DOI:
10.1039/C9FO01951K
(Paper)
Food Funct., 2020,
11, 860-870
Protection by pure and genistein fortified extra virgin olive oil, canola oil, and rice bran oil against acetic acid-induced ulcerative colitis in rats
Received
24th August 2019
, Accepted 13th December 2019
First published on 20th December 2019
Abstract
Genistein is a major isoflavone with antioxidant and anti-inflammatory activities and hydrophobic features. To increase its bioavailability, researchers supplemented genistein with dietary oils. The present study aims to compare the effectiveness of genistein supplementation with water solution or oils and find the best possible dietary oil for fortification. A total of 135 male Sprague-Dawley rats were randomly assigned to nine groups, including one control group and eight acetic acid-induced colitis groups. The rats in the intervention groups were treated with 5 ml per kg body weight of oral pure and genistein fortified forms of extra virgin olive oil (EVOO), canola oil (CaO), and rice bran oil (RBO); the Genistein group (G) received 100 mg per kg body weight of genistein in aqueous solution orally. The colitis and control groups did not receive any treatment. The levels of colonic MDA (malondialdehyde), MPO (myeloperoxidase) activity, and IL-1β (interleukin-1β) were evaluated and a stereological analysis of colonic tissues was performed. All of the dietary oils (EVOO, CaO, and RBO) were effective at ameliorating the rats’ oxidative and inflammatory status (p < 0.05); however, EVOO (with or without genistein) prescription resulted in slightly better results, especially with regard to the inflammatory profile. Additionally, delivering genistein via an oil vehicle was more efficient at reducing MDA formation, MPO activity, and IL-1β concentration than genistein in aqueous solution. The quantitative analysis of colonic tissue was consistent with the biochemical analysis. Our findings suggest that the oral administration of EVOO, canola oil, and rice bran oil can attenuate the elevated IL-1β levels and oxidative damage in induced colitis. Moreover, genistein fortified oils, especially EVOO, showed more beneficial effects in decreasing these markers in comparison with the pure oils or genistein (aqueous solution).
1. Introduction
Ulcerative colitis (UC) is the chronic relapsing and remitting inflammatory disorder of the colon and rectum which annually affects approximately one million people around the world.1 The etiology of UC is unclear but seems to be multifactorial as a result of a complex interaction which exists between the genetic susceptibility and immunological and environmental factors.2 A change in leukotriene and prostaglandin formation due to oxidative damage in the intestine following an increase in the immune response by up-regulation of some inflammatory mediators is the main characteristic of UC.3,4 Currently, drugs used to manage UC include 5-aminosalicylates, glucocorticoids, and immunomodulators, which target different points of the inflammatory cascade. However, they have some serious side effects.5
Functional foods could be a desirable candidate for overcoming the limitations of the current therapeutic agents used in UC treatment. Soy and soy products are well-known functional foods rich in bioactive components such as isoflavones, saponins, and phytosterols.6 Genistein is a major isoflavone found primarily in soy and soy products. Studies in the last few years have shown that genistein has potential anti-oxidative and anti-inflammatory effects on various diseases, from insulin resistance to alcoholic or non-alcoholic fatty liver disease and intestinal inflammation.7–10 All of the functions suggested above could be attributed to different biological activities of genistein, including free radical scavenging activity of phenolic groups,11 ability to bind to estrogen receptors,12 and inhibition of tyrosine kinases.13 Genistein has a small molecular weight (270 g mol−1) with lipophilic features.14 Studies have suggested that the low aqueous solubility of genistein may be the reason for the poor pharmacokinetic response to an increased administered dose.15 Edible oils are an appropriate option for increasing the bioavailability of genistein. However, the effects of dietary oils on intestinal inflammation are different due to the fatty acid composition and the presence of different vitamins (mostly vitamin E) and natural phenolic compounds. These variations have led researchers to question which oil is most suitable to be used in supplementation with genistein or even for general dietary use.
Clinical studies have shown that extra virgin olive oil (EVOO), which is high in mono-unsaturated fatty acid (MUFA) and phenolic compounds, has beneficial effects on oxidative stress.16,17 In addition, EVOO prescription in animal studies with induced inflammatory disease exhibited a significant improvement both in local and systemic inflammation.18,19 Furthermore, epidemiological studies have shown the role of n-6 fatty acids in the etiology of UC and the potential protective effects of n-3 fatty acids against UC.20–22 Clinical and animal studies have revealed the advantages of using oils with an appropriate ratio of ω-3/ω-6 (1/2 or 1/3) polyunsaturated fatty acids (PUFAs). Canola oil (CaO) is one of the most popular edible oils with an appropriate ω-3/ω-6 fatty acid ratio.23 CaO, in combination with other dietary nutrient modifications in severely injured patients, has been shown to have beneficial effects on cellular immune function and inflammatory stimuli.24 In addition, the results of several clinical studies show that a lower ω-3/ω-6 fatty acid ratio or the use of CaO can have a favorable effect on oxidative stress and inflammation, especially on the stressed organ.25–27 Rice bran oil (RBO) is another potential choice for UC patients. RBO, in addition to having a high amount of vitamin E and bioactive phytonutrients, such as γ-oryzanol, has a relatively high proportion of MUFA (about 41%).23,28 Both animal and human clinical studies have suggested that rice bran oil administration can reduce oxidative stress and increase antioxidant power.29–31
The aim of this study was to evaluate the effects of genistein supplementation on the oxidative and inflammatory status and stereological indices in rats with acetic acid-induced UC. In addition, the efficacy of three different edible oils (EVOO, CaO, and RBO) on the UC related factors was examined.
2. Materials and methods
2.1. Animals
135 male Sprague-Dawley rats (200 ± 20 gr) were provided by the animal center of the Shiraz University of Medical Sciences. The rats were randomly divided into nine groups (15 rats in each group, 7 rats for a 3-day intervention, and 8 rats for a 7-day intervention). Each rat was housed individually in a single cage in a room with a controlled temperature of 25 ± 2, an ambient 55% relative humidity, and under a 12 h light and 12 h dark cycle. All animals received a free soy standard diet (Khorak Dam Pars co. Tehran, Iran) and had free access to food and water. Table 2 presents the composition of diet pellets. This study was approved by the state committee on animal ethics, Shiraz University of Medical Sciences, Shiraz, Iran (no. 6886-01-01-92). In addition, all of the study protocols were designed to follow the National Committee of Ethics in Biochemical Research guidelines in animal studies. The sample size was calculated based on the following formula with 80% power, standard deviation 4.6 pg mg−1, and 7.4 pg g−1 effect size.32 The sample size was adjusted with 20% expected attrition.
