DOI:
10.1039/C9RA01623F
(Paper)
RSC Adv., 2019,
9, 24822-24832
Retracted Article: Structural characterization and anti-inflammatory potency of Mesobuthus martensii Karsch oligopeptides in lipopolysaccharide (LPS)-induced RAW264.7 macrophages†
Received
4th March 2019
, Accepted 27th June 2019
First published on 9th August 2019
Abstract
Scorpion venom represents a significant source of bio-active peptides. However, the anti-inflammatory potency of scorpion venom oligopeptides (CMOs) has not been well explored. In the current study, thirty-five CMOs were isolated, the amino acid sequences were identified, and the anti-inflammatory potency was further explored in lipopolysaccharide (LPS)-induced RAW264.7 macrophages. The results showed that CMO-1 (His-Tyr-Gly-His) demonstrated the best anti-inflammatory potency by attenuating inflammatory cytokine (NO, TNF-α, IL-6, and IL-1β) production. CMO-1 also inhibited IκBα degradation and p65 nuclear translocation and suppressed NF-κB activation. Moreover, CMO-1 inhibited the phosphorylation of ERK, JNK, and p38 MAPKs. It is worth noting that CMO-1 exhibited anti-inflammatory potency; thus, it is a potential anti-inflammatory agent.
1 Introduction
Inflammation may be induced by physical factors, noxious chemical stimuli or microbiological toxins and plays decisive roles in inflammatory diseases.1 Epidemiological studies indicate that inflammation presents a fatal risk in various diseases such as cardiovascular damage, diabetes and cancers.2,3 Immune cells play a significant role in the progress of anti-inflammation. During this process, inflammatory mediators such as interleukin (IL)-1β, tumor necrosis factor (TNF)-α and nitric oxide (NO) are produced; meanwhile, their overproduction may lead to fatal damage to the organs.4 NO, which plays a major role in inflammation, is controlled by NO syntheses (NOS). Therefore, the inhibition of these inflammatory mediators has been considered as an effective strategy against inflammatory diseases.5
Nuclear factor kappa-B (NF-κB) plays a remarkable role in regulating the inflammatory responses by increasing the expressions of inflammatory mediators and pro-inflammatory cytokines. It remains in an inactive form with the linkage to the inhibitor of κB (IκB) protein normally, while it is released through the phosphorylation and degradation of IκB when induced by inflammatory stimuli such as LPS and cytokines and the fatal elements of oxidative stress, namely, reactive oxygen species (ROS).6 Furthermore, the generation of pro-inflammatory cytokines or the progression of inflammation is commonly observed with ROS overproduction.
Bio-active peptides are the significant compounds in the areas of life and food science. The antimicrobial activity, anti-hypertensive effect, anti-viral activity and anti-inflammatory activity of peptides have been widely explored over the past years. Previous studies have successfully identified that the traditional Chinese medicines contain a significant diversity of peptides, and various functional peptides have been successfully utilized in inflammatory disease treatments. For example, ghrelin is a novel growth hormone-releasing peptide with potential endogenous anti-inflammatory activities that ameliorate some pathological inflammatory conditions. Egg transferrin (IRW) shows an excellent anti-epithelial inflammatory response and anti-oxidant stress effects. Thymopentin exhibits excellent activity in inhibiting the proliferation, spread and metastasis of cancer cells.7,8,18,23
The Chinese scorpion venom is a famous traditional Chinese medicine that has been widely used to treat paralysis, apoplexy, epilepsy and inflammation.9 Studies have reported that the scorpion venom peptides show a diverse array of biological activities, which are involved in immune, nervous, cardiovascular, and neoplastic diseases. Due to their structural and functional diversities, it has been proposed that scorpion-derived peptides can be used to develop new specific drugs.10 Studies have mentioned that numerous bradykinin-potentiating peptides extracted from a variety of scorpions display excellent anti-hypertensive potency in the treatment of cardiovascular diseases.11 Moreover, the γ-Ts toxin accomplishes its immune regulatory effect through the release of pro- and anti-inflammatory factors, due to which the scorpion venom has the potential to be developed for treating inflammation-related diseases.12 Rheumatoid arthritis is an autoimmune disease that causes chronic inflammation of joints. Of interest, the scorpion (Mesobuthus eupeus) venom is also used as an anti-arthritis agent in the animal model of acute inflammation.13 Scorpion venom represents a library containing a great diversity of bio-active peptides. However, the anti-inflammatory potency of scorpion peptides has not been well elucidated. Therefore, we isolated the scorpion peptides and explored the anti-inflammatory potency and underlying mechanism in LPS-induced RAW264.7 macrophages.
2 Materials and methods
2.1 Materials and cell culture
The Chinese scorpion Mesobuthus martensii Karsch was acquired from Ertiantang pharmacy (Guangzhou, China) and identified by Professor Zhou (Jinan University, Guangzhou, China). Enzyme-linked immunosorbent assay (ELISA) kits for NO, TNF-α, IL-6, and IL-1β were purchased from the Beyotime Institute of Biotechnology (Beyotime, China). RAW264.7 macrophages were purchased from the Cell Bank of the Chinese Academy of Science (Shanghai, China) and were cultured in DMEM with high concentrations of glucose, glutamine and sodium pyruvate, 10% fetal bovine serum and 1% penicillin–streptomycin antibiotics. Cells were maintained in a cell incubator at 37 °C with 5% CO2 and the medium was changed every 2 days. All the antibodies were purchased from Cell Signaling Technology (CST, USA). The other chemicals were purchased from Aldrich or Admas without any further purification.
2.2 Isolation and amino acid sequence identification of the CMOs
2.2.2 Ultrafiltration and hydrophobic chromatography. The total protein was fractionated using ultrafiltration with 1 kDa molecular weight (MW) cut-off membranes (Millipore, Hangzhou, China). Two fractions, i.e., CMO-A (MW < 1 kDa) and CMO-B (MW > 1 kDa) were collected and freeze-dried.Hydrophobic Chromatography: CMO-A was dissolved in 1.40 M (NH4)2SO4 prepared with 30 mM phosphate buffer (pH 7.2) and loaded onto a phenyl sepharose CL-4B hydrophobic chromatography column (2.5 cm × 110 cm), which was previously equilibrated. A stepwise elution was carried out with decreasing concentrations of (NH4)2SO4 (1.40, 0.80 and 0 M) dissolved in 30 mM phosphate buffer (pH 7.2) at a flow rate of 2.0 mL min−1. Each fraction was collected at a volume of 100 mL and monitored at 280 nm. Ten fractions were freeze-dried and the anti-inflammatory activity was detected. The fraction having the strongest anti-inflammatory activity was collected and prepared for anion-exchange chromatography.
