DOI:
10.1039/C8SC02459F
(Edge Article)
Chem. Sci., 2018,
9, 7700-7704
Addition of arylstannanes to alkynes giving ortho-alkenylarylstannanes catalysed cooperatively by a rhodium complex and zinc chloride†
Received
5th June 2018
, Accepted 10th August 2018
First published on 17th August 2018
Abstract
The reaction of arylstannanes ArSnR3 with unfunctionalised alkynes was found to proceed in the presence of a rhodium catalyst and a catalytic amount of zinc chloride to give ortho-alkenylarylstannanes with high selectivity in high yields. The catalytic cycle is very unique, consisting of three transmetalation steps, from Sn to Rh, Rh to Zn, and Zn to Sn, in addition to arylrhodation of alkyne followed by 1,4-migration of Rh from 2-arylalkenyl carbon to ortho-alkenylaryl carbon.
Introduction
Organostannanes are organometallic reagents widely used for organic synthesis.1 They are easy to handle because of their inertness towards oxygen and moisture, while the organostannanes are reactive with various electrophiles in the presence of appropriate activators. A typical example is the palladium-catalysed cross-coupling reaction (Stille coupling).2 The organostannanes have been most commonly prepared by the reaction of highly reactive organometals (RLi, RMgX) with tin electrophiles, and considerable attention has been paid to the development of their new synthetic methods. Palladium- or nickel-catalysed stannylation of organic electrophiles with distannanes is one of the examples.3 Here we report our findings that migratory arylstannylation of unfunctionalised alkynes is catalysed by Rh complexes in the presence of a catalytic amount of ZnCl2 to give high yields of ortho-alkenylarylstannanes with high selectivity. The reaction is proposed to proceed through 1,4-migration of Rh from alkenyl carbon to aryl carbon4–6 (Scheme 1a). Carbostannylation of alkynes with allyl- and alkynylstannanes has been reported to be catalysed by transition metals such as Pd and Ni,7,8 but the addition of arylstannanes has not been reported, to the best of our knowledge (Scheme 1b). This type of arylmetalation reaction that is accompanied by the 1,4-migration of the metal has been reported by Yoshikai for arylzincation catalysed by a cobalt complex (Scheme 1c).9
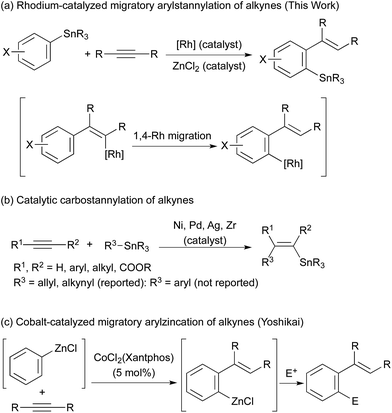 |
| Scheme 1 Arylstannylation of alkynes and 1,4-migration. | |
Results and discussion
The results obtained for the reaction of 4-octyne (2a) with PhSnBu3 (1a, 2.0 equiv. to 2a) under various conditions are summarised in Table 1. The migratory arylstannylation was found to proceed in the presence of ZnCl2 (1.0 equiv. to 2a) and a rhodium catalyst generated from [RhCl(coe)2]2 (5.0 mol% of Rh) and binap10 (5.5 mol%) in dioxane at 130 °C for 16 h to give 85% yield of 4-(2-stannylphenyl)-4-octene (3aa) with perfect E geometry11 (entry 1). The direct arylstannylation product 4aa was not formed in a detectable amount under these reaction conditions. The yield of 3aa was dependent on the amount of ZnCl2 to some extent. With 2.0 equiv. of ZnCl2, the yield was slightly increased (89%) (entry 2). The yields were lower with less amount of ZnCl2, being 73%, 71% and 27%, with 0.50, 0.25 and 0.10 equiv. of ZnCl2, respectively (entries 3–5). It is noted that ZnCl2 is working as a catalyst though a substoichiometric amount is necessary for a high yield of 3aa. The presence of ZnCl2 is essential for the present arylstannylation, 3aa being not formed at all in its absence (entry 6). At lower reaction temperature (100 °C), the yield of 3aa was lower by 10% (entry 7). Other zinc halides, ZnBr2 and ZnI2 were less catalytically active than ZnCl2 (entries 8 and 9). It is difficult to find a substitute of ZnCl2 from other metal salts. CuCl12 gave a trace amount of 3aa (entry 10). The binap ligand on Rh can be replaced by segphos or biphep, both of which are analogous to binap in that their backbones connecting two phosphino groups are biaryls. The yields with segphos13 and biphep were slightly lower (73% and 71%, respectively) than that with binap for the reaction of PhSnBu3 (1a) (entries 11 and 12), but for the reactions of some other arylstannanes, the yields of the arylstannylation products are higher with segphos ligand (see Table 2). The catalytic activity of other phosphine–Rh complexes were much lower. While dppf ligand gave a low yield (16%) of 3aa (entry 13), the reaction did not take place with dppe, dppp, xantphos or PPh3 (entries 14–17). Rh complex with cyclooctadiene (cod) ligand or Ir/binap complex did not catalyse the reaction either (entries 18 and 19). The cobalt complex, CoCl2(xantphos), which has been reported to be an effective catalyst for the migratory arylzincation,9 is not a catalyst of choice for the present arylstannylation, yielding only 7% of 3aa (entry 20).
Table 1 Rhodium-catalysed phenylstannylation of 4-octyne (2a) with PhSnBu3 (1a)a
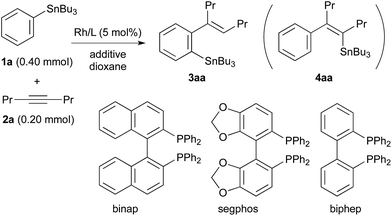
|
Entry |
Rh catalystb (5 mol%) |
Additive (equiv. to 2a) |
Yieldc (%) of 3aa |
Reaction conditions: 4-octyne (2a) (0.20 mmol), PhSnBu3 (1a) (0.40 mmol) and ZnCl2 (0.20 mmol) in dioxane (1.0 mL) at 130 °C (bath temp.) for 16 h.
Rh catalyst (5 mol% of Rh) was generated in situ from [RhCl(coe)2]2 (10 μmol of Rh) and bisphosphine (11 μmol).
Isolated yield.
At 100 °C.
RhCl(PPh3)3 (10 μmol).
[RhCl(cod)]2 (10 μmol of Rh).
[IrCl(coe)2]2 (10 μmol of Ir) + binap (11 μmol).
CoCl2(xantphos) (10 μmol).
|
1 |
Rh/binap |
ZnCl2 (1.0) |
85 |
2 |
Rh/binap |
ZnCl2 (2.0) |
89 |
3 |
Rh/binap |
ZnCl2 (0.50) |
73 |
4 |
Rh/binap |
ZnCl2 (0.25) |
71 |
5 |
Rh/binap |
ZnCl2 (0.10) |
27 |
6 |
Rh/binap |
— |
0 |
7d |
Rh/binap |
ZnCl2 (1.0) |
75 |
8 |
Rh/binap |
ZnBr2 (1.0) |
43 |
9 |
Rh/binap |
ZnI2 (1.0) |
18 |
10 |
Rh/binap |
CuCl (1.0) |
<3 |
11 |
Rh/segphos |
ZnCl2 (1.0) |
73 |
12 |
Rh/biphep |
ZnCl2 (1.0) |
71 |
13 |
Rh/dppf |
ZnCl2 (1.0) |
16 |
14 |
Rh/dppe |
ZnCl2 (1.0) |
0 |
15 |
Rh/dppp |
ZnCl2 (1.0) |
0 |
16 |
Rh/xantphos |
ZnCl2 (1.0) |
0 |
17 |
Rh/PPh3e |
ZnCl2 (1.0) |
<3 |
18 |
Rh/codf |
ZnCl2 (1.0) |
0 |
19 |
Ir/binapg |
ZnCl2 (1.0) |
0 |
20 |
Co/xantphosh |
ZnCl2 (1.0) |
7 |
Table 2 Rhodium-catalysed arylstannylation of 4-octyne (2a) with ArSnR31a
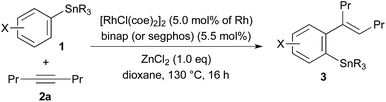
|
Entry |
ArSnR31 |
L on Rhb |
Yieldc (%) of 3 |
Reaction conditions: 4-octyne (2a) (0.20 mmol), ArSnR31 (0.40 mmol), ZnCl2 (0.20 mmol) and Rh catalyst (5 mol% of Rh) in dioxane (1.0 mL) at 130 °C (bath temp.) for 16 h.
