DOI:
10.1039/C9RA09497K
(Paper)
RSC Adv., 2020,
10, 6006-6016
Upregulation of lncRNA SUMO1P3 promotes proliferation, invasion and drug resistance in gastric cancer through interacting with the CNBP protein
Received
14th November 2019
, Accepted 19th January 2020
First published on 7th February 2020
Abstract
Gastric cancer (GC) is one type of the most common malignancies in the world. In the process of exploring the pathological mechanism of GC and searching for treatment methods, long non-coding RNAs (lncRNAs) display significant participation. Small ubiquitin-like modifier 1 pseudogene 3 (SUMO1P3) is a newly identified lncRNA, of which the biological role and underlying mechanism in GC progression have not been elucidated. Here, through the comparisons between GC patients' tumor and normal tissue samples, as well as normal gastric mucosal and GC cell lines, we confirmed a significant upregulation of SUMO1P3 in GC tissues and cell lines. Meanwhile, significant upregulation of SUMO1P3 was observed in advanced GC patients, and patients with high level of SUMO1P3 displayed a poor survival rate. Next, gain- and loss-of-function experiments were performed in GC cells, and the results exhibited that SUMO1P3 positively regulated proliferation and invasion of GC cells. Then, we constructed drug-resistant GC cell strains and explore the role of SUMO1P3 in the resistance of GC cells to cisplatin (DDP) and 5-fluorouracil (5-Fu). Finally, bioinformatics analysis and RNA pull-down assay demonstrated that SUMO1P3 could directly interact with cellular nucleic acid binding protein (CNBP), thus positively regulating CNBP downstream oncogenes c-myc and cyclin D1 (CCND1). Our findings indicate that SUMO1P3 promotes proliferation, invasion and drug resistance of GC cells by interacting with CNBP, which reveals a potential prognostic biomarker and a novel therapeutic target for GC.
1 Introduction
As one of the most frequently occurring cancers, gastric cancer (GC) ranks third among the death-leading cancers worldwide.1 In spite of the incidence of GC decreasing in the bygone decades in the wake of the development of medical practice and technology, in China, the GC mortality is still dreadful with a lower than 30% five-year survival rate due to the poor prognosis in advanced GC.2–4 As such, for improving GC outcomes, it is an urgent task to uncover the mechanism of GC and discover the specific biomarkers for GC prognosis and therapy.
There are many genes whose biological importance was reported to be more outstanding in human malignancies because of their anomalous-expressions.5 Thereinto, the vital role long non-coding RNAs (lncRNAs) played in cancer development and progression was emphasized by the advanced expansion of RNA genomics.6 Accumulating evidence persuasively documented that lncRNAs, which could transcript longer than 200 nucleotides and do not code for proteins, emerged regulatory functions on cancer and tumorigenesis in various aspects, consisting of cell proliferation, cellular cycle, apoptosis, metastasis and invasion.7,8 In GC related research, an increasing number of lncRNAs have been discovered in recent years. As an example included, the lncRNA colon cancer related transcription factor 1 (CCAT1) was overexpressed in GC tissues and cell lines, and it was a contributor for the growth and invasion of GC.9 The upregulation of lncRNA metastasis-associated lung adenocarcinoma transcript 1 (MALAT1) also effectively affected the proliferation and apoptosis of GC, thus to modulate gastric tumorigenesis.10 Besides, the reason why lncRNA HOX transcript antisense RNA (HOTAIR) could be accounted as a novel target of GC prevention and therapy was that lncRNA HOTAIR exerted an inhibitory role in GC occurrence and development.11 However, the poor prognosis of partial lncRNAs is a tricky question for their application on clinical diagnosis.12,13 Therefore, lncRNAs and their functional mechanism in regulation of GC progression need further studies and characterization.
Small ubiquitin-like modifier 1 pseudogene 3 (SUMO1P3) is a newly discerned pseudogene of SUMO1 in human genome, simultaneously, it belongs to a separate class of lncRNAs.14,15 Scientists have validated that lncRNA SUMO1P3 was highly expressed in multiple cancers, such as colon cancer, bladder cancer, breast cancer and hepatocellular carcinoma; on top of that, it was associated with tumor progressions and cancerous cell events; herein, the oncogenic functions of SUMO1P3 makes it a promising prognostic and therapeutic target for cancer patients.6,14,16,17 Howbeit, the evidence of the relationship between the biological function and mechanism of SUMO1P3 and gastric cancer is still deficiency.
In the present study, we verified that the expression of SUMO1P3 was predominantly elevated in GC patient tissues compared with adjacent nontumorous tissues, and high expressed SUMO1P3 was relevant to tumor stage and patient survival. Knockdown and overexpression of SUMO1P3 further indicated that SUMO1P3 could modulate proliferation and invasion of GC cell lines. Except that, we examined the association between SUMO1P3 expression and drug resistance of GC, also we performed RNA-pulldown and transfection assays to explore the potential molecular mechanism of SUMO1P3 combining with databases analysis. In all, we dissected the function and mechanism of SUMO1P3 in GC and provided the value of SUMO1P3 in prognosis and therapeutic strategy for GC.
2 Results
2.1 SUMO1P3 was upregulated in GC
For the determination of the association between SUMO1P3 expression and GC, we performed real time fluorescence quantitative polymerase chain reaction (RT-qPCR) in 69 GC patients' tissue samples and 6 types of various cell lines. The fold change of SUMO1P3 expression was up-modulated in most of GC patients' pathological tissues compared with their matched normal gastric tissues (Fig. 1A), and was still significantly up-regulated in integrated grade (Fig. 1B). In addition, according to Topography-Lymph Node-Metastasis (TNM) staging, the expression of SUMO1P3 in GC individuals was classified and validated that it was obviously elevated in advanced stages (Fig. 1C and D). Five-year survival rate curve told us patients with lower SUMO1P3 expression would survive for a further life (Fig. 1E). Moreover, compared with normal gastric epithelial mucosal cells (RGM-1 and GES-1), GC cells with high metastatic capacity (SGC-7901 and MKN-45) presented predominant up-regulation of SUMO1P3 expression, while the moderate metastatic GC cells expressed SUMO1P3 at a medium level also with significant difference (Fig. 1F). Taken together, we proposed SUMO1P3 as an oncogene in GC.
