DOI:
10.1039/C9RA10069E
(Paper)
RSC Adv., 2020,
10, 10932-10938
Reduction of liquid terminated-carboxyl fluoroelastomers using NaBH4/SmCl3
Received
2nd December 2019
, Accepted 6th January 2020
First published on 17th March 2020
Abstract
Using a simple one-pot method, the reduction of liquid terminated-carboxyl fluoroelastomers (LTCFs) by sodium borohydride and samarium chloride (NaBH4/SmCl3) was successfully realized and liquid terminated-hydroxyl fluoroelastomers (LTHFs) were obtained. The structure and functional group content of LTCFs and LTHFs were analyzed by FTIR, 1H-NMR, 19F-NMR and chemical titration. The results showed that –C
C– and carboxyl groups of LTCFs were reduced efficiently, the reduction rate reached 92% under optimum reaction conditions. Compared with other frequently-used metal chlorides, SmCl3 with a high coordination number could increase the reduction activity of NaBH4 more effectively and the reduction mechanism was explored.
1. Introduction
As an important polymer material, fluoroelastomers have been widely utilized in the field of chemical engineering, machinery and aerospace1–3 due to their inherent resistance to fuel, oil, high temperature and oxidation. The stabilized carbon–fluorine single bond (C–F 485 kJ mol−1) and the shielded effect of fluorine atoms on the main chain4,5 make the chemical properties of fluoroelastomers more stable than other rubbers. Curable liquid fluoroelastomers with lower molecular weight have attracted much attention using solvent-free sealant and adhesive formulators because of their liquidity and plasticity. Low molecular weight LTCFs with Mn ranging from 500 to 10
000 were prepared by oxidative degradation of fluoroelastomers.6,7 However, due to the properties of carboxyl groups, the LTCFs are difficult to react with cross-linking agents at low temperature and have low heat resistance stability,8 which will inevitably affect their comprehensive properties and limit their application fields. Therefore, it is particularly important to convert the carboxyl groups into hydroxyl groups to improve the thermal stability and reduce the curing temperature of liquid fluoroelastomers.
While significant advances had been made in reduction reactions including for carbonyl compounds, these processes often did not reduce carboxyl groups directly.9–12 Because in contrast to ketones,13 aldehydes14 and esters,15 which easily engage in reactions with reductive agents, carboxylic acids are generally unreactive.16 On the other hand, although lithium aluminium hydride was widely used as a strong reductant in carboxyl reduction,17 its poor chemical selectivity, flammability and explosive nature are unfavorable for large-scale production. Kamochi and Kudo reported the reduction of aryl carboxylic acid derivatives and some aliphatic carboxylic acids using SmI2,18,19 however this strategy was low yielding and limited in scope for aliphatic carboxylic acids. Szostak et al.20 developed a method for efficient electron transfer reduction of carboxylic acids using SmI2–H2O–Et3N and achieved excellent yield, the protocol was not suitable for large-scale production because SmI2 was costly and difficult to store.
NaBH4 has attracted much attention due to its applications in reduction in organic chemistry. Compared with lithium aluminium hydride and SmI2, NaBH4, with moderate cost, mild reaction conditions and excellent chemical selectivity, is conducive to safe production. Nonetheless, NaBH4 does not directly reduce the carboxylic acids by reason of its relatively weak reductive activity. To reduce carboxylic acid compounds effectively, many Lewis acids have been developed to improve the reduction activity of NaBH4 including I2,21 ZnCl2,22 LiCl,23 AlCl3,24 CoCl2 (ref. 25) and so on. Although the LTCFs were reduced by NaBH4/I2, the addition of I2 complicated the post-treatment process and the residual iodine would severely affect the stability of products.26 Hence, further systematical studies on the reduction of LTCFs are academically and industrially significant. Rare earth ions with strong positive electricity, high coordination number and outer empty orbit have strong oxygen affinity.27 Therefore, they can complex with ethers, carbonyl compounds, nitrogen compounds and so on.28 The unique electronic and chemical properties of rare earth ions make them have many advantages, for instance, mild reaction conditions, satisfactory selectivity, less environmental pollution and high efficiency catalytic cycles.29 Hence, our interest in a new strategy for reductive transformations by using NaBH4 and rare earth ions led us to introduce NaBH4/SmCl3 as the first reagent to selectively reduce LTCFs. This mainly involves a simple one-pot process with mild reaction conditions and the NaBH4/SmCl3 dosage, reaction temperature, reaction time and organic solvents were systematically studied. To the best of our knowledge, few facile methods have been reported to date for the reduction of carboxylic acids by NaBH4/SmCl3.
