, 57.64; H, 3.52; N, 30.55. Found: C, 57.55; H, 3.60; N, 30.28.
4-Amino-7-(2-chlorophenyl)imidazo[1,2-a][1,3,5]triazine (6c). Yellow solid, yield 73%, mp 312–314 °C (DMF). 1H NMR (300 MHz, DMSO-d6): δ 7.38 (1H, ddd, 4J = 1.8 Hz, 3J = 7.6 Hz, 3J = 7.6 Hz, H-4′), 7.47 (1H, ddd, 4J = 1.4 Hz, 3J = 7.6 Hz, 3J = 7.6 Hz, H-5′), 7.57 (1H, dd, 4J = 1.3 Hz, 3J = 7.9 Hz, H-3′), 7.95 (1H, s, H-2), 8.25 (1H, dd, 4J = 1.8 Hz, 3J = 7.9 Hz, H-6′), 8.55 (1H, s, H-6), 8.60 (2H, brs, NH2). 13C NMR (75 MHz, DMSO-d6): δ 106.3 (C-6), 127.3 (C-6′), 129.1 (C-5′), 130.3 (C-3′), 130.5 (C-4′), 130.6 (C-2′), 131.2 (C-1′), 140.0 (C-7), 148.6 (C-8a), 151.5 (C-4), 155.9 (C-2). Anal. calcd for C11H8ClN5: C, 53.78; H, 3.28; N, 28.51. Found: C, 53.59; H, 3.40; N, 28.45.
4-Amino-7-(4-chlorophenyl)imidazo[1,2-a][1,3,5]triazine (6d). Brown solid, yield 79%, mp 339–340 °C (DMF). 1H NMR (300 MHz, DMSO-d6): δ 7.54 (2H, d, 3J = 8.6 Hz, H-3′ and H-5′), 7.87 (2H, d, 3J = 8.6 Hz, H-2′ and H-6′), 8.15 (1H, s, H-2), 8.24 (1H, s, H-6), 8.52 (2H, brs, NH2). 13C NMR (75 MHz, DMSO-d6): δ 102.5 (C-6), 127.0 (C-2′ and C-6′), 128.9 (C-3′ and C-5′), 132.0 (C-1′), 132.5 (C-4′), 142.3 (C-7), 149.7 (C-8a), 151.3 (C-4), 155.7 (C-2). Anal. calcd for C11H8ClN5: C, 53.78; H, 3.28; N, 28.51. Found: C, 53.63; H, 3.36; N, 28.31.
4-Amino-7-(4-bromophenyl)imidazo[1,2-a][1,3,5]triazine (6e). Brown solid, yield 79%, mp 341–342 °C (DMF). 1H NMR (300 MHz, DMSO-d6): δ 7.68 (2H, d, 3J = 8.6 Hz, H-3′ and H-5′), 7.81 (2H, d, 3J = 8.6 Hz, H-2′ and H-6′), 8.14 (1H, s, H-2), 8.25 (1H, s, H-6), 8.51 (2H, brs, NH2). 13C NMR (75 MHz, DMSO-d6): δ 102.6 (C-6), 121.1 (C-4′), 127.3 (C-2′ and C-6′), 131.8 (C-3′ and C-5′), 132.3 (C-1′), 142.3 (C-7), 149.7 (C-8a), 151.3 (C-4), 155.7 (C-2). Anal. calcd for C11H8BrN5: C, 45.54; H, 2.78; N, 24.14. Found: C, 45.42; H, 2.88; N, 23.98.
4-Amino-7-(4-iodophenyl)imidazo[1,2-a][1,3,5]triazine (6f). Brown solid, yield 83%, mp 331–332 °C (DMF). 1H NMR (300 MHz, DMSO-d6): δ 7.66 (2H, d, 3J = 8.5 Hz, H-3′ and H-5′), 7.85 (2H, d, 3J = 8.5 Hz, H-2′ and H-6′), 8.14 (1H, s, H-2), 8.25 (1H, s, H-6), 8.49 (2H, brs, NH2). 13C NMR (75 MHz, DMSO-d6): δ 94.1 (C-4′), 102.5 (C-6), 127.3 (C-2′ and C-6′), 132.7 (C-1′), 137.6 (C-3′ and C-5′), 142.5 (C-7), 149.6 (C-8a), 151.3 (C-4), 155.6 (C-2). Anal. calcd for C11H8IN5: C, 39.19; H, 2.39; N, 20.77. Found: C, 39.03; H, 2.44; N, 20.59.
