DOI:
10.1039/C8FO02230E
(Communication)
Food Funct., 2019,
10, 26-32
Dietary (−)-epicatechin affects NF-κB activation and NADPH oxidases in the kidney cortex of high-fructose-fed rats
Received
13th November 2018
, Accepted 11th December 2018
First published on 3rd January 2019
Abstract
Inflammation involves the activation of redox-sensitive transcription factors, e.g., nuclear factor κB (NF-κB). Administration of (−)-epicatechin to high-fructose-fed rats prevented NF-κB activation and up-regulation of the NADPH oxidase 4 (NOX4) in the kidney cortex. These results add mechanistic insights into the action of (−)-epicatechin diminishing inflammatory responses.
Introduction
A chronic low-grade inflammatory state is associated with a wide range of chronic conditions such as metabolic syndrome, non-alcoholic fatty liver disease, type 2 diabetes mellitus and cardiovascular disease. The inflammatory process is initiated by the up-regulation of genes encoding for diverse mediators, such as cytokines and chemokines, through the activation of different transcription factors including nuclear factor κB (NF-κB).1
NF-κB can be activated by different additional and intracellular stimuli.2 NF-κB is a heterodimer composed of members of the Rel/NF-κB family of proteins (e.g., p50 and p65) and resides in the cytosol in an inactive form bound to the inhibitory protein κBα (IκBα). During NF-κB activation, IκBα kinases (IKKα/β) phosphorylate IκBα leading to its subsequent ubiquitination and degradation by the proteasome. The released NF-κB is susceptible to phosphorylation in p65 which allows the translocation to the nucleus, where it binds to κB sites in DNA and regulates the transcription of proinflammatory mediators such as interleukin 6, TNFα, and inducible nitric oxide synthase (iNOS).3 The role of toll-like receptors (TLR) in NF-κB activation is well characterized. Mammalian TLRs are sensors of pathogen-associated molecules such as bacterial lipopolysaccharides, lipopeptides and flagellins as well as of endogenous ligands such as diverse damage-associated molecules. TLR-4 recognizes lipopolysaccharides from Gram-negative bacteria and endogenous molecules such as heat shock protein 60, fibronectin and fibrinogen, leading to the activation of the NF-κB signalling pathway.2
The family of NADPH oxidases (NOX) consists of several superoxide anion- or H2O2-producing enzymes. In the rat kidney, NOX1, NOX2 and NOX4 are expressed in mesangial cells, vascular smooth muscle cells, endothelial cells, podocytes, tubular epithelial cells and interstitial fibroblasts. NOX2 consists of a membrane-bound catalytic subunit (gp91phox) and regulatory/activating cytosolic subunits, e.g., p47phox and p67phox.4 The isoform NOX1 has a membrane-bound catalytic subunit with the same name (NOX1) and specific cytosolic regulatory/activating subunits, e.g., NOX1 organizer (NOXO1). Electrons from NADPH are transferred to molecular oxygen, generating the superoxide anion as a product of NOX1 and NOX2 activity. NOX4 activation does not require the recruitment of cytosolic subunits and generates H2O2 rather than the superoxide anion.5
Flavanol (−)-epicatechin, which is present in many plants consumed by humans, has been proposed as a modulator of NOX-dependent oxidant production in vivo in many tissues subjected to stress conditions.6,7 The mechanisms behind such effects imply the inhibition of the overexpression of different NOX subunits.8–16 Moreover, in vivo anti-inflammatory effects have been reported for (−)-epicatechin through downregulation of the NF-κB activation pathway in fructose overload and other stress models.8,11,14–16 In this study, high fructose feeding triggered NF-κB activation in the renal cortex of rats. This was associated with increase in NOXO1 and NOX4 expression levels without changes in TLR-4 expression. Dietary (−)-epicatechin could prevent NF-κB activation at multiple steps in association with a decrease in NOX4 expression.