Rh catalyst (5 mol% of Rh) was generated in situ from [RhCl(coe)2]2 (10 μmol of Rh) and binap or segphos (11 μmol).
Isolated yield.
In THF at 90 °C.
Regioselective 1,4-shift giving the products 3 shown below.
|
1 |
PhSnBu3 (1a) |
Binap |
85 (3aa) |
2 |
PhSnMe3 (1b) |
Binapd |
77 (3ba) |
3 |
PhSnPr3 (1c) |
Binap |
89 (3ca) |
4 |
PhSnOct3 (1d) |
Binap |
78 (3da) |
5 |
4-MeC6H4SnBu3 (1e) |
Binap |
87 (3ea) |
6 |
4-PhC6H4SnBu3 (1f) |
Binap |
91 (3fa) |
7 |
4-Me3SiC6H4SnBu3 (1g) |
Binap |
83 (3ga) |
8 |
4-CF3OC6H4SnBu3 (1h) |
Binap |
83 (3ha) |
9 |
4-MeOC6H4SnBu3 (1i) |
Binapd |
65 (3ia) |
10 |
4-FC6H4SnBu3 (1j) |
Binap |
77 (3ja) |
11 |
4-ClC6H4SnBu3 (1k) |
Binap |
73 (3ka) |
12 |
4-ClC6H4SnBu3 (1k) |
Segphos |
84 (3ka) |
13 |
4-BrC6H4SnBu3 (1l) |
Binap |
47 (3la) |
14 |
4-BrC6H4SnBu3 (1l) |
Segphos |
78 (3la) |
15 |
4-NCC6H4SnBu3 (1m) |
Binap |
51 (3ma) |
16 |
4-NCC6H4SnBu3 (1m) |
Segphos |
67 (3ma) |
17 |
4-MeOOCC6H4SnBu3 (1n) |
Binap |
61 (3na) |
18 |
4-MeOOCC6H4SnBu3 (1n) |
Segphos |
82 (3na) |
19 |
4-CF3C6H4SnBu3 (1o) |
Segphos |
69 (3oa) |
20 |
3-MeC6H4SnBu3 (1p) |
Binap |
77 (3pa)e |
21 |
3-Me3SiC6H4SnBu3 (1q) |
Binap |
84 (3qa)e |
22 |
3-CF3C6H4SnBu3 (1r) |
Binap |
67 (3ra)e |
23 |
2-NaphthylSnBu3 (1s) |
Binap |
83 (3sa)e |
24 |
2-MeC6H4SnBu3 (1t) |
Binap |
<3 (3ta) |
The reaction conditions optimised for the phenylstannylation with PhSnBu3 (1a), that is, with Rh/binap (5 mol%) and ZnCl2 (1.0 equiv.) at 130 °C (entry 1 in Table 1), were successfully applied to the reaction of several other aryltin reagents ArSnR3 with 4-octyne (2a) (Table 2). The phenyltin reagents PhSnR3, where R is methyl (1b), propyl (1c) and octyl (1d), all gave the corresponding phenylstannylation products 3ba–3da in high yields (entries 2–4). The yields are generally high for para-substituted aryltin reagents, those substituted with Me, Ph, Me3Si and CF3O groups giving the corresponding products in 83–91% yields (entries 5–8). The lower yield (65%) for MeO-substituted one 1i is mainly due to the instability of the product 3ia under the reaction conditions (entry 9). For the reaction of aryltin reagents substituted with electron-withdrawing groups at para-position, Cl (1k), Br (1l), CN (1m) and COOMe (1n), the Rh/binap catalyst was not very effective resulting in lower yields of the corresponding arylstannylation products. The use of Rh/segphos as a catalyst instead of Rh/binap improved the reaction for these aryltin reagents (entries 11–18). A typical example is the reaction of 4-BrC6H4SnBu3, where the yields of the product 3la are 47% and 78% with binap and segphos ligands, respectively (entries 13 and 14). In the arylstannylation with meta-substituted aryltin reagents, perfect regioselectivity at the 1,4-migration was observed. Thus, the reaction of those substituted with Me (1p), Me3Si (1q) and CF3 (1r) gave the corresponding 2,4-disubstituted aryltins 3pa–3ra, which are the less hindered isomers, exclusively (entries 20–22). The regioselectivity was also high for the reaction of 2-naphthyltin 1s, where the 1,4-migration took place to the less hindered 3-position selectively (entry 23). Unfortunately, the migratory arylstannylation did not take place for ortho-substituted tin reagent 1t under the present conditions (entry 24).