Sample size = 2SD2 (Zα/2 + Zβ)2/d2 |
Table 1 Fatty acid composition of dietary oils
|
Oil composition |
Amount (%) |
Rice bran oil |
Canola oil |
Extra virgin olive oil |
1 |
Palmitic acid, methyl ester |
21.13 |
7.60 |
12.21 |
2 |
Palmitoleic acid acid, methyl ester (Z) |
0.33 |
0.12 |
1.50 |
3 |
Stearic acid, methyl ester |
2.33 |
2.48 |
1.98 |
4 |
Oleic acid, methyl ester |
39.08 |
60.08 |
68.21 |
5 |
Linoleic acid, methyl ester |
32.69 |
17.73 |
9.89 |
6 |
Alpha linolenic acid, methyl ester |
2.61 |
8.95 |
0.98 |
7 |
Gondoic acid, methyl ester |
0.35 |
1.10 |
0.51 |
8 |
Arachidonic acid, methyl ester |
0.89 |
0.34 |
0.55 |
9 |
Behenic acid, methyl ester |
— |
0.25 |
0.28 |
10 |
Squalene |
— |
— |
1.29 |
11 |
Others |
0.20 |
0.29 |
2.03 |
12 |
Unknown |
0.39 |
1.06 |
0.57 |
Table 2 Composition of a chewing diet
Ingredient |
(g kg−1) |
Casein |
140 |
Corn starch |
495.7 |
Maltodextrin 10 |
115 |
Sucrose |
120 |
Corn oil |
40 |
Cellulose |
50 |
Mineral mix |
35 |
Vitamin mix |
10 |
Choline bitartrate |
2.5 |
L-Cysteine |
1.8 |
2.2. UC induction and intervention
All animals were fasted for 24 hours in order to clean their bowel before the induction of colitis. A polyurethane catheter was used for the rectal injection of acetic acid. Rats were lightly anesthetized by the inhalation of ether (Merck, Darmstadt, Germany). The tip of the catheter (2 mm diameter) was inserted into the colon up to 8 cm proximal to the anus, and 2 ml of 3% acetic acid (Merck, Darmstadt, Germany) was injected rectally into their colon.33 The control group received 2 ml of physiological saline transrectally. Twenty-four hours after the UC induction, the intervention period was initiated. The rats were included in the following groups: the control and colitis groups, which received 5 ml per kg body weight of physiological saline orally; the intervention groups, which received 5 ml per kg BW of oral extra virgin olive oil (EVOO), canola oil (CaO), rice bran oil (RBO), extra virgin olive oil fortified with genistein (EVOO + G), canola oil fortified with genistein (CaO + G), rice bran oil fortified with genistein (RBO + G) and 100 mg per kg BW of genistein dissolved in 1% carboxymethyl cellulose solution. Pure genistein powder (≥98.5%) was purchased from DSM Nutritional Products (Ltd, Switzerland). All of the intervention solutions were gavaged daily by bolus doses.
Oil fortification with genistein was done in such a way that the daily intake of genistein was kept at 100 mg per kg BW in groups that received fortified oils.34 Half of the rats in each group received therapy for three days and were then sacrificed; the other halves were treated for seven days before being sacrificed. Animals were fasted 24 h before and after colitis induction and body weight was measured daily. Eighteen rats were spared during the study because of colonic wall perforation and were excluded from statistical analysis. Half of the animals on the 4th day and the other half on the 8th day were sacrificed by cervical dislocation, and tissue samples were obtained from the rats' colons for stereological and biochemical analyses. Tissue samples were immediately transferred to a nitrogen tank and kept frozen at −80 °C until the analysis.
2.3. Determination of the fatty acid composition of oils
Methyl ester production experiments were carried out using the following procedure. Olive oil (0.5 g) was dissolved in 40 ml methanol in a bottom flask, and 0.5 ml of 2 N methanolic KOH, as a catalyst, was added. The reaction mixture was refluxed for about 1 hour to obtain a clear solution. The product was cooled and followed by a separation process using a separating funnel. 20 ml of H2O and 40 ml of n-heptane were added to a methanolic solution. The mixture was shaken for 1 min and allowed to stand for 10 min. The heptanoic layer was removed and washed with water. Finally, the heptanoic phase was placed in contact with anhydrous sodium sulfate to remove moisture. The same process was done for the other oils. Product samples were analyzed by gas chromatography and methyl esters were observed for further analysis.35
GC–MS analysis was performed on a GC-7890A gas chromatograph (Agilent, US) equipped with a 7000 triple-quad mass spectrometer (Agilent, US). A DB-1 capillary column (30 m × 0.25 mm id, film thickness 0.25 mm; Agilent,) was used for separation. The oven temperature program was initiated at 70 °C, increased to 280 °C at the rate of 3 °C min−1, and then kept at this temperature for about 4 min. The spectrometers were operated in electron-impact (EI) mode with an ionization energy of 70 eV; the scan range was 40–600 amu. The injector, interface, and ion sources were kept at 250, 280, and 230 °C, respectively. 1 μl of the sample was injected into the GC-MS instrument. Helium, as a carrier gas, was used at a constant flow rate of 1.2 mL min−1.36,37
The chemical components in these oils were identified by comparing their RI and mass spectrum (MS) from the NIST library.38
2.4. Myeloperoxidase activity analysis
Myeloperoxidase (MPO) is an enzyme with oxidative properties and is released by activated neutrophils. For an MPO assay, a segment of frozen colonic tissue, weighing 250 mg, was homogenized with 5 ml of 14 mM hexadecyltrimethylammonium bromide (HTAB) (Merck, Darmstadt, Germany) in 50 mM potassium phosphate buffer, pH 6.0, with a Polytron (Bandelin, Germany) for 10 seconds. The supernatants were produced by centrifugation at 3000 rpm for 10 min and used to estimate the MPO activity.39 0.1 ml of the supernatant was mixed with 2.9 ml of 50 mM phosphate buffer, pH 6.0, containing 0.167 mg ml−1o-dianisidinedihydrochloride (Merck, Darmstadt, Germany) and 0.0005% hydrogen peroxide (Merck, Darmstadt, Germany). The changes in the absorbance at 460 nm were measured with a spectrophotometer (Apel, Japan). MPO activity, which was expressed as units per mg tissue, was defined as the number of H2O2 micromoles converted into a product per minute per mg tissue at room temperature.40
2.5. Malondialdehyde analysis
Malondialdehyde (MDA) is a marker of lipid peroxidation. Hence, we used it to measure lipid peroxidation in colonic tissue. A reaction mixture, containing 200 μl of 8.1% SDS, 1500 μl of 20% acetic acid, 1500 μl of 0.8% thiobarbituric acid, 0.0034 mM butylated hydroxytoluene in ethanol, and 540 μl distilled water, was prepared. Afterward, 200 μg of the colonic homogenate (the same colonic homogenate as prepared for the MPO activity analysis) was added to the reaction mixture, then heated for 1 h at 95 °C, and centrifuged at 3000 rpm for 10 min. The absorbance of the supernatants was measured spectrophotometrically at 531 nm. Different concentrations (100–800 nM) of tetramethoxypropane were used to define the standard curve.39
2.6. Interleukin 1-beta analysis
Interleukin 1-beta (IL-1β) levels in tissue extracts were determined by specific enzyme-linked immunosorbent assay (ELISA) techniques according to the manufacturer's instructions (Cristal Day, China) and using the same tissue extraction method applied for the MPO assay.
