DOI:
10.1039/C8RA04035D
(Paper)
RSC Adv., 2018,
8, 21754-21758
Expanding the synthesizable multisubstituted benzo[b]thiophenes via 6,7-thienobenzynes generated from o-silylaryl triflate-type precursors†
Received
11th May 2018
, Accepted 6th June 2018
First published on 13th June 2018
Abstract
Various 2,3-disubstituted 6,7-thienobenzynes have been efficiently generated from the corresponding o-silylaryl triflate-type precursors by activation with fluoride ions. The method has expanded the scope of synthesizable multisubstituted benzothiophenes, including those with various heteroatom substituents, and can be applied to the synthesis of EP4 antagonist analogs.
Introduction
Benzo[b]thiophene is one of the structural units frequently found in molecules applied in various research fields, including medicinal chemistry and materials science.1–3 Although multisubstituted benzothiophenes are promising compounds as pharmaceutical and organic material candidates, their synthetic approaches are limited.4 To improve this situation, we previously reported a facile method to prepare various tetrasubstituted benzothiophenes via thienobenzyne intermediates such as I (Fig. 1A).5 Thienobenzynes I were efficiently generated from o-iodoaryl triflate-type precursors by treatment with a silylmethyl Grignard reagent at −78 °C, rendering a diverse range of tetrasubstituted benzothiophenes easily available.6 We considered that the use of o-silylaryl triflate-type thienobenzyne precursors would further expand the scope of the synthesizable benzothiophenes (Fig. 1B). This is because generation of arynes from this type of precursor has been generally achieved under mild conditions using a basic activator such as the fluoride ion.7–9 Indeed, a wide range of aromatic compounds have become easily available via the transformation of arynes generated from o-silylaryl triflate-type precursors. Herein, we report the synthesis of o-silylaryl triflate-type 6,7-thienobenzyne precursors, the generation of aryne species from these precursors, and the application of the method to the synthesis of various benzothiophenes including potent analogs of a prostaglandin E receptor subtype 4 (EP4) antagonist.
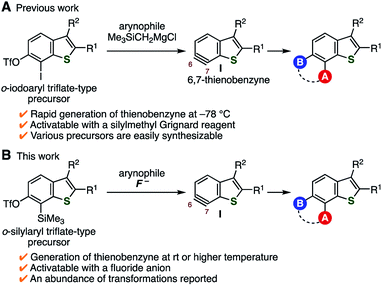 |
| Fig. 1 Transformations via thienobenzyne intermediates I. (A) Our previous work using o-iodoaryl triflate-type precursors. (B) This work using o-silylaryl triflate-type precursors. | |
Results and discussion
Synthesis of thienobenzyne precursors
Similar to our previous synthesis of o-iodoaryl triflate-type 6,7-thienobenzyne precursors, o-silylaryl triflate-type precursors 2a–d were successfully prepared from the corresponding 2,3-disubstituted 6-hydroxybenzo[b]thiophenes 1a–d (Schemes 1 and 2).5 Benzothiophenes 2a–c were prepared from 6-hydroxybenzothiophenes 1a–c according to the facile synthetic method for o-silylaryl triflates from phenols as reported by Garg and coworkers; carbamate formation using isopropyl isocyanate, regioselective C-silylation via ortho-lithiation, removal of the directing group, and triflylation (Scheme 1).10 Although preparation of benzothiophene 2d, bearing a chloro and an amide group, from phenol 1d by the same method was unsuccessful at the step of C-silylation via ortho-lithiation, the C-silylated product was obtained by an alternative method (Scheme 2).11 Thus, regioselective iodination of phenol 1d with a morpholine–iodine complex, followed by O-silylation and treatment with the turbo Grignard reagent to promote the iodine–magnesium exchange reaction and subsequent retro-Brook rearrangement via the anionic intermediate II, afforded o-silylphenol 4, leaving the chloro and amide groups untouched. Finally, triflylation of 4 afforded the desired 2d.12,13 Performing the retro-Brook rearrangement and subsequent O-triflylation in one-pot procedure12a afforded 2d in 13% yield.
