DOI:
10.1039/C8RA00590G
(Paper)
RSC Adv., 2018,
8, 13739-13746
Retracted Article: Chrysin attenuates myocardial ischemia–reperfusion injury by inhibiting myocardial inflammation
Received
22nd January 2018
, Accepted 28th March 2018
First published on 12th April 2018
Abstract
The aim of this study was to investigate the effects of chrysin (CH) on myocardial ischemia–reperfusion injury. Cytokines were reduced by CH in coronary artery occlusion-induced rats and also in H9C2 cells. The ST segment was also restored by CH. Triphenyltetrazolium chloride (TTC) staining and pathological analysis showed that CH could alleviate myocardial injury. Results in H9C2 cells showed that CH improved heart injury in hypoxia/reoxygenation (H/R) of H9C2 cells. In addition, the expressions of the HMGB1-related inflammation pathway in rats and H9C2 cells were significantly decreased by CH. The present study shows the protective effects of CH on myocardial injury via inflammation.
1. Introduction
Cardiovascular diseases (CVDs) remain the leading cause of mortality around the world. Theoretically, restoring the blood after ischemic myocardium will relieve the symptoms of ischemia. However, it may aggravate myocardial damage which is induced by ischemia/reperfusion (I/R) injury.1 The myocardial ischemia/reperfusion injury will cause plenty of diseases such as functional impairment of the heart, arrhythmia and apoptosis.2 Inflammation also participates in the process of I/R lesions in isolated hearts.3 Several researchers have reported that anti-apoptosis and anti-inflammation action can ameliorate the progress of I/R.4
High mobility group box-1 (HMGB1) is generally known as a non-histone DNA binding protein, which is involved in the stabilization of DNA and the promotion of transcription. Recently, HMGB1 has been found to be passively released by necrotic cardiomyocytes in response to ischemia and it also acts as an early mediator of inflammation following I/R injury.5 Moreover, HMGB1 is also actively secreted by immune cells such as monocytes, macrophages and dendritic cells, which further enhances the inflammatory reaction. More importantly, clinical data indicate that increased circulating HMGB1 levels are associated with cardiac and neurological outcomes, and even increased mortality, in cardiac disease patients.6,7
Chrysin is one of a large class of polyphenolic compounds present in the diet and many herbal products, which have long been associated with a variety of important biochemical and pharmacological activities in cancer prevention and health promotion.8–10 However, there has been little research exploring whether CH is beneficial for myocardial injury. Therefore, our study aimed to evaluate the effect of CH on myocardial injury.
2. Materials and methods
2.1 Reagents
CH was purchased from Sigma-Aldrich (St Louis, MO, USA). All the antibodies were provided by Cell Signaling Technology (Danvers, USA).
2.2 Animals
Thirty adult Sprague-Dawley rats (250–300 g) which were obtained from Sun Yat-sen University were fed in a controlled environment (23 ± 2 °C and 12 h light/dark cycle) for one week before the experiment. During the time of habituation, water and food were provided ad libitum. Rats were randomly divided into 3 groups as follows (n = 10): control group, model group (I/R), and CH group (I/R + CH, 50 mg kg−1). CH was administrated intragastrically for 5 days once a day before the operation, and the other group were administered with the same volume of vehicle (saline). All the experimental procedures were performed according to the National Institutes of Health Guidelines for the Care and Use of Laboratory Animals.
Ethical standards statement: all animal operations performed according to the ethical committee of the First Affiliated Hospital of Sun Yat-sen University.
2.3 Experimental protocol of the left coronary artery ligation
Rats were anesthetized and then restrained on the fixator. The trachea was incised and stitched with catgut for further use. A positive pressure respirator was used on the experimental rats. When we became conscious of a regular heart rhythm, we opened the skin above the heart, and made a blunt-dissection of the ribs under the skin. Meanwhile, we tied a line on the left anterior descending coronary for induction of coronary artery occlusion for 30 min. ST elevation was then used to confirm the ischemia. After that, the suture was untied for reperfusion. Then, the thoracic cavity was carefully sutured. The control group of rats was not tied. Rats were euthanized 24 h after the operation. Blood samples were collected from the carotid artery and centrifuged at 3500 g for 15 min, and the supernatant was stored at −80 °C for further analysis. Then the hearts of the rats were collected for other analysis.