2.7. Stereological methods
Half of the animals in each group were euthanized on the 7th day, and the distal 10 cm portion of the colon was transferred into formalin buffer for stereological evaluations. The volume of the colon was measured using the immersion method. Briefly, it was suspended using a thread in a bottle of physiological saline. The difference between the bottle weight before and after the suspension was divided by 1.0048 (the specific gravity of the saline). Histological sections (4 μm) were obtained from the colon and stained with hematoxylin and eosin. The volume density of each histological structure “Vv (structure)”, including mucosal epithelium, muscularis mucosa, submucosa, and muscularis externa, was estimated using the point-counting method with the following formula:
Vv (structure) = ∑P (structure)/∑P (reference) |
where the ∑P (structure) and ∑P (reference) are the total points, which hit the favored structure and the colon sections in each animal. The total volume of the histological structures was obtained by multiplying the density of the colon volume.
2.8. Statistical analysis
The data were examined for normality of distribution. Normally-distributed data were analyzed using one-way Analysis of Variance (ANOVA). When a significant difference was obtained with ANOVA, the source of the difference was located using the Tukey–Kramer test. Data that were not normally distributed were analyzed by the Kruskal–Wallis test followed by Dunn's test. The analysis was performed using SPSS software, version 23. P < 0.05 was considered as significant.
3. Results
The rats were spared during the study because of colonic wall perforation and excluded from statistical analysis. The fatty acid profiles of rice bran, canola, and olive oils are summarized in Table 1. The results showed that the predominant fatty acids in all oils were oleic acid. EVOO and CaO had the lowest amount of linoleic acid and palmitic acid, respectively, whereas RBO had the highest amount of these two fatty acids.
The results of the daily weighing of animals are presented in Fig. 1. No significant differences regarding animals’ weight were observed at the baseline (day −1). However, after inducing colitis (day 0), all the animals lost weight except for the control group that continued to gain weight. Although treatments resulted in the attenuation of weight loss after colitis induction, weight gain in the colitis group was significantly lower than that of the groups treated with edible oil at the end of the study.
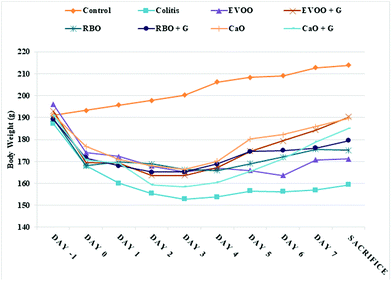 |
| Fig. 1 Daily records of body weight in each study group. | |
Acetic acid induction of colitis led to an increase in the MDA content of colonic tissue (Fig. 2). However, compared to the colitis group, all the dietary groups, except pure RBO (P = 0.14), showed a significant improvement in the colonic MDA levels on the 7th day of the study. It should be mentioned that in the RBO groups the MDA levels also showed a significant reduction on the 3rd day of the study (P-value = 0.04). Genistein supplementation, compared with RBO alone, significantly increased the beneficial effects of RBO in inhibiting the production of MDA (P = 0.01). However, in comparison with the pure forms of oils, EVOO and CaO fortification seemed not to exert any significant changes in diminishing the elevated MDA levels (P = 0.055 and 0.054). Genistein fortified oils were more effective at lowering colonic MDA compared to genistein alone (G group).
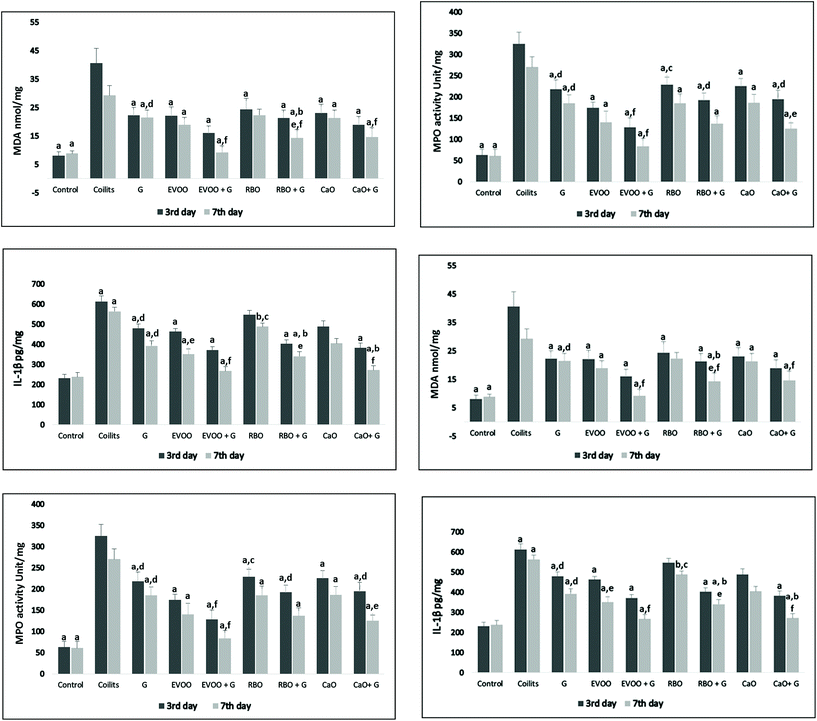 |
| Fig. 2 MDA, MPO activity and IL-1β concentration of colonic tissue from study groups. Symbols represent significant differences vs. the (a) colitis group, (b) pure form of oil, (c) EVOO group, (d) EVOO + G group, (e) 3 days of intervention in the same group, (f) G group. (P < 0.05). P-Value assessed by the Kruskal–Wallis test followed by Dunn's test. | |
The result of tissue-associated MPO activity analysis is shown in Fig. 2. The MPO activity significantly increased in the colitis groups in comparison with that of the control group. There were significant decreases in MPO activity levels in all the groups compared to that of the colitis group on the 3rd and 7th days after treatment (P < 0.05). No significant differences were observed in the attenuation of MPO activity in the same groups on different days, except for the genistein fortified form of CaO which showed a significant difference between the 3rd and 7th days of the intervention (P = 0.02). Among all the treatment groups, only EVOO significantly amplified the effects of genistein in reducing MPO activity. In addition, on day 3, the rats receiving EVOO + G had significantly lower MPO activity than the CaO + G (P-value = 0.03) and RB + G (P = 0.04). Similarly, pure EVOO, compared to RBO, had a stronger effect on MPO activity on the 3rd day of intervention (P = 0.04). However, these differences were not constant on the 7th day. Finally, the genistein supplementation with EVOO suppressed the MPO activity more than did the genistein alone.
The colonic levels of IL-1β were significantly higher in the colitis than those of the control group. Although genistein supplementation alone did not induce any significant reduction in IL-1β, its supplementation with dietary oils (EVOO, CaO, and RBO) effectively down-regulated the IL-1β production. Oil supplementation diminished pro-inflammatory cytokine production, but changes were not statistically significant in the oral supplementation with RBO and CaO on the 3rd and 7th days of intervention. A seven-day intervention, in comparison with a three-day intervention, by EVOO resulted in lower colonic levels of IL-1β (P-value = 0.01). It is noteworthy that fortified canola and rice bran oils, compared with their pure forms, could significantly decrease the cytokine levels of IL-1β on the 7th day of supplementation (P = 0.03 and 0.01), although these changes failed to show significant differences on the 3rd day of the study. Except for RBO, CaO and EVOO fortification improved the IL-1β down-regulation potential more significantly than genistein alone. Data comparing the groups are presented in Fig. 2.