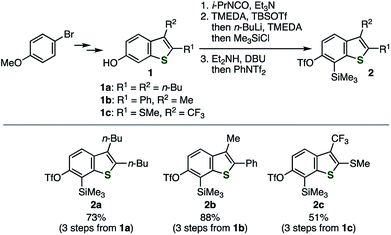 |
| Scheme 1 Synthesis of thienobenzyne precursors 2a–c. See the ESI† for details. | |
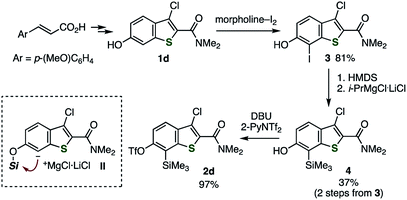 |
| Scheme 2 Synthesis of thienobenzyne precursor 2d. See the ESI† for details. | |
Optimization of the reaction conditions for generation of thienobenzynes
The efficient conditions for generating 6,7-thienobenzyne were screened for the reaction between precursor 2a and azide 5a in tetrahydrofuran (THF) at room temperature, which revealed that various fluoride sources or cesium carbonate with 18-crown-6 were effective as an activator (Table 1). For example, the activation of 2a with potassium fluoride in the presence of 18-crown-6 afforded the desired cycloadduct 6a with a small amount of regioisomer 6a′ (entry 1). The regioselectivity was slightly lower than that observed in the reaction using o-iodoaryl triflate-type 6,7-thienobenzyne precursor probably because the reaction triggered by silicate formation was conducted at a higher temperature. Tetra(n-butyl)ammonium difluoro(triphenyl)silicate and tetra(n-butyl)ammonium fluoride also served as good activators without any additives (entries 2 and 3). While using potassium fluoride alone was ineffective (entry 4), 2a was efficiently activated with cesium fluoride, resulting in the highest combined yield of cycloadducts 6a and 6a′ (entry 5). Considering that the generation of benzyne from o-(trimethylsilyl)phenyl triflate with cesium fluoride in THF was reported as inefficient,9a this result suggests that thienobenzyne precursor 2a is more easily activatable than the simple o-silylphenyl triflate. Decreasing the amount of azide 5a to 2.0 equiv. slightly lowered the yield of 6a/6a′ (entry 6). In addition, 6,7-thienobenzyne was also generated efficiently under fluoride-free conditions using cesium carbonate and 18-crown-6 (entry 7).9a
Table 1 Optimization of the reaction conditions
Synthesis of various multisubstituted benzothiophenes via thienobenzynes
Under the optimal conditions, various arynophiles reacted efficiently with thienobenzyne generated from 2a to afford multisubstituted benzothiophenes in high yields (Fig. 2). These include cycloadducts 7, 8, 9/9′, and 10 obtained from the reactions with 2,5-dimethylfuran, N-phenylpyrrole, N-(tert-butyl)-α-phenylnitrone, and 1,1-dimethoxyethylene, respectively. The nucleophilic addition of morpholine to the 6,7-thienobenzyne also took place, affording 6-morpholinobenzothiophene 11 as the major product. The regioselectivity observed using unsymmetrical arynophiles and the nucleophile showed similar trends to their reactions with the same thienobenzyne species generated from the o-iodoaryl triflate-type precursor.5
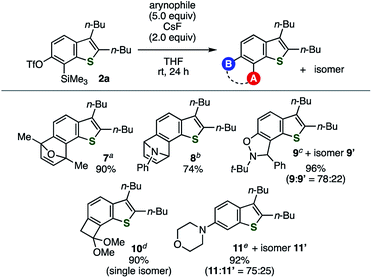 |
| Fig. 2 Reactions of thienobenzyne generated from 2a with various arynophiles. (a) Reaction with 2,5-dimethylfuran. (b) Reaction with N-phenylpyrrole. (c) Reaction with N-(tert-butyl)-α-phenylnitrone. (d) Reaction with 1,1-dimethoxyethylene. (e) Reaction with morpholine. | |
An abundance of utilizable transformations is a great advantage of using o-silylaryl triflates as aryne precursors over the other types. Indeed, the utility of o-silylaryl triflate-type 6,7-thienobenzyne precursor was demonstrated through several unique transformations that we recently developed (Fig. 3).14 For example, the Michaelis–Arbuzov-type reaction of the thienobenzyne generated from 2a with alkoxyphosphine 12 proceeded smoothly, affording a high yield of arylphosphonic diamide 13 as the sole product (Fig. 3A).14a Furthermore, difunctionalizations of the thienobenzyne intermediate with sulfilimine 14,14b sulfoximine 16,14c and sulfoxide 1814d resulted in the selective formation of thioaminated or oxythiolated benzothiophenes 15/15′, 17, and 19, respectively, which are difficult to prepare by conventional methods (Fig. 3B). The yields of thioaminated products 15/15′ and 17 were improved under modified conditions wherein the reactions were carried out at a higher temperature in 1,4-dioxane.
