DOI:
10.1039/C8RA09958H
(Paper)
RSC Adv., 2019,
9, 1602-1612
The modification mechanism and the effect of magnesium chloride on poly(vinyl alcohol) films
Received
4th December 2018
, Accepted 31st December 2018
First published on 11th January 2019
Abstract
The modification mechanism and the effect of MgCl2 on poly(vinyl alcohol) and the effect of water in the system were studied in this paper. The effect of MgCl2 on different fully dried PVA was investigated by Fourier transform infrared spectroscopy, X-ray diffraction, differential scanning calorimeter, thermogravimetric analysis, water content test, dynamic thermomechanical analysis and tensile test, respectively. The results showed that MgCl2 was an effective strengthening agent, which could crosslink with PVA and then effectively decrease the crystallinity of PVA. With the addition of MgCl2, the strength and rigidity of PVA increased and the melting temperature decreased. The effect of MgCl2 on thermal stability of PVA was related to the alcoholysis degree of PVA. The tensile strength of PVA films modified with MgCl2 were all over 120 MPa. Moreover, the effect of MgCl2 on the dynamic thermomechanical properties and tensile properties of PVA with various content of water was studied. Water could interact with MgCl2 to destroy the crosslink between MgCl2 and PVA. In the presence of water, PVA modified with MgCl2 became soft, with lower tensile strength and higher elongation at break.
1 Introduction
Polyvinyl alcohol (PVA) is an environmentally friendly material with excellent gas barrier properties, high strength, tear and flexibility. However, the strong hydrogen bonds among inter- and intra- molecular chains of PVA result in high crystallinity. This makes the pure PVA film brittle and easy to break during preparation and application. The poor mechanical properties have restricted the development of PVA materials. Meanwhile, the high melting point and low decomposition temperature give PVA a narrow thermoplastic processing window resulting in the difficulty of using conventional thermoplastic methods to process. Adding a plasticizer is the most commonly used way to improve the properties of PVA. The most commonly used plasticizer for PVA are water, glycerol, ethylene glycol, poly(ethylene glycol) and caprolactam.1,2 These plasticizers can form interaction with the hydroxyl groups of PVA and then destroy the inter- and intra- hydrogen bonding to reach the aim of plasticizing. However, the plasticizing efficiency of them is not high enough to meet the demand of application. Many kinds of inorganic salts have been reported as a new and high efficient plasticizer for PVA.3,4 Previous studies have proved that some inorganic salts could decrease the crystallinity of PVA and could be helpful to form new crystals.5–7 Moreover, some inorganic salts could play a role as heterogeneous nucleating agent of PVA to increase the crystallinity of PVA.8 This indicated that with the addition of suitable inorganic salts, it could be expected to prepare PVA with predetermined crystallinity. From then on, the studies of modifying PVA with various kinds of inorganic salts increase gradually.9–12 MgCl2 was always considered as a plasticizer of the system in previous studies. However, the effect of water could not be ignored. Thence, the modification mechanism of inorganic salts on PVA remains to further investigation.
Generally, water has a great influence on the properties of PVA films. Water could play a role as plasticizer by forming interaction with the –OH of PVA molecule chains. Lots of researches have been investigating the effect of water on PVA.3,4,13,14 However, these studies always focused on the state of water in PVA, the destroying mechanism and the plasticization of water on the crystal of PVA.15–18 The effect of water on mechanical properties of PVA and the effect of water in the process of modification of PVA with inorganic salts rarely get attention.
The aim of this paper is to study the way of MgCl2 influencing PVA by investigating the effect of MgCl2 on the structure and properties of PVA with different alcoholysis degree and polymerization degree after sufficient drying. In addition, the effect of water in the system was studied in this paper. It could be excepted that the modification mechanism of other inorganic salts could be revealed and PVA with more excellent properties could be prepared based on this study.
2 Experimental
Materials
PVA1799 (DP = 1700, degree of hydrolysis 99%), PVA1788 (DP = 1700, degree of hydrolysis 88%), PVA1792 (DP = 1700, degree of hydrolysis 92%), PVA2488 (DP = 2400, degree of hydrolysis 88%) and PVA2499 (DP = 2400, degree of hydrolysis 99%), were provided by Sichuan Vinylon Factory, SINOPEC (China). MgCl2·6H2O was purchased from Kelong Chemical Co. (Chengdu, China), which was used without any further purification.
Sample preparation
PVA films were prepared by the casting method. First, 5 g PVA and 2 wt%, 4 wt%, 6 wt%, 8 wt%, 10 wt%, 12 wt% and 15 wt% MgCl2·6H2O were dissolved in distilled water by heating in an oil bath at 90 °C for 3 h, and the aqueous solutions were prepared, which called the film-forming solution. The film-forming solutions were casted onto the Petri-dish and dried at 60 °C for 48 h to eliminate water completely. According to the content of MgCl2·6H2O, the PVA1799 films were called as PVA, PM2, PM4, PM6, PM8, PPM10, PM12 and PM15; the PVA1788 films were called as 880, 884, 886, 888, 8810 and 8815; the PVA1792 films were called as 1792-0, 1792-4, 1792-10 and 1792-15; the PVA2488 films were called as 2488-0, 2488-4, 2488-10, 2488-15.
Fully dried sample preparation: the film-forming solutions were coated on the PTFE sheet and dried at 60 °C for 48 h. All samples were dried at 105 °C for 5 h under vacuum (P < 0.3 MPa).