Materials and methods
Materials
Primary antibodies for TLR-4 (#30002), NOX4 (#21860) and β-actin (#47778) and secondary antibodies rabbit anti-goat IgG-HRP (#2768), mouse anti-rabbit IgG-HRP (#2357), and goat anti-mouse IgG-HRP (#2005) were obtained from Santa Cruz Biotechnology (Santa Cruz, CA, USA). The primary and secondary antibodies for IκB kinase α (IKKα) (#11930), p-IKKα/β (Ser176/180) (#2697), inhibitor of κBα (IκBα) (#4814), p-IκBα (Ser32) (#2859), NF-κB p65 (#8242) and p-NF-κB p65 (Ser536) (#3033) were obtained from Cell Signaling Technology (Boston, MA, USA). Primary antibodies for NOX1 (ab46545) and NOXO1 (ab131205) were purchased from Abcam Inc. (Cambridge, MA, USA). (−)-Epicatechin was obtained from Sigma Chemical Co. (St Louis, MO, USA), and fructose (purity ≥99.5%) was obtained from Droguería Saporiti (Buenos Aires, Argentina). Commercial rat chow was purchased from Gepsa-Feeds (Buenos Aires, Argentina).
Animals, diets and experimental design
All procedures were in agreement with the standards for the care of laboratory animals outlined in the National Institutes of Health Guide for Care and Use of Laboratory Animals (NIH Pub. No. 85-23, revised 1996) and were approved by the Secretary of Science and Technology of the School of Medicine, National University of Cuyo. Male Sprague Dawley rats were housed under the conditions of controlled temperature (21–25 °C) and humidity with a 12 h light/dark cycle. Rats weighing 130 ± 20 g were randomly divided into the following groups (8 rats per group): control (C), receiving tap water ad libitum and the standard rat chow diet; fructose (F), receiving 10% fructose (w/v) in the drinking water and the standard rat chow diet; and fructose-(−)-epicatechin (FE), receiving 10% fructose (w/v) in the drinking water and the standard rat chow diet with (−)-epicatechin added to reach 20 mg per kg of body weight per d.14 The (−)-epicatechin provided to the rats is equivalent to 200 mg d−1 for a 70 kg human (considering a factor of 7 to convert rat and human doses),17 an amount that is attainable through optimizing fruit and vegetable intake and/or pharmacological strategies.9,18 After 8 weeks on the respective treatments, the rats were weighed and anesthetized with ketamine (50 mg per kg of body weight) and acepromazine (1 mg per kg of body weight). The kidneys were excised, and the kidney cortex was immediately isolated and processed for western blot analysis.
Western blot analysis
A portion of the renal cortex was homogenized in lysis buffer (150 mM NaCl, 50 mM Trizma-HCl, 1% (v/v) NP-40, pH: 8.0) in the presence of protease and phosphatase inhibitors and centrifuged at 600g for 20 min. The supernatant was collected and used as the total homogenate. Protein content was measured by the Lowry method. The homogenates were added with 2× solution of sample buffer (62.5 mM Tris-HCl, pH: 6.8 containing 2% (w/v) SDS, 25% (w/v) glycerol, 5% (v/v) β-mercaptoethanol, and 0.01% (w/v) bromophenol blue) and heated at 95 °C for 2 min. Sample aliquots containing 30 μg of protein were subjected to reducing 10% (w/v) polyacrylamide gel electrophoresis and then transferred to polyvinylidene difluoride membranes. Colored molecular weight standards (GE Healthcare, Piscataway, NJ, USA) were run simultaneously. Membranes were blotted for 2 h in 5% (w/v) nonfat milk and incubated overnight in the presence of the corresponding primary antibodies (1
:
1000 dilution in PBS). After subsequent incubation for 90 min at room temperature in the presence of the corresponding HRP-conjugated secondary antibody (1
:
5000 dilution in PBS), the complexes were visualized by chemiluminescence. Films were scanned, and densitometric analysis was performed using Image J (National Institute of Health, Bethesda, Maryland, USA). The protein bands were normalized to the β-actin content.
Statistical analysis
Results are shown as mean ± SEM. Data were analyzed by one-way analysis of variance (ANOVA) using StatView 5.0 (SAS Institute, Cary, NC, USA), and Fisher's significance difference test was used to examine the differences between group means. A value of p < 0.05 is considered statistically significant.
Results and discussion
We have previously shown that (−)-epicatechin attenuated the renal pro-inflammatory state induced by the consumption of high-fructose diet in rats. This was associated with the attenuation of NOX2 activation.8 To extend these results, in the present study, we evaluated the effects of (−)-epicatechin on the activation of NF-κB and on NOX expression. The obtained results indicate that by preventing the activation of select NOXs, (−)-epicatechin prevents the activation of NF-κB in the kidney cortex.