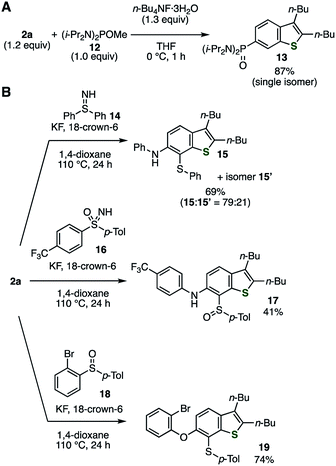 |
| Fig. 3 Transformations via thienobenzyne generated from 2a, involving C–P, C–S, C–N, and C–O bond formations. (A) Reaction with alkoxyphosphine 12. (B) Reactions with sulfilimine 14, sulfoximine 16, and sulfoxide 18. See the ESI† for details. | |
Various 2,3-disubstituted 6,7-thienobenzynes were also generated from precursors 2b–d (Fig. 4). The reactions of these thienobenzynes with azide 5a afforded triazole-fused 3-methyl-2-phenyl-, 2-methylsulfanyl-3-trifluoromethyl-, and 3-chloro-2-(dimethylamino)carbonylbenzothiophene derivatives 6b/6b′, 6c, and 6d/6d′, respectively, in a regioselective manner. Cycloadduct 6c was obtained as a single isomer along with complex mixtures of side-products probably due to the effect of the electron-withdrawing trifluoromethyl group. A similar trend was observed in our previous study,5 wherein 6c was obtained without formation of the regioisomer using o-iodoaryl triflate-type aryne precursor activated with a silylmethyl Grignard reagent.
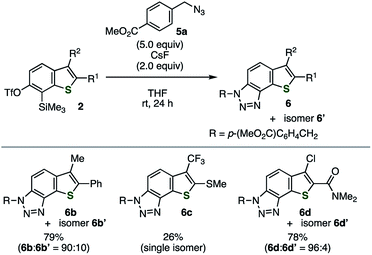 |
| Fig. 4 Cycloadditions of various thienobenzynes generated from precursors 2b–d with azide 5a. | |
Synthesis of the analogs of an EP4 antagonist
The utility of this method was demonstrated in the facile diversification of the benzo-moiety of the EP4 antagonist 20a developed by Li and coworkers (Scheme 3).15 The analogs 20b–d with methyltriazole-fused, benzo-fused, or morpholino-substituted benzothiophene structure, respectively, were easily prepared via the reactions of the thienobenzyne intermediate generated from 2d with (trimethylsilyl)methyl azide, furan, and morpholine, affording adducts 21a–c as the major products. According to the modified method reported previously for the derivatization of 21a to 20b,5 EP4 antagonist analogs 20c and 20d were prepared by the Suzuki–Miyaura cross-coupling, the Mitsunobu-type C–N bond formation followed by treatment with hydrazine, and amidation. Evaluations of the EP4 receptor binding affinities showed that benzo-fused analog 20c (Ki = 0.18 μM) is a potent EP4 antagonist comparable to the original compound 20a (Ki = 0.25 μM), while methyltriazole-fused analog 20b (Ki = 0.47 μM) and morpholino-substituted analog 20d (Ki = 0.70 μM) are slightly weaker antagonists than 20a.16 This result suggests a possibility for developing more potent EP4 antagonists by further modification of the benzo-moiety of 20a.
