DOI:
10.1039/C8RA02616E
(Paper)
RSC Adv., 2018,
8, 15973-15984
Efficiency of brown seaweed (Sargassum longifolium) polysaccharides encapsulated in nanoemulsion and nanostructured lipid carrier against colon cancer cell lines HCT 116
Received
26th March 2018
, Accepted 23rd April 2018
First published on 30th April 2018
Abstract
Bioactive polysaccharides extracted from brown seaweeds have potent antioxidant, antitumor, antibacterial, antiviral, anti-inflammatory activities and nanomedicine applications. In the present study, we have made an attempt to overcome the instability and bioavailability problem of exopolysaccharides extracted from brown seaweed (Sargassum longifolium) by nanoencapsulation technology to enhance its therapeutic applications. Exopolysaccharides was encapsulated in orange oil nanoemulsion (NE) prepared by ultra-sonication method and nanostructured lipid carrier (NLC) prepared by hot solvent diffusion method. The encapsulation efficiency of nanoemulsion was 67.29% and of nanostructured lipid carrier was 78.7%. The prepared nano carriers have particle size 178 nm (NE), 153 nm (NLC) and zeta potential −43.9 mV (NE), −60 mV (NLC). In vitro release kinetics of exopolysaccharides from NE (80%) and NLC (95%) was found to be slow and sustained release which indicates increase in bioavailability. The cytotoxic effect of seaweed polysaccharide, nanocarriers loaded with seaweed polysaccharide was analyzed by MTT method in colon cancer (HCT 116) cell lines with the results revealing that seaweed polysaccharide encapsulated with NLC (80%) was superior to that encapsulated with orange oil nanoemulsion (70%). This is the first report demonstrating the potential of brown seaweed exopolysaccharide encapsulated in orange oil nanoemulsion and nanostructured lipid carrier for its biomedical application.
1. Introduction
Seaweeds are natural resources with bioactive compounds which are used for food, medical1 and industrial products.2 Seaweed is a nutraceutical compound as it is a food with medical and health benefits. Seaweed is an important source of marine polysaccharides that belong to three different families which are classified based on its morphology, cell wall and pigment composition as green (Chlorophyceae), red (Rhodophyceae) and brown (Phaeophyceae) seaweeds.3,4 Seaweeds are found in very harsh marine environment with lots of bioactive metabolites with varied functions. For centuries, seaweeds have been used as a food throughout Asia and because of its high nutrient content seaweeds are of high pharmaceutical interest.5,6 The seaweed polysaccharides are different from those of terrestrial plant polysaccharides.7 They are formed by linking monosaccharides together. The main difference between the seaweed polysaccharides and other polysaccharides is the surface charge. Seaweed polysaccharides are anionic in nature because of the presence of anionic sulfate groups that are absent in polysaccharides of terrestrial origin.8 Though seaweeds are composed of various bioactive compounds, 76% of them are polysaccharides. The structure of those polysaccharides is directly related to the taxonomy and cell structure of seaweed. The major bioactive compounds of seaweeds are polysaccharides and apart from this the other compounds are phenolic, phlorotannins, proteins, peptides, amino acids, terpenes, terpenoids, lipids, and halogenated compounds. Numerous bioactive polysaccharides with interesting functional properties have been discovered from seaweeds.9 Polysaccharides from brown seaweeds have been reported to have potent bioactive functions like anti-inflammatory activity, anti-oxidant activity and anti-proliferative effect on various cancer cells.6 Polysaccharides from Sargassum species showed anticancer activity on lung cancer and melanoma,10 colon and breast cancer cell lines.11,12 Additionally, polysaccharides derived from marine seaweeds were also used to treat goiter, glandular problems, wounds, burns and rashes. It is also being used in bone replacement therapy. Seaweed polysaccharides are used as anticancer agents as it is involved in (i) release of pro-inflammatory cytokines (ii) increase of NK cells activity (iii) apoptosis induction (iv) TLR-4, CD 14 activation.13 Seaweed polysaccharides are considered as a source of dietary fiber because they are not completely digested by human intestinal enzymes and thus interfere with the bioavailability of other dietary components.14 Seaweeds contain a large amount of carbohydrate as source of polysaccharides. There are several methods to extract polysaccharides. All those are time consuming and they are in need of large amount of organic solvents to extract and precipitate polysaccharides which causes a major problem of environmental pollution. Thus hot water extraction method takes its place by overcoming all these limitations. Hot water extracts of polysaccharides from seaweeds were used in the treatment of cancer by Chinese people.15 Since the carbohydrate content in seaweed is considerably high, its greater portion is available as polysaccharide fiber which decreases the bioavailability because it is not taken by the human body. Studies have revealed that high fiber content food can help in reducing the risk of certain cancers, lowers cholesterol and keeps blood sugar balanced. Reports evoke that because of the large size of the macromolecules, a significant portion of it is unable to pass the cell barrier of the gastrointestinal tract. Due to the instability of the nutraceuticals under conditions encountered during processing or in the gut (pH, enzymes, presence of other nutrients) it is difficult to retain the health benefits of those bioactive molecules.16 Therefore, it is necessary to protect these molecules from harsh conditions by encapsulation. Several studies have reported that the bioavailability of the nutraceuticals can be enhanced by increasing the bio accessibility of them during the digestion in small intestine. Nanomedicine is being potently used for rational and targeted delivery for pharmaceutical, therapeutic and diagnostic agents to specific cells and extracellular elements of the body. The mechanism involved in nanoparticles to pass through the gastrointestinal tract barriers includes mainly the particle size. Encapsulation of seaweed polysaccharide increases the efficiency of the bioactive nutraceutical ingredients. Nano carriers with lipids, biosurfactants and water has improved its effectiveness by high entrapment of compound and very low toxic effect because of the components used are generally recognized as safe.17 Essential oils are naturally derived aroma compounds synthesized as secondary metabolites from plants.18 Orange oil obtained from citrus peel is widely used in the food industries as flavoring agent because of its volatile constituents with unique aroma profiles. Orange oil is a complex organic compound with main components being limonene and linalool which are classified as terpenes.19 Nanoemulsions are fine oil-in-water dispersions, having droplet covering the size range from 100–500 nm.20 The essential oils are dispersed in an aqueous medium and stabilized by an emulsifier. Yet another nanoencapsulation technique used is nanostructured lipid carrier (NLC), type of lipid nanocarrier with solid matrix. Advantages of NLC are enhanced drug loading capacity, physical and chemical long term stability, sustained release and prevention of drug expulsion during storage. In our study polysaccharides from Sargassum longifolium was obtained by hot water extraction and ethanol precipitation. Encapsulation of extracted crude polysaccharides was performed using essential oils, solid lipids and biosurfactants for nano carrier formulations. Hence by nanosizing seaweed polysaccharide extracts, it might be efficient enough in bypassing the gastrointestinal tract. Till date no report has been found on nanosizing crude seaweed polysaccharide synthesis by nanoemulsion and NLC technique. Although seaweed polysaccharides have various biological functions, nanosized seaweed polysaccharides have not been investigated yet.