Sample with different water content preparation: PVA, PM4, PM6, PM8 and PM10 were dried at 105 °C for 5 h under vacuum (P < 0.3 MPa). Then these films were stored at the environment of high humidity to prepare PVA films with 4% and 12% content water.
Characterizations
Fourier transform infrared (FT-IR) spectroscopy. The infrared spectra were measured using a Fourier transform infrared spectrophotometer (Nicolet 560). The film-forming solutions prepared according to Table 1 was casted on a Petri dish and dried at 60 °C to remove water. The measurements were carried out with a resolution of 4 cm−1.
Table 1 DSC analysis of thermal characteristic of PVA1799 and PVA1788 with various contents of MgCl2·6H2O
|
PVA |
PM4 |
PM6 |
PM8 |
PM10 |
PM12 |
880 |
884 |
886 |
888 |
8810 |
Tg/°C |
67.8 |
76.9 |
92.1 |
81.5 |
80.4 |
76.8 |
69.8 |
69.8 |
87.8 |
93.2 |
97.4 |
Tα/°C |
139 |
145 |
143 |
146 |
145 |
149 |
116 |
115 |
141 |
|
|
Tm/°C |
228 |
226 |
219 |
221 |
214 |
203 |
196 |
193 |
Double peak |
Double peak |
151 |
X-ray diffraction (XRD) measurements. The XRD test was carried out using an X'Pert Pro MPD diffractometer manufactured by Philips Analytical Company of Netherlands, with nickel filter Cu Kα as the radiation source. The acceleration voltage was 50 kV and the current was 35 mA. The samples were continuously scanned over the 2θ range from 5 to 50°
Differential scanning calorimetry (DSC). The DSC measurements were performed by a differential scanning calorimeter (NETZSCHDSC204). Samples total weight of 5–7 mg were weighted and were heated from 30 °C to 250 °C at a rate of 10 °C min−1 under a flow of nitrogen.
Thermal gravity analysis (TGA). TGA was performed by SDTQ600 TGA thermal analysis instrument (Du Pont). The samples for testing were heated from room temperature to 600 °C at a heating rate of 10 °C min−1.
Dynamic thermomechanical analysis (DMA). The dynamic mechanical measurements were carried out with DMA Q800 (TA, USA). The samples were cut as a dimension of 15.0 mm × 4.0 mm × 0.3 mm. The oscillatory frequency was 1 Hz. The temperature was raised from −50 to 150 °C at a constant rate of 3 °C min−1.
Mechanical testing. The mechanical properties (tensile strength (TS), elongation at break (%Eb) and Young's modulus) of the film was determined using a tensile testing equipment (Instron 5567). The crosshead speed was 20 mm min−1. The initial gauge length of the specimen was 20 mm. The width of each tensile sample was 4 mm. The film thickness was measured with a micrometer in triplicate. The samples were placed in an air environment at a relative humidity of 54% for one week before measurement. The required relative humidity was obtained by placing a saturated Mg(NO3)2 solution in a sealed box at 25 °C. The data were the average of 5–7 specimens.
Water absorption testing. The samples were dried at 105 °C for 5 h and were stored in constant temperature and constant humidity box (SANTN HTC-500) at 25 °C and a relative humidity of 54%. The films were weighed every 12 h and the water content were calculated based on the quality of PVA. The water content (WA) was obtained using the relationship.
We was the weight of the films after storing at the RH of 54% for one week, Wd was the weight of the films after drying sufficiently.
3 Results and discussion
FT-IR analysis
FT-IR was used to characterize the PVA1799 and PVA 1788 films before and after MgCl2 modification, and the results were shown in Fig. 1. In the IR spectrum, the peak at 3000–3500 cm−1 corresponds to the stretching vibration of –OH on PVA molecules, the peaks at 2942, 1430 and 1096 cm−1 are the characteristic of symmetric stretching vibration of C–H and bending stretching of CH2 and stretching vibration of C–O, respectively.19 Due to the lower degree of alcoholysis, there are a large number of ester groups on PVA1788 molecules. Thus, the carbonyl stretching vibration of ester groups at 1733 cm−1 could be found in the FT-IR spectra of PVA1788. It could be seen from Fig. 1(a) and (b) that PVA films exhibited a decrease in frequencies after the addition of MgCl2. This is because the electron interaction between Mg2+ and oxygen atom of –OH in PVA molecules was stronger than intermolecular and intramolecular hydrogen bonds of PVA. Therefore, MgCl2 could weak the hydrogen bonds. As a result, the absorption frequency of hydroxyl group of PVA1799 and PVA1788 shifted 10 cm−1 and 18 cm−1to a lower wavenumber with the addition of 10 wt% MgCl2·6H2O. The difference might arise from the different number of –OH in PVA1788 and PVA1799. There were less –OH in PVA1788, so that the percent of –OH on PVA1788 which could interact with MgCl2 was higher than that of PVA1799.