In a preceding paper using the same set of rats, both high-fructose feeding and (−)-epicatechin modified several metabolic and kidney function parameters. Of importance for the present results, kidney/body weight and serum creatinine were not modified by the diets, but increased proteinuria (71%, F with respect to C) associated with fructose consumption was not observed in FE rats. These results indicate that in F rats, there was incipient renal damage that was not enough in extent and/or time to affect most metabolic parameters and damage was prevented by (−)-epicatechin administration.8
TLR-4 is key in mediating NF-κB activation initiated extracellularly.19 In this study, no changes were found in TLR-4 expression in the kidney cortex as a result of both high-fructose feeding and (−)-epicatechin administration (Fig. 1A). These results suggest that most of the observed changes are not dictated by the basal expression of TLR-4 and can possibly be events initiated by intracellular agents such as oxidants. The observed unmodified basal levels of TLR-4 are in contrast with other previously reported results showing an overexpression of TLR-4 in the kidney of high-fructose-fed20–22 or LPS-treated11,23 rodents. It is important to note that in LPS-treated rats, TLR-4 was overexpressed, and (−)-epicatechin was active to prevent such overexpression and the associated activation of NF-κB.11
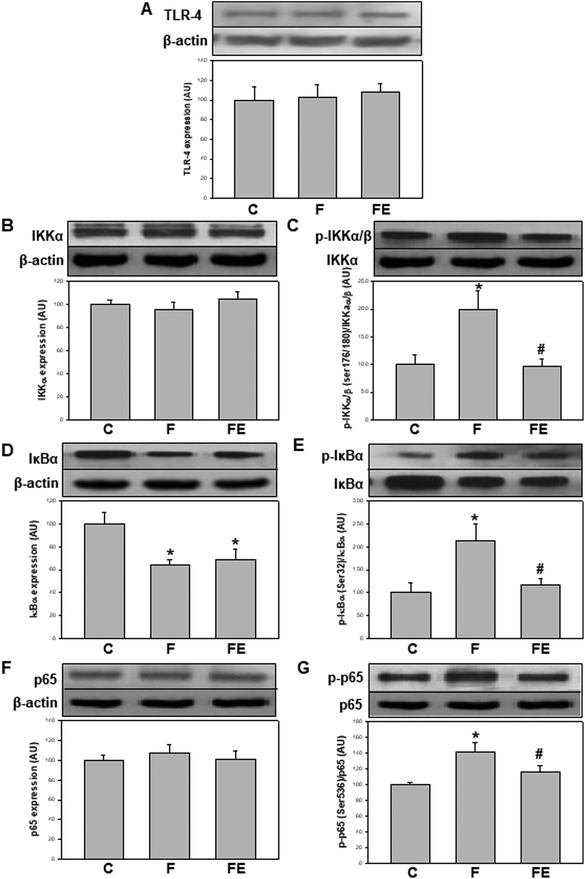 |
| Fig. 1 Effects of dietary (−)-epicatechin on TLR-4 expression and NF-κB signaling pathway in the renal cortex of high-fructose-fed rats. TLR-4 expression (A), IKKα content (B), IKKα/β phosphorylation at Ser(176/180) (C), IκB content (D), IκBα phosphorylation at Ser(32) (E), NF-κB p65 content (F) and NF-κB p65 phosphorylation at Ser(536) (G) determined by western blot in homogenates of the renal cortex from C: control, F: fructose, and FE: fructose-(−)-epicatechin groups. Values are expressed as mean ± SEM; n = 8 rats per group. *p < 0.05 respect to C, #p < 0.05 respect to F. | |
NF-κB activation occurs through several regulatory events. IKKα content was similar in the three experimental groups (Fig. 1B). Ikkα/β phosphorylation at Ser(176/180) (p-IKKα/β) was significantly higher in the F group compared to that in the C and FE groups (+99% and +108%, respectively) (Fig. 1C). The total content of IκBα, which is degraded during NF-κB activation, was significantly lower in the F and FE groups compared to that in the C group (−37% and −31%, respectively) (Fig. 1D). The levels of IκBα phosphorylation at Ser(32) (p-IκBα) were significantly higher in the F group compared to those in the C and FE groups (+113% and +80%, respectively) (Fig. 1E). NF-κB p65 content showed no changes among the experimental groups (Fig. 1F), but the activating phosphorylation at Ser(536) was significantly higher in the F group compared to that in the C and FE groups (+41% and +22% respectively) (Fig. 1G). These results indicate that (−)-epicatechin inhibited the phosphorylation steps that control the activation of the NF-κB pathway induced by high fructose feeding. Similar modulatory effects of (−)-epicatechin on NF-κB activation were observed in the kidney of LPS-treated rats.11 However, in that experimental setting, IκBα protein levels were returned to control values by (−)-epicatechin treatment, whereas in the present experiments, (−)-epicatechin had no effect. Such discrepancies between total IκBα protein content and other parameters of NF-κB activation could be ascribed to the complex turnover of IκBα associated with different kinetics of synthesis and degradation of free and NF-κB-bound IκBα.24