2. Materials and methods
2.1 Materials
Orange oil, biosurfactants and solid lipid (Span 80, Pluronic L81, lecithin, poloaxmer 188, stearic acid), DMEM, FBS, MTT, DMSO were purchased from Sigma (St. Louis, Mo, USA). Solvents (ethanol, acetone, chloroform, n-butanol, acetonitrile, methanol and acetonitrile) were purchased from SRL, INDIA. HCT 116 colon cancer cell lines and 3T3 mouse normal fibroblast cell lines was procured from NCCS (National Centre for Cell Science), Pune, INDIA. All the other chemicals were used of analytical grade.
2.2 Sample collection and preparation
Sargassum longifolium, brown seaweed was collected from coastal areas of Gulf of Mannar (Tamil Nadu, India. Latitude 9.1278° N and Longitude 79.4662° E) region during low tide in sterile polythene bags with seawater and brought to laboratory. Collected seaweeds were kept in ice and gently washed for few times with sterile distilled water to remove the unwanted debris and dried in air. The air dried seaweeds were grinded into powder and kept in a plastic box covered with aluminum foil for further investigation.
2.3 Extraction of crude polysaccharides
Hot water extraction method21,22 was followed with slight modification for extracting polysaccharides from brown seaweed (Sargassum longifolium) extract. Three parameters (Table 1) were studied with different extraction temperature (20, 40, 60, 80 and 100 °C), different pH (2, 4, 7 and 9) and different extraction time (15, 30, 60 and 120 min) to obtain high yield of crude polysaccharides (Table 2). The pH 2 and 4 were adjusted by addition of 2 mol l−1 HCl and pH 9 with 2 mol l−1 NaOH. Dried Sargassum longifolium seaweed powder (1
:
4 w/v) was boiled in deionized water with the above parameters followed by homogenization using magnetic stirrer to extract the bioactive components from the powder. After extraction, the solution was centrifuged at 7155 × g for 15 min and supernatant was collected. The extraction process was repeated three times. The supernatants were pooled and concentrated by a rotary evaporator under vacuum at 45 °C. The concentrates were lyophilized with a freeze-dryer. The lyophilized aqueous seaweed extract was prepared in deionized water (1 mg ml−1) and the polysaccharide fraction was precipitated by the addition of three volumes of 100% ethanol and kept overnight at −20 °C. The precipitated polysaccharide was collected by centrifugation at 5478 × g for 20 min at 4 °C and lyophilized with a freeze dryer to obtain crude seaweed polysaccharide.
Table 1 Polysaccharides yield by following hot water extraction method with different parameters
Method |
Parameters |
Polysaccharides yield |
Temperature (°C) |
pH |
Time (min) |
Ethanol (%) |
Storage temperature (°C) |
Hot water extraction method |
20 |
9 |
15, 30, 60, 120 |
50, 75, 95, 100 |
4, −20 |
1.38% |
2 |
40 |
9 |
15, 30, 60, 120 |
50, 75, 95, 100 |
4, −20 |
1.52% |
2 |
60 |
9 |
15, 30, 60, 120 |
50, 75, 95, 100 |
4, −20 |
1.88% |
2 |
80 |
9 |
15, 30, 60, 120 |
50, 75, 95, 100 |
4, −20 |
3.76% |
2 |
100 |
9 |
15, 30, 60, 120 |
50, 75, 95, 100 |
4, −20 |
10.38% |
2 |
Table 2 Polysaccharides yield by following hot water extraction method
S. no. |
Temperature (°C) |
pH |
Time (min) |
Ethanol (%) |
Storage temperature (°C) |
Polysaccharides yield |
1 |
100 |
2 |
180 |
100 |
−20 |
10.38% |
2.4. Deproteinization of crude polysaccharides
The crude polysaccharides (5 mg) was further dissolved in 10 ml water and partitioned five times with Sevag reagent (chloroform
:
n-butanol, 4
:
1) to remove protein.23 Two layers were formed from which the aqueous layer was taken carefully and mixed with three volumes of 100% ethanol and incubated overnight for precipitation at −20 °C. The precipitated sample was again centrifuged at 5478 × g for 15 min and lyophilized to obtain dried seaweed polysaccharides.
2.5 Preparation of nanoemulsion
Nanoemulsions were prepared with essential oil (orange oil) and biosurfactants (Span 80 and Pluronic L81) by high shear stirring followed by ultra-sonication method at room temperature24 (Table 3). The advantage of using both hydrophilic (Pluronic L81) and lipophilic (Span 80) surfactants is to maintain the synergistic effect on emulsion stability. Essential oils and Span 80 (10
:
1) were mixed together to form oil phase, then 1% Pluronic L81 was dissolved in deionized water to form the water phase by stirring in the beaker. Nanoemulsions were formed by adding the water phase to the oil phase under stirring condition for 30 min at room temperature. Then the formed emulsion was ultra-sonicated for 30 min using probe sonicator (30 s ON and 10 s OFF. 40% amplitude, 140 WATT) and the reaction mixture was stirred at room temperature for 24 hours. Polysaccharide loaded nanoemulsions were prepared by dissolving 5 mg of crude seaweed polysaccharide to the organic phase followed by other steps as mentioned for blank nanoemulsions. All the prepared nanoemulsions were kept at 25 °C for further study.
Table 3 Composition of blank and seaweed polysaccharide (SP) loaded nanoemulsion and NLC formulation
Formulation |
SA (mg) |
PO (mg) |
OO (mg) |
Span 80 + Pluronic L61 (%) |
Lecithin (mg) |
Poloxamer (%) |
Water (ml) |
SP (mg) |
SP – seaweed polysaccharide. |
Orange oil |
|
|
10 |
1 |
|
|
50 |
— |
SPa orange oil |
|
|
10 |
1 |
|
|
50 |
5 |
NLC P |
50 |
|
|
|
20 |
1 |
50 |
— |
SP NLC P |
50 |
|
|
|
20 |
1 |
50 |
5 |
To separate and purify the nanoparticles, 25–30 ml of acetone was added to break the emulsion followed by bath sonication for 15 min and centrifuged at 7155 × g for 15 min. Finally the resultant nanoparticles were washed 3–5 times with acetone and water. The samples were then lyophilized to produce polysaccharide nanoparticle in a powdered form.