3.1. Histological and stereological evaluations
Fig. 3 represents the colonic photomicrograph of the study groups.
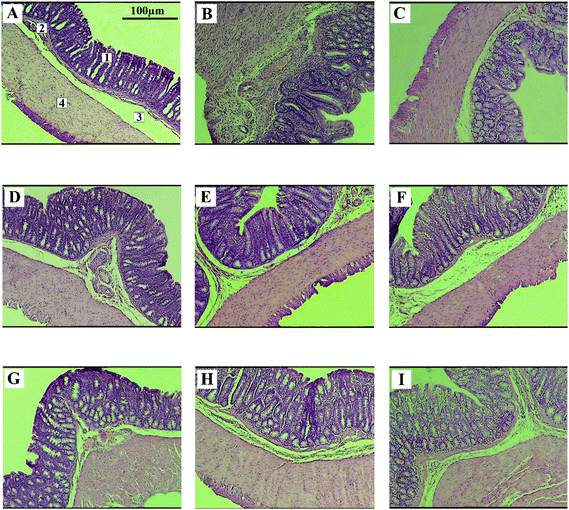 |
| Fig. 3 Photomicrograph of a colonic section after 7 days of intervention from each study group. 1: Mucosa, 2: muscularis mucosa, 3: submucosa, and 4: muscularis externa. Control (A), colitis (B), G (C), EVOO (D), EVOO + G (E), CaO (F), CaO + G (G), RBO (H), and RBO + G (I). The control group shows a normal pattern of stereological tissue. The contrary colitis group exhibits atrophy in the epithelial layer of mucosa and depletion of the crypts as well as lesser submucosal tissue can be observed. Moreover, the muscular layer has shown hypertrophic changes. The normal structure was recovered at least partially in the treatment groups. | |
The total volume of the epithelial layer of the mucosa reduced by 31% (P < 0.001) in the colitis group in comparison with that of the control group. The UC induction reduced the total mucosa volume in the treatment groups (EVOO, CaO, G, EVOO + G, CaO + G, and RBO + G), but to a lower extent than that of the colitis group (p < 0.05). The treatment of the colitis animals with RBO did not cause any significant improvement in them (p = 0.13). Fig. 4 shows the changes in the volume of different mucosal layers after the intervention.
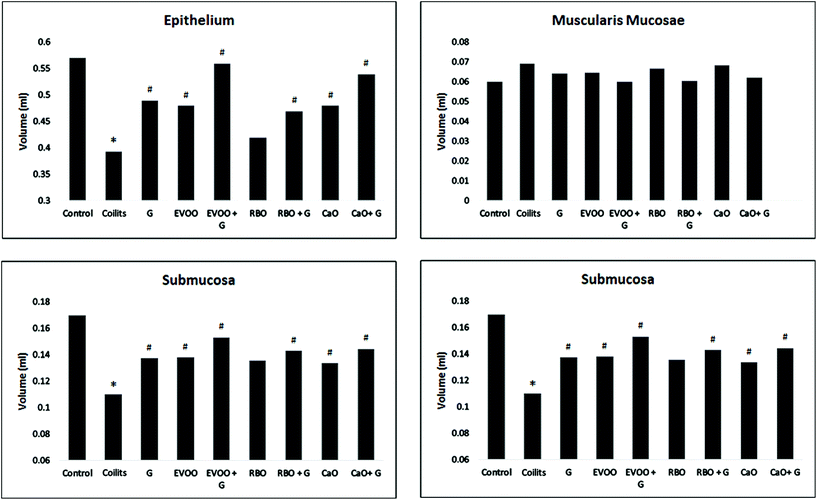 |
| Fig. 4 The volume of each mucosal layer evaluated by stereological counting. * indicates a significant difference (p < 0.05) from the control group. # indicates a significant difference (p < 0.05) from the colitis group. | |
The total volume of muscularis mucosae of the colonic tissue increased by 15% (p > 0.05) in the colitis group in comparison with that of the control group. The total volume of muscularis mucosae was reduced by 13%, 10%, 13%, 6%, 1%, 3%, and 1% in the groups treated with EVOO + G, CaO + G, RBO + G, EVOO, CaO, RBO, and G, respectively, compared with that of the colitis group.
The total volume of submucosa of the colonic tissue was reduced by 45% (p < 0.001) in the colitis group in comparison with that of the control. However, the volume of submucosa in the treatment groups, including EVOO + G, CaO + G, RB + G, EVOO, CaO, RBO, and G, increased by 39% (p < 0.001), 31% (p < 0.001), 31% (p < 0.001), 25% (p = 0.01), 21% (p = 0.04), 23% (p = 0.01), and 19% (p < 0.062), respectively.
The total volume of the muscularis externa layer of the colon tissue increased by 44% (p < 0.01) in the colitis group in comparison with that of the control group. The total volume of muscularis externa increased in the treatment groups (EVOO + G, CaO + G, RBO + G, EVOO, CaO, and G), but to a lower extent than that of the colitis group (p < 0.01). Conversely, RBO administration did not result in a significant improvement in the colitis group. Fig. 1 illustrates the histopathological changes.
4. Discussion
Unlike conventional immunosuppressive drugs with unwanted side effects, using functional food and supplements, as safer therapies, has become more prevalent. The results of our study indicated the efficacy of oil supplementation fortified with genistein. The present study showed that despite the effectiveness of all the dietary oils (EVOO, CaO, and RBO) at ameliorating the oxidative and inflammatory status, EVOO (with or without genistein) prescription resulted in slightly better results, especially with regard to the inflammatory profile. Additionally, delivering genistein via an oil vehicle was more efficient than genistein in aqueous solution at reducing MDA formation, MPO activity, and IL-1β. Eventually, the quantitative analysis of the colonic tissue was consistent with the results obtained from the biochemical analysis.