2.4 Electrocardiogram (ECG) measurement
ECG was detected throughout the operation by a BL-420S Biologic Function Experiment system (Chengdu, China). ST segment elevation was deemed to be the symbol of ischemia which was recorded by ECG.
2.5 Determination of myocardial infarct size
The cardiac infarct area was evaluated using TTC (Sigma, St. Louis, MO, USA) staining. Generally, the heart was kept at −20 °C for 15 min and chopped into 5 pieces parallel to the coronary sulcus. All slices were incubated with PBS with 2% TTC at 37 °C for 15 min in the dark. TTC stained and non-TTC stained areas were analyzed with Image-Pro Plus image analysis software (Version 4.1, Media Cybernetics, LP, USA). The ratio of infarcted myocardium to the risk region was calculated as the whole myocardial tissues (infarct area/whole heart area) × percentage.
2.6 Cell culture
Rat cardiomyocyte H9C2 cells were obtained from the American Type Culture Collection and cultured with Dulbecco's modified Eagle's medium (DMEM, NanJing KeyGen Biotech Co., Ltd) containing 10% fetal bovine serum (FBS, Gibco), 100 IU mL−1 penicillin, and 100 IU mL−1 streptomycin in a humidified incubator at 37 °C under 5% CO2 atmosphere, with passage at preconfluent densities by use of 0.25% trypsin solution (Gibco) every 2 to 3 days.
2.7 Hypoxia/reoxygenation (H/R) injury model in vitro
H9C2 cells were incubated in a hypoxic/ischemic chamber (Anaerocult® A mini, Merck) in serum-free and low-glucose DMEM at 37 °C for 10 h in a humidified atmosphere of 5% CO2 and 95% nitrogen, and then incubated with fresh medium and restored to 95% nitrogen/5% CO2 for 2 h reoxygenation. At the onset of hypoxia, cardiomyocytes were randomly treated with 40 μM CH with Tyrode buffer as a vehicle. H9C2 cells cultured under normal conditions in a CO2 incubator served as negative controls.
2.8 Determination of inflammatory cytokines in serum and cell supernatant
The contents of IL-6 IL-1β and TNF-α in the serum and cell supernatant were measured using ELISA kits according to the manufacturer's instructions. The concentrations of the cytokines were quantified by reference to standard curves. The optical density (OD) of each well was read at 450 nm.
2.9 Determination of cardiac marker enzymes
The concentrations of troponin-I (Tn-I) and Tn-T, as well as CK and LDH activities in the obtained serum, were assayed by commercial kits according to the manufacturer's instructions.
2.10 Histological examination of myocardium
The hearts were harvested and fixed in 10% (v/v) neutral buffered formalin solution. They were embedded in paraffin and cut into 4 μm thick slices. The tissues were stained with H&E and observed by light microscopy (Nikon, Tokyo, Japan).
2.11 Immunohistochemistry staining
The expressions of HMGB1 and p-NF-κB in the heart were evaluated by immunohistochemistry staining. Briefly, the liver tissues were fixed with 4% paraformaldehyde (PFA), embedded in paraffin and sectioned. The paraffin sections were dewaxed in xylene and dehydrated ethanol, microwaved in sodium citrate buffer and washed with PBS. Endogenous peroxidase activity was blocked by 3% hydrogen peroxide for 20 min. Each sample was blocked with 5% goat serum for 20 min and then treated with primary antibodies at 4 °C overnight. Next day, after washing three times with PBS, each sample was treated with goat anti-rabbit IgG secondary antibody for 20 min and then incubated with a horseradish enzyme tag chain enzyme avidin working fluid for 20 min, and washed three times with PBS. Then, samples were stained with 3,3′-diaminobenzidine (DAB) and restained with hematoxylin. After dehydrating and drying, the sections were mounted with neutral gum and observed under a microscope.