4-Amino-7-(3-nitrophenyl)imidazo[1,2-a][1,3,5]triazine (6g). Brown solid, yield 86%, mp 296–298 °C (DMF). 1H NMR (300 MHz, DMSO-d6): δ 7.79 (1H, t, 3J = 8.0 Hz, H-5′), 8.18 (1H, s, H-2), 8.21 (1H, ddd, 4J = 0.9 Hz, 4J = 2.3 Hz, 3J = 8.2 Hz, H-6′), 8.27 (1H, ddd, 4J = 1.0 Hz, 4J = 1.6 Hz, 3J = 7.6 Hz, H-4′), 8.44 (1H, s, H-6), 8.55 (2H, brs, NH2), 8.64 (1H, dd, 4J = 1.6 Hz, 4J = 2.3 Hz, H-2′). 13C NMR (75 MHz, DMSO-d6): δ 103.7 (C-6), 119.5 (C-2′), 122.6 (C-4′), 130.6 (C-5′), 131.3 (C-6′), 134.8 (C-1′), 141.1 (C-7), 148.3 (C-3′), 149.9 (C-8a), 151.5 (C-4), 156.0 (C-2). Anal. calcd for C11H8N6O2: C, 51.56; H, 3.15; N, 32.80. Found: C, 51.46; H, 3.27; N, 32.71.
4-Amino-7-(4-methylphenyl)imidazo[1,2-a][1,3,5]triazine (6h). Brown solid, yield 91%, mp > 380 °C (DMF). 1H NMR (300 MHz, DMSO-d6): δ 2.35 (3H, s, Me), 7.28 (2H, d, 3J = 7.9 Hz, H-3′ and H-5′), 7.76 (2H, d, 3J = 8.1 Hz, H-2′ and H-6′), 8.13 (1H, s, H-2), 8.17 (1H, s, H-6), 8.47 (2H, brs, NH2). 13C NMR (75 MHz, DMSO-d6): δ 20.8 (Me), 101.6 (C-6), 125.3 (C-2′ and C-6′), 129.3 (C-3′ and C-5′), 130.3 (C-1′), 137.5 (C-4′), 143.6 (C-7), 149.5 (C-8a), 151.2 (C-4), 155.3 (C-2). Anal. calcd for C12H11N5: C, 63.99; H, 4.92; N, 31.09. Found: C, 63.85; H, 5.06; N, 30.87.
4-Amino-7-(4-methoxyphenyl)imidazo[1,2-a][1,3,5]triazine (6i). Brown solid, yield 92%, mp > 380 °C (DMF). 1H NMR (300 MHz, DMSO-d6): δ 3.81 (3H, s, OMe), 7.05 (2H, d, 3J = 8.9 Hz, H-3′ and H-5′), 7.80 (2H, d, 3J = 8.9 Hz, H-2′ and H-6′), 8.10 (1H, s, H-6), 8.12 (1H, s, H-2), 8.43 (2H, brs, NH2). 13C NMR (75 MHz, DMSO-d6): δ 55.1 (OMe), 100.8 (C-6), 114.2 (C-3′ and C-5′), 125.6 (C-1′), 126.7 (C-2′ and C-6′), 143.6 (C-7), 149.5 (C-8a), 151.2 (C-4), 155.2 (C-2), 159.3 (C-4′). Anal. calcd for C12H11N5O: C, 59.74; H, 4.60; N, 29.03. Found: C, 59.66; H, 4.67; N, 28.91.
4-Amino-7-(3,4-dimethoxyphenyl)imidazo[1,2-a][1,3,5]triazine (6j). Brown solid, yield 79%, mp 252–254 °C (DMF). 1H NMR (300 MHz, DMSO-d6): δ 3.80 (3H, s, OMe), 3.85 (3H, s, OMe), 7.06 (1H, d, 3J = 8.4 Hz, H-5′), 7.39–7.45 (2H, m, H-2′ and H-6′), 8.11 (2H, s, H-6 and H-2), 8.40 (2H, brs, NH2). 13C NMR (75 MHz, DMSO-d6): δ 55.3 (OMe), 55.5 (OMe), 101.1 (C-6), 108.9 (C-2′), 112.0 (C-5′), 117.8 (C-6′), 125.9 (C-1′), 143.7 (C-7), 148.9 (C-3′ and C-4′), (C-8a), 151.1 (C-4), 155.2 (C-2). Anal. calcd for C13H13N5O2: C, 57.56; H, 4.83; N, 25.82. Found: C, 57.41; H, 4.60; N, 25.59.