Our data showed that EVOO could suppress the elevated levels of MDA, MPO activity, and IL-1β in the colonic tissue of rats. This was in line with the results of a previous study in which EVOO supplementation in an experimental model of ulcerative colitis led to an improvement in the levels of pro-inflammatory cytokines, such as TNF-α (tumor necrosis factor- α) and IL-10 (interleukin 10).41 The beneficial effects of EVOO are not limited to pro-inflammatory cytokine down-regulation. As studies on animals with inflammation origin diseases (colitis and arthritis) have reported, EVOO can drastically reduce the activity of the inflammatory-related pathways, including cyclooxygenase-2 (COX-2), inducible nitric oxide synthase (iNOS), JAK/STAT, MAPKs, and NF-κB.42,43 These findings suggest that EVOO could be considered as an alternative therapy for inflammatory conditions like UC. Its beneficial effects may be due to its high oleic acid (ω9) content and/or the presence of polyphenolic and bioactive compounds. Oleic acid has been shown to have anti-inflammatory and immune-modulatory properties.44 The results of other studies seem to approve our results. For example, a reduction was observed in IL-1β when sepsis-induced mice were administered oleic acid; the mice exhibited lower IL-1β and TNF-α, along with an increase in the anti-inflammatory cytokine IL-10.45 In addition, bioactive compounds, including squalene and phenolic compounds, present in EVOO have been shown to reduce the intracellular level of reactive oxygen species (ROS), augment the expression of transcriptional factors with anti-inflammatory properties, such as nuclear factor erythroid 2-related factor 2 (Nrf2) and peroxisome proliferator-activated receptor gamma (PPARγ), and change the cell-signaling pathways.46 Furthermore, previous studies on the UC model have shown that oleuropein, the main polyphenol in olive oil, supplementation can mitigate the inflammatory symptoms, in addition to decreasing the intestinal IL-6, IL-1β concentration, and COX-2 expression.47–49
In the present investigation, we observed that CaO could significantly reduce MDA levels and MPO activities, although the reduction in the tissue content of IL-1β did not reach a significant level. CaO composition analysis showed a high concentration of oleic acid (≈60%) and a balanced ratio [nearly 2
:
1] of ω6 (≈18%)/ω3 PUFAs (≈9%).50 It also contained plant sterols (0.53–0.97%) and tocopherols (700–1200 ppm).51,52 It has been shown that treating colitis models with ω3 fatty acids can lead to reduced intestine mucosal myeloperoxidase activity and pro-inflammatory cytokines, such as TNF-α and IL-1β.53 Eicosanoids are a family of lipid mediators that regulate immune responses. The role of eicosanoids is well established in immunological and autoimmune diseases. Prostaglandin E2 and leukotriene B4 are proinflammatory molecules from the large family of eicosanoids and are mostly generated from arachidonic acid (ω6 fatty acid) oxidation. The use of a suitable amount of ω3 fatty acids may be helpful as these fatty acids are a potent competitor for the arachidonic acid cascade in producing leukotriene B4, prostaglandin E2, and thromboxane A2.54 Similar to EVOO, CaO is also a great source of oleic acid, which makes it a potential candidate to have the beneficial effects of MUFA in EVOO. On the other hand, plant sterols and tocopherols available in CaO can have antithrombotic and anti-oxidative properties;52 thus, it may have the aforementioned positive effects attributed to CaO supplementation.
Moreover, the result of this study showed that RBO could suppress the increased MPO activity on the 3rd and 7th day of intervention. Although it had a significant effect on IL-1β and MDA production on the 3rd day of intervention, these changes were not statistically significant after 7 days. Oil composition analysis showed that RBO had a moderate amount of linoleic acid (about 32% of total fatty acids), oleic acid (39%), and palmitic acid (21%) and a low concentration of alpha-linolenic acid (2.6%). The lower efficiency of RBO, compared with EVOO and CaO, in reducing the inflammatory and oxidative status may be partially due to its higher amount of saturated fatty acids (SFAs) and high ω6/ω3 ratio. Previous studies have shown that SFA can increase the expression of inflammatory cytokines via the activation of nuclear factor-kappaB (NF-κB), a transcriptional factor, which in turn is activated by protein kinase Cθ and Toll-like receptor activation.55,56 However, RBO is a rich source of bioactive phytochemicals, such as oryzanol and phenolic compounds.57 γ-Oryzanol, a rice bran isolated mixture of the phytosterol ferulates (100 mg per kg body weight), can diminish the level of inflammatory cytokines, such as TNF-α, IL-1β, and COX-2.58 In addition, tocotrienols have been shown to have antioxidant properties in several in vitro systems. According to the United States department of agriculture (USDA) nutrient database, the analysis of dietary oils shows that for the same amounts of oil, RBO provides twice as much alpha-tocopherol as EVOO or CO does.59 Alpha-tocopherol, a major member of the vitamin E family, is a strong lipophilic vitamin present in vegetable oils. The number of studies performed to evaluate the effects of vitamin E on inflammatory bowel disease is limited. However, it has been shown that tocopherols can protect the cell membrane against ROS and, consequently, modulate the immune system. Vitamin E can reverse the injury of colonic tissue in acetic acid-induced colitis and reduce the elevated levels of TNF-α, IL-1β, IL-6, myeloperoxidase, and malondialdehyde.60 Furthermore, there is a promising report of remission when colitis patients in the active state were rectally treated with alpha-tocopherol for 12 weeks.61 Due to the inappropriate fatty acid composition of RBO, an increase is expected to happen in the oxidative and inflammatory indicators, although significant improvement in at least one of the assessed factors (MPO activity) may be explained by the considerable amount of vitamin E in RBO.
RBO has also been known as one of the richest dietary oils with vitamin E (approximately 1% of oil unsaponified).28
Leaving aside these findings, there has been no strong methodological study which has used RBO for therapeutic purposes to treat UC; therefore, our results cannot be compared with those of any other study. However, the results of some studies on other inflammatory-related diseases, including rheumatoid arthritis and non-alcoholic steatohepatitis, indicate that RBO supplementation can down-regulate inflammation and reduce oxidative damage.62,63
Based on our findings, genistein in aqueous solution was able to induce a biochemical and stereological improvement in rats with UC. However, adding genistein to oils, especially EVOO, led to higher antioxidative and anti-inflammatory activities. This may partially be due to the beneficial effects of the dietary oils, as shown in EVOO, CaO, and RBO groups, but this supplementation rendered better results than a pure form of oils or genistein solution alone. A previous study showed that the oral supplementation of genistein (100 mg per kg BW) decreased MPO activity and COX-2 expression in an animal model of TNBS (2,4,6-trinitrobenzenesulfonic acid)-induced colitis. It has been suggested that genistein might inhibit the COX-2 expression via an ER-dependent mechanism, which can reduce prostaglandin levels.34 It should be noted that EVOO fortification with genistein was more effective than CaO and RBO fortification with genistein at reducing the biochemical and stereological alteration damage. Data from the stereological analysis follow similar patterns regarding the oxidative and inflammatory factors, i.e. the EVOO + G group had the best remission in comparison with the colitis groups, followed by CaO + G and RBO + G. Taking into consideration that each dietary oil exhibited significant improvement in nearly all of the ulcerative colitis related factors, it is difficult to distinguish the role of each of the oil components (fatty acid composition, the content of vitamin E, and bioactive compounds) in colitis remission; however, in this study, the cumulative effects of these components on ulcerative colitis are presented and compared. Our study had several strengths and limitations. We did not measure the vitamin E content of the oils, which can play an important role in the anti-oxidant and anti-inflammatory properties of dietary oils. On the other hand, in the present study, we determined the fatty acid composition of the dietary oils and used stereological analysis to accurately quantify changes in the microscopic level. Furthermore, our study is the first study that has investigated the effects of CaO and RBO, along with genistein fortification, on oxidative and inflammatory markers and offered a stereological evaluation of colonic tissue.