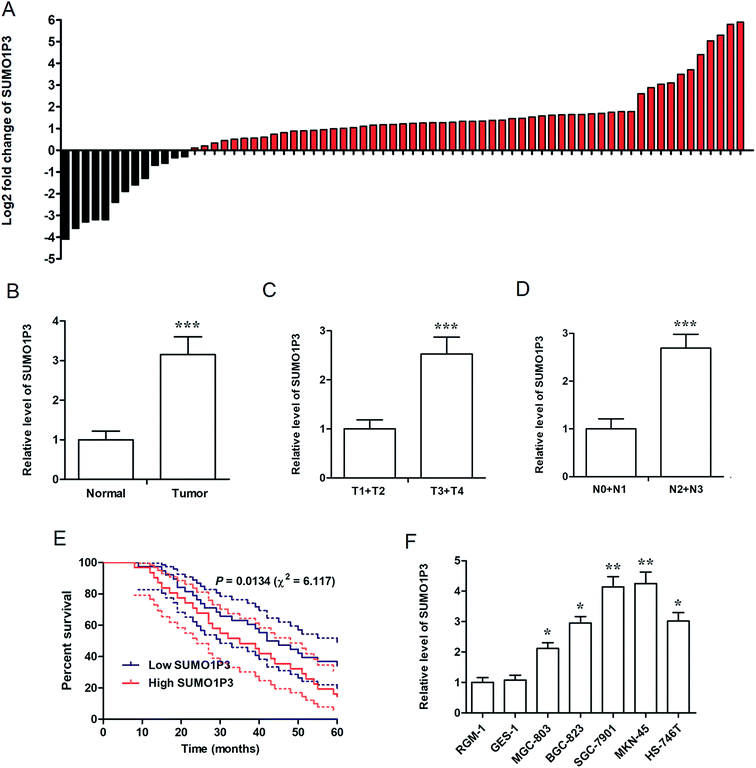 |
| Fig. 1 High level of SUMO1P3 was observed in advanced GC patients and was associated with poor survival rate of GC patients. Using RT-qPCR method, the relative expression level of SUMO1P3 was detected. (A) The log2 fold change of SUMO1P3 in GC tissues was elevated compared with adjacent normal tissues. (B) Relative SUMO1P3 expression of GC tissues was significantly higher than normal gastric tissues. ***P < 0.005 versus normal. (C) The expression of SUMO1P3 was upregulated in GC patients with advanced T stages. ***P < 0.005 versus T1 + T2. (D) The expression level of SUMO1P3 in GC tissues at advanced N stages was obviously higher than those at early stages. ***P < 0.005 versus N0 + N1. (E) The five-year survival percentage of patients with low SUMO1P3 expression and high SUMO1P3 expression. The dashed curves stand for 95% confidence interval of survival rates. (F) Higher expression of SUMO1P3 was presented in GC cell lines compared with normal epithelial cell lines of gastric mucosa. *P < 0.05 versus RGM-1, **P < 0.01 versus RGM-1. We express data as the mean ± SEM of three repetitions. | |
2.2 Knockdown of SUMO1P3 inhibits proliferation and invasion of GC cell
To analyze the effects of SUMO1P3 knockdown on gastric cancer cellular proliferation and invasion, were transfected different concentrations of SUMO1P3 siRNA into BGC-826 and SGC-7901 cells. The results of RT-qPCR showed that SUMO1P3 siRNA substantially down-regulated the relative level of SUMO1P3 in both BGC-826 and SGC-7901, and the transfection efficiency was affected by the concentration of siRNA (Fig. 2A and D). Subsequently, the detection of cell proliferation indicated that the interference of SUMO1P3 remarkably decreased the proliferative capacities of GC cells which presented as the absorbance at 450 nm; besides, the reduction extent was more significant as the concentration of SUMO1P3 siRNA augmenting (Fig. 2B and E). The similar restrained effect of SUMO1P3 knockdown was also observed in the invasion percentages of GC cell lines (Fig. 2C and F). These results could give the evidence that SUMO1P3 interfered GC cell is characterized by low proliferative and invasive activities.
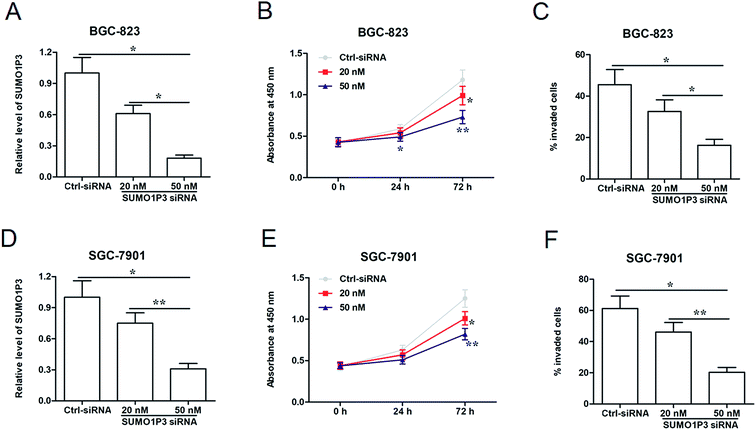 |
| Fig. 2 SUMO1P3 siRNA inhibited proliferation and invasion of GC cells in a manner of dose-dependent. BGC-823 and SGC-7901 GC cell lines were transfected by SUMO1P3 siRNA at the concentrations of 20 nM and 50 nM and ctrl siRNA for 24 h, followed by the detections of relevant indicators. (A and D) The expression levels of SUMO1P3 in BGC-823 and SGC-7901 GC cell lines were detected using RT-qPCR. *P < 0.05 versus 50 nM SUMO1P3 siRNA. **P < 0.01 versus 50 nM SUMO1P3 siRNA. (B and E) CCK-8 assays showed the cell proliferation of BGC-823 and SGC-7901 GC cell lines. *P < 0.05 versus ctrl-siRNA. **P < 0.01 versus ctrl-siRNA. (C and F) The percentages of invaded cells in BGC-823 and SGC-7901 GC cell lines were detected by transwell assays. *P < 0.05 versus 50 nM SUMO1P3 siRNA. **P < 0.01 versus 50 nM SUMO1P3 siRNA. Data are represented as the mean ± SEM of three replicates. | |
2.3 Overexpression of SUMO1P3 has a facilitating effect on proliferation and invasion of GC cells
Then, SUMO1P3 overexpression assay was performed to further corroborate the moderation that SUMO1P3 exerted on cancer cellular proliferation and invasion. pcDNA-SUMO1P3 expression vector was transfected into BGC-826 and SGC-7901 cell lines. We found that the expression of SUMO1P3 was significantly upregulated in GC cells (Fig. 3A and D). SUMO1P3 overexpression increased the proliferation (Fig. 3B and E) and invasion (Fig. 3C and F) of GC cells.