4-Amino-7-(4-hydroxy-3-methoxyphenyl)-midazo[1,2-a][1,3,5]triazine (6k). Grey solid, yield 85%, mp 273–275 °C (DMF). 1H NMR (300 MHz, DMSO-d6): δ 3.87 (3H, s, OMe), 6.87 (1H, d, 3J = 8.2 Hz, H-5′), 7.29 (1H, dd, 4J = 1.9 Hz, 3J = 8.2 Hz, H-6′), 7.44 (1H, d, 4J = 1.9 Hz, H-2′), 8.07 (1H, s, H-6), 8.11 (1H, s, H-2), 8.39 (2H, brs, NH2), 9.21 (1H, s, OH). 13C NMR (75 MHz, DMSO-d6): δ 55.4 (OMe), 100.6 (C-6), 109.4 (C-2′), 115.8 (C-5′), 118.2 (C-6′), 124.5 (C-1′), 144.1 (C-7), 146.9 (C-3′), 147.7 (C-4′), 149.4 (C-8a), 151.1 (C-4), 155.1 (C-2). Anal. calcd for C12H11N5O2: C, 56.03; H, 4.31; N, 27.22. Found: C, 55.88; H, 4.54; N, 27.06.
4-Amino-7-(biphenyl-4-yl)imidazo[1,2-a][1,3,5]triazine (6l). Brown solid, yield 90%, mp > 380 °C (DMF). 1H NMR (300 MHz, DMSO-d6): δ 7.38 (1H, t, 3J = 7.3 Hz, H-4′′), 7.49 (2H, t, 3J = 7.5 Hz, H-3′′ and H-5′′), 7.74 (2H, d, 3J = 7.1 Hz, H-2′′ and H-6′′), 7.80 (2H, d, 3J = 8.5 Hz, H-3′ and H-5′), 7.96 (2H, d, 3J = 8.5 Hz, H-2′ and H-6′), 8.15 (1H, s, H-2), 8.27 (1H, s, H-6), 8.49 (2H, brs, NH2). 13C NMR (75 MHz, DMSO-d6): δ 102.2 (C-6), 125.9 (C-2′ and C-6′), 126.4 (C-2′′ and C-6′′), 127.0 (C-3′ and C-5′), 127.5 (C-4′′), 128.9 (C-3′′ and C-5′′), 132.2 (C-1′), 139.5 (C-1′′), 139.7 (C-4′), 143.1 (C-7), 149.7 (C-8a), 151.3 (C-4), 155.5 (C-2). Anal. calcd for C17H13N5: C, 71.06; H, 4.56; N, 24.37. Found: C, 70.94; H, 4.62; N, 24.28.
4-Amino-7-(napthalene-2-yl)imidazo[1,2-a][1,3,5]triazine (6m). Brown solid, yield 83%, mp 317–319 °C (DMF). 1H NMR (300 MHz, DMSO-d6): 1H NMR (300 MHz, DMSO-d6): δ 7.51–7.58 (2H, m, H-6′ and H-7′), 7.91–8.05 (4H, m, H-3′, H-4′, H-5′ and H-8′), 8.16 (1H, s, H-2), 8.36 (1H, s, H-6), 8.46 (1H, s, H-1′), 8.50 (2H, brs, NH2). 13C NMR (75 MHz, DMSO-d6): δ 102.7, 123.4, 124.1, 126.2, 126.5, 127.6, 128.1, 128.4, 130.5, 132.7, 133.1, 143.4, 149.8, 151.3, 155.6. Anal. calcd for C15H11N5: C, 68.95; H, 4.24; N, 26.80. Found: C, 68.79; H, 4.41; N, 26.63.