5. Conclusion
According to the results of this study, all of the dietary oils could improve the oxidative and inflammatory status to a different extent and in some aspects. EVOO was found to be more effective in this regard. Moreover, adding genistein to the dietary oils resulted in a significantly better outcome regarding the oxidative and inflammatory status in comparison with the pure oils or genistein (aqueous solution) alone. Furthermore, the analysis of the stereological data approved the results obtained from the biochemical indices. Hence, using these properties can possibly open new horizons in the treatment of ulcerative colitis by nutritional supplementation.
Conflicts of interest
There are no conflicts of interest to declare.
Acknowledgements
The authors wish to thank the Research Consultation Center (RCC) at Shiraz University of Medical Sciences for their invaluable assistance in editing this manuscript. Shiraz University of Medical Sciences financially supported this study.
References
- S. H. Itzkowitz, Molecular biology of dysplasia and cancer in inflammatory bowel disease, Gastroenterol. Clin. North Am., 2006, 35, 553–571 CrossRef PubMed.
- S. C. Ng, C. N. Bernstein, M. H. Vatn, P. L. Lakatos, E. V. Loftus, C. Tysk, C. O'Morain, B. Moum and J.-F. Colombel, Geographical variability and environmental risk factors in inflammatory bowel disease, Gut, 2013, 62, 630–649 CrossRef PubMed.
- V. L. Kumar, A. Pandey, S. Verma and P. Das, Protection afforded by methanol extract of Calotropis procera latex in experimental model of colitis is mediated through inhibition of oxidative stress and pro-inflammatory signaling, Biomed. Pharmacother., 2019, 109, 1602–1609 CrossRef CAS PubMed.
- L. Mayer, Evolving paradigms in the pathogenesis of IBD, J. Gastroenterol., 2010, 45, 9–16 CrossRef CAS PubMed.
- C. T. Xu, S. Y. Meng and B. R. Pan, Drug therapy for ulcerative colitis, World J. Gastroenterol., 2004, 10, 2311–2317 CrossRef CAS PubMed.
- H. Jooyandeh, Soy products as healthy and functional foods, Middle East J. Sci. Res., 2011, 7, 71–80 Search PubMed.
- N. L. V. Braber, I. N. Nuñez, L. Bohl, C. Porporatto, F. N. Nazar, M. A. Montenegro and S. G. Correa, Soy genistein administered in soluble chitosan microcapsules maintains antioxidant activity and limits intestinal inflammation, J. Nutr. Biochem., 2018, 62, 50–58 CrossRef PubMed.
- L. Zhao, Y. Wang, J. Liu, K. Wang, X. Guo, B. Ji, W. Wu and F. Zhou, Protective effects of genistein and puerarin against chronic alcohol-induced liver injury in mice via antioxidant, anti-inflammatory, and anti-apoptotic mechanisms, J. Agric. Food Chem., 2016, 64, 7291–7297 CrossRef CAS PubMed.
- R. Zhang, J. Xu, J. Zhao and Y. Chen, Genistein improves inflammatory response and colonic function through NF-κB signal in DSS-induced colonic injury, Oncotarget, 2017, 8, 61385 Search PubMed.
- X. Xin, C. Chen, Y.-Y. Hu and Q. Feng, Protective effect of genistein on nonalcoholic fatty liver disease (NAFLD), Biomed. Pharmacother., 2019, 117, 109047 CrossRef CAS PubMed.
- K. B. Pandey and S. I. Rizvi, Plant polyphenols as dietary antioxidants in human health and disease, Oxid. Med. Cell. Longevity, 2009, 2, 270–278 CrossRef PubMed.
- Z.-R. Du, X.-Q. Feng, N. Li, J.-X. Qu, L. Feng, L. Chen and W.-F. Chen, G protein-coupled estrogen receptor is involved in the anti-inflammatory effects of genistein in microglia, Phytomedicine, 2018, 43, 11–20 CrossRef CAS PubMed.
- A. A. Elmarakby, A. S. Ibrahim, J. Faulkner, M. S. Mozaffari, G. I. Liou and R. Abdelsayed, Tyrosine kinase inhibitor, genistein, reduces renal inflammation and injury in streptozotocin-induced diabetic mice, Vasc. Pharmacol., 2011, 55, 149–156 CrossRef CAS PubMed.
- J.-G. Wu, J. Ge, Y.-P. Zhang, Y. Yu and X.-Y. Zhang, Solubility of genistein in water, methanol, ethanol, propan-2-ol, 1-butanol, and ethyl acetate from (280 to 333) K, J. Chem. Eng. Data, 2010, 55, 5286–5288 CrossRef CAS.
- S. Zhou, Y. Hu, B. Zhang, Z. Teng, H. Gan, Z. Yang, Q. Wang, M. Huan and Q. Mei, Dose-dependent absorption, metabolism, and excretion of genistein in rats, J. Agric. Food Chem., 2008, 56, 8354–8359 CrossRef CAS PubMed.
- B. Barbaro, G. Toietta, R. Maggio, M. Arciello, M. Tarocchi, A. Galli and C. Balsano, Effects of the olive-derived polyphenol oleuropein on human health, Int. J. Mol. Sci., 2014, 15, 18508–18524 CrossRef PubMed.
- A. I. Katsarou, A. C. Kaliora, A. Chiou, N. Kalogeropoulos, A. Papalois, G. Agrogiannis and N. K. Andrikopoulos, Amelioration of oxidative
and inflammatory status in hearts of cholesterol-fed rats supplemented with oils or oil-products with extra virgin olive oil components, Eur. J. Nutr., 2016, 55, 1283–1296 CrossRef CAS PubMed.
- A. Voltes, A. Bermúdez, G. Rodríguez-Gutiérrez, M. Reyes, C. Olano, J. Fernández-Bolaños and F. D. L. Portilla, Anti-Inflammatory Local Effect of Hydroxytyrosol Combined with Pectin-Alginate and Olive Oil on Trinitrobenzene Sulfonic Acid-Induced Colitis in Wistar Rats, J. Invest. Surg., 2018, 1–7 CAS.
- C. Santangelo, R. Vari, B. Scazzocchio, P. De Sanctis, C. Giovannini, M. D'Archivio and R. Masella, Anti-inflammatory activity of extra virgin olive oil polyphenols: which role in the prevention and treatment of immune-mediated inflammatory diseases?, Endocr., Metab. Immune Disord.: Drug Targets, 2018, 18, 36–50 CAS.
- I. B. D. i. E. S. Investigators, A. Tjonneland, K. Overvad, M. M. Bergmann, G. Nagel, J. Linseisen, G. Hallmans, R. Palmqvist, H. Sjodin, G. Hagglund, G. Berglund, S. Lindgren, O. Grip, D. Palli, N. E. Day, K. T. Khaw, S. Bingham, E. Riboli, H. Kennedy and A. Hart, Linoleic acid, a dietary n-6 polyunsaturated fatty acid, and the aetiology of ulcerative colitis: a nested case-control study within a European prospective cohort study, Gut, 2009, 58, 1606–1611 CrossRef PubMed.