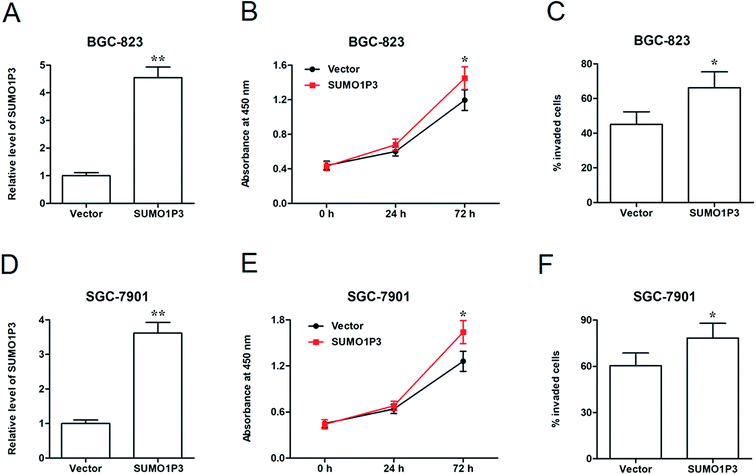 |
| Fig. 3 Overexpression of SUMO1P3 promoted proliferation and invasion of GC cells. BGC-823 and SGC-7901 GC cell lines were transfected by 1.2 μg mL−1 pcDNA-SUMO1P3 and empty vector, then the relevant indicators were detected. (A and D) The determination of SUMO1P3 relative levels in BGC-823 and SGC-7901 GC cell lines were performed by RT-qPCR. **P < 0.01 versus vector. (B and E) CCK-8 assays presented the cell proliferation of BGC-823 and SGC-7901 GC cell lines. *P < 0.05 versus vector. (C and F) Transwell assays were conducted to detect the percentages of invaded cells in BGC-823 and SGC-7901 GC cell lines. *P < 0.05 versus vector. Data are expressed as the mean ± SEM with three replicates. | |
2.4 Knockdown of SUMO1P3 suppressed the drug resistance of GC cell
As far as we know, drug resistance is one of the biggest enemies of the effective chemotherapy to cancers at present; however, some studies have pointed out that lncRNAs have to do with the mechanism of drug resistance of cancer.18–20 Under these circumstances, explorations on SUMO1P3 expression and drug resistance of GC cell became highly necessary. Therefore, two drug resistant GC cell strains, respectively resistant to cisplatin (DDP) and 5-fluorouracil (5-Fu), were established based on SGC-7901 cells, which were named as SGC-7901/DDP and SGC-7901/5-Fu. We found that the expression of SUMO1P3 in drug-resistant GC cells was significantly higher than that in normal SGC-7901 cells (Fig. 4A). SGC-7901/DDP cells transfected SUMO1P3 overexpressed vector presented a decreased inhibitory rate after 48 h DDP treatment, while the value of half-inhibitory concentration (IC50) in SGC-7901/DDP cells for DDP got an obvious elevation due to the overexpression of SUMO1P3; simultaneously, the knockdown of SUMO1P3 increased the inhibitory rate of 48 h DDP treatment on SGC-7901/DDP cells, and also reduced the IC50 for DDP (Fig. 4B and D). Moreover, the overexpression and interference of SUMO1P3 presented declined and elevated effects on the 5-Fu inhibitory rate as well as the increased and decreased functions on IC50 for 5-Fu in SUMO1P3/5-Fu cells, respectively (Fig. 4C and E). Generally, the drug resistance of GC cells could be suppressed by SUMO1P3 down-regulation, providing clue for the clinical application of SUMO1P3 to the diagnosis and treatment of GC.
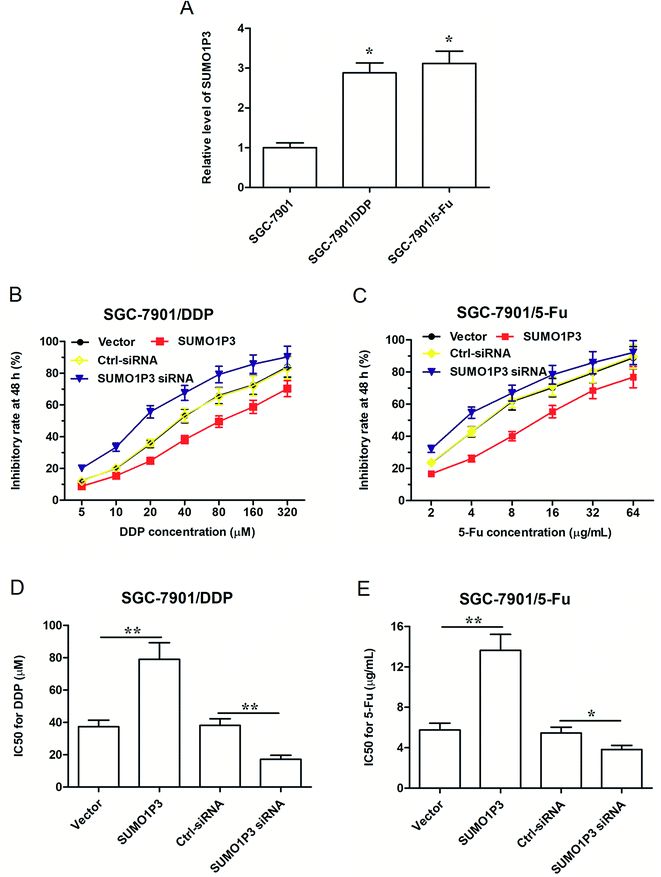 |
| Fig. 4 The expression of SUMO1P3 had association with drug resistance of GC cell lines. SGC-7901 cell line was pre-treated to generate drug resistant SGC-7901/DDP and SGC-7901/5-Fu. Then the two drug resistant cell lines were transfected by SUMO1P3 siRNA and pcDNA-SUMO1P3 for 24 h, taking pcDNA3.1 vector and ctrl-siRNA as control. After that, SGC-7901/DDP and SGC-7901/5-Fu were treated with DDP and 5-Fu for 48 h, then the indexes were determined. (A) SUMO1P3 expression levels of SGC-7901/DDP and SGC-7901/5-Fu were detected through RT-qPCR method. *P < 0.05 versus SGC-7901. (B and C) The determinations of drug sensitivities of DDP and 5-Fu were executed by MTS assays in SGC-7901/DDP and SGC-7901/5-Fu. (D and E) IC50 for drugs was calculated from the results of MTS assays. In (D) **P < 0.01 versus vector and SUMO1P3 siRNA, respectively; in (E) *P < 0.05 versus SUMO1P3 siRNA, **P < 0.01 versus vector. The values are presented as the means ± SEM from at least three independent experiments. | |
2.5 SUMO1P3 could directly bind to CNBP and affect the expression of c-myc and cyclin D1 (CCND1) proteins