2.12 Western blot
The heart and cells were homogenized and lysed in a RIPA buffer (Beyotime, Nanjing, China). Then, the tissues were centrifuged at 12
000 rpm for 20 min and the supernatant collected. The concentration of the protein was determined by a BCA protein assay (Beyotime, Nanjing, China). The samples were separated by 10% sodium dodecyl sulphate polyacrylamide gels and then transferred onto nitrocellulose membranes. The membranes were blocked with 5% skim milk for 2 h and incubated with specific antibodies at 4 °C for a whole night. After washing the membranes 4 times on the second day, the blots were incubated with horseradish peroxidase-conjugated secondary antibodies for 2 h. The content of the protein was shown by a gel imaging system (Tanon Science & Technology Co., Ltd., China).
2.13 Statistical analysis
Data are expressed as means ± SDs of at least three separate experiments. Statistical comparisons between experimental groups were performed by ANOVA with a Tukey multiple comparison test. A value of P < 0.05 was considered statistically significant.
3. Results
3.1 Effect of CH on myocardial infarct size
TTC staining was an important index for the development of ischemic injury. As shown in Fig. 1, there was a very low percentage of infarct in the control group, whereas the infarct size in the I/R group was increased over that in the control group. In contrast, treatment with CH notably reduced the infarct size.
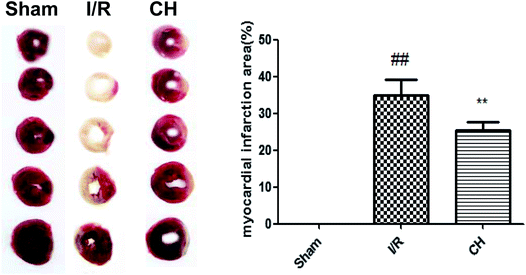 |
| Fig. 1 Effect of CH on myocardial infarct size in I/R-induced isolated hearts. The data are expressed as mean values ± SDs. #p < 0.05, ##p < 0.01 compared with the control group. *p < 0.05, **p < 0.01 compared with the I/R group. n = 10. | |
3.2 Effect of CH on myocardial histology
As can be seen in Fig. 2, the tissues in the control group showed an integrated myocardial membrane, a well-balanced myofibrillar structure and a continuous adjacent myofibril. However, the hearts which suffered from ligation exhibited plenty of inflammatory cells, myocardial cell swelling or degeneration, cardiac necrosis and loss of transverse striations. CH significantly ameliorated the above pathological changes.
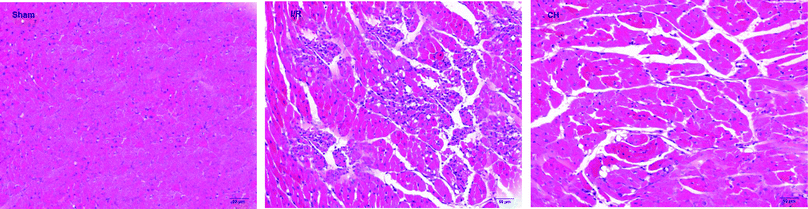 |
| Fig. 2 Effect of CH on myocardial histology. Original magnification (×200). n = 10. | |
3.3 Effects of CH on ST-segment elevation
The results of the electrocardiographs are revealed in Fig. 3. The ST-segment was elevated and the R-amplitude was decreased in the I/R group compared with those in the control group. CH obviously ameliorated the above changes, which provides partial evidence of its protective effects.