Oxidant levels in cells are closely associated with NF-κB activation.25 Early reports have demonstrated that the activation of NF-κB is associated with cellular oxidative stress26–28 and the expressions of some NOX subunits are regulated by NF-κB.29,30 Then, a cycle involving NOX-derived oxidants and activation of NF-κB has been proposed and supported by extensive experimental evidence.31–33 However, the initiating event in this cycle, i.e., the NF-κB pathway activation or NOX activation is highly dependent on challenging conditions and physiological response.25
In terms of the regulation of both, oxidant production and NOX activities, we previously showed that the consumption of high-fructose diets increases superoxide anion production and the expression of NOX2 subunits p47phox and gp91phox in rat kidneys. The administration of (−)-epicatechin counteracted the increments in superoxide anion production and only the expression of p47phox in the renal cortex.8 Here, we investigated the regulation of other NOX isoforms following high fructose consumption and (−)-epicatechin administration. NOX1 expression was similar in the three experimental groups (Fig. 2A), but NOXO1 protein levels were 86% higher in F and FE groups compared to that for the C group (p < 0.05) (Fig. 2B). These results suggest greater NOX1 activity mediated by higher NOXO1 expression in high-fructose-fed rats, an effect that was not modified by (−)-epicatechin administration. The absence of the effect of (−)-epicatechin on the activation of NOX-1, especially on NOXO1, can sustain increased superoxide anion production that is responsible for the remaining/residual effects of the high-fructose diet on some renal parameters, for which alterations were not completely prevented by (−)-epicatechin. NOX4 expressions were 61% and 46% higher in the F group compared to that for C and FE groups (p < 0.05) (Fig. 2C), indicating increased intracellular production of H2O2 after high fructose consumption. This effect was prevented by (−)-epicatechin, as it was previously observed in the aorta, liver, and adipose tissue of high-fructose-fed rats.9,14 This regulation of NOX4 by (−)-epicatechin can be of relevance because NOX4 activity is dictated solely by the expression of this protein, which is a direct manner of controlling oxidant production.
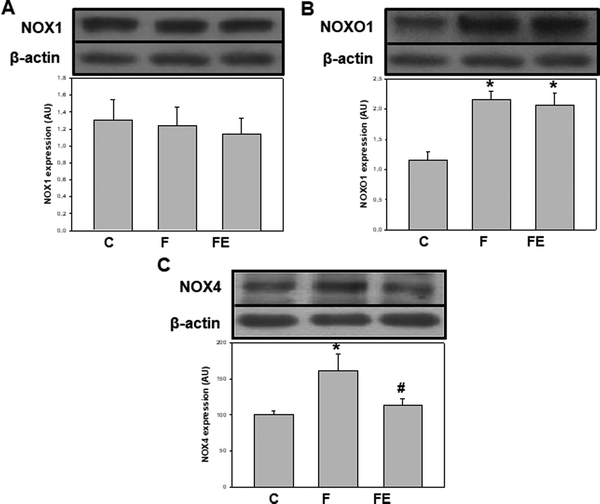 |
| Fig. 2 Effects of dietary (−)-epicatechin on NOX1, NOXO1, and NOX4 expressions in the renal cortex of high-fructose-fed rats. Protein expression levels were measured by western blot in homogenates of the renal cortex from C: control, F: fructose; and FE: fructose-(−)-epicatechin groups. Values are expressed as mean ± SEM; n = 8 rats per group. *p < 0.05 respect to C, #p < 0.05 respect to F. | |
By integrating the present experiments with previous observations,32–36 we can conclude that renal NOXs responded differentially to a high-fructose diet and to (−)-epicatechin administration. As summarized in Table 1, excessive fructose in the diet was mostly associated with higher NOX activation that followed different patterns: (i) higher expression of NOX2 activating and catalytic subunits, (ii) higher expression of the NOX1 activating subunit, and (iii) higher expression of the NOX4 catalytic subunit. These differences could reflect specific activation mechanisms for each NOX isoform. (−)-Epicatechin supplementation led to changes only in NOX2 and NOX4 that could decrease enzymatic activation by avoiding the overexpression of the organizer subunit of NOX2 and that of the catalytic subunit of NOX4.