According to previous studies, cellular nucleic acid binding protein (CNBP) has both single strand DNA and RNA binding abilities and reportedly regulates DNA transcription in both negative and positive ways.21–24 Herein, to study the function mechanism of SUMO1P3 in GC, we took CNBP as a target gene and conducted interaction prediction between SUMO1P3 and CNBP by using the online database RNA-Protein Interaction Prediction (RPISeq). The output from this prediction showed an extremely high interaction probability, up to 0.95, of SUMO1P3 and CNBP (Fig. 5A). Subsequently, we analyzed the secondary structure of SUMO1P3 to find a possible combining manner with CNBP. The results presented that the minimum free energy of this 1210-nucleotide lncRNA molecule is about −308.0 kcal mol−1, and there were three stem-loop structures at different ends in the secondary structure of SUMO1P3 (Fig. 5B). To validate the interaction between SUMO1P3 and CNBP, as well as the CNBP binding region on SUMO1P3, we performed RNA pull-down assay. Then, we drew the conclusion that SUMO1P3 was bond to CNBP by the predicted truncated region 1, from 82 nt to 233 nt on SUMO1P3 sequence (Fig. 5C). As far as we know, there was evidence which confirmed that ectopic c-myc transcription was a common performance of human malignancies; CNBP could combine c-myc at NHE III1 region, then promote c-myc transcription.25 Additionally, CCND1, as one of the commonly and frequently amplified in GC, was found to have the interaction with CNBP thus to obtain the ability of ensuring orderly cell cycle progression.26,27 Then, through the siRNA and pcDNA vector transfection into GC cells and the detection of c-myc and CCND1 protein levels, it was easy to see that overexpressed SUMO1P3 facilitated the expression of c-myc and CCND1, while the down-modulation of SUMO1P3 exerted a depressed function on them (Fig. 5D and E). To further understand the interaction between SUMO1P3 and CNBP, CNBP binding motifs were searched by using the online databases based on crosslinking-immunoprecipitation and high-throughput sequencing (CLIP-seq), degradome-seq and RNA–RNA interactome, which are focus on RNA–RNA and RNA–RBP interactions, including catRAPID (http://s.tartaglialab.com/page/largeRNAs_group), Encyclopedia of RNA Interactomes (ENCORI, http://starbase.sysu.edu.cn/) and RNA-Protein Interaction Prediction (RPISeq, http://pridb.gdcb.iastate.edu/RPISeq/). From these databases, we revealed that CNBP display a high affinity with A/G-rich motifs on RNA sequences, based on which we noticed that, at the positions 184–190 in SUMO1P3 sequence, there was a motif “GAAGGAA” (Fig. 5F and G) that is just located in region 1 (82-233).
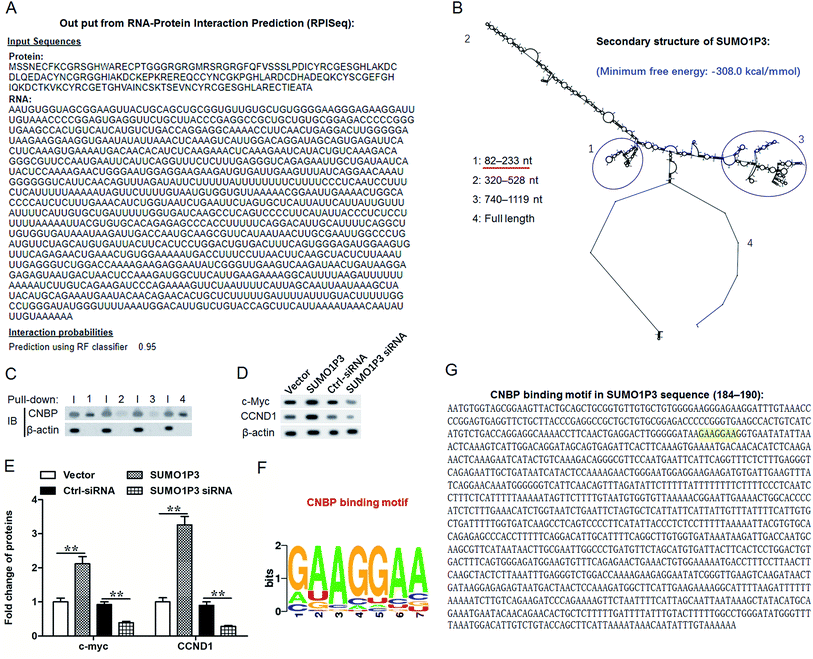 |
| Fig. 5 SUMO1P3 could bind to CNBP at a specific motif and effect the expressions of c-myc and CCND1. (A) The output from RPISeq presented the interaction probability of SUMO1P3 and CNBP. (B) Graphic illustration of SUMO1P3 secondary structure prediction and the SUMO1P3 truncation diagram based on the stem-loop structure were obtained by the utilization of RNAstructure software. (C) Verification result of SUMO1P3 and CNBP interaction was achieved using RNA pull-down assay. (D and E) SGC-7901/5-Fu cell line transfected by siRNAs and pcDNA vectors was used for determination of target protein expression, and the visualized and quantized expressions of c-myc and CCND1 proteins were represented using western blot analysis. Data are expressed as means ± SEM from three independent experiments. **P < 0.01 versus vector and SUMO1P3 siRNA, respectively. (F) CNBP binding motif. (G) The position of CNBP binding motif in SUMO1P3 sequence (highlighted). | |
To sum up, SUMO1P3 and CNBP are both upregulated during tumorigenesis of gastric cells, and they bind with each other through a covalent bond; then, the proto-oncogenes c-myc and CCND1 are upregulated, which causes an increase in drug resistance and cell proliferation (Fig. 6).