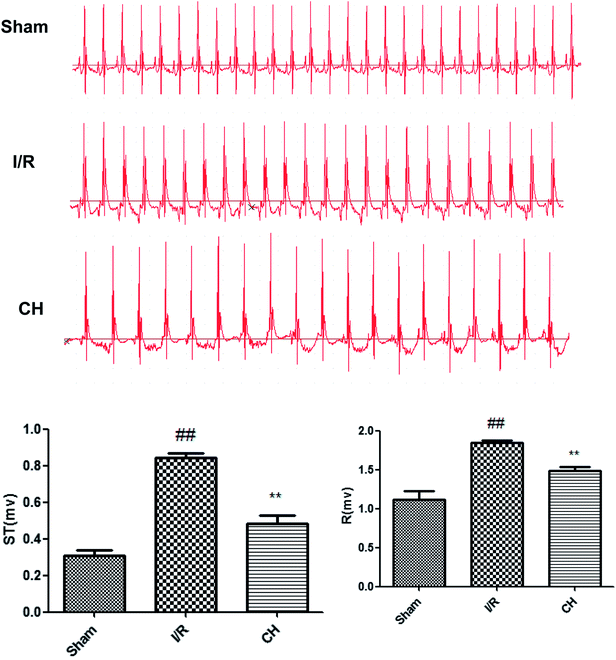 |
| Fig. 3 Effects of CH on ST-segment elevation. n = 10. | |
3.4 Effects of CH on inflammatory cytokines in serum and cell supernatant
The serum and cell supernatant levels of TNF-α, IL-6 and IL-1β were assessed. It was proved that there were significant increases in the serum and cell supernatant levels of TNF-α, IL-6 and IL-1β in the I/R group. In contrast, the serum and cell supernatant levels of TNF-α, IL-6 and IL-1β contents were effectively decreased in the CH-treated group compared with those in the I/R group rats. Our results indicated that CH reduced the inflammatory cytokine contents in the serum of I/R injury rats as well as in the cell supernatant (Fig. 4).
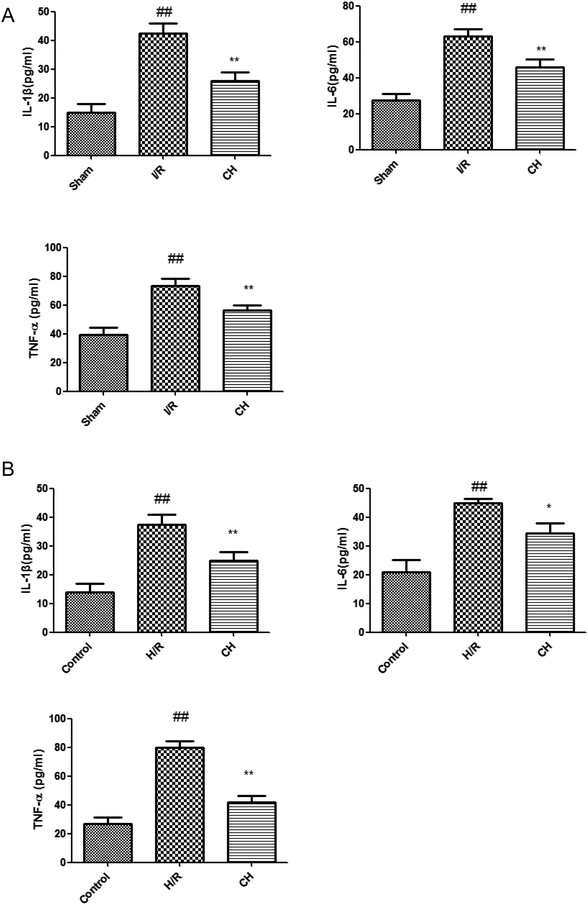 |
| Fig. 4 Effects of CH on inflammatory cytokines in serum (A) and cell supernatant (B) the data are expressed as mean values ± SDs. #p < 0.05, ##p < 0.01 compared with the control group. *p < 0.05, **p < 0.01 compared with the I/R group. n = 10. | |
3.5 Effects of CH on cardiac marker enzymes
The levels of CK, LDH and Tn-T in serum were measured in order to detect myocardial injury. As depicted in Fig. 5, the activities of CK, LDH and Tn-T were obviously increased in the I/R injury group compared with those in the control group. As expected, CH significantly decreased the levels of CK, LDH and Tn-T compared with the I/R group.