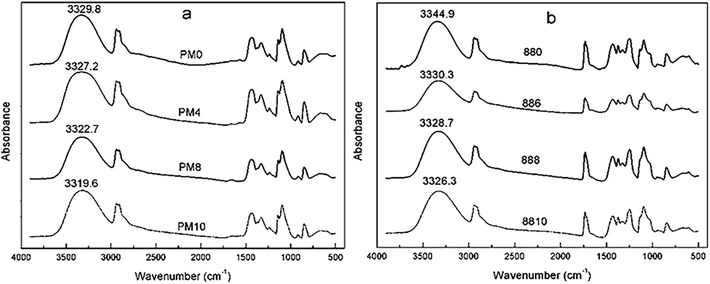 |
| Fig. 1 The FT-IR spectra of PVA1799 (a) and PVA1788 (b) with various contents of MgCl2·6H2O. | |
Crystalline properties
The X-ray diffraction patterns of PVA1799 and PVA1788 films before and after MgCl2 modification were displayed in Fig. 2(a) and (b). It could be seen that the diffraction peak of PVA appears at 2θ = 20° (d = 0.46 nm) and the diffraction peaks of PVA film decreased in intensity with the increasing of MgCl2 content. This phenomenon indicated that MgCl2 had a damaging effect on the crystals of PVA. This is because that MgCl2 could form strong interaction with PVA molecules and this interaction could interrupt the hydrogen bonding in the crystalline structure of PVA. The previous work has proved that the area under the diffraction peak or its intensity could be taken as a measurement of the degree of crystallinity. The decrease of the intensity of the diffraction peak indicated that the addition of MgCl2 could effectively decrease the degree of crystallinity of PVA films. It could also be noticed that the diffraction peaks of MgCl2 did not appear in the patterns. This also suggests that MgCl2 was completely dissolved in PVA films and no MgCl2 crystals or congregates existed.
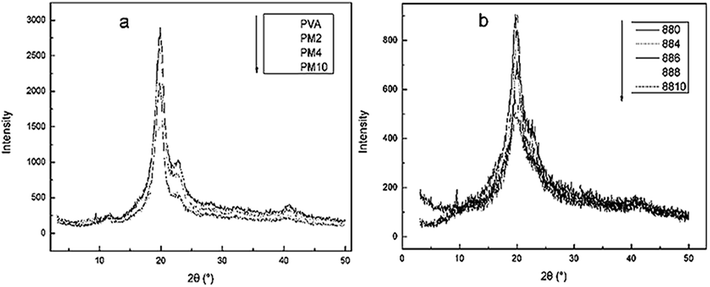 |
| Fig. 2 The XRD curves of PVA1799 (a) and PVA1788 (b) with various contents of MgCl2·6H2O. | |
Thermal properties
The DSC curves of PVA films modified with various contents of MgCl2·6H2O are displayed in Fig. 2, and the corresponding data are shown in Table 1.
With the increasing of MgCl2 content, both the Tg value of PVA1799 and PVA1788 increased. This is because MgCl2 could crosslink with PVA as Fig. 4 to some extent. This interaction between MgCl2 and PVA could weak the activity of PVA chain segments. Tα is the temperature at which chain segments of amorphous region near crystal region in PVA begin to motion. It could be found that the Tα value of PVA1799 increased with the increasing of content of MgCl2. This might be due to the influence of MgCl2 on crystal orientation and crystallinity of PVA.8 Moreover, this influence could be reflected by the change of melting peak. It could be seen from Fig. 3(a) that increasing content of MgCl2 decreased melting point, broadened melting peak and melting range.20 This indicated that the crystal structure of PVA was destroyed and the distribution of the crystal size became wider after the addition of MgCl2. In the case of PVA1788, with the addition of over 4 wt% MgCl2 content, the melting peak of PVA1788 turned from single peak to double peak and moved forward low temperature. While with the addition of 10 wt% MgCl2 content, the melting peak became single peak and the melting temperature decreased form 45 °C of pure PVA1788 to 151 °C. This proved that the effect of MgCl2 on PVA1788 was higher than PVA1799.
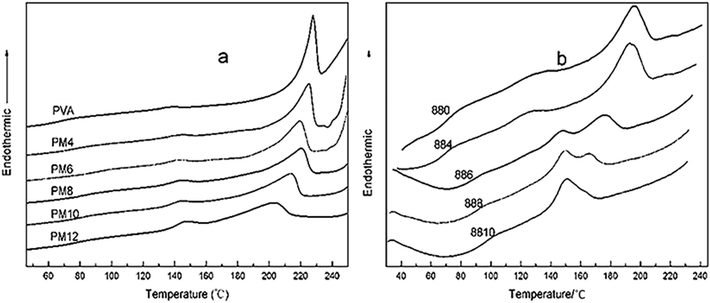 |
| Fig. 3 The DSC curves of PVA1799 (a) and PVA1788 (b) with various contents of MgCl2·6H2O. | |
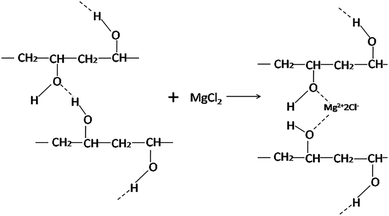 |
| Fig. 4 The interaction between MgCl2 and PVA. | |
In addition, the effect of MgCl2 on the thermal stability of PVA1799 and PVA1788 were studied. The thermal degradation of PVA could mainly be divided into two steps. Firstly, –OH and –OCOCH3 were eliminated to form H2O and CH3COOH. In the second step, the main chain of PVA broke and some organic volatiles were produced.21,22 It could be seen from Fig. 5, with the addition of 4 wt% MgCl2·6H2O, the Ti (the initial thermal decomposition temperature) and Tmax1 (the temperature of the biggest decomposition rate in the first step) increased from 237 °C, 256 °C of pure PVA1799 to 259 °C and 270 °C, respectively. Meanwhile, with the increasing dosage of MgCl2·6H2O, the Ti and Tmax1 increased constantly. This indicated that MgCl2 could increase the thermal stability of PVA1799. From Fig. 5(c) and (d) it could be seen that the temperature of two steps of pure PVA1788 were nearly the same, which was different with PVA1799. With the addition of 4 wt% MgCl2·6H2O, the TGA curve was basically in coincidence with that of pure PVA1788. However, when the dosage of MgCl2·6H2O reached 10 wt%, the first step moved toward the lower temperature. In addition, with the increase of the dosage of MgCl2·6H2O, the thermal stability of PVA1788 decreased constantly. Thus, the effect of MgCl2·6H2O on the thermal stability of PVA1792, PVA2488 and PVA2499 were studied to figure out the law of MgCl2 on the thermal stability of PVA.