Table 1 Changes in the expression of NOX subunits by high-fructose and (−)-epicatechin supplemented diets in rat kidney cortex
|
Protein expression (fold increase with respect to untreated controls) |
F |
FE |
F: fructose and FE: fructose-(−)-epicatechin groups. Taken from ref. 8. |
NOX2a |
Catalytic subunit (gp91phox) |
1.5 |
1.5 |
Activator subunit (p47phox) |
1.3 |
1.0 |
NOX1 |
Catalytic subunit (NOX1) |
1.0 |
1.0 |
Activator subunit (NOXO1) |
1.9 |
1.9 |
NOX4 |
Catalytic subunit (NOX4) |
1.6 |
1.0 |
Regarding the sequence of NF-κB and NOXs activations, our results suggest that (−)-epicatechin regulates the NF-κB signaling pathway through the modulation of NOX2 and NOX4. Then, the production of superoxide anion by NOX2 and/or the production of H2O2 by NOX4 can define the redox regulation of NF-κB activation probably initiated by the phosphorylation and stabilization of IKKα. In this regard, it was demonstrated that H2O2 favors the activating phosphorylation of the IKK complex by inducing protein kinase D activity through Src-mediated signaling pathways37 and mitogen-activated protein kinase kinase kinase (MAP3K).38 Additionally, H2O2 can maintain high levels of activated IKK complexes through inactivation of protein phosphatases by oxidative modifications.39 Finally, in agreement with the increased ratio p-IκBα/IκBα and the lower IκBα protein expression found in the F group, it has been postulated that H2O2 induces IκBα phosphorylation at several tyrosine residues, favoring its proteasomal degradation.40,41
Overall, the present results further describe the mechanisms associated with the prevention of the deleterious effects of high fructose feeding by (−)-epicatechin supplementation. In addition, this study supports the potential use of (−)-epicatechin-enriched foods or supplements as a nutritional tool to help down-regulate redox-sensitive pro-inflammatory conditions such as those found in diabetes, hypertension and dyslipidemia.42,43
Abbreviations
IκBα | Inhibitor of κBα |
NF-κB | Nuclear factor κB |
NOX | NADPH oxidase |
NOXO1 | NOX1 organizer |
TLR-4 | Toll-like receptor 4 |
Conflicts of interest
There are no conflicts of interest to declare.
Acknowledgements
This work was supported by grants from the Universidad de Buenos Aires (UBACyT 20020160100132BA to CGF, and 20020170100586BA to MG) and the Consejo Nacional de Investigaciones Científicas y Técnicas (CONICET) (PIP 11220170100585CO to MG).
References
- C. Franceschi, P. Garagnani, P. Parini, C. Giuliani and A. Santoro, Inflammaging: a new immune-metabolic viewpoint for age-related diseases, Nat. Rev. Endocrinol., 2018, 14, 576–590 CrossRef CAS PubMed.
- C. Erridge and J. Endogenous, ligands of TLR2 and TLR4: agonists or assistants?, J. Leukocyte Biol., 2010, 87, 989–999 CrossRef CAS PubMed.
- A. G. Erlejman, G. Jaggers, C. G. Fraga and P. I. Oteiza, TNFalpha-induced NF-kappaB activation and cell oxidant production are modulated by hexameric procyanidins in Caco-2 cells, Arch. Biochem. Biophys., 2008, 476, 186–195 CrossRef CAS PubMed.
- P. S. Gill and C. S. Wilcox, NADPH oxidases in the kidney, Antioxid. Redox Signaling, 2006, 8, 1597–1607 CrossRef CAS PubMed.