The results obtained for the reaction of PhSnBu3 (1a) with several unfunctionalised alkynes substituted with alkyl and aryl groups are summarised in Table 3. The migratory arylstannylation proceeded well for longer-chained dialkylacetylenes, 5-decyne (2b) and 8-hexadecyne (2c), to give high yields of the corresponding products, 3ab and 3ac, respectively. In the reaction of unsymmetrically substituted dialkylacetylene 2d, the regioselectivity at the addition to alkyne was low, resulting in the formation of a mixture of 3ad and its regioisomer in a ratio of 1.2/1.0. Diarylacetylenes also underwent the migratory arylstannylation, although the yields are generally lower than those for dialkylacetylenes.14 The reaction of alkyl(aryl)alkynes 2h–2l, proceeded with high regioselectivity for the bond formation between phenyl group of phenyltin 1a and alkyl-substituted alkyne carbon. This selectivity is as expected from the reported regiochemistry at carbometalation of alkyl(aryl)alkynes.15
Table 3 Rhodium-catalysed arylstannylation of alkynes 2 with PhSnBu3 (1a)a
Reaction conditions: alkyne 2 (0.20 mmol), PhSnBu31a (0.40 mmol), ZnCl2 (0.20 mmol) and Rh catalyst (5 mol% of Rh), generated in situ from [RhCl(coe)2]2 (10 μmol of Rh) and binap (11 μmol), in dioxane (1.0 mL) at 130 °C (bath temp.) for 16 h. The structures of main regioisomers are shown for the products from unsymmetrically substituted alkynes.
|
|
The reaction pathway of the present migratory arylstannylation of alkynes, which is catalysed cooperatively by Rh complex and ZnCl2, is proposed as shown in Scheme 2. Thus, the transmetalation of phenyl group from Sn to Rh takes place at the reaction of PhSnR31 with a Cl–Rh species A to generate Ph–Rh intermediate B and ClSnR3,16 the latter being to be involved at the final step leading to the stannylation product 3. The syn-addition of Ph–Rh B to alkyne 2 generates 2-arylalkenyl–Rh C and 1,4-migration of Rh from alkenyl to phenyl4,5 gives ortho-alkenylphenyl–Rh intermediate D, which has been reported to be thermodynamically more stable than C.5e Transmetalation between the ortho-alkenylpheny–Rh D and ZnCl2 takes place to give arylzinc chloride E and the Cl–Rh species A. Finally, the reaction of arylzinc chloride E with ClSnR3,17 which was formed at the transmetalation between PhSnR31 and Cl–Rh A, leads to ortho-alkenylphenystannane 3, with regenerating ZnCl2. Direct transmetalation between the Ar–Rh intermediate D and PhSnR31 giving Ph–Rh B and the product 3 is less likely because a catalytic amount of ZnCl2 is essential for the present arylstannylation to take place (see entries 1–6 in Table 1).