Step-wise approach for the synthesis of 4-amino-7-phenylimidazo[1,2-a][1,3,5]triazine (6a)
N′,N′-Dimethyl-N-[3(5)-phenylimidazolo-5(3)yl formamidine (11). A mixture of 2-amino-1H-imidazole 5a (320 mg, 2 mmol) with N,N-dimethylformamide dimethyl acetal (0.4 mL, 3 mmol) in methanol (5 mL) was heated under reflux for 4 h. Upon completion of the reaction, the solvent was removed under vacuum and the residue was triturated with diethyl ether. The precipitate was filtered and recrystallised from toluene to give pure formamidine 11. Brown solid, yield 58%, mp 133–135 °C (toluene). 1H NMR (300 MHz, DMSO-d6): δ 2.94 (3H, s, NMe), 3.07 (3H, s, NMe), 7.10 (2H, t, 3J = 7.3 Hz, H-4′), 7.19 (1H, brs, CH), 7.28 (2H, t, 3J = 7.7 Hz, H-3′ and H-5′), 7.66 (2H, d, 3J = 7.4 Hz, H-2′ and H-6′), 8.41 (1H, s, CH), 11.23 & 11.64 (1H, brs, NH). Anal. calcd for C12H14N4: C, 67.27; H, 6.59; N, 26.15. Found: C, 67.16; H, 6.64; N, 26.08.
4-Amino-7-phenylimidazo[1,2-a][1,3,5]triazine (6a). To the sodium methoxide solution prepared by dissolving sodium (60 mg, 2.5 mmol) in methanol (5 mL), formamidine 11 (215 mg, 1 mmol) and cyanamide (105 mg, 2.5 mmol) were added. The reaction mixture was heated under reflux for 24 h, cooled and the precipitate was filtered to obtain a compound, which was identical to 6a prepared using the multicomponent reaction. Yield 24%.
X-Ray crystallographic analysis
The crystals for X-ray diffraction study were grown from the very dilute MeOH solution. Intensity data for 6i were measured at T = 100(2) K on a SuperNova Dual AtlasS2 diffractometer fitted with Mo Kα radiation so that θmax was 33.0°. Data reduction, including absorption correction, was accomplished with CrysAlisPro.25 Of the 29
046 measured reflections, 3968 were unique (Rint = 0.017) and of these, 3510 data satisfied the I ≥ 2σ(I) criterion. The structure was solved by direct-methods26 and refined (anisotropic displacement parameters, C-bound H atoms in the riding model approximation, N-bound H atoms with N–H = 0.88 ± 0.01 Å and a weighting scheme w = 1/[σ2(Fo2) + 0.067P2 + 0.331P] where P = (Fo2 + 2Fc2)/3) on F2.27 Owing to poor agreement, the (−3 3 6) reflection was omitted from the final cycles of refinement. Based on the refinement of 170 parameters, the final values of R and wR (all data) were 0.040 and 0.114, respectively. The molecular structure diagram was generated with ORTEP for Windows28 and the packing diagram with DIAMOND.29
Crystal data for 4-amino-7-(4-methoxyphenyl)imidazo[1,2-a][1,3,5]triazine (6i). M = 241.26, monoclinic, P21/c, α = 6.97780(10), b = 12.4862(2), c = 12.6501(2) Å, β = 93.808(2)°, V = 1099.72(3) Å3, Z = 4, Dx = 1.457 g cm−3, F(000) = 504, μ = 0.100 mm−1. CCDC deposition number: 1578001.‡
Conflicts of interest
There are no conflicts to declare.
Acknowledgements
This work is supported by the Ministry of Higher Education, Malaysia under Fundamental Research Grant Scheme (FRGS). We would like to thank Nexus Analytics Sdn. Bhd. and Anton Paar Malaysia Sdn. Bhd. for their technical support.
Notes and references
- F. P. L. Lim, K. K. Kow, E. H. Yeo, S. C. Chow and A. V. Dolzhenko, Heterocycles, 2016, 92, 1121–1131 CrossRef CAS.
- G. Burnstock and A. Verkhratsky, Acta Physiol., 2009, 195, 415–447 CrossRef CAS PubMed.
-
(a) J. M. Murray and D. E. Bussiere, Methods Mol. Biol., 2009, 575, 47–92 CrossRef CAS PubMed;
(b) C. Volonté and N. D'Ambrosi, FEBS J., 2009, 276, 318–329 CrossRef PubMed;
(c) T. A. J. Haystead, Curr. Top. Med. Chem., 2006, 6, 1117–1127 CrossRef CAS PubMed.
- A. V. Dolzhenko, A. V. Dolzhenko and W.-K. Chui, Heterocycles, 2006, 68, 1723–1759 CrossRef CAS.