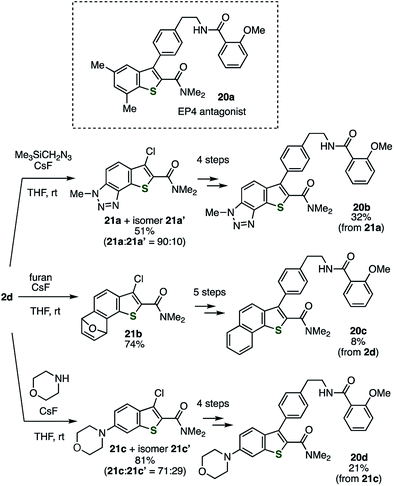 |
| Scheme 3 Synthesis of the analogs of EP4 antagonist 20a. See the ESI† for details. | |
Conclusions
This study showed that 7-silyl-6-triflyloxybenzo[b]thiophenes served as useful precursors of 6,7-thienobenzynes, thus expanding the range of synthesizable multisubstituted benzothiophenes. The utility of the method was demonstrated for the synthesis of various heteroatom-substituted benzothiophenes and the facile structural diversification of an EP4 antagonist that resulted in identification of a potent analog.
Conflicts of interest
There are no conflicts to declare.
Acknowledgements
This work was supported by AMED under Grant Numbers JP18am0101098 (Platform Project for Supporting Drug Discovery and Life Science Research (BINDS)) and JP18gm0910007 (CREST; T. Kobayashi and T. H); JSPS KAKENHI Grant Numbers 15H03118 and 18H02104 (B; T. H.), 16H01133 and 18H04386 (Middle Molecular Strategy; T. H.), and 26350971 (C; S. Y.); Suntory Foundation for Life Sciences (S. Y.); Naito Foundation (S. Y.); and the Cooperative Research Project of Research Center for Biomedical Engineering.
Notes and references
-
(a) K. Koike, Z. Jia, T. Nikaido, Y. Liu, Y. Zhao and D. Guo, Org. Lett., 1999, 1, 197–198 CrossRef;
(b) R. S. Keri, K. Chand, S. Budagumpi, S. B. Somappa, S. A. Patil and B. M. Nagaraja, Eur. J. Med. Chem., 2017, 138, 1002–1033 CrossRef PubMed.
- For our study on bioactive benzo[b]thiophenes, see: S. Shimizu, T. Hosoya, M. Murohashi and S. Yoshida, WO 2013/118842, 2013.
-
(a) M. Irie, Chem. Rev., 2000, 100, 1685–1716 CrossRef PubMed;
(b) T. Yamamoto and K. Takimiya, J. Am. Chem. Soc., 2007, 129, 2224–2225 CrossRef PubMed.
- For selected reports on the synthesis of benzo[b]thiophenes, see:
(a) B. L. Flynn, P. Verdier-Pinard and E. Hamel, Org. Lett., 2001, 3, 651–654 CrossRef PubMed;
(b) I. Nakamura, T. Sato and Y. Yamamoto, Angew. Chem., Int. Ed., 2006, 45, 4473–4475 CrossRef PubMed;
(c) S. Yoshida, H. Yorimitsu and K. Oshima, Org. Lett., 2007, 9, 5573–5576 CrossRef PubMed;
(d) C. S. Bryan, J. A. Braunger and M. Lautens, Angew. Chem., Int. Ed., 2009, 48, 7064–7068 CrossRef PubMed;
(e) L.-L. Sun, C.-L. Deng, R.-Y. Tang and X.-G. Zhang, J. Org. Chem., 2011, 76, 7546–7550 CrossRef PubMed;
(f) T. Kunz and P. Knochel, Angew. Chem., Int. Ed., 2012, 51, 1958–1961 CrossRef PubMed;
(g) T. Mori, T. Nishimura, T. Yamamoto, I. Doi, E. Miyazaki, I. Osaka and K. Takimiya, J. Am. Chem. Soc., 2013, 135, 13900–13913 CrossRef PubMed;
(h) B. Wu and N. Yoshikai, Angew. Chem., Int. Ed., 2013, 52, 10496–10499 CrossRef PubMed.
- T. Morita, S. Yoshida, M. Kondo, T. Matsushita and T. Hosoya, Chem. Lett., 2017, 46, 81–84 CrossRef.