- R. P. Brandes, N. Weissmann and K. Schröder, Nox family NADPH oxidases in mechano-transduction: mechanisms and consequences, Antioxid. Redox Signaling, 2014, 20, 887–898 CrossRef CAS PubMed.
- C. G. Fraga, P. I. Oteiza and M. Galleano, Plant bioactives and redox signaling: (-)-Epicatechin as a paradigm, Mol. Aspects Med., 2018, 61, 31–40 CrossRef CAS PubMed.
- C. G. Fraga and P. I. Oteiza, Dietary flavonoids: Role of (-)-epicatechin and related procyanidins in cell signaling, Free Radicals Biol. Med., 2011, 51, 813–823 CrossRef CAS PubMed.
- P. D. Prince, C. R. Lanzi, J. E. Toblli, R. Elesgaray, P. I. Oteiza, C. G. Fraga and M. Galleano, Dietary (-)-epicatechin mitigates oxidative stress, NO metabolism alterations, and inflammation in renal cortex from fructose-fed rats, Free Radicals Biol. Med., 2016, 90, 35–46 CrossRef CAS PubMed.
- M. C. Litterio, M. A. Vazquez Prieto, A. M. Adamo, R. Elesgaray, P. I. Oteiza, M. Galleano and C. G. Fraga, (-)-Epicatechin reduces blood pressure increase in high-fructose-fed rats: effects on the determinants of nitric oxide bioavailability, J. Nutr. Biochem., 2015, 26, 745–751 CrossRef CAS PubMed.
- V. Calabró, B. Piotrkowski, L. Fischerman, M. A. Vazquez Prieto, M. Galleano and C. G. Fraga, Modifications in nitric oxide and superoxide anion metabolism induced by fructose overload in rat heart are prevented by (-)-epicatechin, Food Funct., 2016, 7, 1876–1883 RSC.
- P. D. Prince, L. Fischerman, J. E. Toblli, C. G. Fraga and M. Galleano, LPS-induced renal inflammation is prevented by (−)-epicatechin in rats, Redox Biol., 2017, 11, 342–349 CrossRef CAS PubMed.
- B. Piotrkowski, V. Calabró, M. Galleano and C. G. Fraga, (-)-Epicatechin prevents alterations in the metabolism of superoxide anion and nitric oxide in the hearts of L-NAME-treated rats, Food Funct., 2015, 6, 155–161 RSC.
- M. C. Litterio, G. Jaggers, G. Sagdicoglu Celep, A. M. Adamo, M. A. Costa, P. I. Oteiza, C. G. Fraga and M. Galleano, Blood pressure-lowering effect of dietary (-)-epicatechin administration in L-NAME-treated rats is associated with restored nitric oxide levels, Free Radicals Biol. Med., 2012, 53, 1894–1902 CrossRef CAS PubMed.
- A. Bettaieb, M. A. Vazquez Prieto, C. Rodriguez Lanzi, R. M. Miatello, F. G. Haj, C. G. Fraga and P. I. Oteiza, (-)-Epicatechin mitigates high-fructose-associated insulin resistance by modulating redox signaling and endoplasmic reticulum stress, Free Radicals Biol. Med., 2014, 72, 247–256 CrossRef CAS PubMed.
- A. Bettaieb, E. Cremonini, H. Kang, J. Kang, F. G. Haj and P. I. Oteiza, Anti-inflammatory actions of (-)-epicatechin in the adipose tissue of obese mice, Int. J. Biochem. Cell Biol., 2016, 81, 383–392 CrossRef CAS PubMed.
- E. Cremonini, Z. Wang, A. Bettaieb, A. M. Adamo, E. Daveri, D. A. Mills, K. M. Kalanetra, F. G. Haj, S. Karakas and P. I. Oteiza, (-)-Epicatechin protects the intestinal barrier from high fat diet-induced permeabilization: Implications for steatosis and insulin resistance, Redox Biol., 2018, 14, 588–599 CrossRef CAS PubMed.
- E. J. Freireich, E. A. Gehan, D. P. Rall, L. H. Schmidt and H. E. Skipper, Quantitative comparison of toxicity of anticancer agents in mouse, rat, hamster, dog, monkey, and man, Cancer Chemother. Rep., 1966, 50, 219–244 CAS.