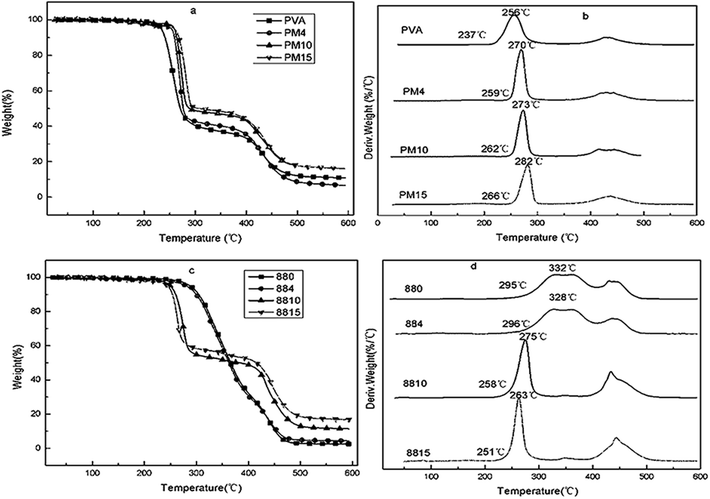 |
| Fig. 5 The TG and DTG curves of PVA1799 (a and b) and PVA1788 (c and d) with various contents of MgCl2·6H2O. | |
Thermal stability analysis
The effect of MgCl2·6H2O on the thermal stability of PVA1792, PVA2488 and PVA2499 were studied and the obtained TGA and DTG curves were shown in Fig. 6. The Ti and Tmax1 of PVA with different alcoholysis degree and polymerization degree before and after modifying with MgCl2 were recorded in Table 2.
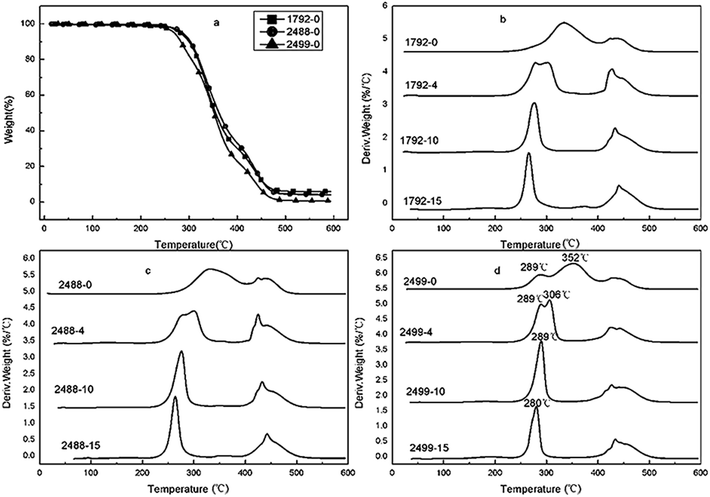 |
| Fig. 6 The TG (a) and DTG curves of PVA1792 (b), PVA2488 (c) and PVA2499 (d) with various contents of MgCl2·6H2O. | |
Table 2 The initial and the first maximum decomposition temperature of different types of PVA
|
Low alcoholysis |
High alcoholysis |
|
PVA1792-0 |
PVA1788-0 |
PVA2488-0 |
PVA1799-0 |
PVA2499-0 |
Ti |
296 °C |
295 °C |
296 °C |
237 °C |
265°C/293 °C |
Tmax1 |
333 °C |
332 °C |
332 °C |
256 °C |
289°C/352 °C |
![[thin space (1/6-em)]](https://www.rsc.org/images/entities/char_2009.gif) |
|
PVA1792-10 |
PVA1788-10 |
PVA2488-10 |
PVA1799-10 |
PVA2499-10 |
Ti |
260 °C |
258 |
258 °C |
262 °C |
274 °C |
Tmax1 |
276 °C |
275 °C |
276 °C |
273 °C |
289 °C |
![[thin space (1/6-em)]](https://www.rsc.org/images/entities/char_2009.gif) |
|
PVA1792-15 |
PVA1788-15 |
PVA2488-15 |
PVA1799-15 |
PVA2499-15 |
Ti |
253 °C |
251 °C |
252 °C |
266 °C |
267 °C |
Tmax1 |
265 °C |
263 °C |
264 °C |
282 °C |
280 °C |
For PVA1792, PVA1788 and PVA 2488 with low alcoholysis degree, both the Ti and Tmax1 of them were substantially the same. With the addition of 10 wt% MgCl2·6H2O, the Ti and Tmax1 of PVA1792, PVA1788 and PVA 2488 decreased from about 295 °C, 332 °C to 260 °C and 276 °C or so. While with the addition of 15 wt%, the Ti and Tmax1 would decrease to lower values. This indicated that MgCl2 could decrease the thermal stability of PVA with low alcoholysis degree, which was independent of the polymerization degree of PVA. This is probably because there were lots of residual ester groups in PVA with low alcoholysis degree. These ester groups had an effect on the interaction between MgCl2 and the –OH of PVA. As a result, hydroxyl and ester groups would remove at a lower temperature.