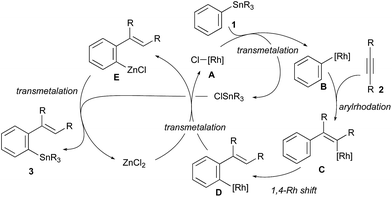 |
| Scheme 2 A catalytic cycle proposed for migratory arylstannylation of alkynes catalysed by Rh complex and ZnCl2. | |
The reactions shown in Scheme 3 gave us further information on the catalytic cycle. Stoichiometric reactions of Ph–Rh complex, RhPh(PPh3) (binap) (5),18 with ClSnBu3 (2.0 equiv.) in the presence of ZnCl2 (1.2 equiv.) in dioxane at 130 °C gave 93% yield of PhSnBu3 (1a), while the yield of 1a is very low (12%) in the absence of ZnCl2 under otherwise the same conditions (Scheme 3a). These reactions are related to the last transmetalation step producing 3 from Ar–Rh intermediate D in the catalytic cycle. The results show that the direct transmetalation between intermediate D and ClSnR3 is slow and that ZnCl2 greatly accelerates the transmetalation. The fast transmetalation in the presence of ZnCl2 is probably because of a lower energy caused by the double transmetalations from Rh to Zn and from Zn to Sn by way of arylzinc species E. Rhodium-catalysed 1,4-migration of Sn from alkenylstannane 4ae to arylstannane 3ae was observed in the presence of ZnCl2, albeit in a low yield (16%).19 The 1,4-migration of Sn did not take place in the absence of ZnCl2 (Scheme 3b). The catalytic cycle involving the 1,4-Rh shift from intermediates C to D is supported by these results. The deuterium-labeling study using C6D5SnBu3 (1a-d5) (Scheme 3c), where the deuterium is incorporated at olefinic carbon in 3aa-d5, further supports this catalytic cycle involving the 1,4-Rh shift. The present Rh/ZnCl2 cocatalyst system is also applicable to the addition of PhZnCl (6a) to 4-octyne (2a) generating ortho-alkenylphenylzinc species 7,20 the reaction of which with ClSnBu3 gave 78% yield of the tin compound 3aa (Scheme 3d).
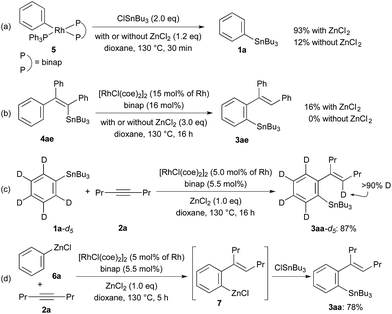 |
| Scheme 3 Reactions to support the catalytic cycle. | |
The synthetic utility of arylstannanes has been well established.1 According to the reported procedures,21–23 tributylstannyl group in 3aa was converted into deuterio (8), iodo (9) and fluoro (10) successfully (Scheme 4). The palladium-catalysed cross-coupling with an aryl iodide2 and the rhodium-catalysed conjugate addition to 2-cyclohexenone16 gave high yields of the corresponding products, 11 and 12, respectively, as expected.
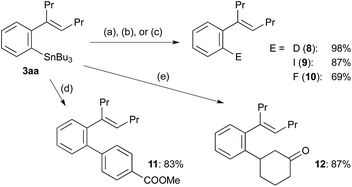 |
| Scheme 4 Transformation of ortho-alkenylarylstannane 3aa. (a) (CF3CO)2O, D2O. (b) I2, CH2Cl2. (c) Selectfluor, AgOTf, acetone. (d) 4-IC6H4COOMe, PdCl2(PPh3)2 (10 mol%), CuI, DMF. (e) 2-Cyclohexenone, [RhCl(cod)]2 (5 mol% Rh), KOH, dioxane/H2O. | |
Conclusions
To summarise, migratory arylstannylation was found to take place in the reaction of arylstannanes ArSnR3 with unfunctionalised alkynes in the presence of a bisphosphine–rhodium catalyst and a catalytic amount of zinc chloride to produce ortho-alkenylarylstannanes in high yields. A catalytic cycle involving three transmetalation steps is proposed. That is, transmetalation of aryl groups from Sn to Rh, Rh to Zn, and Zn to Sn.