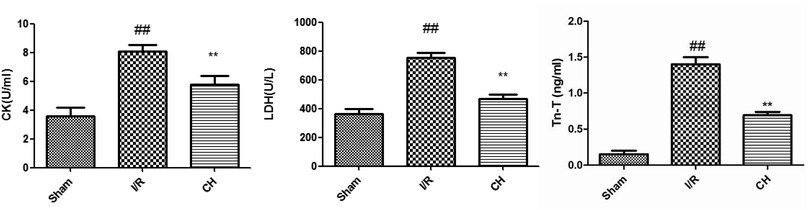 |
| Fig. 5 Effects of CH on cardiac marker enzymes. The data are expressed as mean values ± SDs. #p < 0.05, ##p < 0.01 compared with the control group. *p < 0.05, **p < 0.01 compared with the I/R group. n = 10. | |
3.6 Effects of CH on HMGB1and p-NF-κB by immunohistochemistry
As shown in Fig. 6, we found that the expressions of HMGB1 and p-NF-κB in the heart were significantly upregulated in heart tissues after I/R stimulation in coronary artery ligated rats. However, treatment with CH obviously inhibited the levels of HMGB and p-NF-κB.
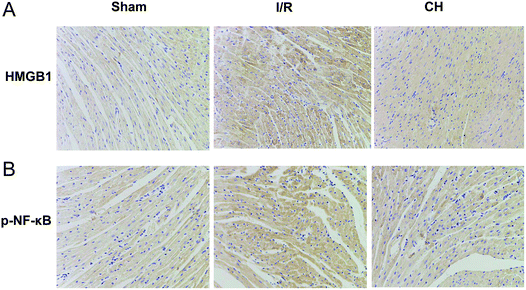 |
| Fig. 6 Effects of CH on HMGB1 (A) and p-NF-κB (B) by immunohistochemistry. n = 10. | |
3.7 Effects of CH on the HMGB1/NF-κB pathway in I/R-induced heart tissue
As shown in Fig. 7, we found that the expressions of the HMGB1/NF-κB pathway were significantly up-regulated in heart tissues after I/R stimulation in rats. However, treatment with CH obviously ameliorated these situations. The obtained results demonstrated that CH successfully reduced the expressions of the HMGB1/NF-κB pathway.
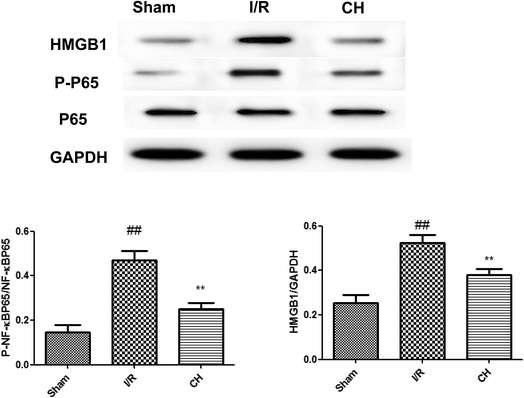 |
| Fig. 7 Effects of CH on the HMGB1/NF-κB pathway in coronary artery ligated rats. The data are expressed as mean values ± SDs. #p < 0.05, ##p < 0.01 compared with the control group. *p < 0.05, **p < 0.01 compared with the I/R group. n = 10. | |
3.8 Effects of CH on HMGB1/NF-κB in H9C2 cells
As shown in Fig. 8, we found that the expressions of the HMGB1/NF-κB pathway were significantly up-regulated in H9C2 cells. However, treatment with CH obviously inhibited the expressions of the HMGB1/NF-κB pathway.
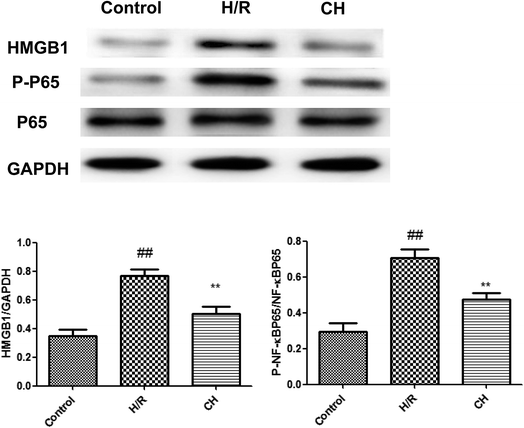 |
| Fig. 8 Effects of CH on HMGB1/NF-κB in H9C2 cells. The data are expressed as mean values ± SDs. #p < 0.05, ##p < 0.01 compared with the control group. *p < 0.05, **p < 0.01 compared with the H/R group. n = 10. | |
4 Discussion
Currently, there have been various interventions in cardiovascular diseases including thrombolytic therapy and coronary artery bypass grafting. However, the curative effect on I/R injuries with these treatments is not good. Thus, the treatment of I/R injury needed further research.