However, the effect of MgCl2 on PVA with higher alcoholysis degree was different with above situation. For PVA2499 with higher polymerization degree, there were two decomposition peaks in the first step at 289 °C and 352 °C, respectively. This is maybe because the residual ester groups showed an uneven distribution, thus the alcoholysis degree was higher than 99% in some parts but lower than 99% in other parts of PVA2499. According to the above analysis, it could be seen that the Tmax1 of PVA with lower alcoholysis degree was 332 °C. Thence, the peaks of the first step corresponded to the HA (decomposition of high alcoholysis parts) and LA (decomposition of low alcoholysis parts). With the addition of 4 wt% MgCl2·6H2O, the Tmax1 of HA had no changes, but the Tmax1 of LA decreased from 352 °C of pure PVA2499 to 306 °C. While with the addition of 10 wt% and 15 wt% MgCl2·6H2O, there was only one peak in the first decomposition step at 289 °C and 280 °C, respectively. Oppositely, for PVA1799 with lower polymerization degree, increasing dosage of MgCl2 increased the Ti and Tmax1. This indicated that the effect of MgCl2 on PVA with high alcoholysis was related to the polymerization degree of PVA: the addition of MgCl2 could increase the thermal stability of PVA with low polymerization degree. In the case of PVA with high polymerization degree, the effect of MgCl2 on the thermal stability of PVA with high polymerization degree was complicated, because the ester groups showed an uneven distribution resulting in various alcoholysis degree on PVA molecules.
Dynamic mechanical properties
DMA was used to study the tan
δ and storage modulus (G′) of PVA1799 and PVA1788. tan
δ is a damping term defined as the ration of energy dissipated as heat to the maximum energy stored in the materials. It is an index of the material viscoelasticity. It could be seen from Fig. 7 that the tan
δ values of PVA increased as the MgCl2 content increased from 4 wt% to 10 wt%. This indicated that with the increasing content of MgCl2, the viscoelasticity of PVA increased. The size of the tan
δ peak is believed to relate to the volume fraction of the material undergoing the transition. It could be found that the peak size of tan
δ increased with the increasing content of MgCl2. This was because the destroy of MgCl2 on the crystals would increase the amorphous fraction and the relaxation time of chain segments. The peak temperature of the tan
δ curve is determined as the Tg of the polymer material. It could be found that the Tg of PVA increased with the addition of MgCl2. This is probably because that the Mg2+ could crosslink with the –OH of PVA, which limited the chain movement of PVA resulting in the improvement of Tg.
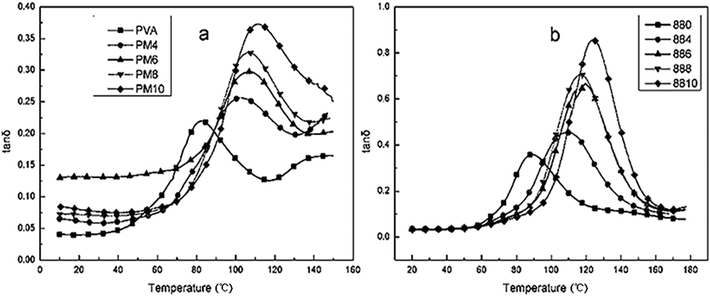 |
| Fig. 7 Plots of tan δ against temperature of PVA1799 (a) and PVA1788 (b) with various contents of MgCl2·6H2O. | |
G′ is a measurement of the rigidity of the material. Fig. 8 showed the plots of G′ versus temperature of PVA1799 and PVA1788. The G′ of PVA1799 and PVA1788 decreased as the temperature increased. This was because increasing temperature could destroy the intermolecular and intramolecular hydrogen bonds of PVA and crosslinking between MgCl2 and PVA resulting in the decrease of chain rigidity. Moreover, it could be seen from Fig. 8(a) that the descending speed of pure PVA1799 was higher than that of PVA1799 modified with MgCl2. When the temperature was low, G′ of pure PVA was higher than that of PM, but it became the opposite as the temperature increased. This might be due to the high alcoholysis degree and high regularity arise from high density hydrogen bonds. PVA1799 had higher crystallinity and thus crystallinity was the main factor to influence G′. However, crystal of PM was destroyed by MgCl2. As the temperature increased, crystal of pure PVA was destroyed, but there was crosslink between Mg2+ and PVA in MgCl2 modified PVA1799 resulting in high thermo-mechanical stability. For PVA1788 with low alcoholysis degree and low crystallinity, crystallinity had little influence on G′. Thus, the crosslink between MgCl2 and PVA1788 was the main influence factors on G′. Thence, G′ of PVA1788 was greater than or equal to that of pure PVA1788.