Conflicts of interest
There are no conflicts to declare.
Acknowledgements
This work was supported by funding from Nanyang Technological University and the Singapore Ministry of Education (AcRF MOE 2016-T1-001-247 and 2017-T2-1-064).
Notes and references
-
(a)
M. Gielen, A. G. Davies, K. H. Pannell and E. Tiekink, Tin chemistry: fundamentals, frontiers, and applications, Wiley, 2008 Search PubMed;
(b)
A. G. Davies, in Organotin Chemistry, Wiley-VCH, Weinheim, 2nd edn, 2004, p. 140 CrossRef;
(c)
M. Pereyre, J.-P. Quintard and A. Rahm, Tin in Organic Synthesis, Butterworths & Co., 1987 Search PubMed.
-
(a) C. Cordovilla, C. Bartolomé, J. M. Martínez-Ilarduya and P. Espinet, ACS Catal., 2015, 5, 3040 CrossRef;
(b)
A. de Meijere, S. Bräse and M. Oestreich, Metal-Catalyzed Cross-Coupling Reactions and More, Wiley-VCH, Weinheim, 2014 CrossRef;
(c) M. M. Heravi, E. Hashemi and F. Azimian, Tetrahedron, 2014, 70, 7 CrossRef;
(d) B. Carsten, F. He, H. J. Son, T. Xu and L. Yu, Chem. Rev., 2011, 111, 1493 CrossRef PubMed;
(e)
V. Farina, V. Krishnamurthy and W. J. Scott, The Stille Reaction, Wiley, New York, 2004 Search PubMed;
(f) P. Espinet and A. M. Echavarren, Angew. Chem., Int. Ed., 2004, 43, 4704 Search PubMed.
-
(a) D. Azarian, S. S. Dua, C. Eaborn and D. R. M. Walton, J. Organomet. Chem., 1976, 117, C55 CrossRef;
(b) A. N. Kashin, I. G. Bumagina, N. A. Bumagin, V. N. Bakunin and I. P. Beletskaya, J. Org. Chem. USSR, 1981, 17, 789 Search PubMed;
(c) M. Kosugi, T. Ohya and T. Migita, Bull. Chem. Soc. Jpn., 1983, 56, 3855 CrossRef;
(d) W. D. Wulff, G. A. Peterson, W. E. Bauta, K.-S. Chan, K. L. Faron, S. R. Gilbertson, R. W. Kaesler, D. C. Yang and C. K. Murray, J. Org. Chem., 1986, 51, 277 CrossRef;
(e) E. B. Corcoran, A. B. Williams and R. N. Hanson, Org. Lett., 2012, 14, 4630 CrossRef PubMed;
(f) J. E. Pickett, A. Váradi, T. C. Palmer, S. G. Grinnell, J. M. Schrock, G. W. Pasternak, R. R. Karimov and S. Majumdar, Bioorg. Med. Chem. Lett., 2015, 25, 1761 CrossRef PubMed;
(g) Y. Gu and R. Martín, Angew. Chem., Int. Ed., 2017, 56, 3187 CrossRef PubMed;
(h) H. Yue, C. Zhu and M. Rueping, Org. Lett., 2018, 20, 385 CrossRef PubMed.
- For reviews dealing with 1,4-metal shift, see:
(a) M. F. Croisant, R. van Hoveln and J. M. Schomaker, Eur. J. Org. Chem., 2015, 5897 CrossRef;
(b) F. Shi and R. C. Larock, Top. Curr. Chem., 2010, 292, 123 CrossRef PubMed;
(c) T. Miura and M. Murakami, Chem. Commun., 2007, 217 RSC;
(d) S. Ma and Z. Gu, Angew. Chem., Int. Ed., 2005, 44, 7512 CrossRef PubMed.