2.2.3 Anion-exchange chromatography. The CMO-A-4 solution (5.0 mL, 45.8 mg mL−1) was loaded onto a DEAE-52 cellulose (Yuanju, Shanghai, China) anion-exchange column (2.4 × 150 cm) pre-equilibrated with deionized water and was eluted stepwise with 1000 mL distilled water as well as 0.10, 0.70, and 1.40 M (NH4)2SO4 at a flow rate of 2.0 mL min−1. Each eluted fraction (100 mL) was collected and detected at 280 nm. Ten fractions (CMO-A-4-1 to CMO-A-4-10) were freeze-dried and the anti-inflammatory activity was detected. The fraction having the strongest anti-inflammatory activity was collected and prepared for gel filtration chromatography.
2.2.4 Gel filtration chromatography. The CMO-A-4-5 solution (3.50 mL, 10.1 mg mL−1) was fractionated on a Sephadex G-25 (Sigma-Aldrich, Shanghai, China) column (2.2 × 100 cm) at a flow rate of 2.0 mL min−1. Each eluate (50 mL) was collected and monitored at 280 nm, and four fractions (CMO-A-4-5-1 to CMO-A-4-5-4) were freeze-dried and the anti-inflammatory activity was detected. The fraction having the strongest anti-inflammatory activity was collected and used for reversed-phase high-performance liquid chromatography (RP-HPLC).
2.2.5 CMO isolation by RP-HPLC. CMO-A-4-5-2 was finally separated by RP-HPLC (Agilent 1200 HPLC) on a Zorbax, SB C-18 column (4.6 × 250 mm, 5 μm). The elution solvent system was composed of water–trifluoroacetic acid (solvent A; 100
:
0.1, v/v) and acetonitrile–trifluoroacetic acid (solvent B; 100
:
0.1, v/v). The peptide was separated using a gradient elution from 30% to 65% of solvent B for 40 min at a flow rate of 1.0 mL min−1. The detection wavelength was set at 280 nm and the column temperature was 4 °C.
2.2.6 Amino acid sequence analysis and molecular mass determination by HPLC-ESI-MS. HPLC-ESI-MS was carried out on a SCIEX X500R Q-TOF mass spectrometer (Framingham, USA), and the MS conditions were as follows: ESI-MS analysis was performed using a SCIEX X500R Q-TOF mass spectrometer equipped with an ESI source. The mass range was set at m/z 100–1500. The Q-TOF MS data were acquired in the positive mode and the conditions of MS analysis were as follows: CAD gas flow-rate, 7 L min−1; drying gas temperature, 550 °C; ion spray voltage, 5500 V; declustering potential, 80 V. Software generated data file: SCIEX OS 1.0.
2.3 Cytotoxicity assay
To avoid cytotoxicity, suitable concentrations of CMOs were detected. RAW264.7 cells were plated in 96-well plates (Corning, USA) at densities of 1 × 105 cells per milliliter. Cells were treated with 10 μL of fresh FBS-free DMEM (control group) or FBS-free DMEM containing different concentrations (0, 1, 2, 4, 8, 16, 32, 64, and 128 μM) of CMOs for 24 h. Cell viability assays were conducted using the CCK-8 (Cell Counting Kit-8) method.14 Cell viability was detected relative to the control group.
2.4 NO, TNF-α, IL-6, and IL-1β assays
Briefly, the RAW264.7 cells were cultured in a 6-well plate at a density of 5 × 106 cells per mL and induced with 1 μg mL−1 LPS for 12 h. Then, the CMO-1 (4.0 and 20.0 μM) treatments were performed for 24 h. After that, the culture media was collected and the NO, TNF-α, IL-6, and IL-1β concentrations were detected with the ELISA kits.
2.5 Reactive oxygen species (ROS) and mitochondrial membrane potential (Δψm) assays
The generation of intracellular ROS has been associated with chemical toxicity and inflammation, cancer and ageing. Therefore, the ability to assess the generation of intracellular ROS as a component of the pathways leading to toxicity and diseases is critically important. Numerous studies demonstrate the utility of DCFH as an index of free radical reactions that take place in the living animals following exposure to toxic chemicals.15 Thus, the intracellular ROS level was measured with a DCFH-DA assay kit.
After the cells were treated according to the experimental design, the cells were washed thrice with a serum-free medium. Afterwards, they were incubated in 200 μL serum-free medium containing DCFH-DA (25 μM) for 30 min at room temperature. The cells were then washed with the serum-free medium and collected and assayed by flow cytometric analysis (BD FACS Calibur, Franklin Lakes, CA, USA).
The Δψm decline of RAW264.7 cells was detected by JC-1 staining (Beyotime, China). Briefly, 2 mL of 1 × 106/mL cells were treated according to experimental design in 6-well plates. The cells were washed three times with cold PBS and incubated with 1 μg mL−1 of JC-1 at 37 °C for 30 min without light. The cells were collected and washed three times with cold PBS and then assayed by flow cytometry (FACScan, CA).
2.6 Apoptosis and cell cycle assays
Apoptosis or programmed cell death is a physiological process by which cells are removed from tissues in a controlled manner. The cells that undergo apoptosis show characteristic morphological changes, including condensation of the cell cytoplasm and nucleus, DNA fragmentation, dissociation of cell organelles, and disruption of the cell plasma membrane.15 In the current experiment, apoptosis was detected by Annexin V-fluorescein isothiocyanate (FITC) and propidium iodide (PI) labeling. Viable cells were negative for both PI and Annexin V-FITC; apoptotic cells were positive for Annexin V-FITC and negative for PI, whereas late apoptotic dead cells displayed strong Annexin V-FITC and PI labeling. Non-viable cells, which underwent necrosis, were positive for PI and negative for Annexin V-FITC.16
Briefly, 1.5 × 105 cells per mL were incubated with 1 μg mL−1 LPS for 12 h. After that, the cells were collected and co-cultured with CMO-1 (4.0 and 20.0 μM) for 24 h. Next, the cells were washed with cold PBS, and 5 μL Annexin V-FITC and 5 μL PI (PharMingen, San Diego, CA, USA) were applied for staining. The fluorescences of PI and Annexin-V-FITC were monitored at 630 nm and 525 nm, respectively. The cell status was analyzed using flow cytometry (BD FACS Calibur, Franklin Lakes, CA, USA).
As for the cell cycle analysis, RAW264.7 cells were plated on a 6-well plate with 2 × 105 cells per well and treated as described above. After that, cells were harvested and fixed in 70% ethanol and stored at −20 °C for 12 h. Then, the cells were washed with PBS and cell cycle distribution was detected by PI (propidium iodide) staining with flow cytometry (BD FACS Calibur, Franklin Lakes, CA, USA).