2.6 Preparation of nanostructured lipid carrier (NLC)
Nanostructured lipid carrier was formulated by hot solvent diffusion method25 method. Briefly, 20 mg of soy lecithin, 50 mg of stearic acid and 50 mg of orange oil (melted at 72–75 °C) were fully dissolved into ethanol
:
acetone (40
:
60 v/v) mixture at 60 °C and kept for stirring. The organic solution was then rapidly poured into 50 ml of aqueous solution containing 1% (w/v) Poloxamer 188 kept under magnetic stirring at 80 °C. The suspension was then cooled to room temperature and the organic solvents were evaporated under reduced pressure using rotary evaporator and the final volumes were adjusted to 20 ml. This suspension was filtered through 8 μm filter paper and freeze dried to obtain into powder form. The final formulations are shown in Table 3.
2.7 Nanoparticle characterization
The Z-average, polydispersity index (PDI) and zeta potential was analyzed by dynamic light scattering using Malvern Zetasizer instrument.26–28 The mean particle size is a measure of the distribution of nanoparticle population. PDI is a dimensionless measure of the width of the size distribution calculated by the instrument. Zeta potential is a parameter characterizing electrochemical equilibrium on interfaces. Electrostatic repulsion between particles depends on the value of zeta potential. Measuring zeta potential is very useful for the assessment of the physical stability of colloidal dispersions. The particle surface develops a charge due to ionization of surface groups or adsorption of ions in the suspension. The charge obtained depend both on surface chemistry of particles and media surrounded by these particles. Measurements were carried out at 25 °C with a scattering angle of 90°. The samples were diluted about 1
:
100 times in deionized water just before the measurements. All the measurements were performed in triplicate.
2.8 Scanning electron microscopy (SEM)
The morphological characteristics of seaweed polysaccharide, nanoemulsions and NLC were examined using scanning electron microscope (EVO-18 plus, Carl Zeiss, Germany). The samples were diluted with deionized water and sonicated for 30 s in probe sonicator. The samples were prepared by fixing one drop of the sample on the glass slide. After air dried, the samples were observed under HR-SEM to evaluate the morphological characteristics.
2.9 Transmission electron microscopy (TEM)
The structure of crude polysaccharides loaded orange oil nanoemulsion and NLCs were examined by high resolution transmission electron microscope (HR-TEM, Tecnai T20) using negative staining technique. The nano carrier formulated samples were diluted with deionized water and 10 μl of the diluted sample was applied on to the copper grid and air dried for 30 min at room temperature. For negative staining 10 μl of 1% phosphotungstic acid solution was dropped on the sample grid and allowed to dry at room temperature for 10 min. Photographs were taken at accelerating voltage of 200 kV.
2.10 Fourier transform infrared spectroscopy (FTIR)
FTIR spectra reveal the characteristic peaks of all the functional groups present in the sample. FTIR spectra of essential oils, seaweed polysaccharide and seaweed polysaccharide nanoparticles in KBr pellets were analyzed using an FTIR spectrometer between the range of 500 cm−1 to 4000 cm−1.
2.11 Entrapment efficiency
Seaweed polysaccharide entrapment efficiency29 in nanoemulsion and NLCs were studied by completely breaking down the NLCs. A volume of 2 ml of seaweed polysaccharide loaded nanoemulsion and NLCs were taken and centrifuged at 12
000 rpm for 30 min. The supernatant was then diluted with acetonitrile and methanol mixture (1
:
1). 1 ml of the formulated NLCs were added to 4 ml of and acetonitrile methanol mixture and centrifuged for 20 min at 8000 rpm and the supernatant was analyzed by UV-VIS spectrophotometer at 490 nm by Dubois et al. method. The entrapment efficiency was calculated by using eqn (1). |
 | (1) |
2.12 In vitro release
The release of seaweed polysaccharide from the nanoemulsion and NLCs were performed using dialysis membrane bags with 6000–8000 Da pore size.29 The dialysis membrane bags were soaked in water overnight ahead of use. The bags were filled with 2 ml of 5 mg ml−1 seaweed polysaccharide nanoparticle loaded NLCs. The bags were immersed in enzyme free simulated intestinal medium (SIM) and simulated gastric fluid medium (SGF) rotated at 50 rpm at 37 °C. A total of 1 ml of sample was taken at sequential time interval followed by replacing the same amount of releasing media soon after withdrawal. The samples were analyzed by UV-VIS spectrophotometer at 490 nm by Dubois et al. method. For each formulation, the release study was performed in triplicate.
2.13 Cell culture
HCT 116 (human colon carcinoma) cell lines and 3T3 mouse normal fibroblast cell lines were cultured and maintained in DMEM supplemented with 10% (v/v) FBS. The cells were incubated at 37 °C in a humidified 95% air or 5% CO2 environment by seeding into 96 well plates at the density of 200
000 cells per wells in the media. Different concentration of seaweed polysaccharide and the same loaded into nanoemulsion and NLC were dissolved in deionized water and sonicated for one hour to get rid of aggregation.
The medium with 10% FBS was removed after 8 h, and replaced by 20 μl of samples from low to high concentrations. Controls were cells treated with an equal volume of serum free medium without any samples. Cells were incubated for another 48 h before the cell viability assay was performed.
2.14 Mitochondrial activity
In vitro cytotoxicity30 of seaweed polysaccharide and the same loaded into nanoemulsion and NLC was assessed using the MTT (3-(4,5-dimethylthiazol-2-yl)-2,5-diphenyl tetrazoniumbromide) assay to measure the succinate dehydrogenase mitochondrial activity. Fresh media with 100 μl of 5 mg ml−1 MTT stain was pipetted into every well and incubated for 4 h. The media was removed and the formation purple color crystals were further dissolved with DMSO. The absorbance was read at 570 nm in microplate reader (BIOTEK, POWER WAVE XS2). Blank was kept as medium without cells and negative control as medium without samples. All experiments were repeated thrice to ensure reproducibility. Cell viability was expressed as the percent absorbance relative to that obtained for cells not exposed to samples.