- For 1,4-Rh shift from alkenyl to aryl, see:
(a) T. Hayashi, K. Inoue, N. Taniguchi and M. Ogasawara, J. Am. Chem. Soc., 2001, 123, 9918 CrossRef PubMed;
(b) T. Miura, T. Sasaki, H. Nakazawa and M. Murakami, J. Am. Chem. Soc., 2005, 127, 1390 CrossRef PubMed;
(c) R. Shintani, K. Takatsu and T. Hayashi, Angew. Chem., Int. Ed., 2007, 46, 3735 CrossRef PubMed;
(d) R. Shintani, S. Isobe, M. Takeda and T. Hayashi, Angew.
Chem., Int. Ed., 2010, 49, 3795 CrossRef PubMed;
(e) K. Sasaki, T. Nishimura, R. Shintani, E. A. B. Kantchev and T. Hayashi, Chem. Sci., 2012, 3, 1278 RSC;
(f) K. Sasaki and T. Hayashi, Tetrahedron: Asymmetry, 2012, 23, 373 CrossRef.
- For examples of other types of 1,4-Rh shift, see:
(a) K. Oguma, M. Miura, T. Satoh and M. Nomura, J. Am. Chem. Soc., 2000, 122, 10464 CrossRef;
(b) T. Matsuda, M. Shigeno and M. Murakami, J. Am. Chem. Soc., 2007, 129, 12086 CrossRef PubMed;
(c) J. Panteleev, F. Menard and M. Lautens, Adv. Synth. Catal., 2008, 350, 2893 CrossRef;
(d) T. Seiser, O. A. Roth and N. Cramer, Angew. Chem., Int. Ed., 2009, 48, 6320 CrossRef PubMed;
(e) M. Shigeno, T. Yamamoto and M. Murakami, Chem.–Eur. J., 2009, 15, 12929 CrossRef PubMed;
(f) T. Seiser and N. Cramer, Chem.–Eur. J., 2010, 16, 3383 CrossRef PubMed;
(g) T. Seiser and N. Cramer, Angew. Chem., Int. Ed., 2010, 49, 10163 CrossRef PubMed;
(h) T. Matsuda, Y. Suda and A. Takahashi, Chem. Commun., 2012, 48, 2988 RSC;
(i) H. Yu, C. Wang, Y. Yang and Z.-M. Dang, Chem.–Eur. J., 2014, 20, 3839 CrossRef PubMed;
(j) R. Shintani, R. Iino and K. Nozaki, J. Am. Chem. Soc., 2014, 136, 7849 CrossRef PubMed;
(k) H. B. Hepburn and H. W. Lam, Angew. Chem., Int. Ed., 2014, 53, 11605 CrossRef PubMed;
(l) T. Sawano, M. Hashizume, S. Nishimoto, K. Ou and T. Nishimura, Org. Lett., 2015, 17, 2630 CrossRef PubMed;
(m) J. Ming and T. Hayashi, Org. Lett., 2016, 18, 6452 CrossRef PubMed.
- For reviews on carbostannylation of C–C multiple bonds:
(a) H. Yoshida, Synthesis, 2016, 48, 2540 CrossRef;
(b)
E. Shirakawa, Carbostannylation of Carbon–Carbon Unsaturated Bonds, in Tin Chemistry: Fundamentals, Frontiers, and Applications, ed. M. Gielen, A. G. Davies, K. H. Pannell and E. R. Tiekink, John Wiley & Sons, Chichester, 2008, pp. 640–652 Search PubMed;
(c) E. Shirakawa and T. Hiyama, Bull. Chem. Soc. Jpn., 2002, 75, 1435 CrossRef;
(d) E. Shirakawa and T. Hiyama, J. Organomet. Chem., 2002, 653, 114 CrossRef.
- Examples of carbostannylation of alkynes:
(a) M. Shimizu, G. Jiang, M. Murai, Y. Takeda, Y. Nakao, T. Hiyama and E. Shirakawa, Chem. Lett., 2005, 34, 1700 CrossRef;
(b) E. Shirakawa, Y. Yamamoto, Y. Nakao, S. Oda, T. Tsuchimoto and T. Hiyama, Angew. Chem., Int. Ed., 2004, 43, 3448 CrossRef PubMed;
(c) E. Shirakawa, H. Yoshida, Y. Nakao and T. Hiyama, J. Am. Chem. Soc., 1999, 121, 4290 CrossRef.