- For our studies on generation of various arynes from o-iodoaryl triflates with a silylmethyl Grignard reagent, see:
(a) S. Yoshida, T. Nonaka, T. Morita and T. Hosoya, Org. Biomol. Chem., 2014, 12, 7489–7493 RSC;
(b) S. Yoshida, K. Uchida, K. Igawa, K. Tomooka and T. Hosoya, Chem. Commun., 2014, 50, 15059–15062 RSC;
(c) S. Yoshida, K. Uchida and T. Hosoya, Chem. Lett., 2015, 44, 691–693 CrossRef;
(d) S. Yoshida, T. Morita and T. Hosoya, Chem. Lett., 2016, 45, 726–728 CrossRef;
(e) K. Uchida, S. Yoshida and T. Hosoya, Synthesis, 2016, 48, 4099–4109 CrossRef;
(f) S. Yoshida, T. Yano, Y. Nishiyama, Y. Misawa, M. Kondo, T. Matsushita, K. Igawa, K. Tomooka and T. Hosoya, Chem. Commun., 2016, 52, 11199–11202 RSC;
(g) S. Yoshida, Y. Nakamura, K. Uchida, Y. Hazama and T. Hosoya, Org. Lett., 2016, 18, 6212–6215 CrossRef PubMed;
(h) T. Morita, Y. Nishiyama, S. Yoshida and T. Hosoya, Chem. Lett., 2017, 46, 118–121 CrossRef;
(i) S. Yoshida, A. Nagai, K. Uchida and T. Hosoya, Chem. Lett., 2017, 46, 733–736 CrossRef;
(j) S. Yoshida, K. Shimizu, K. Uchida, Y. Hazama, K. Igawa, K. Tomooka and T. Hosoya, Chem.–Eur. J., 2017, 23, 15332–15335 CrossRef PubMed.
- Y. Himeshima, T. Sonoda and H. Kobayashi, Chem. Lett., 1983, 12, 1211–1214 CrossRef.
- For selected recent reviews on arynes, see:
(a) C. M. Gampe and E. M. Carreira, Angew. Chem., Int. Ed., 2012, 51, 3766–3778 CrossRef PubMed;
(b) P. M. Tadross and B. M. Stoltz, Chem. Rev., 2012, 112, 3550–3577 CrossRef PubMed;
(c) H. Yoshida and K. Takaki, Heterocycles, 2012, 85, 1333–1349 CrossRef;
(d) H. Miyabe, Molecules, 2015, 20, 12558–12575 CrossRef PubMed;
(e) S. Yoshida and T. Hosoya, Chem. Lett., 2015, 44, 1450–1460 CrossRef;
(f) J.-A. García-López and M. F. Greaney, Chem. Soc. Rev., 2016, 45, 6766–6798 RSC;
(g) J. Shi, Y. Li and Y. Li, Chem. Soc. Rev., 2017, 46, 1707–1719 RSC;
(h) T. Roy and A. T. Biju, Chem. Commun., 2018, 54, 2580–2594 RSC.
- For selected recent reports on arynes generated from o-silylaryl triflates, see:
(a) S. Yoshida, Y. Hazama, Y. Sumida, T. Yano and T. Hosoya, Molecules, 2015, 20, 10131–10140 CrossRef PubMed;
(b) S. S. Bhojgude, D. R. Baviskar, R. G. Gonnade and A. T. Biju, Org. Lett., 2015, 17, 6270–6273 CrossRef PubMed;
(c) J. Shi, D. Qiu, J. Wang, H. Xu and Y. Li, J. Am. Chem. Soc., 2015, 137, 5670–5673 CrossRef PubMed;
(d) M. Pawliczek, L. K. B. Garve and D. B. Werz, Org. Lett., 2015, 17, 1716–1719 CrossRef PubMed;
(e) S. Yoshida, K. Shimomori, T. Nonaka and T. Hosoya, Chem. Lett., 2015, 44, 1324–1326 CrossRef;
(f) E. Demory, K. Devaraj, A. Orthaber, P. J. Gates and L. T. Pilarski, Angew. Chem., Int. Ed., 2015, 54, 11765–11769 CrossRef PubMed;
(g) C. M. Holden, S. M. A. Sohel and M. F. Greaney, Angew. Chem., Int. Ed., 2016, 55, 2450–2453 CrossRef PubMed;