All the tests mentioned above were repeated at least 3 times.
2.7 Western blot
The RAW264.7 cells were treated according to the experimental design. After that, the cells were collected, washed with PBS, lysed in the lysis buffer containing protease and phosphatase inhibitor cocktails, and centrifuged at 1.3 × 104 g for 12 min. The soluble lysates (15 μL per lane) were subjected to 10% sodium dodecyl sulfate-polyacrylamide gel electrophoresis (SDS-PAGE), transferred onto nitrocellulose membranes, and blocked with 5% non-fat milk in Tris-buffered saline with Tween (TBST) for 2 h at room temperature. Membranes were incubated at 4 °C overnight with the primary antibody solution, diluted at 1
:
500 (v/v), specific for the detection. β-Actin protein was used for the measurement of the proteins. After washing with TBST, the membranes were incubated with horseradish peroxidase-conjugated anti-bodies for 1 h at room temperature.17 The protein bands were measured and β-actin was used as an internal standard of process control. Blot band densitometry was conducted with the Image J software.
2.8 Statistical analysis
The data were achieved from at least three independent experiments and were listed as means ± SD. The data were evaluated through analysis of variance (ANOVA), followed by Student's t-test. The values with *p < 0.05 were considered statistically significant. The analyses were performed with the Origin 7.0 software (Origin Lab Corporation, Northampton, MA, USA).
3 Results
3.1 Isolation and identification of CMOs
We successfully isolated thirty-five CMOs, and the structures were identified by HPLC-ESI-MS analysis. Peptides are usually protonated under ESI-MS/MS conditions, and fragmentation mostly occurs at the amide bonds because it is difficult to break the chemical bonds of the side chains at such low energy. Therefore, the b and y ions are the main fragment ions when the collision energy is <200 eV.18
CMO-1 was analyzed by HPLC-ESI-MS for molecular mass determination. The molecular mass of CMO-1 was determined to be 513.2182 Da. The ion fragment m/z 156.0760 was regarded as the y1 ion and proved to be [His + H]+, while m/z 213.0973 was regarded as the y2 ion and represented the [His-Gly + H]+ ion. The ion m/z 110.0698 was ascribed to the typical fragment [His-COOH]+; m/z 301.1286 was the b2 ion, m/z 376.1613 was the y3 ion, and m/z 273.1338 was the [His-Tyr-COOH]+ ion. On the basis of this, we concluded that the sequence of the peptide is HYGH (Fig. 1). The rest of the CMOs were identified as above and after the analysis of the MS/MS spectra, we successfully identified thirty-five CMOs; the amino acid sequences are listed in Table 1.
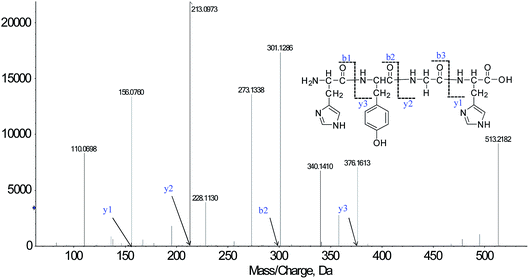 |
| Fig. 1 Structure and MS/MS spectra of CMO-1. | |
Table 1 Amino acid sequences and protective effect of CMOsa
CMOs |
Amino acid sequence |
Survival rate (%) |
IC50 values are shown as mean ± standard error of the mean (SD) from at least three independent experiments. |
CMO-1 |
HYGH |
91.6 ± 6.63 |
CMO-2 |
FRHALS |
76.2 ± 7.04 |
CMO-3 |
WAGHAYE |
84.2 ± 7.61 |
CMO-4 |
WARYQHG |
71.3 ± 7.12 |
CMO-5 |
HRWQGKH |
70.4 ± 6.29 |
CMO-6 |
HESGWL |
70.9 ± 5.91 |
CMO-7 |
KSHEFG |
78.6 ± 8.09 |
CMO-8 |
KYAVHS |
79.2 ± 6.58 |
CMO-9 |
TYKRHS |
77.4 ± 7.02 |
CMO-10 |
HKYRHD |
66.3 ± 6.37 |
CMO-11 |
FSHRGH |
74.3 ± 7.51 |
CMO-12 |
FHGKGHE |
85.9 ± 6.22 |
CMO-13 |
KYGHEHS |
74.1 ± 5.99 |
CMO-14 |
FDHGWK |
76.7 ± 6.82 |
CMO-15 |
HREWKGH |
74.3 ± 7.17 |
CMO-16 |
HGEHRVY |
76.7 ± 8.08 |
CMO-17 |
WEGHES |
74.5 ± 7.29 |
CMO-18 |
SHAYSH |
79.2 ± 7.20 |
CMO-19 |
GEYHSHE |
81.4 ± 8.15 |
CMO-20 |
EHGEYF |
73.5 ± 8.10 |
CMO-21 |
EGFHL |
76.1 ± 7.69 |
CMO-22 |
KHGEL |
81.0 ± 8.21 |
CMO-23 |
EGHGF |
71.9 ± 7.08 |
CMO-24 |
YEEGAH |
73.6 ± 6.52 |
CMO-25 |
AHEFEL |
83.7 ± 8.07 |
CMO-26 |
EAHGHSF |
76.2 ± 6.66 |
CMO-27 |
YSLHLHG |
76.6 ± 7.04 |
CMO-28 |
YESHGA |
76.3 ± 6.26 |
CMO-29 |
AHGHS |
76.5 ± 7.62 |
CMO-30 |
LHLH |
74.7 ± 7.04 |
CMO-31 |
GFHL |
69.2 ± 6.11 |
CMO-32 |
SHEFG |
79.3 ± 7.16 |
CMO-33 |
AVHS |
77.6 ± 7.63 |
CMO-34 |
TYKR |
76.1 ± 6.60 |
CMO-35 |
YHSHE |
81.8 ± 7.11 |
Control |
— |
100.0 ± 9.16 |
LPS |
— |
63.5 ± 6.31 |
3.2 CMOs attenuated the damage in RAW264.7 cells with LPS stimulation
Studies have reported that natural products can protect human umbilical vein endothelial cells against oxidative stress and reduce amyloid-β peptide-induced oxidative stress.19 Glutathione, a natural antioxidant active peptide, can inhibit free radicals, scavenge peroxides and inhibit the activity of melanogenesis.20 Antioxidant peptides DSGVT, IEAEGE, DAQEKLE, EELDNALN, and VPSIDDQEELM obtained from porcine myofibrillar proteins exhibit excellent antioxidant activities in a linolenic acid peroxidation system induced by Fe2+.21
First, the cytotoxicity of the CMOs was detected in RAW264.7 cells. The results indicated that all the CMOs showed no cytotoxicity in RAW264.7 cells up to 64 μM. We then investigated the protective effects of the CMOs under no cytotoxicity concentrations, and the LPS group was set as the proliferative group. Compared with the result for the control group, the RAW264.7 cell survival rate in the LPS group decreased by 36.5%, which indicated that severe damage was caused to the RAW264.7 cells. Therefore, further experiments were performed to investigate the protective effects of CMOs. Interestingly, most CMOs demonstrated protective activity and effectively elevated the survival under LPS stimulation. Table 1 shows that with the CMO treatments and compared with the survival rates for the LPS group, the survival rates in CMO-1, 3, 12, 18, 19, 25 and 32 groups increased by 28.1%, 20.7%, 22.4%, 15.7%, 17.9%, 20.2% and 18.3%, respectively. We inferred that the CMOs may improve the anti-inflammatory capacity to attenuate the damage caused by LPS. Hence, the protective mechanism was further explored with CMO-1.