3. Results
3.1 Yield of crude polysaccharide
Sargassum longifolium (Fig. 1A) belonging to the class Phaeophyceae was shade dried and ground into powder (Fig. 1B) to extract polysaccharides (Fig. 1C) using hot water extraction method. The yield of crude polysaccharides obtained from hot water extraction was showed in Table 1. The extraction process gave different yields of crude polysaccharides depending on the heating temperature, pH, extraction time, ethanol concentration and storage temperature. The highest yield was 10.38% with heating temperature 100 °C, pH 2, extraction time 180 min, 100% absolute ethanol for precipitation and −20 °C for storage.
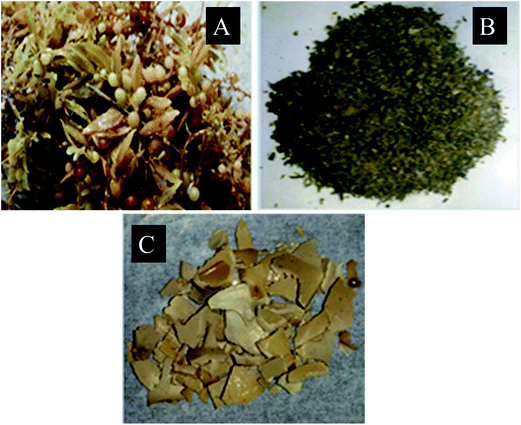 |
| Fig. 1 (A) Sargassum longifolium, (B) ground seaweed powder and (C) as extracted seaweed polysaccharide. | |
3.2 Encapsulation of crude polysaccharides in nanocarriers
Different ratios of orange oil Span 80 and Pluronic L81 were used to find out the suitable nanoemulsion formation. Similarly different ratios of stearic acid, orange oil, lecithin and Poloaxmer 188 were formulated for NLC. The optimized ratios for both the nanocarriers are demonstrated in Table 3. The concentration of crude polysaccharides was kept constant for both formulations as 5 mg ml−1.
3.3 Physiochemical characteristics of nanocarriers encapsulated with crude polysaccharides
The Z-average, polydispersity index (PI) and zeta potential of the formulated nanocarriers was obtained by dynamic light scattering (DLS). The obtained values are tabulated in Table 4 and Fig. 4A. The particle size distribution of crude seaweed polysaccharide loaded orange oil nanoemulsion was 178 nm with PI 0.009 and zeta potential −43.9 mV. NLC loaded with crude polysaccharide ranged with size 203 nm, 0.18 PI and −60 mV zeta potential.
Table 4 Particle size, PDI, zeta potential and entrapment efficiency of the formulations
Formulation |
Size (nm) |
PDI |
Zeta potential |
EE (%) |
SP – seaweed polysaccharide. |
Orange oil |
111 |
0.564 |
−39.2 mV |
|
SPa orange oil |
178.7 |
0.009 |
−43.9 mV |
67.29 |
NLC |
123 |
0.29 |
−59 mV |
|
SPa NLC |
153 |
0.18 |
−60 mV |
78.7 |
3.4 SEM and TEM
The surface morphologies of the formulated samples were examined using scanning electron microscopy (SEM) and transmission electron microscopy (TEM). Crude seaweed polysaccharide (Fig. 2A and 3A) showed irregular shape which is highly undesirable for biomedical applications. But the nanocarrier images relatively smoother surface morphology. Nanoemulsion (Fig. 2B and 3B) shape was not fully spherical but its clear shape and size adds to its advantage. NLC (Fig. 2C and 3C) was fully spherical with very small size proving to be the best suitable nanocarrier.
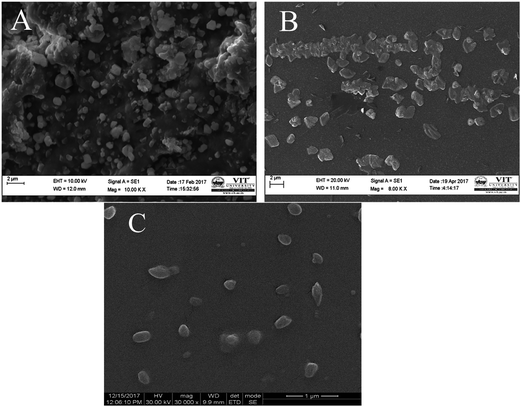 |
| Fig. 2 SEM image of (A) as extracted seaweed polysaccharide (B) orange oil nanoemulsion encapsulated with seaweed polysaccharide (C) NLC encapsulated with seaweed polysaccharide. | |
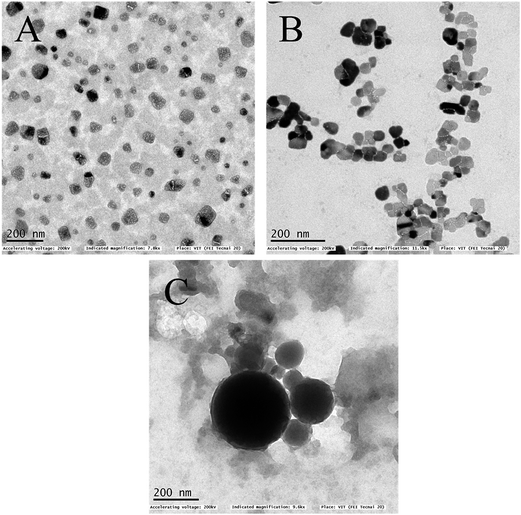 |
| Fig. 3 TEM image of (A) as extracted seaweed polysaccharide (B) orange oil nanoemulsion encapsulated with seaweed polysaccharide (C) NLC encapsulated with seaweed polysaccharide. | |
3.5 Fourier transform infra-red spectroscopy (FTIR)
Functionality of seaweed polysaccharide loaded and unloaded in nanoemulsion and NLCs were shown in (Fig. 4B–D). It is observed that the presence of functional groups in both the cases is the same, showing that nanoemulsion and nanostructured carrier processing did not change the chemical functionality of the polysaccharides. The characteristic peaks for seaweed polysaccharide are 1381 cm−1, 1126 cm−1, 1087 cm−1, 1037 cm−1, 650 cm−1, 597 cm−1 and 482 cm−1 (Table 5) while the characteristic peaks for orange oil is similar to limonene 1641 cm−1, 1438 cm−1 and 885 cm−1. The polysaccharides peaks are not found in the loaded one and thus it indicates that the polysaccharide has been encapsulated into emulsion (Table 6). The characteristic peaks for NLC are 1639 cm−1, 1465 cm−1, 1350 cm−1, 1056 cm−1, and 885 cm−1. The corresponding seaweed polysaccharide peaks are absent in the loaded NLC which also indicates that the polysaccharide has been encapsulated into NLC (Table 7).