(h) E. Yoshioka, M. Nishimura, T. Nakazawa, S. Kohtani and H. Miyabe, J. Org. Chem., 2015, 80, 8464–8469 CrossRef PubMed;
(i) T. Ikawa, S. Masuda, A. Takagi and S. Akai, Chem. Sci., 2016, 7, 5206–5211 RSC;
(j) Y. Li, D. Qiu, R. Gu, J. Wang, J. Shi and Y. Li, J. Am. Chem. Soc., 2016, 138, 10814–10817 CrossRef PubMed;
(k) Y. Li, C. Mück-Lichtenfeld and A. Studer, Angew. Chem., Int. Ed., 2016, 55, 14435–14438 CrossRef PubMed;
(l) X. Li, Y. Sun, X. Huang, L. Zhang, L. Kong and B. Peng, Org. Lett., 2017, 19, 838–841 CrossRef PubMed;
(m) T. Kitamura, K. Gondo and J. Oyamada, J. Am. Chem. Soc., 2017, 139, 8416–8419 CrossRef PubMed;
(n) J. B. Lin, T. K. Shah, A. E. Goetz, N. K. Garg and K. N. Houk, J. Am. Chem. Soc., 2017, 139, 10447–10455 CrossRef PubMed;
(o) H. Xu, J. He, J. Shi, L. Tan, D. Qiu, X. Luo and Y. Li, J. Am. Chem. Soc., 2018, 140, 3555–3559 CrossRef PubMed;
(p) P. Garg and A. Singh, Org. Lett., 2018, 20, 1320–1323 CrossRef PubMed;
(q) V. G. Pandya and S. B. Mhaske, Org. Lett., 2018, 20, 1483–1486 CrossRef PubMed;
(r) Y. Sumii, Y. Sugita, E. Tokunaga and N. Shibata, ChemistryOpen, 2018, 7, 204–211 CrossRef PubMed;
(s) P. Gouthami, L. N. Chavan, R. Chegondi and S. Chandrasekhar, J. Org. Chem., 2018, 83, 3325–3332 CrossRef PubMed.
- S. M. Bronner and N. K. Garg, J. Org. Chem., 2009, 74, 8842–8843 CrossRef PubMed.
- A complex mixture of products was obtained.
- For selected reports on the synthesis of o-silylaryl triflates via the retro-Brook rearrangement, see:
(a) D. Peña, A. Cobas, D. Pérez and E. Guitián, Synthesis, 2002, 1454–1458 Search PubMed;
(b) D. J. Atkinson, J. Sperry and M. A. Brimble, Synthesis, 2010, 911–913 Search PubMed;
(c) B. Michel and M. F. Greaney, Org. Lett., 2014, 16, 2684–2687 CrossRef PubMed.
- For a review of the turbo Grignard reagent, see: R. L.-Y. Bao, R. Zhaoa and L. Shi, Chem. Commun., 2015, 51, 6884–6900 RSC.
-
(a) S. Yoshida and T. Hosoya, Chem. Lett., 2013, 42, 583–585 CrossRef;
(b) S. Yoshida, T. Yano, Y. Misawa, Y. Sugimura, K. Igawa, S. Shimizu, K. Tomooka and T. Hosoya, J. Am. Chem. Soc., 2015, 137, 14071–14074 CrossRef PubMed;
(c) S. Yoshida, H. Nakajima, K. Uchida, T. Yano, M. Kondo, T. Matsushita and T. Hosoya, Chem. Lett., 2017, 46, 77–80 CrossRef;
(d) T. Matsuzawa, K. Uchida, S. Yoshida and T. Hosoya, Org. Lett., 2017, 19, 5521–5524 CrossRef PubMed.
- L. Li, M.-C. Mathieu, D. Denis, A. G. Therien and Z. Wang, Bioorg. Med. Chem. Lett., 2011, 21, 734–737 CrossRef PubMed.
- See the ESI† for details.
Footnote |
† Electronic supplementary information (ESI) available: Experimental procedures, characterization for new compounds including NMR spectra. See DOI: 10.1039/c8ra04035d |
|
This journal is © The Royal Society of Chemistry 2018 |
Click here to see how this site uses Cookies. View our privacy policy here.