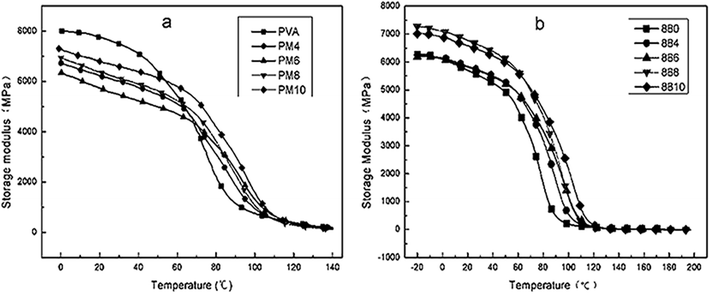 |
| Fig. 8 Plots of storage modulus E′ against temperature of PVA1799 (a) and PVA1788 (b) with various contents of MgCl2·6H2O. | |
Mechanical properties
The influence of MgCl2·6H2O on the mechanical properties of PVA1799 and PVA1788 was evaluated by tensile testing. Fig. 9 showed the measured values of tensile strength and elongation at break of PVA1799 and PVA1788 before and after modified with various content of MgCl2. After the addition of MgCl2, the tensile strength increased, while the elongation at break decreased. This proved that MgCl2 was an effective strengthening agent, which could improve the strength and rigidity of PVA. The strengthening effect of MgCl2 on PVA could be evaluated by the slope of curves in Fig. 9(a). With the increasing content of MgCl2, the slope of PVA1799 decreased while the slope of PVA1788 increased. This indicated that the strengthening effect of MgCl2 on PVA1788 was higher than that of PVA1799. This is because that the high crystallinity played a major role in increasing the tensile strength of PVA1799. MgCl2 could destroy the crystal but crosslink with PVA. As a result, the strengthening effect of MgCl2 on PVA1799 decreased with the increasing content of MgCl2. While the content of MgCl2 reached 8%wt, the tensile strength did not increase any more. For PVA1788 with low crystallinity, the crosslink between MgCl2 and PVA was the key factor to increase strength. Thus, the slope of PVA1788 increased as the dosage of MgCl2 increased.
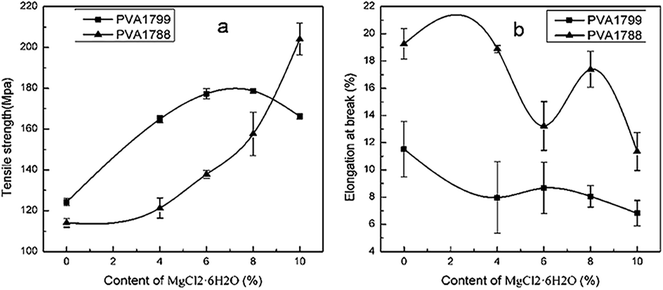 |
| Fig. 9 The tensile strength (a) and elongation at break (b) of PVA1799 and PVA1788 with various contents of MgCl2·6H2O. | |
Water sorption properties
Due to the lots of –OH, PVA is hydrophilic. However, the compact crystal would prevent H2O from penetrating.4 As a result, the water absorption content of PVA depended on the rate of amorphous region. Fig. 10 showed the water absorption rate of PVA 1799 and PM10 at RH of 54%. As shown in Fig. 10, the water content in PM10 was always higher than that of pure PVA, and the difference became larger with the time going. This proved that MgCl2 could destroy the crystal of PVA and increased the amorphous fraction resulting in the high water content in PM10. Meanwhile, the hydrophilicity of MgCl2 could also increase the water content of PM10. As a result, the water sorption of PM was higher than that of PVA.
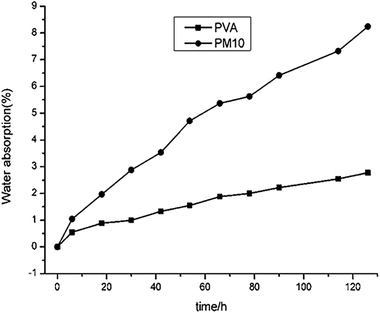 |
| Fig. 10 The water absorption of PVA and PM10. | |
The effect of water on dynamic mechanical properties of PVA1799 modified with MgCl2
MgCl2 could increase the water sorption of PVA. However, there were few studies on the effect of water on the modification of MgCl2 on PVA. Thence, the role of water in the modification was studied.
Fig. 11 showed the plots of storage modulus (G′) and tan
δ versus temperature of PVA1799 with 0, 4%, 12% content of water. It could be seen from Fig. 11(a) that Tg of PVA decreased with the increasing content of water. While with 12% content of water, Tg decreased from 82 °C of fully dried PVA to 28 °C. In addition, increasing water content could also decreased G′ of PVA. This proved that water was an efficient plasticizer of PVA.
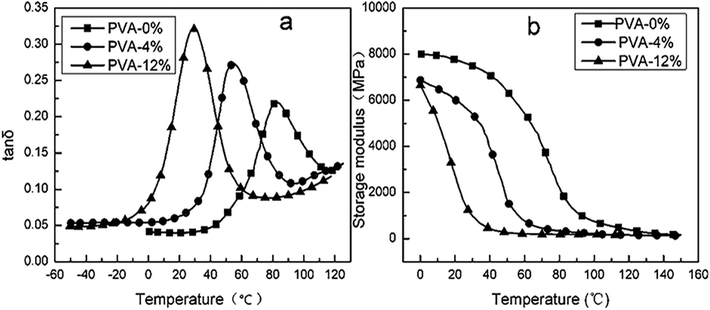 |
| Fig. 11 Plots of tan δ and storage modulus G′ against temperature of PVA with 0, 4%, 12% content of water. | |
The effect of MgCl2·6H2O and water on the dynamic mechanical properties of PVA were studied, respectively. As shown in Fig. 12, the Tg of PVA modified with MgCl2 was higher than that of PVA modified with the same content water. Meanwhile, G′ of PM was lower than PVA modified with water at low temperature, but it reversed as the temperature increased. This was because that crystallinity was the major factor affecting G′ when it was at lower temperature. The addition of MgCl2 would destroy the crystal of PVA. As a result, the storage modulus of PM was lower than PVA modified with water. With the increasing temperature, the crystal was destroyed and thus crystallinity had lower difference on the storage modulus. Thence, the crosslink between Mg2+ and PVA took an increasing impact on G′. Therefore, G′ of PM was higher than PVA modified with water as the temperature increased.