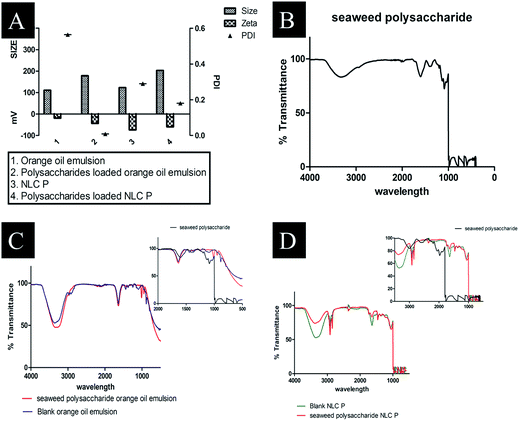 |
| Fig. 4 (A) DLS size distribution of prepared formulations (B) FTIR spectra of seaweed polysaccharide (C) FTIR spectra of blank orange oil nanoemulsion and seaweed polysaccharide loaded orange oil nanoemulsion (D) FTIR spectra of blank NLC and seaweed polysaccharide loaded NLC. | |
Table 5 List of infrared (IR) vibrational modes characteristic to polysaccharides
Frequency |
Seaweed polysaccharide |
Bond |
Functional groups |
3327.21 |
O–H stretch |
Alcohol |
2939.52 |
O–H stretch |
Carboxylic acids |
1600.92 |
C–C stretch |
Carbonyl group |
1381.03 |
SO |
Sulphate groups |
1126.43 |
C–O stretch |
Carboxylic acids |
1087.85 |
RO–SO3− |
Sulphate groups |
1037.70 |
RO–SO3− |
Sulphate groups |
650.01 |
C–Cl stretch |
Alkyl halides |
597.93 |
C–Br stretch |
Alkyl halides |
Table 6 Characteristic peaks of blank and seaweed polysaccharide loaded orange oil nanoemulsion
Frequency |
Blank orange oil |
Seaweed polysaccharide loaded orange oil nanoemulsion |
Bond |
Functional groups |
Bond |
Functional groups |
3360 |
O–H stretch |
Alcohol |
O–H stretch |
Alcohol |
2966.52 |
|
|
O–H stretch |
Carboxylic acids |
2922.16 |
O–H stretch |
Carboxylic acids |
|
|
1641.42 |
–C C–stretch |
Alkenes |
|
|
1637.56 |
|
|
N–H bend |
Primary amine |
1438.90 |
C–C stretch |
Aromatics |
|
|
1249.94 |
C–N stretch |
Aromatic amines |
|
|
1097.50 |
C–N stretch |
Aliphatic amines |
|
|
1010.70 |
|
|
C–O stretch |
Alcohols, carboxylic acids, esters, ethers |
948.98 |
|
|
O–H stretch |
Carboxylic acids |
885.33 |
C–H stretch |
Aromatics |
|
|
790.81 |
C–Cl stretch |
Alkyl halides |
|
|
538.14 |
C–Br stretch |
Alkyl halides |
|
|
Table 7 Characteristic peaks of blank and seaweed polysaccharide loaded NLC
Frequency |
Blank NLC |
Seaweed polysaccharide loaded NLC |
Bond |
Functional groups |
Bond |
Functional groups |
3375.43 |
|
|
O–H stretch |
Alcohol |
3363.86 |
O–H stretch |
Alcohol |
|
|
2916.37 |
O–H stretch |
Carboxylic acids |
O–H stretch |
Carboxylic acids |
2850.79 |
C–H stretch |
Alkanes |
C–H stretch |
Alkanes |
2360.87 |
C N stretch |
Nitriles |
C N stretch |
Nitriles |
1732.08 |
|
|
C O stretch |
Carbonyls |
1639.49 |
–C C–stretch |
Alkenes |
–C C–stretch |
Alkenes |
1512.19 |
|
|
N–O asymmetric stretch |
Nitro compounds |
1465.90 |
C–C stretch (in-ring) |
Aromatics |
C–C stretch (in-ring) |
Aromatics |
1369.46 |
|
|
C–H rock |
Alkanes |
1350.17 |
C–H rock |
Alkanes |
|
|
1219.01 |
|
|
–CH2X |
Alkyl halides |
1056.99 |
C–O stretch |
Alcohols, carboxylic acids, esters, ethers |
C–O stretch |
Alcohols, carboxylic acids, esters, ethers |
721.38 |
|
|
C–H rock |
Alkanes |
3.6 Entrapment efficiency and release study
Entrapment efficiency of the formulated nanocarriers was calculated using eqn (1) and found that seaweed polysaccharide loaded orange oil nanoemulsion was 67.29 ± 2% (Fig. 5A) and in seaweed polysaccharide loaded NLC was 78.7 ± 2% (Fig. 5B). Thus keeping 80% as control for release study seaweed polysaccharide from orange oil nanoemulsion and NLC formulations were analyzed in vitro in enzyme free simulated intestinal medium. Over a period of 12 h ∼80% of seaweed polysaccharide was released from orange oil nanoemulsion and ∼95% was released from NLC. Slow and sustained release of seaweed polysaccharide was noted (Fig. 5C and D). From the results high entrapment and more amount of seaweed polysaccharide release was seen in NLC when compared to nanoemulsion formulation.
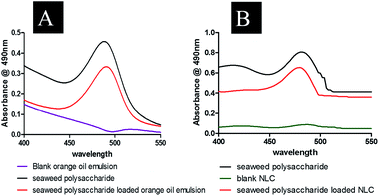 |
| Fig. 5 Encapsulation efficiency of (A) seaweed polysaccharide in orange oil nanoemulsion (B) seaweed polysaccharide in NLC. | |
3.7 Cytotoxic activity
The cytotoxicity activity of seaweed polysaccharide encapsulated with nanoemulsion and NLC was analyzed with MTT assay against normal mouse fibroblast 3T3 (Fig. 6) and colon cancer cell lines HCT 116 (Fig. 7). The cytotoxic activity was performed for seaweed polysaccharide, orange oil, biosurfactants, nanoemulsion and NLC. From the results we infer that the seaweed polysaccharide, emulsion and NLC exhibited no inhibitory effect on normal mouse fibroblast cell lines (99.9 ± 0.05% viability), whereas seaweed polysaccharide alone provides cytotoxicity activity against HCT 116 cells but the activity was more efficient when loaded into nanoemulsion and NLC nanocarriers. The percentage of cell viability in seaweed polysaccharide loaded NLC is 23 ± 0.7% at 1000 μg ml−1. 80% of cells was killed at this concentration. At the same concentration seaweed polysaccharide loaded nanoemulsion kills 70 ± 0.4% of cells whereas the seaweed polysaccharide alone kills 55 ± 0.6%. Thus seaweed polysaccharide encapsulated with NLC has better efficiency when compared to nanoemulsion and seaweed polysaccharide alone (Fig. 8).