3.3 CMO-1 decreased the ROS level and reversed the Δψm collapse
The overproduction of free radicals, such as ROS, or antioxidant capacity decline will lead to fatal damage to DNA and subsequently induce cell apoptosis. ROS play an important role in the oxidative process as oxidization molecules. The interaction of the cellular immune system with endogenous or exogenous inflammatory stimulation determines the generation of ROS. This can result in the hyper-activation of inflammatory responses, leading to tissue damage and oxidative stress.22 Therefore, intracellular ROS production can be used as an evaluating indicator for oxidative stress. Studies have revealed that atherosclerosis is associated with increased intracellular oxidative stress, and LPS is believed to play a fatal role in ROS-induced oxidative stress in macrophages.23
Natural products can protect RAW264.7 cells against oxidative stress-induced damage and reduce H2O2-induced oxidative stress.24 To investigate the ROS eliminating ability of CMO-1, the ROS level was detected, and the ROS level in the control group was set as 1. As shown in Fig. 2, the ROS level in the LPS group increases by a factor of 8.32. However, after 4.0 and 20.0 μM CMO-1 treatments and compared with the ROS levels of the LPS group, the ROS levels were down-regulated by 40.9% and 76.5%, respectively.
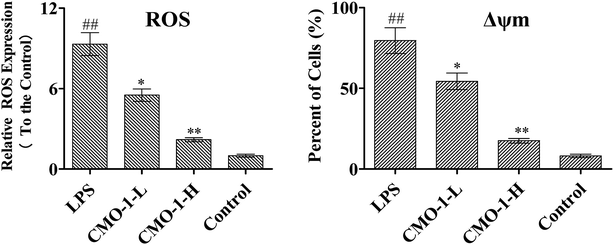 |
| Fig. 2 CMO-1 down-regulated the ROS level and attenuated the Δψm collapse in RAW264.7 cells. RAW264.7 cells were treated with 1 μg mL−1 of LPS for 12 h; then, the cells were treated with CMO-1 (4.0, and 20.0 μM) for 24 h. The intracellular ROS levels in the RAW264.7 cells were measured with the Reactive Oxygen Species assay kit. The changes in Δψm in the RAW264.7 cells were detected by JC-1 staining according to the manufacturer's protocol. The values are expressed as means ± SD of triplicate tests. The contents in the control groups were set as 1, and the values in the other groups were relative values to the control group. **p < 0.01, *p < 0.05, vs. LPS, ##p < 0.01, #p < 0.05, vs. control, indicate statistically significant difference. | |
Remarkably, LPS-induced ROS up-regulation was significantly attenuated when the cells were treated with CMO-1. Taken together, CMO-1 decreased the accumulation of intracellular ROS in RAW264.7 cells, and the results confirmed that CMO-1 could reduce the intracellular oxidative stress and protect the RAW264.7 cells from oxidative stress damage by scavenging ROS.
The Δψm balance and mitochondrial integrity are significant for the physiological function of cells. Once the concentration of ROS and oxygen stress reach a certain level, Δψm changes, resulting in the release of apoptosis factors.25,26 Here, the JC-1 fluorescent probe was used to detect Δψm.
As shown in Fig. 2, the RAW264.7 cells under LPS stimuli show a clearly decreased Δψm signal, which means that LPS caused Δψm decline. However, the CMO-1 treatment attenuated the Δψm decline, and we concluded that CMO-1 may have a protective effect by attenuating the mitochondrial damage induced by LPS.
3.4 Effect of CMO-1 on cell apoptosis and cell cycle arrest in LPS-induced RAW264.7 cells
Apoptosis is a form of programmed cell death that occurs in cellular organisms, whose biochemical events lead to characteristic cell morphology changes including cell shrinkage, nuclear fragmentation and chromatin condensation.27,28 Here, the cell apoptosis was detected with an Annexin V-FITC/PI assay using flow cytometry; the results are shown in Fig. 3. Compared with the results for the control group, the total apoptosis and necrosis in the LPS group increased by 18.2%. Compared with the LPS group, the CMO-1 treatments attenuated the total apoptosis and necrosis rates by 10.3% and 14.7%, respectively. Of interest, cell apoptosis provides visual evidence for the intracellular protective potency of CMO-1. On this basis, the protective potency and the underlying mechanism of CMO-1 were further explored.