-
(a) B.-H. Tan, J. Dong and N. Yoshikai, Angew. Chem., Int. Ed., 2012, 51, 9610 CrossRef PubMed ; see also: ;
(b) B.-H. Tan and N. Yoshikai, Org. Lett., 2014, 16, 3392 CrossRef PubMed;
(c) J. Wu and N. Yoshikai, Angew. Chem., Int. Ed., 2016, 55, 336 CrossRef PubMed;
(d) J. Yan and N. Yoshikai, Org. Chem. Front., 2017, 4, 1972 RSC.
- H. Takaya, K. Mashima, K. Koyano, M. Yagi, H. Kumobayashi, T. Taketomi, S. Akutagawa and R. Noyori, J. Org. Chem., 1986, 51, 629 CrossRef.
- The E geometry was confirmed by nOe studies of 3aa and 3na and X-ray crystal structure analysis of 3ag. See ESI†.
- CuBr has been used as a cocatalyst in Fe-catalysed arylmagnesiation of alkynes: E. Shirakawa, T. Yamagami, T. Kimura, S. Yamaguchi and T. Hayashi, J. Am. Chem. Soc., 2005, 127, 17164 CrossRef PubMed.
- T. Saito, T. Yokozawa, T. Ishizaki, T. Moroi, N. Sayo, T. Miura and H. Kumobayashi, Adv. Synth. Catal., 2001, 343, 264 CrossRef.
- The lower yields are due mainly to the formation of higher molecular weight compounds.
- For a pertinent review on catalytic carbometallation of alkynes: K. Murakami and H. Yorimitsu, Beilstein J. Org. Chem., 2013, 9, 278 CrossRef PubMed.
- The transmetalation from Sn to Rh has been reported for the conjugate addition of aryl- and alkenylstannanes to α,β-unsaturated carbonyl compounds:
(a) S. Oi, M. Moro, S. Ono and Y. Inoue, Chem. Lett., 1998, 83 CrossRef;
(b) S. Venkatraman, Y. Meng and C.-J. Li, Tetrahedron Lett., 2001, 42, 4459 CrossRef;
(c) S. Oi, M. Moro, H. Ito, Y. Honma, S. Miyano and Y. Inoue, Tetrahedron, 2002, 58, 91 CrossRef;
(d) S. J. Mahoney, A. M. Dumas and E. Fillion, Org. Lett., 2009, 11, 5346 CrossRef PubMed.
- The reaction of ClSnR3 with ArZnX giving ArSnR3 has been reported: as an early example, X. Zhu, B. E. Blough and F. I. Carroll, Tetrahedron Lett., 2000, 41, 9219 CrossRef.
- T. Hayashi, M. Takahashi, Y. Takaya and M. Ogasawara, J. Am. Chem. Soc., 2002, 124, 5052 CrossRef PubMed , see also, G. E. Rudebusch, L. N. Zakharov and S.-Y. Liu, Angew. Chem., Int. Ed., 2013, 52, 9316 CrossRef PubMed.
- The low yield is mainly because of low conversion of the alkenylstannane 4ae.
- The reaction of arylzinc reagents with unfunctionalized alkynes cocatalysed by a Rh complex and ZnCl2 will be reported in detail in a separate paper.
- D. E. Seitz, R. A. Milius and H. El-Wakil, Synth. Commun., 1981, 11, 281 CrossRef.
- D. E. Seitz, G. L. Tonnesen, S. Hellman, R. N. Hanson and S. J. Adelstein, J. Organomet. Chem., 1980, 186, C33 CrossRef.
- T. Furuya, A. E. Strom and T. Ritter, J. Am. Chem. Soc., 2009, 131, 1662 CrossRef PubMed.
Footnote |
† Electronic supplementary information (ESI) available: Experimental procedures, compound characterization data and X-ray crystallographic data of compound 3ag. CCDC 1841177. For ESI and crystallographic data in CIF or other electronic format see DOI: 10.1039/c8sc02459f |
|
This journal is © The Royal Society of Chemistry 2018 |
Click here to see how this site uses Cookies. View our privacy policy here.