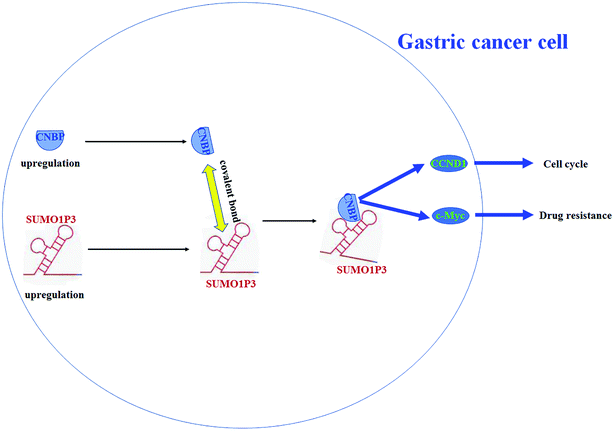 |
| Fig. 6 The scheme for the role of SUMO1P3 and CNBP protein during progression of gastric cancer and their interaction. SUMO1P3 and CNBP are both upregulated during tumorigenesis of gastric cells. They bind with each other through a covalent bond and upregulated the downstream proto-oncogenes c-myc and CCND1 that promoted drug resistance and cell proliferation of gastric cells. | |
3 Discussion
GC is reported to increase the cancer mortality worldwide to a large extend, but it has not been successfully managed especially in advanced stages.28 However, accumulating progresses have been executed to discover and delineate novel biomarkers with high sensitivity and specificity which can be applied to the clinical application of GC prevention, diagnosis and therapy.29 SUMO1P3, as an upregulated lncRNA in certain cancers, has been validated to target to the treatment of several type cancers, GC included.6,14,17,30 In the present study, we confirmed the significant upregulation of SUMO1P3 in gastric tissues of GC patients; besides, tissue samples in advanced T and N stages presented high expression of SUMO1P3, and the patients with high SUMO1P3 expression survived worse than those with lower SUMO1P3 expression. The consistent evidence has also been documented in the research about breast cancer.14 With regard to the of determination in GC cell lines, our results made known that cell lines with high metastatic capacity showed higher SUMO1P3 expression compared with those had moderate metastatic capacity; in other words, we achieved the consequence in agreement with Zhan and colleagues that SUMO1P3 had relevant to cancer metastasis.6 These results verified that elevated level of SUMO1P3 expression might serve a new prognostic and therapeutic biomarker of GC.
In recent years, in order to explore the function of SUMO1P3 in cancer cell progression, many efforts had been made and numerous species of evidence had been obtained. SUMO1P3 was demonstrated to have the ability of cell growth and metastasis induction in bladder cancer.6 In breast cancer, SUMO1P3 played a stimulating role of cell proliferation, migration and invasion.14 Furthermore, SUMO1P3 had association with the proliferative, migratory, invasive and pro-angiogenic abilities of colon cancer cells.17 Similarly, SUMO1P3 presented a facilitating function on cell proliferation, migration and invasion of pancreatic cancer.31 To the best of our knowledge, this is the first report of how SUMO1P3 functions on the progress of GC cell. In the present study, the knockdown of SUMO1P3 by siRNA presented a dose-dependent inhibited part in the proliferation and invasion of GC cells; howbeit, overexpressed SUMO1P3 predominantly enhanced the proliferative and invasive abilities of GC cells. In general, SUMO1P3 was indicated to facilitate gastric cancerous cellular proliferation and invasion.
Nowadays, chemotherapy combining with surgery gradually becomes one of the most common methods for GC treatment. The development of molecular-targeted drugs has promoted the wide acceptance of molecular-targeted treatment of cancers.32 However, drug resistance limits the effectiveness of these drugs in clinical therapy.33 During the course of exploration on the mechanism of drug resistance, the expression of lncRNA was proposed to have relevance with cancerous drug resistance. Taken as an example, the silenced lncRNA HOTAIR improved the drug sensitivity to DDP and thus decreased the drug resistance of non-small cell lung cancer.34 The study focused on anaplastic thyroid cancer discovered that lncRNA papillary thyroid carcinoma susceptibility candidate 3 (PTCSC3) could affect its drug resistance.35 Additionally, the overexpression of lncRNA UCA1, abbreviation of urothelial carcinoma associated 1, apparently increased the IC50 of adriamycin (ADR), DDP and 5-Fu; that was to say, lncRNA urothelial carcinoma associated 1 (UCA1) heightened the multi-drug resistance of GC.36 In accordance with these previous studies, except for the highly upregulated expression of SUMO1P3 had been detected in both SGC7901 cells which were resistant to DDP and 5-Fu, we also found that SUMO1P3 increased the IC50 value for DDP and 5-Fu, and suppressed the drug sensitivities to these two drugs. These clues indicated that SUMO1P3 is a crucial player in drug resistance of GC cells.
SUMO1P3 could modulate the proliferation and invasion, and influence the drug resistance of GC cells, subsequently, we investigated the potential function mechanism of SUMO1P3. According to a previous study, CNBP, well known as a highly conserved zinc-finger protein, was suggested to have a contradictory effect on transcriptional and post-transcriptional regulation.25 Apart from that, CNBP could exert as combining chaperone with both single strand DNA and RNA.23,37,38 CNBP was also provided as a mediator between lncRNA assisted stabilization of transcript (LAST) and CCND1.27 The modes of lncRNAs binding with RNA binding proteins are being revealed. In these modes, both molecules of the interaction contain some special motifs. They bind with each other and form covalent bonds at the position of the motifs. Therefore, the motifs are an important characteristic for the binding between lncRNAs and proteins and also an important cut-in point for studying RNA–protein interaction. Existing reports have revealed that the motifs in the sequence of lncRNA molecules usually contain special repetitive sequences or are rich in some nucleotides. For example, as a lncRNA interacting with CNBP, LAST contains G-rich motifs, a type of motif that CNBP prefers to bind.27 Here, our secondary structure analysis showed that SUMO1P3 displayed a relatively stable secondary structure with a minimum free energy of about −308.0 kcal mol−1 and three stem-loop structures at different ends. On one of the ends, there is an A/G-rich motif (GAAGGAA) through which we estimated that SUMO1P3 form covalent bonds with the zinc finger motif on CNBP protein. Our pull-down assay validated the interaction between SUMO1P3 and CNBP through the binding motif.
For the further explorations on the mechanism of SUMO1P3 functions in GC cell behaviors, we proposed two genes, c-myc and CCND1. Among them, the transcription of c-myc was reported to be regulated by CNBP through the nuclease hypersensitive element (NHE) III1 region.25 Besides, studies had demonstrated that c-myc is recognized as a molecular hallmark of carcinogenesis due to its common activation in great majority of human cancers.39 With regard to the target genes of c-myc, CCND1 was a typical one which in charge of cell cycle, and the abnormal expression of CCND1 could result in tumor diseases due to the derangements of cell cycle and proliferation.40 Furthermore, it had been validated that lncRNA LAST interacted with CNBP, then bind to the 5′-untranslated region (5′-UTR) of CCND1 and promote tumorigenesis.27 In our present study, the fold change of proteins in SUMO1P3 interfered and overexpressed SGC-7901 cells suggested that the expression of SUMO1P3 positively regulated the protein expressions of c-myc and CCND1. The aforementioned results indicated that SUMO1P3 could regulate the expression of c-myc and CCND1, via directly binding to CNBP, thus regulating the development of GC. But the further studies were still necessary for affirmation.