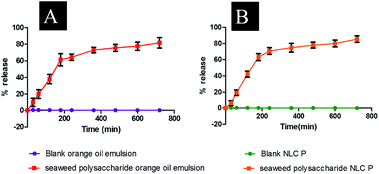 |
| Fig. 6 Release study of seaweed polysaccharide from (A) orange oil nanoemulsion (B) NLC. | |
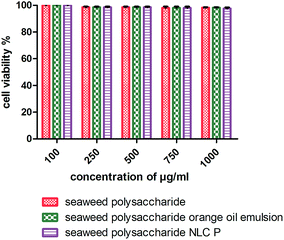 |
| Fig. 7 Cell viability of unloaded and loaded seaweed polysaccharide in orange oil nanoemulsion and NLC in 3T3 mouse normal fibroblast cell lines. | |
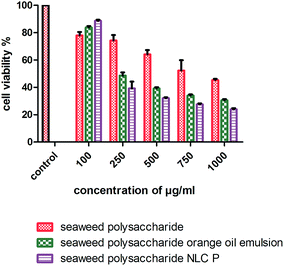 |
| Fig. 8 Cell viability and proliferation of unloaded and loaded seaweed polysaccharide in orange oil nanoemulsion and NLC in HCT 116 colon cancer cell lines. | |
4. Discussion
4.1 Extraction and yield of water soluble polysaccharides
In this study, extraction of crude seaweed polysaccharides was done according to the procedure of ref. 21 and 22 with slight modification. The seaweed extract was boiled in distilled water at 100 °C by adjusting the pH to 2 with hydrochloric acid (HCl). The sample was allowed to boil for 3 h and the supernatant was collected and precipitated with 100% ethanol to obtain high yield of crude polysaccharides. The result obtained was similar to the studies reported by ref. 31 because of the parameters used in the hot water extraction method. The yield of crude polysaccharide from brown seaweed Sargassum longifolium in this study was higher (10.38%) than in previous reports for S. latifolium (4.75%),29 S. fulvellum (8.9%) and S. thunbergii (9.6%).32 The difference in the yield compared to other reports might be because of the higher acid concentration (2 mol l−1 HCl) used in the present study. The other reason for low yield is due to the different solubility of the polysaccharides in the solvents.33
4.2 Importance of nanocarriers
Marine polysaccharides have different biomedical applications and have drawn its attention in the present research. Seaweed derived polysaccharides has extended its potential activities against cancer and virus. Polysaccharides, the major bioactive compound of seaweeds have a wide role in nutraceutical and medical applications.13 Though seaweed polysaccharides are being used in a list of applications, the efficiency is still low because of its large size and irregular shape. Till date there are so many reports stating that polysaccharides from seaweeds have a potent role in treating various cancers. The conventional mean of drug administration is oral route for patients requiring long term treatment. Hence nanoparticle approach for natural products has been explored. Natural products encapsulated in inert systemic nanocarriers tend to be more active and efficient than their original nature because of their small size, easy penetration into the cells and cell organelles, large surface area, enhanced bioavailability and long term stability.34 Advantage of using nanoemulsion as a nanocarrier is that it protects the encapsulated compound from hydrolysis and oxidation. It is also considered to be effective and safe with increased bioavailability.35 NLC is a nanocarrier with the same advantages of nanoemulsion but because of its disrupted matrices, the major advantage of NLC leads to higher drug entrapment and sustained release which ultimately enhances drug absorption when compared with other lipid based formulations having uniform matrices of lipids.36 In our study the efficiency of both nanoemulsion and NLC nanocarriers encapsulated with seaweed derived polysaccharides has been studied and compared.
4.3 Size distribution and zeta potential
In Table 4 the particle size distribution of the nanocarrier formulation is tabulated. In order to improve the bioavailability of the seaweed polysaccharide, nanoemulsion and NLC nanocarrier encapsulated with seaweed polysaccharide were formulated using ultra sonication and hot solvent diffusion method respectively. The main role of nanocarriers is their size as it determines the bio accessibility of the entrapped compounds by affecting the gastrointestinal tract residence time, dissolution rate and action of digestive enzymes.37 From the literature it was noted that generally particles with <200 nm are preferred for oral delivery. Particle size analysis confirms that all the formulations were within the required range. The particle size and PI of orange oil nanoemulsion was 111 nm and 0.5 whereas for NLC 153 nm and 0.18 respectively. Addition of seaweed polysaccharide to the nanocarriers increased the particle size but PI was decreased. This increase in particle size indicates the incorporation of seaweed polysaccharide into the formulated matrix and decrease in PI reveals the homogenous distribution of the particle in the media. The surface charge density (zeta potential) represents the in vitro stability of the formulated nano carriers. The preferable range for determining good stability is more than ±30 mV. The surface charge densities of the nanoemulsion and NLC (blank and seaweed polysaccharide loaded) indicated excellent stability as it ranged from −30 mV to −60 mV. Similar study was reported38 where NLC prepared by solvent diffusion method using stearic acid and oleic acid showed size ranging from 150 nm to 380 nm in blank and in drug loaded NLC 160 nm to 430 nm. The PI of this fabricated NLC ranges from 0.005 to 0.4 and zeta potential from −40 to −50 mV.
4.4 Entrapment and release study
Seaweed polysaccharide is considered as a nutraceutical because it is used both as a food and medicine. But to show its potent therapeutic benefit nanosize of this seaweed polysaccharide is found to be successful by encapsulating it into nano carriers. From the literature it was stated that38 the drug entrapment efficiency in NLC prepared by solvent diffusion method ranged from 45 to 70%. Ref. 39, 40 and 41 resulted that the embodiment of liquid lipids to solid lipids could lead to enormous crystal order disturbance, and the resulting matrix of lipid particles indicates deformity in the crystal lattice and leaves adequate space to attune drug molecules, thus, leading to enhanced drug entrapment efficiency. Reports stated that Antrodia camphorata polysaccharides encapsulated in silica chitosan nanoparticles showed encapsulation efficiency 66% and with silica nanoparticles alone was 63.5%.42 Thus high entrapment efficiency is observed due to the lipophilic nature of our fabricated nanocarriers with 67.29 ± 2% in nanoemulsion and 78.7 ± 2% in NLC. High entrapment of seaweed polysaccharide was seen in NLC when compared to nanoemulsion. This may be because of the irregular matrix of the NLC which incorporates more amounts of compounds.