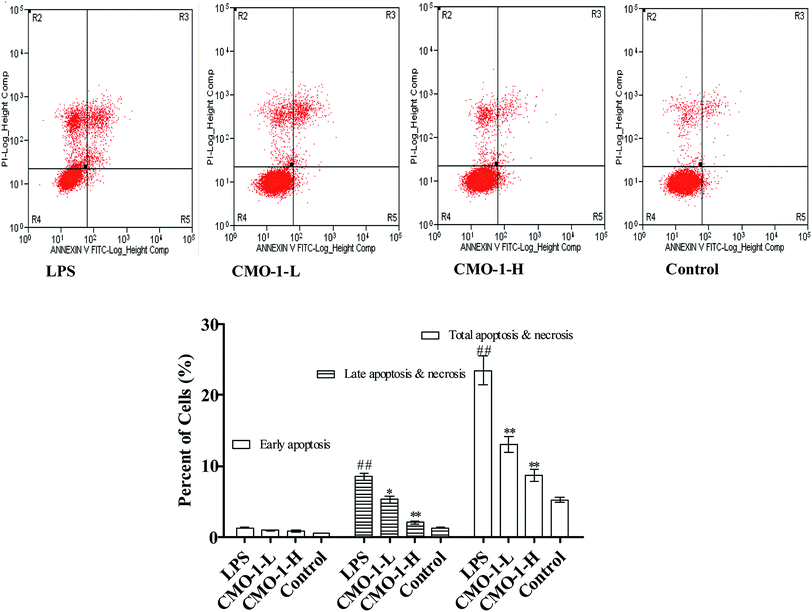 |
| Fig. 3 The protective potency of CMO-1 on LPS-induced apoptosis in RAW264.7 macrophages. Representative scatter diagrams. RAW264.7 cells treated without the addition of CMO-1 were set as the control group. The evaluation of apoptosis was via an Annexin V: FITC Apoptosis Detection Kit and flow cytometry. In each scatter diagram, the abscissa represents the fluorescence intensity of the cells dyed with Annexin V; the ordinate represents the fluorescence intensity of the cells dyed by PI. The lower left quadrant shows viable cells, the upper left quadrant shows necrotic cells, the lower right quadrant shows early apoptotic cells, and the upper right quadrant shows late apoptotic cells. The values are expressed as means ± SD of triplicate tests. **p < 0.01, *p < 0.05, vs. LPS, ##p < 0.01, #p < 0.05, vs. control, indicate statistically significant difference. | |
Of note, according to the content of DNA in cells, which can regulate the cell growth and replication, the cell cycle can be divided into three phases: G0/G1, S and G2/M.29,30 To further investigate the mechanism of cell cycle in LPS-induced damage, the cell cycle phase distributions of CMO-1 treated in RAW264.7 cells were analyzed; the profiles are given in Fig. 4. An increase of 12.1% in the S population was observed in cells with LPS stimulation. We inferred that the RAW264.7 cells were significantly blocked in the S phase. Interestingly, with the treatments of CMO-1, the S population decreased by 8.9% and 11.0%, respectively. It was concluded that the cell cycle arrest also contributed to the protective effect of CMO-1.
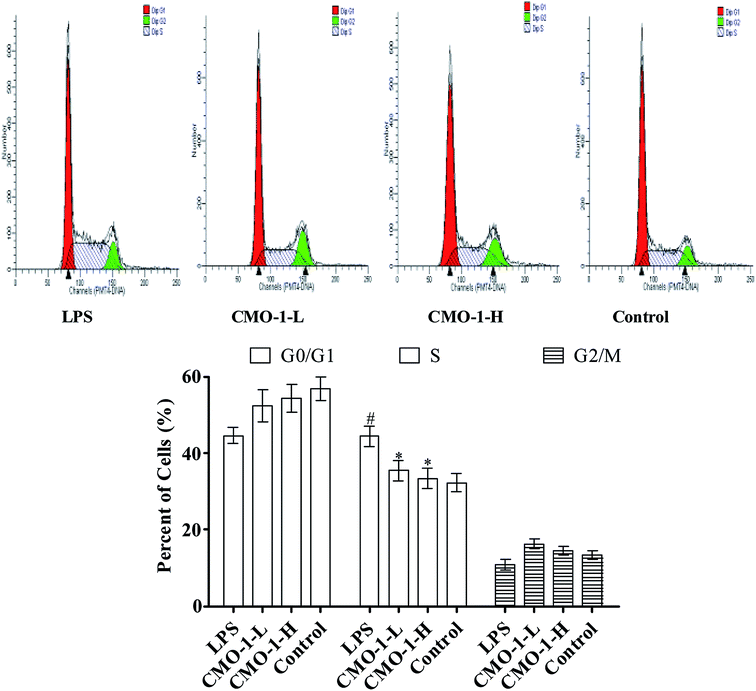 |
| Fig. 4 Cell cycle analysis of RAW264.7 cells exposed to CMO-1. The control group was treated without CMO-1. After the CMO-1 treatments, the cells were collected, fixed in 70% ethanol overnight, and stained with propidium iodide solution. G0/G1: quiescent state/growth phase; S: initiation of DNA replication; G2/M: bio-synthesis/mitosis phases. The values are expressed as means ± SD of triplicate tests. **p < 0.01, *p < 0.05, vs. LPS, ##p < 0.01, #p < 0.05, vs. control, indicate statistically significant difference. | |
3.5 Effect of CMO-1 on NO, TNF-α, IL-6, and IL-1β production and iNOS expression
NO is produced by nitric oxide synthase (NOS), which exists in three NOS isoforms: endothelial NOS (eNOS), neuronal NOS (nNOS), and inducible NOS (iNOS). Studies have reported that the macrophages produce NO by up-regulating the iNOS expression through mitogen-activated protein kinases (MAPKs) and NF-κB signaling pathways.31,32 To investigate whether CMO-1 possesses anti-inflammatory and anti-oxidant potencies in LPS-induced RAW264.7 cells, we detected the NO levels in the culture media with the NO assay kit.
Compared with the control group, LPS significantly increased the NO level by 7.31-fold (Fig. 5, p < 0.01). Of interest, the NO level was significantly attenuated in the CMO-1-treated groups. With the CMO-1 treatments, the NO levels decreased by 38.4% and 76.8%. To further explore the underlying mechanism of NO down-regulation by CMO-1, the iNOS expression was explored by Western blot. As shown in Fig. 6, LPS significantly increases the iNOS expression. However, the iNOS expression was down-regulated by the CMO-1 treatment, which suggested that CMO-1 may decrease the NO production by inhibiting the expression of iNOS.