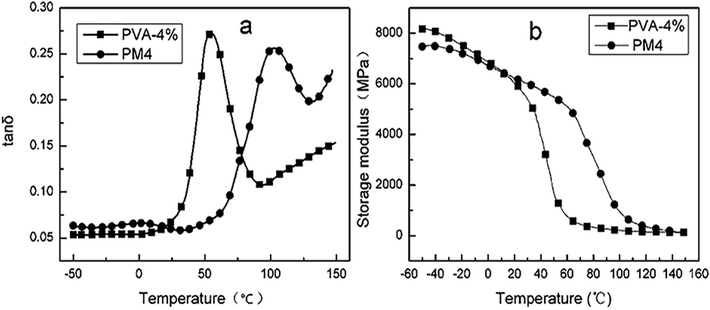 |
| Fig. 12 Plots of tan δ and storage modulus G′ against temperature of PVA with 4% content of water and MgCl2·6H2O, respectively. | |
It could be seen from Fig. 13 that increasing dosage of MgCl2 could increase Tg of PVA in the presence of water. This indicated that MgCl2 still could crosslink with PVA. Table 3 showed the difference of the Tg between PM and PVA with the same content of water. Increasing water content decreased the difference with any content of MgCl2. This indicated that water could destroy the crosslink between MgCl2 and PVA. Oxygen atom of H2O could interact with Mg2+, which would hinder the crosslink between Mg2+ and PVA resulting in the increase of molecular segmental mobility. The way of water damaging the interaction between PVA and MgCl2 was shown as Fig. 14 With the 12% content water, both the difference between PM4 and PVA, PM6 and PVA were negative. This proved that the crosslink had been destroyed by water completely. In addition, MgCl2 existed as hydrated MgCl2 in the system which had larger size and could increase the free volume of PVA. As a result, both the Tg of PM4 and PM6 were lower than that of pure PVA.
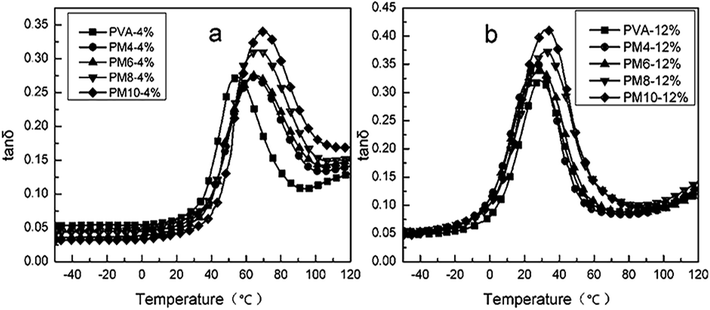 |
| Fig. 13 Plots of tan δ against temperature of PM with 4% (a) and 12% (b) water. | |
Table 3 The difference of Tg values between PVA and PM with various content of water
The difference of Tg |
PM4 minus PVA |
PM6 minus PVA |
PM8 minus PVA |
PM10 minus PVA |
0% |
20.7 °C |
25.09 °C |
25.07 °C |
29.17 °C |
4% |
10.48 °C |
10.77 °C |
12.65 °C |
15.53 °C |
12% |
−1.96 °C |
−0.81 °C |
2.94 °C |
3.16 °C |
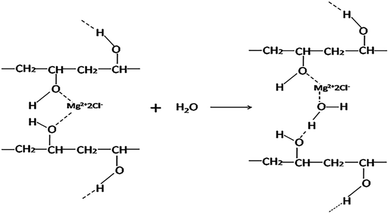 |
| Fig. 14 The way of water damaging the interaction between PVA and MgCl2·6H2O. | |
The effect of MgCl2 on mechanical properties of PVA1799 in the presence of water
The effect of the dosage of MgCl2 on the tensile strength and elongation at break of PVA in 12% water content was shown in Fig. 15. It could be seen from Fig. 15(a) and 9(a) that the tensile strength of PVA modified with MgCl2 was 50 MPa or so, but it could be over 120 MPa after sufficient drying. This proved that water played a significant role in decreasing the strength of PVA modified with MgCl2. In addition, with the increasing MgCl2 content, the tensile strength of PVA increased, but the elongation at break decreased. This indicated that MgCl2 still could crosslink with PVA in the presence of water. However, the mechanical properties in Fig. 15 varied more slightly than that of PM after sufficient drying. This was might because that water could destroy the crosslink between MgCl2 and PVA resulting in the decrease of the enhancement of MgCl2 on PVA.