The release of seaweed polysaccharide from nanoemulsion and NLC was tested in enzyme free SIM (simulated intestinal medium). Gastrointestinal tract is an unfavorable environment for seaweed polysaccharide as it may degrade before reaching the intestine. Thus, seaweed polysaccharide is protected by encapsulating into nanocarriers so it passes safely until it reaches the intestine. In the intestine the formulated nanocarriers form into a micelle like structure as it is composed of bile salts, phospholipids and lipid degradation products like monoglycerides, fatty acids etc.43 The seaweed polysaccharide release behavior from nanocarriers (nanoemulsion and NLC) did not exhibit a biphasic pattern with burst release initially followed by sustained release. The release in our study was controlled release from the beginning and high amount of release was seen from NLC compared to nanoemulsion. This may be because of the lecithin which helps in hydrolysis of the formulated NLC by digestive enzymes.38
4.5 Cytotoxic activity against normal mouse fibroblast cells and colon cancer cell lines
Polysaccharides from brown seaweed cannot be hydrolyzed by digestive enzymes in the human small intestine and therefore it proves to be efficient compound to prevent colon carcinogenesis.31 It becomes necessary to study the toxicity of anti-cancer compounds in normal cells as the compound should not compromise normal cells. The error bars for normal cell line 3T3 mouse fibroblast cells is very low because the values were very close to the control (more than 95% of cells were viable). The compounds (seaweed polysaccharide, orange oil nanoemulsion and NLC) were non-toxic to 3T3 mouse fibroblast normal cell line. Similar results have been found in human normal dermal fibroblasts cell line in the presence of betulinic acid obtained from various traditional plants.44 Thus, these compounds seem to be selective for tumor cells which matches with the very minimal toxicity against normal cells observed during our study. Previous studies have reported that seaweed polysaccharides exhibit anti-cancer effects, metastasis and angiogenesis in various cancer cells.11,45 Studies have reported that fucoidan induced apoptosis in HCT-15 human colon cancer cells at a concentration of 100 μg ml−1. In this study crude seaweed polysaccharide kills 55% of cancer cells at 1000 μg ml−1. But nanocarriers loaded with crude polysaccharides killed 70–80% of cancer cells proving to be more efficient. The cell viability test was also done for individual components of nanocarriers like orange oil, biosurfactants stearic acid. At low concentrations (10–20 μg ml−1) these components exerts anti-cancer activity by killing cancer cells. But at higher concentration (>50 μg ml−1) it helps in proliferating the cancer cells thus inhibiting apoptosis. But the formulated nanocarriers with seaweed polysaccharide possessed efficient activity against HCT 116 cells even at higher concentrations upto 1 mg ml−1. Studies have reported that NLCs loaded with bioactive compounds exhibited enhanced cell growth inhibition in in vitro cell viability assay when compared to unloaded bioactive compounds.29 The mechanism involved in the selective and reduced toxicity of compounds to normal cells as compared to tumor cells is that cancer cells which are capable of rapid proliferation are more effectively killed by these compounds whereas the normal cell populations which have lower proportion of cells remain alive because of their slow entry into the S phase of cell cycle. This selective toxicity is because the compounds reduce the saturation density of untransformed cells (normal cells) thus arresting them in the G1 phase of the cell cycle.46
5. Conclusions
In summary, encapsulation of seaweed polysaccharides in orange oil nanoemulsion and NLC exhibited successfully and showed significantly increased cytotoxicity against colon cancer cell lines HCT 116. From the results we infer that the seaweed polysaccharide encapsulated in NLC formulated by hot solvent diffusion method has high entrapment efficiency with reliable particle size and zeta potential when compared to orange oil nanoemulsion and the release profile of polysaccharide from NLC was high with sustained pattern compared to nanoemulsion. Though both the nanocarriers encapsulated with seaweed polysaccharide exhibited good anticancer activity, the NLC takes a better place when compared with nanoemulsion. Therefore, encapsulating seaweed polysaccharides in nanocarriers illustrates a promising approach to provide cytotoxic activity with improved efficacy and safety.
Conflicts of interest
There are no conflicts of interest.
Acknowledgements
The authors are grateful for the support provided by VIT University and Madurai Kamaraj University to carry out the research work. Saghya Infant Shofia acknowledges the Maulana Azad National Fellowship (UGC-MANF) for the financial support (F117.1/201516/MANF201517PON48888/(SAIII/Website)).
References
- A. J. Smit, J. Appl. Phycol., 2004, 16, 245–262 CrossRef CAS.
- K. H. Cardozo, T. Guaratini, M. P. Barros, V. R. Falcão, A. P. Tonon, N. P. Lopes, S. Campos, M. A. Torres, A. O. Souza and P. Colepicolo, Comp. Biochem. Physiol., Part C: Toxicol. Pharmacol., 2007, 146, 60–78 CrossRef PubMed.
- K.-X. Yu, I. Jantan, R. Ahmad and C.-L. Wong, Parasitol. Res., 2014, 113, 3121–3141 CrossRef PubMed.
- Singh A., Bhat T. K. and Sharma O. P., Clinical biochemistry of hepatotoxicity, J. Clin. Toxicol., 2011, 4(001), 1–19 Search PubMed.
- H. Stegenga, Bot. Mar., 2011, 54, 109 Search PubMed.
- M. T. Ale and A. S. Meyer, RSC Adv., 2013, 3, 8131–8141 RSC.
- L. G. Ferreira, M. D. Noseda, A. G. Gonçalves, D. R. Ducatti, M. T. Fujii and M. E. Duarte, Carbohydr. Res., 2012, 347, 83–94 CrossRef CAS PubMed.
- J. Venkatesan, S. Anil, S.-K. Kim and M. S. Shim, Polymers, 2016, 8, 30 CrossRef.
- W. Black, J. Mar. Biol. Assoc. U. K., 1954, 33, 49–60 CrossRef CAS.
- M. T. Ale, H. Maruyama, H. Tamauchi, J. D. Mikkelsen and A. S. Meyer, Int. J. Biol. Macromol., 2011, 49, 331–336 CrossRef CAS PubMed.
- E. J. Kim, S. Y. Park, J.-Y. Lee and J. H. Y. Park, BMC Gastroenterol., 2010, 10, 96 CrossRef PubMed.