4 Conclusion
SUMO1P3 is upregulated in GC tissues and cell lines, and patients with high level of SUMO1P3 displayed a poor survival rate. SUMO1P3 promotes proliferation and invasion of GC cells and increased the resistance of GC cells to cisplatin (DDP) and 5-fluorouracil (5-Fu) through directly interacting with the CNBP protein and positively regulating oncogenes c-myc and CCND1. Our findings reveal a potential prognostic biomarker and a novel therapeutic target for GC.
5 Materials and methods
5.1 Patient tissue samples
All experiments were performed in accordance with the Guidelines of the First People's Hospital of Shangqiu City (Shangqiu, China), and experiments were approved by the ethics committee at the First People's Hospital of Shangqiu City. Informed consents were obtained from human participants of this study. The GC tissue samples we obtained were derived from 69 GC patients who suffered surgical resection but not received previous chemotherapy or radiotherapy at our hospital during May 2016 to Aug 2017, also we collected the paired normal gastric tissues of every individual. After immediately frozen by liquid nitrogen, the samples were stored at −80 °C for the future participations. Before the conduction of this research, informed consent for the sampling and the follow-up studies was acquired from all the patients. Besides, the patients' personal information was anonymous to us. Referring the clinical stages, we executed in line with the protocols of the American Joint Committee on Cancer Staging System.
5.2 Cell culture and drug treatment
The human normal gastric epithelial cells (RGM-1 and GES-1) and GC cell lines (MGC-803, BGC-7901, MKN-45 and HS-746T) utilized in the present study were purchased from the Institute of Cell Research, Chinese Academy of Sciences, Shanghai, China. Short tandem repeat analysis was conducted for the verification of cell lines' authenticity. Cells were cultured in Roswell Park Memorial Institute 1640 medium (RPMI-1640; Gibco, BRL, Carlsbad, CA, USA) with the supplementation of 100 U mL−1 penicillin, 10% fetal bovine serum and 100 mg mL−1 streptomycin (all provided by Sigma-Aldrich, St. Louis, MO, USA), and were incubated in a 37 °C incubator with a humidified atmosphere of 5% CO2.
For the drug resistance experiments, we referred a predecessor's research to conduct a habitual stepwise method to generate cisplatin (DDP) and 5-fluorouracil (5-Fu) resistant SGC-7901 cell line.41 DDP and 5-Fu reagents (Sigma-Aldrich, St. Louis, MO, USA) were prepared for the incubation of cultured cells those treated and non-treated with siRNA or pcDNA vector.
5.3 RNA extraction and reverse transcription-qPCR (RT-qPCR)
We extracted total RNA according to the instruction of TRIzol solution (Promega, Madison, Wisconsin, WI, USA). Then, we utilized a PrimeScript RT reagent Kit (Takara, Dalian, China) to reverse-transcribed RNA to cDNA. Using specific primers, the gene expressions were qualified by RT-qPCR based on a commercial kit, SYBR® Premix DimerEraser Kit (Takara, Dalian, China), β-actin as control. PCR program was conducted on a Real-Time PCR System (ABI7500, Applied Biosystems, Waltham, MA, USA) and the detailed process went as follows: 1 min at 95 °C, 20 s at 95 °C and 10 s at 56 °C and 15 s at 72 °C for 35 cycles, then held at 4 °C. Finally, the calculation of gene expression was achieved by using the 2−ΔΔCt method.
5.4 Knockdown, overexpression assays and cell transfection
We got the synthetic service of SUMO1P3 siRNA and control (ctrl) siRNA from Invitrogen (Carlsbad, CA, USA). Meanwhile, to overexpress SUMO1P3, we cloned SUMO1P3 from genomic DNA; moreover, we chose pcDNA3.1 (Thermo Fisher Scientific Inc., Waltham, MA, USA) plasmid as overexpression vector and inserted SUMO1P3 onto it.
Before transfection, the cultured cells were seeded at the density of 5 × 105 cells per well in 6 well plates for reaching an approximate confluence of 80%. According to the manufacturer instruction of Lipofectamine Plus reagent (Invitrogen, Carlsbad, CA, USA), we transfected cells with 20 nM or 50 nM SUMO1P3 siRNA and 1.2 μg mL−1 pcDNA-SUMO1P3.
5.5 Cell proliferation and invasion assays
Cell proliferation after 0 h, 24 h and 72 h siRNA transfection was measured, using Cell Counting Kit-8 (CCK-8; Dojindo Molecular technologies, Inc., Kyushu, Japan), according to the manuscript instruction. Finally, we detected the absorbance at 450 nm wavelength using xMark Microplate Absorbance Spectrophotometer (Bio-Rad, Hercules, CA).
For evaluation of cell invasion, a 24-well transwell chamber (Coring Costar, Cambridge, MA, USA) was used in the light of manuscript's protocol. The counting of cells invading the membrane was manipulated in at least five random fields through a microscopy. The final data was presented as a percentage of invaded cells.
5.6 Determination of drug sensitivity of the cells
We conducted One Solution Cell Proliferation Assay (MTS) assay to detect the drug sensitivity of cells. Firstly, cells were incubated overnight after seeding 0.1 mL of 5 × 104/mL cells into 96 well plates. The DDP reagents at varied concentrations were added to the wells and incubated cells for another 72 h. After that, we added CellTiter 96 Aqueous One Solution Reagent (Promega, Madison, Wisconsin, WI, USA) for 4 h incubation in line with the instruction. The final data was measured by a BioRad microplate-reader (Bio-Rad, Hercules, CA) at 490 nm.
5.7 Bioinformatics analysis
An online database developed and operated by IOWA State University, RNA-Protein Interaction Prediction (RPISeq) (http://pridb.gdcb.iastate.edu/RPISeq/), was utilized to predict the interaction probability between SUMO1P3 and SUMO1P3 and cellular nucleic acid binding protein (CNBP). The secondary structure of SUMO1P3 was predicted by RNAstructure, a software for RNA secondary structure prediction and analysis which could be reached from Mathews Lab (Web Servers for RNA Secondary Structure Prediction, http://rna.urmc.rochester.edu/index.html). In addition, prediction and search for the specific binding motif of CNBP on SUMO1P3 was achieved by using starBase (http://starbase.sysu.edu.cn/index.php).
5.8 RNA pull-down
We seeded the cultured cells in plates at the density of 1 × 106 followed by transfecting cells with 50 nm biotin-labeled β-actin probe and four probes of different segments intercepted from SUMO1P3. The pre-coating bead manipulation was done with 10 mg yeast tRNA (Ambion, Austin, TX, USA) followed by an incubation at 4 °C for 2 h with rotation. 48 h probe incubation later, 50 μL pre-coated and pre-washed Dyna Beads M-280 Streptavidin beads (Invitrogen, Carlsbad, CA, USA) were mixed with the collected cell lysates of per sample, then the compound was incubated at 4 °C on a rotator for overnight. We centrifuged and washed the beads using 500 mL ice-cold lysis buffer repeated six times to remove dissociative materials. Finally, to detect the protein expression of CNBP from the pulldown samples, western blot assay was conducted.