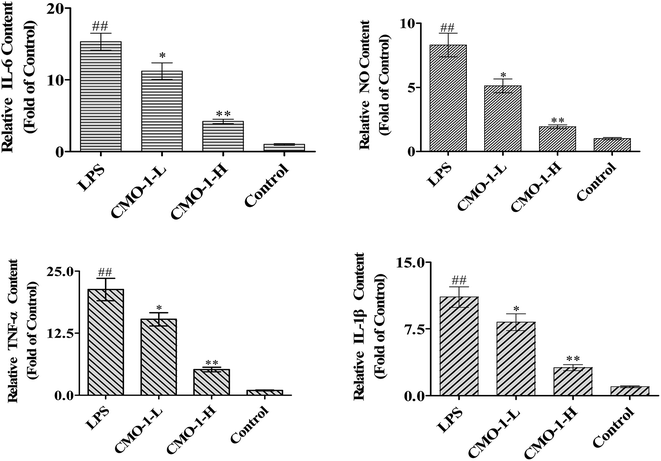 |
| Fig. 5 Effect of CMO-1 on IL-6, NO, TNF-α, IL-1β production. RAW264.7 cells were stimulated with 1 μg mL−1 of LPS for 12 h; then, the cells were treated with CMO-1 (4.0, and 20.0 μM) for 24 h. Afterwards, the culture media were collected and the NO, TNF-α, IL-6, and IL-1β concentrations were measured with the appropriate kits according to the manufacturer's instructions. The contents in the control groups were set as 1, and the values in the other groups were relative values to the control group. The values are expressed as means ± SD of triplicate tests. **p < 0.01, *p < 0.05, vs. LPS, ##p < 0.01, #p < 0.05, vs. control, indicate statistically significant difference. | |
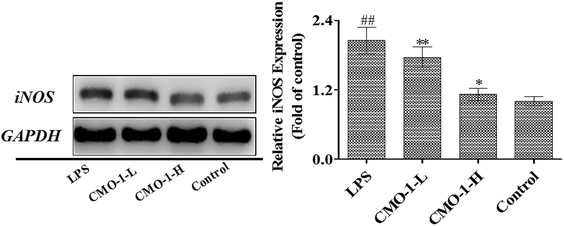 |
| Fig. 6 CMO-1 attenuated LPS-induced iNOS expression. RAW264.7 cells were stimulated with 1 μg mL−1 of LPS for 12 h; then, the cells were treated with CMO-1 (0, 4.0, and 20.0 μM) for 24 h. The total protein lysate of each RAW264.7 cell was prepared, and cell-signaling proteins were detected by Western blot analysis. The values are expressed as means ± SD of triplicate tests. The iNOS expression in the control group was set as 1, and the values in the other groups were relative values to the control group. **p < 0.01, *p < 0.05, vs. LPS, ##p < 0.01, #p < 0.05, vs. control, indicate statistically significant difference. β-Actin was used as an internal standard of process control and blot band densitometry was analyzed with Image J software. | |
It is known that IL-1β, TNF-α and IL-6 are widely responsible for autoimmune diseases. Furthermore, excess cytokine and iNOS-mediated NO production has been linked to many pathophysiological conditions such as inflammation and tumorigenesis.33,34 To determine the protective potency of CMO-1 on the production of pro-inflammatory cytokines, the TNF-α, IL-6, and IL-1β levels in the culture media were measured using appropriate assay kits.
Compared with the results for control groups, the TNF-α, IL-6, and IL-1β levels markedly increased by 20.3, 14.5 and 10.1-fold, respectively (Fig. 5). However, the CMO-1 treatments down-regulated the LPS-induced TNF-α, IL-6 and IL-1β generation by 75.6% (p < 0.01), 72.4% (p < 0.01), and 71.6% (p < 0.01) at 20.0 μM, respectively. We inferred that CMO-1 may demonstrate anti-inflammatory potency by inhibiting iNOS-mediated NO production as well as by attenuating the TNF-α, IL-6, and IL-1β production.
3.6 Effect of CMO-1 on the NF-κB pathway
Studies have reported that NF-κB is chronically activated in many inflammatory diseases. NF-κB is also known as the transcription factor for pro-inflammatory mediators such as iNOS, TNF-α, and IL-1β, controlling many genes involved in inflammation.35
Compared with the LPS group, CMO-1 decreased the phosphorylation of IκBα protein expression by 51.9% at a concentration of 20.0 μM (p < 0.05, Fig. 7). Furthermore, CMO-1 at 20.0 μM also significantly inhibited the LPS-induced phosphorylation of IKKα expression by 56.4%. Furthermore, the treatment with 20.0 μM CMO-1 also obviously suppressed the phosphorylation of p65 and NF-κB.
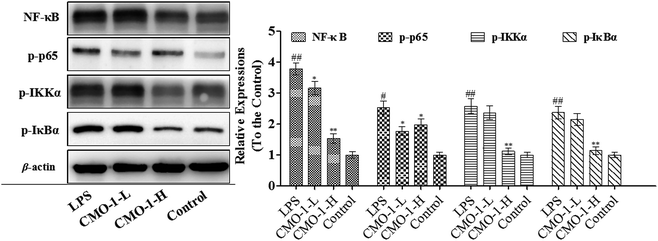 |
| Fig. 7 Effect of CMO-1 on the NF-κB signaling pathways in RAW 264.7 cells. RAW 264.7 cells were stimulated with 1 μg mL−1 of LPS for 12 h and then treated with CMO-1 (4.0, and 20.0 μM) for additional 24 h. After that, the total protein lysate of each treatment group was prepared, and cell-signaling proteins were detected by Western blot analysis. The values are expressed as means ± SD of triplicate tests. The protein expressions of the control groups were set as 1, and the values in the other groups were relative values to the control group. **p < 0.01, *p < 0.05, vs. LPS, ##p < 0.01, #p < 0.05, vs. control, indicate statistically significant difference. β-Actin was used as an internal standard of process control and blot band densitometry was analyzed with Image J software. | |
3.7 Effect of CMO-1 on MAPKs
We know that MAPK is a special serine/threonine kinase that plays an important role in transducing extracellular stimulus signals to cells as well as their nuclei and causing cellular biological reactions, such as apoptosis, involving extracellular signal-regulated kinase 1/2 (ERK1/2), p38 and c-Jun NH2 terminal kinase (JNK). Similar to NF-κB, the MAPK signaling pathway is associated with the activation of the iNOS expression and the regulation of inflammatory mediators in macrophages induced by LPS.36 To further investigate the anti-inflammatory mechanism of CMO-1 against LPS stimulation, we investigated the phosphorylation of ERK, JNK, and p38 MAPK.
As presented in Fig. 8, LPS significantly increases the expressions of the phosphorylation of ERK, JNK, and p38; meanwhile, CMO-1 down-regulated the expressions of the phosphorylation of ERK, JNK, and p38. With 20.0 μM CMO-1 treatment, the expressions of ERK, JNK and p38 phosphorylation were down-regulated by 35.8%, 62.3% and 31.7%, respectively. Of note, the protective potency of CMO-1 was involved in the MAPK signaling pathway by diminishing the phosphorylation of p38, ERK, and JNK and inhibiting the activation of NF-κB.