- A. N. Ananthakrishnan, H. Khalili, G. G. Konijeti, L. M. Higuchi, P. de Silva, C. S. Fuchs, W. C. Willett, J. M. Richter and A. T. Chan, Long-term intake of dietary fat and risk of ulcerative colitis and Crohn's disease, Gut, 2014, 63, 776–784 CrossRef CAS PubMed.
- P. S. de Silva, R. Luben, S. S. Shrestha, K. T. Khaw and A. R. Hart, Dietary arachidonic and oleic acid intake in ulcerative colitis etiology: a prospective cohort study using 7-day food diaries, Eur. J. Gastroenterol. Hepatol., 2014, 26, 11–18 CrossRef CAS PubMed.
- R. C. Zambiazi, R. Przybylski, M. W. Zambiazi and C. B. Mendonça, Fatty acid composition of vegetable oils and fats, Bol. Cent. Pesqui. Process. Aliment., 2007, 25, 111–120 CAS.
- C. Mendez, G. J. Jurkovich, M. H. Wener, I. Garcia, M. Mays and R. V. Maier, Effects of supplemental dietary arginine, canola oil, and trace elements on cellular immune function in critically injured patients, Shock, 1996, 6, 7–12 CrossRef CAS PubMed.
- L. G. Yang, Z. X. Song, H. Yin, Y. Y. Wang, G. F. Shu, H. X. Lu, S. K. Wang and G. J. Sun, Low n-6/n-3 PUFA ratio improves lipid metabolism, inflammation, oxidative stress and endothelial function in rats using plant oils as n-3 fatty acid source, Lipids, 2016, 51, 49–59 CrossRef PubMed.
- M. Atefi, G. R. Pishdad and S. Faghih, The effects of canola and olive oils on insulin resistance, inflammation and oxidative stress in women with type 2 diabetes: a randomized and controlled trial, J. Diabetes Metab. Disord., 2018, 17, 85 CrossRef PubMed.
- A. Salar, S. Faghih, G. Pishdad and P. Rezaie, Comparison of the effects of canola oil and rice bran oil consumption on oxidative stress and blood pressure in postmenopausal type 2 diabetic women: A Randomized Controlled Clinical Trial, J. Nutr. Sci. Diet., 2015, 199–205 Search PubMed.
- E. De Deckere and O. Korver, Minor constituents of rice bran oil as functional foods, Nutr. Rev., 1996, 54, S120–S126 CrossRef CAS PubMed.
- A. Sengupta, M. Ghosh and D. K. Bhattacharyya, Antioxidative Effect of Rice Bran Oil and Medium Chain Fatty Acid Rich Rice Bran Oil in Arsenite Induced Oxidative Stress in Rats, J. Oleo Sci., 2014, 63, 1117–1124 CrossRef CAS PubMed.
- A. Salar, S. Faghih, G. Pishdad and P. Rezaie, Comparison of the effects of canola oil and rice bran oil consumption on oxidative stress and blood pressure in postmenopausal type 2 diabetic women: A randomized controlled clinical trial, J. Nutr. Sci. Diet., 2015, 199–205 Search PubMed.
- T. Utarwuthipong, S. Komindr, V. Pakpeankitvatana, S. Songchitsomboon and N. Thongmuang, Small dense low-density lipoprotein concentration and oxidative susceptibility changes after consumption of soybean oil, rice bran oil, palm oil and mixed rice bran/palm oil in hypercholesterolaemic women, J. Int. Med. Res., 2009, 37, 96–104 CrossRef CAS PubMed.
- X.-L. Yang, T.-K. Guo, Y.-H. Wang, Y.-H. Huang, X. Liu, X.-X. Wang, W. Li, X. Zhao, L.-P. Wang and S. Yan, Ginsenoside Rd attenuates the inflammatory response via modulating p38 and JNK signaling pathways in rats with TNBS-induced relapsing colitis, Int. Immunopharmacol., 2012, 12, 408–414 CrossRef CAS PubMed.
- N. Tanideh, E. Afaridi, D. Mehrabani, N. Azarpira, M. Hosseinzadeh, M. Amini and S. Hosseini, The healing effect of berberis vulgaris in acetic acid-induced ulcerative colitis in rat, Middle East J. Sci. Res., 2014, 21, 1288–1294 Search PubMed.
- J. Seibel, A. F. Molzberger, T. Hertrampf, U. Laudenbach-Leschowski and P. Diel, Oral treatment with genistein reduces the expression of molecular and biochemical markers of inflammation in a rat model of chronic TNBS-induced colitis, Eur. J. Nutr., 2009, 48, 213–220 CrossRef CAS PubMed.
- X. Liu, F. Wang, X. Liu, Y. Chen and L. Wang, Fatty acid composition and physicochemical properties of ostrich fat extracted by supercritical fluid extraction, Eur. J. Lipid Sci. Technol., 2011, 113, 775–779 CrossRef CAS.
- A. Abbaszadegan, S. Sahebi, A. Gholami, A. Delroba, A. Kiani, A. Iraji and P. V. Abbott, Time-dependent antibacterial effects of Aloe vera and Zataria multiflora plant essential oils compared to calcium hydroxide in teeth infected with Enterococcus faecalis, J. Invest. Clin. Dent., 2016, 7, 93–101 CrossRef PubMed.
- M. Mardani, S. M. Afra, N. Tanideh, A. Andisheh Tadbir, F. Modarresi, O. Koohi-Hosseinabadi, A. Iraji and M. Sepehrimanesh, Hydroalcoholic extract of Carum carvi L. in oral mucositis: a clinical trial in male golden hamsters, Oral Dis., 2016, 22, 39–45 CrossRef CAS PubMed.
- G. Pourghanbari, H. Nili, A. Moattari, A. Mohammadi and A. Iraji, Antiviral activity of the oseltamivir and Melissa officinalis L. essential oil against avian influenza A virus (H9N2), VirusDisease, 2016, 27, 170–178 CrossRef PubMed.
- A. Al-Jarallah, M. A. Oriowo and I. Khan, Mechanism of reduced colonic contractility in experimental colitis: role of sarcoplasmic reticulum pump isoform-2, Mol. Cell. Biochem., 2007, 298, 169–178 CrossRef CAS PubMed.
- P. P. Bradley, D. A. Priebat, R. D. Christensen and G. Rothstein, Measurement of cutaneous inflammation: estimation of neutrophil content with an enzyme marker, J. Invest. Dermatol., 1982, 78, 206–209 CrossRef CAS PubMed.
- S. Sánchez-Fidalgo, L. S. De Ibargüen, A. Cardeno and C. A. de la Lastra, Influence of extra virgin olive oil diet enriched with hydroxytyrosol in a chronic DSS colitis model, Eur. J. Nutr., 2012, 51, 497–506 CrossRef PubMed.