5.9 Western blotting
Using RIPA lysis buffer (CW Biotech, Beijing, China), we lysed cells for the extraction of total proteins, and the protein concentrations were measured by a BCA protein assay kit (Thermo Fisher Scientific Inc., Waltham, MA, USA). 10% SDS-PAGE was necessary for the separation of protein samples in equal amounts. Then, the complex was transferred and blocked to nitrocellulose membranes (General Electric Co, USA), and antibodies against CNBP, CCND1 and c-myc (dilution of 1
:
500, Cell Signaling Technology, Inc. Danvers, MD, USA) was used. The secondary antibody was horseradish peroxidase at the dilution of 1
:
1000 (Cell Signaling Technology, Inc. Danvers, MD, USA). At last, the bound antibodies were visualized by Odyssey Infrared Imaging System (LI-COR Biosciences, Lincoln, USA). β-Actin was endogenous control to normalize protein expressions. The fold change versus control group was the display for target proteins' relative expression levels.
5.10 Statistical analysis
During the entire experiment, triplicate reactions repeated independently were necessary. The results were presented as a mean ± standard error of the mean (SEM). Besides, we applied the SPSS software package (Statistical Package for the Social Sciences, version 17.0, SPSS Inc. Chicago, IL, USA) to performed statistical analysis. Kaplan–Meier method and the log rank test were respectively conducted for creating survival curves and determining statistical significance. The expression differences of SUMO1P3 tissues in various stages and conditions were compared using Student's t-test and two-way analysis, as well as unpaired t-test. P < 0.05 was considered statistically significant.
Conflicts of interest
The authors have no conflict of interest.
References
- T. Sano, Gastric cancer: Asia and the world, Gastric Cancer, 2017, 20, 1–2 CrossRef PubMed.
- R. L. Siegel, K. D. Miller and A. Jemal, Cancer statistics, Ca-Cancer J. Clin., 2018, 68, 7–30 CrossRef PubMed.
- Y. Nie, K. Wu, J. Yu, Q. Liang, X. Cai, Y. Shang, J. Zhou, K. Pan, L. Sun and J. Fang, A global burden of gastric cancer: the major impact of China, Expert Rev. Gastroenterol. Hepatol., 2017, 11, 651–661 CrossRef PubMed.
- D. C. Lazăr, S. Tăban, M. Cornianu, A. Faur and A. Goldiş, New advances in targeted gastric cancer treatment, World J. Gastroenterol., 2016, 22, 6776–6799 CrossRef PubMed.
- X. Lin, Z. Dexin, D. Rui, P. Yanglin, Z. Lina, S. Shiren, H. Liu, L. Jie and F. Daiming, miR-15b and miR-16 modulate multidrug resistance by targeting BCL2 in human gastric cancer cells, Int. J. Cancer, 2010, 123, 372–379 Search PubMed.
- Y. Zhan, Y. Liu, C. Wang, J. Lin, M. Chen, X. Chen, C. Zhuang, L. Li, X. Wen and Q. Zhou, Increased expression of SUMO1P3 predicts poor prognosis and promotes tumor growth and metastasis in bladder cancer, Oncotarget, 2016, 7, 16038–16048 Search PubMed.
- S. Dhamija and S. Diederichs, From junk to master regulators of invasion: lncRNA functions in migration, EMT and metastasis, Int. J. Cancer, 2016, 139, 269–280 CrossRef PubMed.
- J. Zheng, S. Zhao, X. He, Z. Zheng, W. Bai, Y. Duan, S. Cheng, J. Wang, X. Liu and Z. Gang, The up-regulation of long non-coding RNA CCAT2 indicates a poor prognosis for prostate cancer and promotes metastasis by affecting epithelial-mesenchymal transition, Biochem. Biophys. Res. Commun., 2016, 480, 508–514 CrossRef PubMed.
- Y. Li, G. Zhu, Y. Ma and H. Qu, LncRNA CCAT1 contributes to the growth and invasion of gastric cancer via targeting miR-219-1, J. Cell. Biochem., 2017, 120, 19457–19468 CrossRef PubMed.
- H. Xia, Q. Chen, Y. Chen, X. Ge, W. Leng, Q. Tang, M. Ren, L. Chen, D. Yuan and Y. Zhang, The lncRNA MALAT1 is a novel biomarker for gastric cancer metastasis, Oncotarget, 2016, 7, 56209–56218 Search PubMed.
- X. Feng and S. Huang, Effect and mechanism of lncRNA HOTAIR on occurrence and development of gastric cancer, J. Cell. Biochem., 2017 DOI:10.1002/jcb.26594.
- S. W. Wu, Y. P. Hao, J. H. Qui, D. B. Zhang, C. G. Yu and H. W. Li, High expression of long noncoding RNA CCAT2 indicates poor prognosis of gastric cancer and promotes cell proliferation and invasion, Minerva Med., 2017, 108, 317–323 Search PubMed.
- J. N. Liu and Y. M. Shangguan, Long non-coding RNA CARLo-5 upregulation associates with poor prognosis in patients suffering gastric cancer, Eur. Rev. Med. Pharmacol. Sci., 2017, 21, 530–534 CrossRef PubMed.
- J. Liu, Z. Song, C. Feng, Y. Lu, Y. Zhou, Y. Lin and C. Dong, The long non-coding RNA SUMO1P3 facilitates breast cancer progression by negatively regulating miR-320a, Am. J. Transl. Res., 2017, 9, 5594–5602 Search PubMed.
- H. L. Su and S. L. Li, Molecular features of human ubiquitin-like SUMO genes and their encoded proteins, Gene, 2002, 296, 65–73 CrossRef PubMed.
- Y. Zhou, P. He, X. Xie and C. Sun, Knockdown of SUMO1P3 represses tumor growth and invasion and enhances radiosensitivity in hepatocellular carcinoma, Mol. Cell. Biochem., 2018, 1–10 Search PubMed.
- L. M. Zhang, P. Wang, X. M. Liu and Y. J. Zhang, LncRNA SUMO1P3 drives colon cancer growth, metastasis and angiogenesis, Am. J. Transl. Res., 2017, 9, 5461–5472 Search PubMed.
- Z. H. Siddik, Cisplatin: mode of cytotoxic action and molecular basis of resistance, Oncogene, 2003, 22, 7265–7279 CrossRef PubMed.