- Y. Yamasaki-Miyamoto, M. Yamasaki, H. Tachibana and K. Yamada, J. Agric. Food Chem., 2009, 57, 8677–8682 CrossRef CAS PubMed.
- N. Ruocco, S. Costantini, S. Guariniello and M. Costantini, Molecules, 2016, 21, 551 CrossRef PubMed.
- M. S. Kim, J. Y. Kim, W. H. Choi and S. S. Lee, Nutr. Res. Pract., 2008, 2, 62–67 CrossRef CAS PubMed.
- H. Ye, K. Wang, C. Zhou, J. Liu and X. Zeng, Food Chem., 2008, 111, 428–432 CrossRef CAS PubMed.
- A. Jiménez-Escrig and F. Sánchez-Muniz, Nutr. Res., 2000, 20, 585–598 CrossRef.
- L. Montenegro, F. Lai, A. Offerta, M. G. Sarpietro, L. Micicchè, A. M. Maccioni, D. Valenti and A. M. Fadda, J. Drug Delivery Sci. Technol., 2016, 32, 100–112 CrossRef CAS.
- K. Bouchemal, S. Briançon, E. Perrier and H. Fessi, Int. J. Pharm., 2004, 280, 241–251 CrossRef CAS PubMed.
- F. Donsì and G. Ferrari, J. Biotechnol., 2016, 233, 106–120 CrossRef PubMed.
- Y. Chang and D. J. McClements, J. Agric. Food Chem., 2014, 62, 2306–2312 CrossRef CAS PubMed.
- T. Ozawa, J. Yamamoto, T. Yamagishi, N. Yamazaki and M. Nishizawa, J. Nat. Med., 2006, 60, 236–239 CrossRef CAS PubMed.
- A. Kantachumpoo and A. Chirapart, Kasetsart J.: Nat. Sci., 2010, 44, 220–233 CAS.
- K. F. Akhter, M. A. Mumin, E. K. Lui and P. A. Charpentier, ACS Biomater. Sci. Eng., 2015, 2, 96–103 CrossRef.
- Z. Du, C. Wang, X. Tai, G. Wang and X. Liu, ACS Sustainable Chem. Eng., 2016, 4, 983–991 CrossRef CAS.
- C. L. Dora, J.-L. Putaux, I. Pignot-Paintrand, F. Dubreuil, V. Soldi, R. Borsali and E. Lemos-Senna, J. Braz. Chem. Soc., 2012, 23, 1972–1981 CrossRef CAS.
- Y. Liu, F. Wei, Y. Wang and G. Zhu, Colloids Surf., A, 2011, 389, 90–96 CrossRef CAS.
- P. Izquierdo, J. Feng, J. Esquena, T. F. Tadros, J. C. Dederen, M. J. Garcia, N. Azemar and C. Solans, J. Colloid Interface Sci., 2005, 285, 388–394 CrossRef CAS PubMed.
- A. K. Sachan, A. Gupta and M. Arora, J. Drug Delivery Ther., 2016, 6, 4–13 CAS.
- N. Aditya, M. Shim, I. Lee, Y. Lee, M.-H. Im and S. Ko, J. Agric. Food Chem., 2013, 61, 1878–1883 CrossRef CAS PubMed.
- X.-Y. Yang, Y.-X. Li, M. Li, L. Zhang, L.-X. Feng and N. Zhang, Cancer Lett., 2013, 334, 338–345 CrossRef CAS PubMed.
- M. Mohsen, S. F. Mohamed, F. Ali and O. H. El-Sayed, J. Appl. Sci. Res., 2007, 3, 1178–1185 CAS.
- J. Kang, M. Khan, N. Park, J. Cho, M. Lee, H. Fujii and Y. Hong, J. Ethnopharmacol., 2008, 116, 187–190 CrossRef CAS PubMed.
- A. Usov, G. Smirnova, Z. Kamenarska, K. Stefanov and S. Popov, Russ. J. Bioorg. Chem., 2004, 30, 161–167 CrossRef CAS.
- R. Kimura, T. Rokkaku, S. Takeda, M. Senba and N. Mori, Mar. Drugs, 2013, 11, 4267–4278 CrossRef PubMed.
- M. Jaiswal, R. Dudhe and P. Sharma, 3 Biotech, 2015, 5, 123–127 CrossRef PubMed.
- S. Khan, S. Baboota, J. Ali, S. Khan, R. S. Narang and J. K. Narang, Int. J. Pharm. Invest., 2015, 5, 182 CrossRef CAS PubMed.
- P. Severino, T. Andreani, A. S. Macedo, J. F. Fangueiro, M. H. A. Santana, A. M. Silva and E. B. Souto, J. Drug Delivery, 2012, 2012, 750891 Search PubMed.
- F.-Q. Hu, S.-P. Jiang, Y.-Z. Du, H. Yuan, Y.-Q. Ye and S. Zeng, Colloids Surf., B, 2005, 45, 167–173 CrossRef CAS PubMed.
- V. Jenning, A. F. Thünemann and S. H. Gohla, Int. J. Pharm., 2000, 199, 167–177 CrossRef CAS PubMed.
- V. Jenning and S. H. Gohla, J. Microencapsulation, 2001, 18, 149–158 CrossRef CAS PubMed.
- E. Souto, S. Wissing, C. Barbosa and R. Müller, Int. J. Pharm., 2004, 278, 71–77 CrossRef CAS PubMed.
- Z.-L. Kong, J.-S. Chang and K. L. B. Chang, J. Nanopart. Res., 2013, 15, 1945 CrossRef.
- K. R. Walsh, Y. C. Zhang, Y. Vodovotz, S. J. Schwartz and M. L. Failla, J. Agric. Food Chem., 2003, 51, 4603–4609 CrossRef CAS PubMed.
- V. Zuco, R. Supino, S. C. Righetti, L. Cleris, E. Marchesi, C. Gambacorti-Passerini and F. Formelli, Cancer Lett., 2002, 175, 17–25 CrossRef CAS PubMed.
- J.-H. Hyun, S.-C. Kim, J.-I. Kang, M.-K. Kim, H.-J. Boo, J.-M. Kwon, Y.-S. Koh, J.-W. Hyun, D.-B. Park and E.-S. Yoo, Biol. Pharm. Bull., 2009, 32, 1760–1764 CAS.
- E. Rozengurt and C. C. Po, Nature, 1976, 261, 701 CrossRef CAS PubMed.
|
This journal is © The Royal Society of Chemistry 2018 |
Click here to see how this site uses Cookies. View our privacy policy here.