- M. A. Rosillo, M. Sánchez-Hidalgo, S. Sánchez-Fidalgo, M. Aparicio-Soto, I. Villegas and C. Alarcón-de-la-Lastra, Dietary extra-virgin olive oil prevents inflammatory response and cartilage matrix degradation in murine collagen-induced arthritis, Eur. J. Nutr., 2016, 55, 315–325 CrossRef CAS PubMed.
- S. Sánchez-Fidalgo, I. Villegas, M. Aparicio-Soto, A. Cárdeno, M. Á. Rosillo, A. González-Benjumea, A. Marset, Ó. López, I. Maya and J. G. Fernández-Bolaños, Effects of dietary virgin olive oil polyphenols: hydroxytyrosyl acetate and 3, 4-dihydroxyphenylglycol on DSS-induced acute colitis in mice, J. Nutr. Biochem., 2015, 26, 513–520 CrossRef PubMed.
- H. Kim, K. Youn, E.-Y. Yun, J.-S. Hwang, W.-S. Jeong, C.-T. Ho and M. Jun, Oleic acid ameliorates Aβ-induced inflammation by downregulation of COX-2 and iNOS via NFκB signaling pathway, J. Funct. Foods, 2015, 14, 1–11 CrossRef CAS.
- I. M. Medeiros-de-Moraes, C. F. Gonçalves-de-Albuquerque, A. R. Kurz, F. M. D. J. Oliveira, V. H. P. D. Abreu, R. C. Torres, V. F. Carvalho, V. Estato, P. T. Bozza and M. Sperandio, Omega-9 oleic acid, the main compound of olive oil, mitigates inflammation during experimental sepsis, Oxid. Med. Cell. Longevity, 2018, 2018, 6053492 Search PubMed.
- A. Cárdeno, M. Aparicio-Soto, S. Montserrat-de la Paz, B. Bermudez, F. J. Muriana and C. Alarcón-de-la-Lastra, Squalene targets pro-and anti-inflammatory mediators and pathways to modulate over-activation of neutrophils, monocytes and macrophages, J. Funct. Foods, 2015, 14, 779–790 CrossRef.
- E. Giner, M. C. Recio, J. L. Ríos, J. M. Cerdá-Nicolás and R. M. Giner, Chemopreventive effect of oleuropein in colitis-associated colorectal cancer in c57bl/6 mice, Mol. Nutr. Food Res., 2016, 60, 242–255 CrossRef CAS PubMed.
- E. Giner, M.-C. Recio, J.-L. Rios and R.-M. Giner, Oleuropein protects against dextran sodium sulfate-induced chronic colitis in mice, J. Nat. Prod., 2013, 76, 1113–1120 CrossRef CAS PubMed.
- S. Sanchez-Fidalgo, A. Cardeno, M. Sanchez-Hidalgo, M. Aparicio-Soto and C. A. de la Lastra, Dietary extra virgin olive oil polyphenols supplementation modulates DSS-induced chronic colitis in mice, J. Nutr. Biochem., 2013, 24, 1401–1413 CrossRef CAS PubMed.
- P. Nigam, S. Bhatt, A. Misra, D. S. Chadha, M. Vaidya, J. Dasgupta and Q. M. Pasha, Effect of a 6-month intervention with cooking oils containing a high concentration of monounsaturated fatty acids (olive and canola oils) compared with control oil in male Asian Indians with nonalcoholic fatty liver disease, Diabetes Technol. Ther., 2014, 16, 255–261 CrossRef CAS PubMed.
-
F. Gunstone, Vegetable oils in food technology: composition, properties and uses, John Wiley & Sons, 2011 Search PubMed.
- L. Lin, H. Allemekinders, A. Dansby, L. Campbell, S. Durance-Tod, A. Berger and P. J. Jones, Evidence of health benefits of canola oil, Nutr. Rev., 2013, 71, 370–385 CrossRef PubMed.
- A. Tyagi, U. Kumar, S. Reddy, V. S. Santosh, S. B. Mohammed, N. Z. Ehtesham and A. Ibrahim, Attenuation of colonic inflammation by partial replacement of dietary linoleic acid with alpha-linolenic acid in a rat model of inflammatory bowel disease, Br. J. Nutr., 2012, 108, 1612–1622 CrossRef CAS PubMed.
- A. Gil, Polyunsaturated fatty acids and inflammatory diseases, Biomed. Pharmacother., 2002, 56, 388–396 CrossRef CAS.
- T. Coll, E. Eyre, R. Rodríguez-Calvo, X. Palomer, R. M. Sánchez, M. Merlos, J. C. Laguna and M. Vázquez-Carrera, Oleate reverses palmitate-induced insulin resistance and inflammation in skeletal muscle cells, J. Biol. Chem., 2008, 283, 11107–11116 CrossRef CAS PubMed.
- J. J. Senn, Toll-like receptor-2 is essential for the development of palmitate-induced insulin resistance in myotubes, J. Biol. Chem., 2010, 285, 14842–14842 CrossRef CAS.
- R. Jariwalla, Rice-bran products: phytonutrients with potential applications in preventive and clinical medicine, Drugs Exp. Clin. Res., 2000, 27, 17–26 Search PubMed.
- M. Islam, T. Murata, M. Fujisawa, R. Nagasaka, H. Ushio, A. Bari, M. Hori and H. Ozaki, Anti-inflammatory effects of phytosteryl ferulates in colitis induced by dextran sulphate sodium in mice, Br. J. Pharmacol., 2008, 154, 812–824 CrossRef CAS PubMed.
-
S. Gebhardt, L. Lemar, D. Haytowitz, P. Pehrsson, M. Nickle, B. Showell, R. Thomas, J. Exler and J. Holden, USDA national nutrient database for standard reference, release 21, United States Department of Agriculture-Agricultural Research Service, 2008.
- G. Tahan, E. Aytac, H. Aytekin, F. Gunduz, G. Dogusoy, S. Aydin, V. Tahan and H. Uzun, Vitamin E has a dual effect of anti-inflammatory and antioxidant activities in acetic acid–induced ulcerative colitis in rats, Can. J. Surg., 2011, 54, 333 CrossRef PubMed.
- S. A. Mirbagheri, B. G. Nezami, S. Assa and M. Hajimahmoodi, Rectal administration of d-alpha tocopherol for active ulcerative colitis: a preliminary report, World J. Gastroenterol., 2008, 14, 5990 CrossRef CAS PubMed.
- N. V. Yadav, B. Ramaiyan, P. Acharya, L. Belur and R. R. Talahalli, Sesame oil and Rice bran oil ameliorates adjuvant-induced arthritis in rats: distinguishing the role of minor components and fatty acids, Lipids, 2016, 51, 1385–1395 CrossRef CAS PubMed.
- S. Y. Al-Okbi, D. A. Mohamed, T. E. Hamed and R. S. Esmail, Rice bran oil and pumpkin seed oil alleviate oxidative injury and fatty liver in rats fed high fructose diet, Pol. J. Food Nutr. Sci., 2014, 64, 127–133 CrossRef CAS.
|
This journal is © The Royal Society of Chemistry 2020 |
Click here to see how this site uses Cookies. View our privacy policy here.