- A. Vogiatzoglou, A. A. Mulligan, M. A. H. Lentjes, R. N. Luben, J. P. E. Spencer, H. Schroeter, K.-T. Khaw and G. G. C. Kuhnle, Flavonoid intake in European adults (18 to 64 years), PLoS One, 2015, 10, e0128132 CrossRef PubMed.
- G. Zhang and S. Ghosh, Toll-like receptor-mediated NF-kappaB activation: a phylogenetically conserved paradigm in innate immunity, J. Clin. Invest., 2001, 107, 13–19 CrossRef CAS PubMed.
- A. R. Aroor, G. Jia, J. Habibi, Z. Sun, F. I. Ramirez-Perez, B. Brady, D. Chen, L. A. Martinez-Lemus, C. Manrique, R. Nistala, A. T. Whaley-Connell, V. G. Demarco, G. A. Meininger and J. R. Sowers, Uric acid promotes vascular stiffness, maladaptive inflammatory responses and proteinuria in western diet fed mice, Metab., Clin. Exp., 2017, 74, 32–40 CrossRef CAS PubMed.
- Y. Yang, D.-M. Zhang, J.-H. Liu, L.-S. Hu, Q.-C. Xue, X.-Q. Ding and L.-D. Kong, Wuling San protects kidney dysfunction by inhibiting renal TLR4/MyD88 signaling and NLRP3 inflammasome activation in high fructose-induced hyperuricemic mice, J. Ethnopharmacol., 2015, 169, 49–59 CrossRef PubMed.
- M.-X. Wang, X.-J. Zhao, T.-Y. Chen, Y.-L. Liu, R.-Q. Jiao, J.-H. Zhang, C. Ma, J.-H. Liu, Y. Pan and L.-D. Kong, Nuciferine alleviates renal injury by inhibiting inflammatory responses in fructose-fed rats, J. Agric. Food Chem., 2016, 64, 7899–7910 CrossRef CAS PubMed.
- A. R. Nair, G. S. Masson, P. J. Ebenezer, F. Del Piero and J. Francis, Role of TLR4 in lipopolysaccharide-induced acute kidney injury: protection by blueberry, Free Radicals Biol. Med., 2014, 71, 16–25 CrossRef CAS PubMed.
- E. Mathes, E. L. O'Dea, A. Hoffmann and G. Ghosh, NF-kappaB dictates the degradation pathway of IkappaBalpha, EMBO J., 2008, 27, 1357–1367 CrossRef CAS PubMed.
- M. J. Morgan and Z. Liu, Crosstalk of reactive oxygen species and NF-κB signaling, Cell Res., 2011, 21, 103–115 CrossRef CAS PubMed.
- L. Flohé, R. Brigelius-Flohé, C. Saliou, M. G. Traber and L. Packer, Redox regulation of NF-kappa B activation, Free Radicals Biol. Med., 1997, 22, 1115–1126 CrossRef.
- H. Zhang, H. Spapen, D. N. Nguyen, M. Benlabed, W. A. Buurman and J. L. Vincent, Protective effects of N-acetyl-L-cysteine in endotoxemia, Am. J. Physiol., 1994, 266, H1746–H1754 CrossRef CAS PubMed.
- W. Erl, C. Weber, C. Wardemann and P. C. Weber, alpha-Tocopheryl succinate inhibits monocytic cell adhesion to endothelial cells by suppressing NF-kappa B mobilization, Am. J. Physiol., 1997, 273, H634–H640 CrossRef CAS PubMed.
- A. Manea, L. I. Tanase, M. Raicu and M. Simionescu, Transcriptional regulation of NADPH oxidase isoforms, Nox1 and Nox4, by nuclear factor-kappaB in human aortic smooth muscle cells, Biochem. Biophys. Res. Commun., 2010, 396, 901–907 CrossRef CAS PubMed.
- A. Manea, S. A. Manea, A. V. Gafencu and M. Raicu, Regulation of NADPH oxidase subunit p22(phox) by NF-kB in human aortic smooth muscle cells, Arch. Physiol. Biochem., 2007, 113, 163–172 CrossRef CAS PubMed.
- Q. Li, N. Y. Spencer, F. D. Oakley, G. R. Buettner and J. F. Engelhardt, Endosomal Nox2 facilitates redox-dependent induction of NF-kappaB by TNF-alpha, Antioxid. Redox Signaling, 2009, 11, 1249–1263 CrossRef CAS PubMed.