CH is an important type of flavone which can be found in many herbal products. It has been reported that CH exerts beneficial effects on the cardiovascular function, with anti-oxidant and anti-inflammation activity. Previous work has proved that CH could reduce ischemia–reperfusion injury in a Langendorff-perfused rat heart model (Testai et al. 2013).11 However, the rat acute myocardial infarction model which is established by LAD occlusion (at least 30 minutes) with reperfusion is much more stable and suitable for clinical research. Therefore, we investigated the protective effective effects of CH on myocardial ischemia–reperfusion injury and its underlying mechanism.
HMGB1, a chromosomal protein with high electrophoretic mobility, has distinctive roles depending upon cell location: in the nucleus, it promotes nuclear transcription, replication, recombination and DNR repair.12 On other hand, translocation of HMGB1 from the nucleus to the cytoplasm or the extracellular phase makes it act as a cytokine.13 Much evidence confirms that the HMGB1 mediated inflammatory reaction could be mediated through NF-κB, resulting in downstream danger signaling activation.14,15 The interaction of HMGB1-NF-κB leads to a trigger inflammatory reaction.16 NF-κB governs the mediation of inflammatory cytokine production, oxidative stress and apoptotic reaction.17 HMGB1-TLRs interaction can activate the NF-κB signaling pathway, and activated NF-κB plays a significant part in inflammatory phenotypic changes in endothelial dysfunction and various heart diseases.18 Our results confirmed that CH successfully inhibited the expression of HMGB1/NF-κB, which might be conducive to the suppression of NF-κB activation.
In conclusion, the present study investigated the cardioprotective role of CH on I/R injury-induced isolated heart tissues and tried to elucidate the potential mechanism of HMGB1/NF-κB induced apoptotic pathogenesis. Further detailed research is warranted in the future.
Conflicts of interest
All authors have no conflict of interest statement.
Acknowledgements
This work was supported by Guangdong Provincial Science and Technology Project (2016A020215054).
References
- Q. Hu, B. Wei, L. Wei, K. Hua, X. Yu and H. Li, et al., Sodium tanshinone IIA sulfonate ameliorates ischemia-induced myocardial inflammation and lipid accumulation in Beagle dogs through NLRP3 inflammasome, Int. J. Cardiol., 2015, 196, 183–192 CrossRef PubMed.
- L. Zhu, T. Wei, J. Gao, X. Chang, H. He and F. Luo, et al., The cardioprotective effect of salidroside against myocardial ischemia reperfusion injury in rats by inhibiting apoptosis and inflammation, Apoptosis, 2015, 20, 1433–1443 CrossRef CAS PubMed.
- M. Weinreuter, M. M. Kreusser, J. Beckendorf, F. C. Schreiter, F. Leuschner and L. H. Lehmann, et al., CaM Kinase II mediates maladaptive post-infarct remodeling and pro-inflammatory chemoattractant signaling but not acute myocardial ischemia/reperfusion injury, EMBO Mol. Med., 2014, 6, 1231–1245 CrossRef CAS PubMed.
- K. Hua, X. Sheng, T. T. Li, L. N. Wang, Y. H. Zhang and Z. J. Huang, et al., The edaravone and 3-n-butylphthalide ring-opening derivative 10b effectively attenuates cerebral ischemia injury in rats, Acta Pharmacol. Sin., 2015, 36, 917–927 CrossRef CAS PubMed.
- M. Andrassy, H. C. Volz, J. C. Igwe, B. Funke, S. N. Eichberger and Z. Kaya, et al., High-mobility group box-1 in ischemia-reperfusion injury of the heart, Circulation, 2008, 117, 3216–3226 CrossRef CAS PubMed.
- J. R. Klune, R. Dhupar, J. Cardinal, T. R. Billiar and A. Tsung, HMGB1: endogenous danger signaling, Mol. Med., 2008, 14, 476–484 CAS.