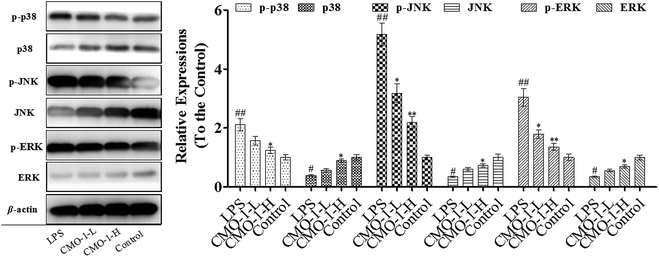 |
| Fig. 8 Effect of CMO-1 on the MAPK signaling pathways in RAW264.7 cells. RAW264.7 cells were stimulated with 1 μg mL−1 of LPS for 12 h; then, the cells were treated with CMO-1 (4.0, and 20.0 μM) for additional 4 h. The total protein lysate of each group was prepared, and cell-signaling proteins were detected by Western blot analysis. The values are expressed as means ± SD of triplicate tests. The protein expressions of the control groups were set as 1, and the values in the other groups were relative values to the control group. **p < 0.01, *p < 0.05, vs. LPS, ##p < 0.01, #p < 0.05, vs. control, indicate statistically significant difference. β-Actin was used as an internal standard of process control and blot band densitometry was analyzed with Image J software. | |
4 Discussion and conclusion
Scorpion venom represents a great diversity of bio-active proteins and peptides. Furthermore, the scorpion venom is widely used in the treatment of cardiovascular diseases such as for anti-thrombosis and anti-inflammation and for suppressing chronic pain due to its anti-inflammatory potency. To date, numerous studies have shown that scorpion venom peptides have been identified as potential anti-inflammatory agents, which can strongly scavenge inflammatory mediators and occupy a significant position. However, there is still a lack of further pharmacological studies on the monomers of peptides isolated from the scorpion venom. In the present study, a series of scorpion oligopeptides were screened out, which demonstrated excellent potent anti-inflammatory effects in LPS-induced RAW264.7 cells. Furthermore, the underlying mechanisms of anti-inflammatory potency were also explored.
NO is one of the most important inflammatory mediators. When attacked by inflammatory inducers, for example, by LPS, immune cells will produce NO, which is catalyzed by iNOS. Furthermore, high concentrations of NO can cause oxidative damage and even inflammatory and autoimmune diseases. Pro-inflammatory cytokines such as TNF-α and IL-1β also play crucial roles in the development of inflammatory diseases. The results in the current study indicated that CMO-1 significantly inhibited the NO, TNF-α and IL-1β production and significantly suppressed the iNOS expression in LPS-induced RAW264.7 cells. These results suggest that CMO-1 may demonstrate anti-inflammatory activity via inhibiting the production of pro-inflammatory cytokines.
NF-κB and MAPK signaling pathways can collaborate synergistically to promote the expression and release of target genes. NF-κB is a key signaling pathway related to regulating the transcription of numerous pro-inflammatory mediators and cytokines, including iNOS, IL-1β, and IL-6. The phosphorylation of IκBα by IKKα is a key process for the activation of NF-κB. When activated, p65 translocates into the nucleus and then promotes the transcription of the corresponding pro-inflammatory genes. To confirm the anti-inflammatory mechanisms of CMO-1, we evaluated the inhibitory effects of CMO-1 on the NF-κB and MAPK signaling pathways. In the current study, CMO-1 was found to markedly inhibit the NF-κB activation via suppressing the phosphorylation of IκBα and IKKα. MAPKs, including ERK, JNK and p38, also participated in the expression regulation of inflammation-related genes, leading to the overproduction of pro-inflammatory cytokines. The results revealed that CMO-1 obviously inhibited the LPS-induced phosphorylation of p38, ERK and JNK. We concluded that CMO-1 may demonstrate its anti-inflammatory potency through the inhibition of NF-κB and MAPK pathways in LPS-induced RAW264.7 cells.
In conclusion, we explored the anti-inflammatory potencies of the scorpion oligopeptides that were separated from the Chinese scorpion Mesobuthus martensii Karsch. The results demonstrated that CMO-1 is the most effective anti-inflammatory oligopeptide. CMO-1 suppressed the production of NO, TNF-α and IL-1β in LPS-induced RAW264.7 cells and the possible mechanisms might involve the down-regulation of the pro-inflammatory cytokines TNF-α and IL-1β and iNOS expression via suppressing the NF-κB and MAPK signaling pathways. In summary, for the first time, we indicated that CMOs exhibit anti-inflammatory potency and may be considered as potential anti-inflammatory agents.
Conflicts of interest
The authors declare no conflicts of interest.
Acknowledgements
We thank Professor Chen (Jinan University, Guangzhou, China) for technical assistance as well as critical editing of the manuscript.
References
- J. S. Silver, et al., Inflammatory triggers associated with exacerbations of COPD orchestrate plasticity of group 2 innate lymphoid cells in the lungs, Nat. Immunol., 2016, 17(6), 626–638 CrossRef CAS PubMed.
- E. D. Fang, et al., Association between diet-related inflammation, all-cause, all-cancer, and cardiovascular disease mortality, with special focus on prediabetics: findings from NHANES III, Eur. J. Nutr., 2016, 56(3), 1–9 Search PubMed.
- A. W. Gardner, et al., Endothelial Cell Inflammation and Antioxidant Capacity are Associated With 6-Minute Walk Performance in Patients With Symptomatic Peripheral Artery Disease, Angiology, 2017, 69(11), 000331971772693 Search PubMed.
- T. Padmore, et al., Quantitative analysis of the role of fiber length on phagocytosis and inflammatory response by alveolar macrophages, Biochim. Biophys. Acta, 2016, 1861(2), 58–71 CrossRef PubMed.
- Y. Chen, et al., Evaluation of serum concentrations of tumor necrosis factor (TNF)-α, interleukin (IL)-2, IL-10, and nitric oxide (NO) during the estrous cycle, early pregnancy and abortion in goats, Anim. Reprod. Sci., 2016, 174, 73–79 CrossRef CAS PubMed.
- Q. Zhang, et al., MCL Plays an Anti-Inflammatory Role in Mycobacterium tuberculosis-Induced Immune Response by Inhibiting NF-κB and NLRP3 Inflammasome Activation, Mediators Inflammation, 2017, 2017(4), 2432904–2432911 Search PubMed.
- V. Gebbia, et al., A prospective randomized trial of thymopentin versus granulocyte-colony stimulating factor with or without thymopentin in the prevention of febrile episodes in cancer patients undergoing highly cytotoxic chemotherapy, Anticancer Res., 1994, 14(2B), 731–738 CAS.
- Z. J. Dai, et al., Effects of Scutellaria Barbata drug-containing serum on apoptosis and mitochondrial transmembrane potential of hepatoma H22 cells, Zhongxiyi Jiehe Xuebao, 2008, 6(8), 821–826 CAS.
- Y. Wang, et al., SVP-B5 peptide from Buthus martensii Karsch scorpion venom exerts hyperproliferative effects on irradiated hematopoietic cells, Exp. Ther. Med., 2017, 14(5), 5081–5088 CAS.
- B. N. Hmed, H. T. Serria and Z. K. Mounir, Scorpion Peptides: Potential Use for New Drug Development, J. Toxicol., 2013, 2013(10), 1–15 Search PubMed.
- Q. Du, et al., AaeAP1 and AaeAP2: novel antimicrobial peptides from the venom of the scorpion, Androctonus aeneas: structural characterisation, molecular cloning of biosynthetic precursor-encoding cDNAs and engineering of analogues with enhanced antimicrobial and antican, Toxins, 2015, 7(2), 219–237 CrossRef PubMed.