- A. V. Dolzhenko, A. V. Dolzhenko and W.-K. Chui, Heterocycles, 2008, 75, 1575–1622 CrossRef CAS.
- F. P. L. Lim and A. V. Dolzhenko, Eur. J. Med. Chem., 2014, 85, 371–390 CrossRef CAS PubMed.
- X. Jiao, D. J. Kopecky, J. Liu, J. Liu, J. C. Jaen, M. G. Cardozo, R. Sharma, N. Walker, H. Wesche, S. Li, E. Farrelly, S.-H. Xiao, Z. Wang and F. Kayser, Bioorg. Med. Chem. Lett., 2012, 22, 6212–6217 CrossRef CAS PubMed.
- P. Dao, N. Smith, C. Tomkiewicz-Raulet, E. Yen-Pon, M. Camacho-Artacho, D. Lietha, J.-P. Herbeuval, X. Coumoul, C. Garbay and H. Chen, J. Med. Chem., 2015, 58, 237–251 CrossRef CAS PubMed.
- J. Feng, S. L. Gwaltney, J. A. Stafford, M. B. Wallace and Z. Zhang, US Pat., 20060135767, 2006.
-
(a) D. Matosiuk and S. Fidecka, PL Pat., 186228, 2003;
(b) D. Matosiuk, S. Fidecka, L. Antkiewicz-Michaluk, J. Lipkowski, I. Dybala and A. E. Koziol, Eur. J. Med. Chem., 2002, 37, 761–772 CrossRef CAS PubMed;
(c) M. Rzadkowska, E. Szacoń, D. Matosiuk, E. Kedzierska and S. Fidecka, Acta Pol. Pharm., 2012, 69, 1270–1275 CAS;
(d) M. Rzadkowska, E. Szacon, D. Matosiuk, E. Kedzierska and S. Fidecka, PL Pat., 211865, 2012.
-
(a) F. Da Settimo, G. Primofiore, S. Taliani, C. La Motta, E. Novellino, G. Greco, A. Lavecchia, B. Cosimelli, M. ladanza, K.-N. Klotz, D. Tuscano, M. L. Trincavelli and C. Martini, Drug Dev. Res., 2004, 63, 1–7 CrossRef CAS;
(b) E. Novellino, E. Abignente, B. Cosimelli, G. Greco, M. Iadanza, S. Laneri, A. Lavecchia, M. G. Rimoli, F. Da Settimo, G. Primofiore, D. Tuscano, L. Trincavelli and C. Martini, J. Med. Chem., 2002, 45, 5030–5036 CrossRef CAS PubMed.
-
(a) J. O. Ojwang, US Pat., 20060035848, 2006;
(b) G. Gosselin, P. La Colla, F. Seela, R. Storer, D. Dukhan and F. Leroy, WO Pat., 2006000922, 2006;
(c) P. La Colla, G. Gosselin, F. Seela, D. Dukhan, and F. Leroy, WO Pat., 2004096197, 2004;
(d) J. O. Ojwang, S. Ali, D. F. Smee, J. D. Morrey, C. D. Shimasaki and R. W. Sidwell, Antiviral Res., 2005, 68, 49–55 CrossRef CAS PubMed;
(e) D. Dukhan, F. Leroy, J. Peyronnet, E. Bosc, D. Chaves, M. Durka, R. Storer, P. La Colla, F. Seela and G. Gosselin, Nucleosides, Nucleotides Nucleic Acids, 2005, 24, 671–674 CrossRef CAS PubMed.
-
(a) M. Bessodes, G. Bastian, E. Abushanab, R. P. Panzica, S. F. Berman, E. J. Marcaccio Jr, S.-F. Chen, J. D. Stoeckler and R. E. Parks Jr, Biochem. Pharmacol., 1982, 31, 879–882 CrossRef CAS PubMed;
(b) P.-F. Méry, C. Pavoine, F. Pecker and R. Fischmeister, in Phosphodiesterase Inhibitors, ed. C. S. Dent and K. F. Rabe, Academic Press, San Diego, 1996, pp. 81–88 Search PubMed.
- F. Da Settimo, G. Primofiore, C. La Motta, S. Taliani, F. Simorini, A. M. Marini, L. Mugnaini, A. Lavecchia, E. Novellino, D. Tuscano and C. Martini, J. Med. Chem., 2005, 48, 5162–5174 CrossRef CAS PubMed.