- H. C. Volz, D. Laohachewin, D. Schellberg, A. R. Wienbrandt, M. Nelles and C. Zugck, et al., HMGB1 is an independent predictor of death and heart transplantation in heart failure, Clin. Res. Cardiol., 2012, 101, 427–435 CrossRef CAS PubMed.
- M. Erden Inal and A. Kahraman, The protective effect of flavonol quercetin against ultraviolet a induced oxidative stress in rats, Toxicology, 2000, 154, 21–29 CrossRef CAS PubMed.
- M. Lee, M. Son, E. Ryu, Y. S. Shin, J. G. Kim, B. W. Kang, H. Cho and H. Kang, Quercetin-induced apoptosis prevents EBV infection, Oncotarget, 2015, 6, 12603–12624 Search PubMed.
- X. Chang, K. Zhang, R. Zhou, F. Luo, L. Zhu and J. Gao, et al., Cardioprotective effects of salidroside on myocardial ischemia-reperfusion injury in coronary artery occlusion-induced rats and Langendorff-perfused rat hearts, Int. J. Cardiol., 2016, 215, 532–544 CrossRef PubMed.
- L. Testai, A. Martelli, M. Cristofaro, M. C. Breschi and V. Calderone, Cardioprotective effects of different flavonoids against myocardial ischaemia/reperfusion injury in langendorff-perfused rat hearts, J. Pharm. Pharmacol., 2013, 65, 750–756 CrossRef CAS PubMed.
- Y. H. Qin, S. M. Dai, G. S. Tang, J. Zhang, D. Ren and Z. W. Wang, et al., HMGB1 enhances the proinflammatory activity of lipopolysaccharide by promoting the phosphorylation of MAPK p38 through receptor for advanced glycation end products, J. Immunol., 2009, 183(10), 6244–6250 CrossRef CAS PubMed.
- V. Karuppagounder, S. Arumugam, R. A. Thandavarayan, V. Pitchaimani, R. Sreedhar and R. Afrin, et al., Modulation of HMGB1 translocation and RAGE/NF kappaB cascade by quercetin treatment mitigates atopic dermatitis in NC/Nga transgenic mice, Exp. Dermatol., 2015, 24, 418–423 CrossRef CAS PubMed , pmid:25739980.
- J. R. van Beijnum, W. A. Buurman and A. W. Griffioen, Convergence and amplification of toll-like receptor (TLR) and receptor for advanced glycation end products (RAGE) signaling pathways via high mobility group B1 (HMGB1), Angiogenesis, 2008, 11(1), 91–99 CrossRef PubMed.
- J. J. de Haan, M. B. Smeets, G. Pasterkamp and F. Arslan, Danger signals in the initiation of the inflammatory response after myocardial infarction, Mediators Inflammation, 2013, 2013, 206039 CAS.
- J. Favre, P. Musette, V. Douin-Echinard, K. Laude, J. P. Henry and J. F. Arnal, et al., Toll-like receptors 2-deficient mice are protected against postischemic coronary endothelial dysfunction, Arterioscler., Thromb., Vasc. Biol., 2007, 27(5), 1064–1071 CrossRef CAS PubMed.
- K. Zhang, J. Liu, X. You, P. Kong, Y. Song and L. Cao, et al., P2X7 as a new target for chrysophanol to treat lipopolysaccharide-induced depression in mice, Neurosci. Lett., 2016, 613, 60–65 CrossRef CAS PubMed.
- E. Chorianopoulos, T. Heger, M. Lutz, D. Frank, F. Bea and H. A. Katus, et al., FGF-inducible 14-kDa protein (Fn14) is regulated via the RhoA/ROCK kinase pathway in cardiomyocytes and mediates nuclear factor-kappaB activation by TWEAK, Basic Res. Cardiol., 2010, 105, 301–313 CrossRef CAS PubMed.
Footnote |
† These authors contributed equally to this work. |
|
This journal is © The Royal Society of Chemistry 2018 |
Click here to see how this site uses Cookies. View our privacy policy here.