2. Experimental section
2.1 Chemicals
Vinylidene fluoride-hexafluoropropylene copolymer (VDF-HFP) with VDF/HFP = 3/1 and Mn = 78
000 was purchased from Chenguang Research Institute of Chemical Industry (China). Hydrogen peroxide (H2O2), potassium biphthalate (C8H5KO4), phenolphthalein, bromothymol blue, potassium hydroxide (KOH), acetone and benzyltriethylammonium chloride (BTEAC) were purchased from Alfa Aesar (China). NaBH4, AlCl3, CoCl2, ZnCl2, CaCl2 and SmCl3 were purchased from Aladdin Industrial Corporation (Shanghai, China). Tetrahydrofuran (THF), 0.1 mol L−1 hydrochloric acid (HCl) and diglyme were purchased from Kermel Reagent (Tianjin, China).
2.2 Synthesis of LTCFs9
LTCFs were prepared through chemically degradation of high molecular weight solid VDF-HFP copolymer. High molecular weight VDF-HFP (100 g) and acetone (300 mL) were added into a 2000 mL flask. The mixture was stirred at room temperature for 24 h till the copolymer was completely dissolved. Under stirring at 0 °C, BTEAC (6.5 g), 30 wt% H2O2 aqueous solution (60 g), 45 wt% KOH aqueous solution (43 g) were added sequently. The reaction mixture was then warmed up to 24 °C and stirred for 7 h. After the reaction, the organic and inorganic phases were separated by filtration. HCl was used to acidify the organic phases and then the mixture was washed by excess deionized water for 3 times. Finally, the deposition was dried under 60 °C for 48 h in a vacuum drying chamber to obtain LTCFs.
2.3 Synthesis of LTHFs
5.000 g LTCFs (2.66% carboxyl content) were dissolved in a mixture of THF (15 mL) and diglyme (15 mL) for 1 h, then 0.447 g NaBH4 was dissolved into the solution under stirring for 1 h at 0 °C. Subsequently, 1.506 g SmCl3 was added into the system and temperature rose to 90 °C. After 6 h, the inorganic impurities were dissolved by 30 mL 0.1 mol L−1 HCl and the mixture was washed by excess deionized water for 3 times. Finally, the deposition was dried under 60 °C for 48 h in a vacuum drying chamber to obtain LTHFs.
2.4 Characterization
ATR-FTIR measurement was performed using a PerkinElmer Instruments Spectrum One. FTIR spectra were obtained at the resolution 4 cm−1 in the range 650–4000 cm−1. The NMR spectra were analyzed by Bruker AC 80 spectrometers (500 MHz for 1H, 470 MHz for 19F) at room temperature, using acetone-d6 as the solvent and TMS as internal standard the references for 1H (or 19F) nuclei. Chemical shifts are reported in ppm.
2.5 Chemical titration
2.5.1 Preparation and calibration of KOH/C2H5OH solution. 0.1 mol L−1 KOH/C2H5OH solution was prepared in volumetric bottle according to the China National Standards GB/T 603-2002 and GB/T 601-2002. 0.2 g of potassium biphthalate and 40 μL phenolphthalein solution (10 g L−1) was dissolved in water (50 mL). KOH/C2H5OH solution was added dropwise into the potassium biphthalate and phenolphthalein aqueous solution and when the aqueous solution changed from colorless to pink, it was the end point of titration. Blank experiment as the comparison was done. The concentration of KOH/C2H5OH standard titration solution was calculated by formula (1): |
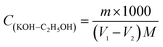 | (1) |
V1: volume of KOH/C2H5OH solution consumed by the potassium biphthalate and phenolphthalein solution (mL), V2: volume of KOH/C2H5OH solution consumed by blank sample (mL), m: weight of potassium biphthalate (g), M: 204.22 g mol−1.
2.5.2 Determination of carboxyl content. 0.75 g of LTCFs and 40 μL bromothymol blue (10 g L−1) was dissolved in acetone (40 mL). KOH/C2H5OH solution was used for titration and the LTCFs and bromothymol blue solution changed from light yellow to green, which was the end point of titration. Carboxyl content of LTCFs was calculated by formula (2): |
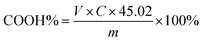 | (2) |
V: volume of KOH/C2H5OH solution consumed by the LTCFs and bromothymol blue solution (mL), C: the concentration of KOH/C2H5OH standard titration solution (mol L−1), m: weight of LTCFs (g).
2.5.3 Determination of hydroxyl content. Reductive rate of hydroxyl was calculated by the LTCFs and LTHFs content of carboxyl (3). |
 | (3) |
where ω0: LTCFs content of carboxyl, ω1: LTHFs content of carboxyl.
3. Results and discussion
3.1 Effect of reaction conditions
Firstly, the effect of temperature on reductive rate is examined. As shown in Fig. 1, the reduction rate of LTCFs increases with the increasing temperature and reaches the maximum at 90 °C. Secondly, the effect of reaction time is also investigated and the results are shown in Fig. 2, the reductive rate of LTCFs increases with increasing reaction time. When reaction time is 6 h, the reductive rate reaches the maximum. It indicates that the optimum reaction temperature is 90 °C and optimum reaction time is 6 h.