- F. Cameron and M. Sanford, Drugs, 2014, 74, 263–271 CrossRef CAS PubMed.
- M. Missbach, E. Altmann, L. Widler, M. Susa, E. Buchdunger, H. Mett, T. Meyer and J. Green, Bioorg. Med. Chem. Lett., 2000, 10, 945–949 CrossRef CAS PubMed.
- G. V. Ullas, C. K. Chu, M. K. Ahn and Y. Kosugi, J. Org. Chem., 1988, 53, 2413–2418 CrossRef CAS.
- F. C. Schaefer, J. Am. Chem. Soc., 1955, 77, 5922–5928 CrossRef CAS.
- J. Kobe, B. Stanovnik and M. Tisler, Chem. Commun., 1968, 1456 RSC.
-
(a) S.-H. Kim, D. G. Bartholomew, L. B. Allen, R. K. Robins, G. R. Revankar and P. Dea, J. Med. Chem., 1978, 21, 883–889 CrossRef CAS PubMed;
(b) T. Hanami, H. Oda, A. Nakamura, H. Urata, M. Itoh and Y. Hayashizaki, Tetrahedron Lett., 2007, 48, 3801–3803 CrossRef CAS;
(c) P. Cui, T. L. Macdonald, M. Chen and J. L. Nadler, Bioorg. Med. Chem. Lett., 2006, 16, 3401–3405 CrossRef CAS PubMed;
(d) P. Dao, C. Garbay and H. Chen, Tetrahedron, 2013, 69, 3867–3871 CrossRef CAS.
-
(a) F. P. L. Lim, G. Luna and A. V. Dolzhenko, Tetrahedron Lett., 2014, 55, 5159–5163 CrossRef CAS;
(b) F. P. L. Lim, G. Luna and A. V. Dolzhenko, Tetrahedron Lett., 2015, 56, 521–524 CrossRef CAS;
(c) F. P. L. Lim, G. Luna and A. V. Dolzhenko, Tetrahedron Lett., 2015, 56, 7016–7019 CrossRef CAS;
(d) A. V. Dolzhenko, S. A. Kalinina and D. V. Kalinin, RSC Adv., 2013, 3, 15850–15855 RSC;
(e) S. A. Kalinina, D. V. Kalinin and A. V. Dolzhenko, Tetrahedron Lett., 2013, 54, 5537–5540 CrossRef CAS.
-
(a) D. S. Ermolatev, E. P. Svidritsky, E. V. Babaev and E. Van der Eycken, Tetrahedron Lett., 2009, 50, 5218–5220 CrossRef CAS;
(b) D. S. Ermolat'ev and E. V. Van der Eycken, J. Org. Chem., 2008, 73, 6691–6697 CrossRef PubMed;
(c) D. S. Ermolat'ev, B. Savaliya, A. Shah and E. Van der Eycken, Mol. Diversity, 2011, 15, 491–496 CrossRef PubMed.
- D. V. Kalinin, S. A. Kalinina and A. V. Dolzhenko, Heterocycles, 2013, 87, 147–154 CrossRef CAS.
- Y. S. Agasimundin, F. T. Oakes and N. J. Leonard, J. Org. Chem., 1984, 50, 2474–2480 CrossRef.
- Rigaku Oxford Diffraction, CrysAlis PRO, Agilent Technologies Inc., Santa Clara, CA, USA, 2015 Search PubMed.
- G. M. Sheldrick, Acta Crystallogr., Sect. A: Found. Crystallogr., 2008, 64, 112–122 CrossRef CAS PubMed.
- L. J. Farrugia, J. Appl. Crystallogr., 2012, 45, 849–854 CrossRef CAS.
- G. M. Sheldrick, Acta Crystallogr., Sect. C: Struct. Chem., 2015, 71, 3–8 CrossRef PubMed.
- K. Brandenburg, DIAMOND, Crystal Impact GbR, Bonn, Germany, 2006 Search PubMed.
Footnotes |
† Part 31 in the series “Fused heterocyclic systems with an s-triazine ring.” For part 30 see ref. 1. |
‡ Electronic supplementary information (ESI) available. CCDC 1578001. For ESI and crystallographic data in CIF or other electronic format see DOI: 10.1039/c7ra11305f |
|
This journal is © The Royal Society of Chemistry 2017 |
Click here to see how this site uses Cookies. View our privacy policy here.