- J. Kim, M. Seo, S. K. Kim and Y. S. Bae, Flagellin-induced NADPH oxidase 4 activation is involved in atherosclerosis, Sci. Rep., 2016, 6, 25437 CrossRef CAS PubMed.
- F. J. Miller, M. Filali, G. J. Huss, B. Stanic, A. Chamseddine, T. J. Barna and F. S. Lamb, Cytokine activation of nuclear factor kappa B in vascular smooth muscle cells requires signaling endosomes containing Nox1 and ClC-3, Circ. Res., 2007, 101, 663–671 CrossRef CAS PubMed.
- K. L. Siu, L. Gao and H. Cai, Differential roles of protein complexes NOX1-NOXO1 and NOX2-p47phox in mediating endothelial redox responses to oscillatory and unidirectional laminar shear stress, J. Biol. Chem., 2016, 291, 8653–8662 CrossRef CAS PubMed.
- J. A. Byrne, D. J. Grieve, J. K. Bendall, J.-M. Li, C. Gove, J. D. Lambeth, A. C. Cave and A. M. Shah, Contrasting roles of NADPH oxidase isoforms in pressure-overload versus angiotensin II-induced cardiac hypertrophy, Circ. Res., 2003, 93, 802–805 CrossRef CAS PubMed.
- A. C. C. Loureiro, I. C. do Rêgo-Monteiro, R. A. Louzada, V. H. Ortenzi, A. P. de Aguiar, E. S. de Abreu, J. P. A. Cavalcanti-de-Albuquerque, F. Hecht, A. C. de Oliveira, V. M. Ceccatto, R. S. Fortunato and D. P. Carvalho, Differential expression of NADPH oxidases depends on skeletal muscle fiber type in rats, Oxid. Med. Cell. Longevity, 2016, 2016, 6738701 Search PubMed.
- P. Storz, H. Döppler and A. Toker, Protein kinase Cdelta selectively regulates protein kinase D-dependent activation of NF-kappaB in oxidative stress signaling, Mol. Cell. Biol., 2004, 24, 2614–2626 CrossRef CAS PubMed.
- H. Kamata, T. Manabe, S. Ichi Oka, K. Kamata and H. Hirata, Hydrogen peroxide activates IkappaB kinases through phosphorylation of serine residues in the activation loops, FEBS Lett., 2002, 519, 231–237 CrossRef CAS.
- F. Rusnak and T. Reiter, Sensing electrons: protein phosphatase redox regulation, Trends Biochem. Sci., 2000, 25, 527–529 CrossRef CAS PubMed.
- S. Schoonbroodt, V. Ferreira, M. Best-Belpomme, J. R. Boelaert, S. Legrand-Poels, M. Korner and J. Piette, Crucial role of the amino-terminal tyrosine residue 42 and the carboxyl-terminal PEST domain of I kappa B alpha in NF-kappa B activation by an oxidative stress, J. Immunol., 2000, 164, 4292–4300 CrossRef CAS.
- Y. Takada, A. Mukhopadhyay, G. C. Kundu, G. H. Mahabeleshwar, S. Singh and B. B. Aggarwal, Hydrogen peroxide activates NF-kappa B through tyrosine phosphorylation of I kappa B alpha and serine phosphorylation of p65: evidence for the involvement of I kappa B alpha kinase and Syk protein-tyrosine kinase, J. Biol. Chem., 2003, 278, 24233–24241 CrossRef CAS PubMed.
- D. Pitocco, M. Tesauro, R. Alessandro, G. Ghirlanda and C. Cardillo, Oxidative stress in diabetes: implications for vascular and other complications, Int. J. Mol. Sci., 2013, 14, 21525–21550 CrossRef PubMed.
- Y. Solak, B. Afsar, N. D. Vaziri, G. Aslan, C. E. Yalcin, A. Covic and M. Kanbay, Hypertension as an autoimmune and inflammatory disease, Hypertens. Res., 2016, 39, 567–573 CrossRef CAS PubMed.
Footnote |
† These authors share senior authorship. |
|
This journal is © The Royal Society of Chemistry 2019 |
Click here to see how this site uses Cookies. View our privacy policy here.