- M. Rebucci and C. Michiels, Molecular aspects of cancer cell resistance to chemotherapy, Biochem. Pharmacol., 2013, 85, 1219–1226 CrossRef PubMed.
- X. D. Xiong, X. Ren, M. Y. Cai, J. W. Yang, X. Liu and J. M. Yang, Long non-coding RNAs: an emerging powerhouse in the battle between life and death of tumor cells, Drug Resist. Updates, 2016, 26, 28–42 CrossRef PubMed.
- T. B. Rajavashisth, A. K. Taylor, A. Andalibi, K. L. Svenson and A. J. Lusis, Identification of a zinc finger protein that binds to the sterol regulatory element, Science, 1989, 245, 640–643 CrossRef PubMed.
- I. L. Flink and E. Morkin, Organization of the gene encoding cellular nucleic acid-binding protein, Gene, 1995, 163, 279–282 CrossRef PubMed.
- E. F. Michelotti, T. Tomonaga, H. Krutzsch and D. Levens, Cellular nucleic acid binding protein regulates the CT element of the human c-myc protooncogene, J. Biol. Chem., 1995, 270, 9494–9499 CrossRef PubMed.
- M. Liu, K. U. Kumar, M. M. Pater and A. Pater, Identification and characterization of a JC virus pentanucleotide repeat element binding protein: cellular nucleic acid binding protein, Virus Res., 1998, 58, 73–82 CrossRef PubMed.
- S. Chen, L. Su, J. Qiu, N. Xiao, J. Lin, J. H. Tan, T. M. Ou, L. Q. Gu, Z. S. Huang and D. Li, Mechanistic studies for the role of cellular nucleic-acid-binding protein (CNBP) in regulation of c-myc transcription, Biochim. Biophys. Acta, Gen. Subj., 2013, 1830, 4769–4777 CrossRef PubMed.
- A. Ooi, T. Oyama, R. Nakamura, R. Tajiri, H. Ikeda, S. Fushida and Y. Dobashi, Gene amplification of CCNE1, CCND1, and CDK6 in gastric cancers detected by multiplex ligation-dependent probe amplification and fluorescence in situ hybridization, Hum. Pathol., 2017, 61, 58–67 CrossRef.
- L. Cao, P. Zhang, J. Li and M. Wu, LAST, a c-Myc-inducible long noncoding RNA, cooperates with CNBP to promote CCND1 mRNA stability in human cells, eLife, 2017, 6, e30433 CrossRef PubMed.
- C. Gravalos and A. Jimeno, HER2 in gastric cancer: a new prognostic factor and a novel therapeutic target, Ann. Oncol., 2008, 19, 1523–1529 CrossRef.
- L. Yong, X. Jianzhong, J. Min, N. Lingna, C. Yun and L. Yang, Association Between Functional PSMD10 Rs111638916 Variant Regulated by MiR-505 and Gastric Cancer Risk in a Chinese Population, Cell. Physiol. Biochem., 2015, 37, 1010–1017 CrossRef.
- M. Danyi, S. Haojun, W. Kai, L. Yichao, S. Weiliang, L. Zhong, D. Xiaoyun and G. Junming, Up-regulation of SUMO1 pseudogene 3 (SUMO1P3) in gastric cancer and its clinical association, Med. Oncol., 2013, 30, 709, DOI:10.1007/s12032-013-0709-2.
- C. Tian, Y. Jin and S. Shi, Long non-coding RNA SUMO1P3 may promote cell proliferation, migration, and invasion of pancreatic cancer via EMT signaling pathway, Oncol. Lett., 2018, 16, 6109–6115 Search PubMed.
- Y. Yang, C. Jiang, Y. Yang, L. Guo, J. Huang, X. Liu, C. Wu and J. Zou, Silencing of lncRNA-HOTAIR decreases drug resistance of non-small cell lung cancer cells by inactivating autophagy via suppressing the phosphorylation of ULK1, Biochem. Biophys. Res. Commun., 2018, 497, 1003–1010 CrossRef.
- J. E. Jang, J. I. Eom, H. K. Jeung, J. W. Cheong, J. Y. Lee, J. S. Kim and Y. H. Min, Targeting AMPK-ULK1-mediated autophagy for combating BET inhibitor resistance in acute myeloid leukemia stem cells, Autophagy, 2017, 13, 761–762 CrossRef PubMed.
- F. Guo, Z. Cao, H. Guo and S. Li, The action mechanism of lncRNA-HOTAIR on the drug resistance of non-small cell lung cancer by regulating Wnt signaling pathway, Exp. Ther. Med., 2018, 15, 4885–4889 Search PubMed.
- X. M. Wang, Y. Liu, Y. X. Fan, Z. Liu, Q. L. Yuan, M. Jia, G. Zu-Shi, L. Gu and X. B. Lu, LncRNA PTCSC3 affects drug resistance of anaplastic thyroid cancer through STAT3/INO80 pathway, Cancer Biol. Ther., 2018, 19, 1–18 CrossRef.
- D. Han, W. Fang, R. Zhang, J. Wei, N. D. Kodithuwakku, W. Ma, F. Li and Y. Li, Clematichinenoside protects blood brain barrier against ischemic stroke superimposed on systemic inflammatory challenges through up-regulating A20, Brain, Behav., Immun., 2016, 51, 56–69 CrossRef.
- J. Yasuda, S. Mashiyama, R. Makino, S. Ohyama, T. Sekiya and K. Hayashi, Cloning and characterization of rat cellular nucleic acid binding protein (CNBP) cDNA, DNA Res., 1995, 2, 45–49 CrossRef.
- P. Armas, S. Nasif and N. B. Calcaterra, Cellular nucleic acid binding protein binds G-rich single-stranded nucleic acids and may function as a nucleic acid chaperone, J. Cell. Biochem., 2010, 103, 1013–1036 CrossRef.
- G. Meital, L. Yulin and D. W. Felsher, MYC activation is a hallmark of cancer initiation and maintenance, Cold Spring Harbor Perspect. Med., 2014, 4, 256–260 Search PubMed.
- J. Luo, R. Yan, X. He and J. He, SOX2 inhibits cell proliferation and metastasis, promotes apoptotic by downregulating CCND1 and PARP in gastric cancer, Am. J. Transl. Res., 2018, 10, 639–647 CAS.
- N. Traverso and R. Ricciarelli, Role of glutathione in cancer progression and chemoresistance, Oxid. Med. Cell. Longevity, 2013, 2013, 972913–997245 Search PubMed.
|
This journal is © The Royal Society of Chemistry 2020 |
Click here to see how this site uses Cookies. View our privacy policy here.