- A. Borges, et al., Isolation, molecular cloning and functional characterization of a novel β-toxin from the Venezuelan scorpion, Tityus zulianus, Toxicon, 2004, 43(6), 671–684 CrossRef CAS PubMed.
- M. Ahmadi, et al., Study on anti inflammatory effect of scorpion (Mesobuthus eupeus) venom in adjuvant-induced arthritis in rats, Arch. Razi Inst., 2009, 51–56 Search PubMed.
- M. Ji, et al., Nicotine Component of Cigarette Smoke Extract (CSE) Decreases the Cytotoxicity of CSE in BEAS-2B Cells Stably Expressing Human Cytochrome P450 2A13, Int. Res. J. Public Environ. Health, 2017, 14(10), 1221–1228 CrossRef PubMed.
- X. H. Liu and Y. G. Yao, PDCD5 transfection increases cisplatin sensitivity and decreases invasion in hepatic cancer cells, Oncol. Lett., 2015, 9(1), 411–417 CrossRef PubMed.
- G. J. Wilmink, et al., Determination of Death Thresholds and Identification of Terahertz (THz)-Specific Gene Expression Signatures, in Optical Interactions with Tissues & Cells XXI, 2010 Search PubMed.
- X. Hu, et al., Ubiquitin-fold modifier 1 inhibits apoptosis by suppressing the endoplasmic reticulum stress response in Raw264.7 cells, Int. J. Mol. Med., 2014, 33(6), 1539–1546 CrossRef CAS PubMed.
- M. J. Raftery and C. L. Geczy, Electrospray low energy CID and MALDI PSD fragmentations of protonated sulfinamide cross-linked peptides, J. Am. Soc. Mass Spectrom., 2002, 13(6), 709–718 CrossRef CAS PubMed.
- M. C. Kang, et al., Protective effect of fucoxanthin isolated from Ishige okamurae against high-glucose induced oxidative stress in human umbilical vein endothelial cells and zebrafish model, J. Funct. Foods, 2014, 11, 304–312 CrossRef CAS.
- S. U. Rakesh, P. R. Patil and S. R. Mane, Use of natural antioxidants to scavenge free radicals: a major cause of diseases, Int. J. PharmTech Res., 2010, 2(2), 1074–1081 Search PubMed.
- E. S. Ai and N. Toshihide, Antioxidative Properties of Peptides Obtained from Porcine Myofibrillar Proteins by a Protease Treatment in an Fe(II)-Induced Aqueous Lipid Peroxidation System, J. Agric. Chem. Soc. Jpn., 2013, 77(11), 2201–2204 Search PubMed.
- Y. Jun-Jie, et al., Dual role of selected antioxidants found in dietary supplements: crossover between anti- and pro-oxidant activities in the presence of copper, J. Agric. Food Chem., 2012, 60(10), 2554–2564 CrossRef PubMed.
- S. R. Martini and T. A. Kent, Hyperglycemia in acute ischemic stroke: a vascular perspective, J. Cereb. Blood Flow Metab., 2007, 27(3), 435–451 CrossRef CAS PubMed.
- P. Shuyu, C. Young-Nam and K. Chaekyun, Taurine chloramine protects RAW 264.7 macrophages against hydrogen peroxide-induced apoptosis by increasing antioxidants, J. Clin. Biochem. Nutr., 2011, 49(1), 50–56 CrossRef PubMed.
- L. D. Zorova, et al., Functional Significance of the Mitochondrial Membrane Potential, Biochem. Suppl. Ser. A Membr. Cell Biol., 2018, 12(1), 20–26 Search PubMed.
- R. A. Kirkland, et al., Bax regulates production of superoxide in both apoptotic and nonapoptotic neurons: role of caspases, J. Neurosci., 2010, 30(48), 16114–16122 CrossRef CAS PubMed.
- S. Kaczanowski, M. Sajid and S. E. Reece, Evolution of apoptosis-like programmed cell death in unicellular protozoan parasites, Parasites Vectors, 2011, 4(1), 44 CrossRef PubMed.
- T. Atlung and F. G. Hansen, Effect of Different Concentrations of H-NS Protein on Chromosome Replication and the Cell Cycle in Escherichia coli, J. Bacteriol., 2002, 184(7), 1843–1856 CrossRef CAS PubMed.
- Y. Bagryantseva, et al., Oxidative damage to biological macromolecules in Prague bus drivers and garagemen: impact of air pollution and genetic polymorphisms, Toxicol. Lett., 2010, 199(1), 60–68 CrossRef CAS PubMed.
- J. Zhao, N. Xu and H. Liu, Quantitative Assessment of the Effects of Reducing Agents on Biological Macromolecules and on the Possible Repair of Oxidative Damage, BioMed Res. Int., 2018, 2018, 1–7 Search PubMed.
- K. M. Braun and M. P. Diamond, The biology of adhesion formation in the peritoneal cavity, Semin. Pediatr. Surg., 2014, 23(6), 336–343 CrossRef PubMed.
- J. L. Yeh, et al., Eugenolol and glyceryl-isoeugenol suppress LPS-induced iNOS expression by down-regulating NF-kappaB AND AP-1 through inhibition of MAPKS and AKT/IkappaBalpha signaling pathways in macrophages, Int. J. Immunopathol. Pharmacol., 2011, 24(2), 345–356 CrossRef CAS PubMed.
- K. Hanako, et al., Interleukin-32beta propagates vascular inflammation and exacerbates sepsis in a mouse model, PLoS One, 2010, 5(3), 9458–9465 CrossRef PubMed.
- A. Hasturk, et al., Analysis of serum pro-inflammatory cytokine levels after rat spinal cord ischemia/reperfusion injury and correlation with tissue damage, Turkish Neurosurgery, 2009, 19(4), 353–362 Search PubMed.
- Y. Kyung-Jin, et al., Anti-inflammatory effects of sinapic acid through the suppression of inducible nitric oxide synthase, cyclooxygase-2, and proinflammatory cytokines expressions via nuclear factor-kappaB inactivation, J. Agric. Food Chem., 2008, 56(21), 10265–10272 CrossRef PubMed.
- P. C. Chang, et al., Advanced glycosylation end products induce inducible nitric oxide synthase (iNOS) expression via a p38 MAPK-dependent pathway, Kidney Int., 2004, 65(5), 1664–1669 CrossRef CAS PubMed.
Footnotes |
† Electronic supplementary information (ESI) available. See DOI: 10.1039/c9ra01623f |
‡ Contributed equally to this work |
|
This journal is © The Royal Society of Chemistry 2019 |
Click here to see how this site uses Cookies. View our privacy policy here.