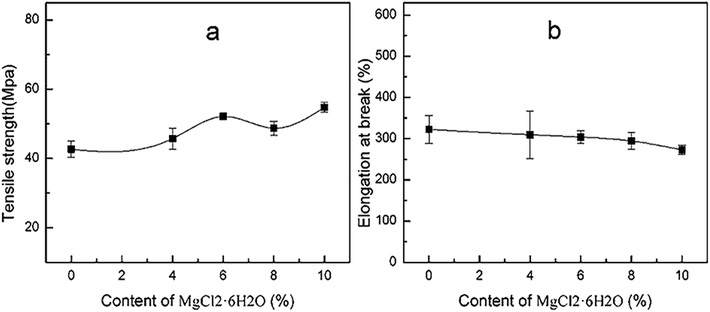 |
| Fig. 15 The tensile strength (a) and elongation (b) at break of PVA with various contents of MgCl2·6H2O in certain water content (12%). | |
4 Conclusion
The various PVA films modified with MgCl2·6H2O were prepared and the effect of MgCl2·6H2O on PVA with different alcoholysis degree and polymerization degree were investigated. The modification mechanism of MgCl2 on PVA and the effect of water in this system were revealed. Basically, MgCl2 was an effective strengthening agent, which could crosslink with PVA and then effectively destroy the crystals of PVA. With the addition of MgCl2, the strength and rigidity of PVA increased significantly and the melting temperature decreased. The tensile strength of PVA films modified with MgCl2 were all over 120 MPa. The effect of MgCl2 on the thermal stability of PVA was related to the alcoholysis degree of PVA. MgCl2 could decrease the thermal stability of PVA with lower alcoholysis degree. In the case of high alcoholysis PVA, the effect was related to the polymerization degree of PVA. MgCl2 could increase the thermal stability of PVA with low polymerization degree. In addition, water could interact with MgCl2 to destroy the crosslink between MgCl2 and PVA resulting in the decrease of the enhancement of MgCl2 on PVA. Taken together, the above studies revealed that MgCl2 could interact with PVA to increase the strength and rigidity of PVA and could increase the water sorption properties of PVA; however, the presence of water could decrease the modification effect. The further cognize on the way of MgCl2 influencing PVA could do help in revealing the modification mechanism of other inorganic salts on PVA and preparing the materials with more excellent properties.
Conflicts of interest
The authors declare no conflict of interest.
Acknowledgements
This research was supported by the National Natural Science Foundation of China (No. 51273129) and Applied Basic Research Project of Sichuan Province (No. 2017JY0247).
References
- R. M. Hodge, T. J. Bastow, G. H. Edward, G. P. Simon and A. J. Hill, Macromolecules, 1996, 29, 8137–8143 CrossRef CAS.
- J. Jang and D. K. Lee, Polymer, 2003, 44, 8139–8146 CrossRef CAS.
- R. Gref, Z. H. Ping and Q. T. Nguyen, et al., Polymer, 1995, 36, 1655–1661 CrossRef.
- G. J. Pritchard, Poly(vinyl alcohol): basic properties and uses, Macdonald Technical & Scientific, 1970 Search PubMed.
- W. Q. Wu, H. F. Tian and A. Xiang, J. Polym. Environ., 2012, 20, 63–69 CrossRef CAS.
- H. M. Zidan, Polym. Test., 1999, 18, 449–461 CrossRef CAS.
- H. M. Zidan, J. Appl. Polym. Sci., 2003, 88, 516–521 CrossRef CAS.
- Z. Peng and D. Chen, J. Polym. Sci., Part B: Polym. Phys., 2006, 44, 534–540 CrossRef CAS.
- G. Vijaya Kumar and R. Chandramani, Appl. Surf. Sci., 2009, 255, 7047–7050 CrossRef.
- R. Singh and S. G. Kulkarni, Int. J. Polym. Mater. Polym. Biomater., 2013, 62, 351–357 CrossRef CAS.
- J. I. Kubo, N. Rahman, N. Takahashi, T. Kawai, G. Matsuba, K. Nishida, T. Kanaya and M. Yamamoto, J. Appl. Polym. Sci., 2009, 112, 1647–1652 CrossRef CAS.
- X. C. Jiang, X. F. Zhang, D. Z. Ye, X. Zhang and D. Hua, Polym. Eng. Sci., 2012, 52, 1565–1570 CrossRef CAS.
- R. M. Hodge, G. H. Edward and G. P. Simon, Polymer, 1996, 37, 1371–1376 CrossRef CAS.
- G. Mann, Acta Polym., 1986, 37, 744–745 CrossRef.
- R. Iwamoto, M. Miya and S. Mima, J. Polym. Sci., Part B: Polym. Phys., 1979, 17, 1507–1515 CAS.
- R. M. Hodge, T. J. Bastow and G. H. Edward, et al., Macromolecules, 1996, 29, 8137–8143 CrossRef CAS.
- R. Wang, Q. Wang and L. Li, Polym. Int., 2003, 52, 1820–1826 CrossRef CAS.
- S. J. Peng, S. X. Liu, Y. J. Sun, N. P. Xiang, X. C. Jiang and L. X. Hou, Eur. Polym. J., 2018, 106, 206–213 CrossRef CAS.
- C. A. Finch, Polyvinyl alcohol: properties and applications, Wiley London, 1973 Search PubMed.
- P. D. Garrett and D. T. Grubb, J. Polym. Sci., Part B: Polym. Phys., 1988, 26, 2509–2523 CrossRef CAS.
- Y. Tsuchiya and K. Sumi, J. Polym. Sci., Part A-1: Polym. Chem., 1969, 7, 3151–3158 CrossRef CAS.
- P. Thomas, J.-P. Guerbois and G. Russell, et al., J. Therm. Anal. Calorim., 2001, 64, 501–508 CrossRef CAS.
|
This journal is © The Royal Society of Chemistry 2019 |
Click here to see how this site uses Cookies. View our privacy policy here.