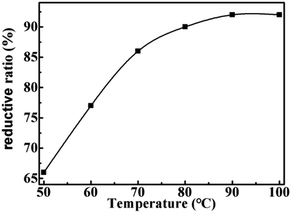 |
| Fig. 1 The effect of temperature on the reductive rate of LTCFs. | |
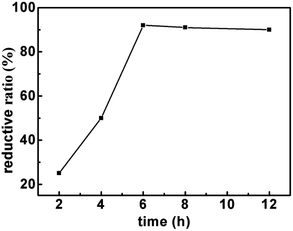 |
| Fig. 2 The effect of time on the reductive rate of LTCFs. | |
3.2 Effect of reduction system proportion
Table 1 shows the effect of NaBH4/SmCl3 system and solvents on the reduction reaction under the optimum reaction temperature and time. We find that on the basis of the chemical titration, the NaBH4/SmCl3 system in THF/diglyme could reduce LTCFs efficiently. As shown in No. 1 and 2 of Table 1, the necessity of each component of the reduction system is investigated. No reductive reaction is observed without NaBH4 and only 12% reduction rate is obtained at the absence of SmCl3, which indicates that SmCl3 has no reducibility to LTCFs and NaBH4 only has little reducibility. However, the reducibility increases significantly when the reductive system is composed of the two above reagents. The reductive rate of LTCFs increases with increasing the NaBH4/SmCl3 dosage and the optimal molar ratio of R0COOH/NaBH4/SmCl3 is 1/4/2 (No. 3–6). When molar ratio of R0COOH/NaBH4/SmCl3 further increases to 1/5/2.5, the reduction rate is decreasing due to the coating of the NaBH4 particles with the excess SmCl3, which likely impedes the efficient solubility of NaBH4 (No. 7). Furthermore, as shown in Fig. 3, the SmCl3 addition amount is investigated. When the molar ratio of NaBH4/SmCl3 changes from 4/0 to 4/2, the reductive rate of LTCFs increases with increasing amount of SmCl3. Nevertheless, when the molar ratio is 4/3, the excess SmCl3 make the reductive rate decrease because the coating of the NaBH4 particles with the excess SmCl3.
Table 1 The effect of NaBH4/SmCl3 system on the reduction of LTCFs
No. |
R0COOH |
NaBH4 |
SmCl3 |
THF/diglyme (mL) |
Reductive rate (%) |
1 |
1 |
0 |
3 |
1/1 (30 mL) |
0 |
2 |
1 |
6 |
0 |
1/1 (30 mL) |
12 |
3 |
1 |
1 |
0.5 |
1/1 (30 mL) |
13 |
4 |
1 |
2 |
1 |
1/1 (30 mL) |
48 |
5 |
1 |
3 |
1.5 |
1/1 (30 mL) |
61 |
6 |
1 |
4 |
2 |
1/1 (30 mL) |
92 |
7 |
1 |
5 |
2.5 |
1/1 (30 mL) |
88 |
8 |
1 |
4 |
2 |
1/0 (30 mL) |
80 |
9 |
1 |
4 |
2 |
2/1 (30 mL) |
85 |
10 |
1 |
4 |
2 |
1/2 (30 mL) |
86 |
11 |
1 |
4 |
2 |
1/1 (20 mL) |
53 |
12 |
1 |
4 |
2 |
1/1 (40 mL) |
59 |
13 |
1 |
4 |
2 |
1/1 (50 mL) |
42 |
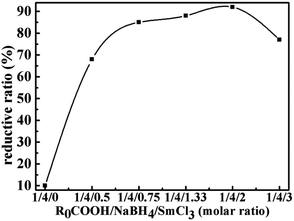 |
| Fig. 3 The effect of amount of SmCl3 on the reductive rate of LTHFs. | |
3.3 Effect of solvent on reductive rate
As shown in Table 1 from No. 8, although the NaBH4/SmCl3 system in THF readily reduces LTCFs to their corresponding LTHFs, it has a lower reduction rate. The low reduction rate is possibly due to the weak of solubility of NaBH4 in THF. To improve the solubility of NaBH4 in THF, varying amounts of diglyme, a known excellent solvent for NaBH4, are added. After some optimizations, the 1/1 volume ratio of THF/diglyme mixed solvent is adequate to solubilize NaBH4 (No. 6, 8–10). In addition, the effect of mixed solvent amount on reduction rate is also investigated (No. 6, 11, 12, 13). The results show that the maximum reduction rate is obtained as the amount of mixed solvent is 30 mL. The lower solvent amount will lead to the decrease solubility of NaBH4 and the excessive use will affect the complexation of NaBH4/SmCl3 with carboxyl group. Consequently, 30 mL of THF/diglyme (1/1 volume ratio) mixed solvent is selected to investigate the reduction of LTCFs.
From above experimental results we can conclude that the optimal reaction conditions are as follows: the reaction temperature is 90 °C, the reaction time is 6 h, the molar ratio of the R0COOH/NaBH4/SmCl3 is 1/4/2 and the THF/diglyme volume ratio is 1/1. Under the optimal reaction conditions the LTCFs are converted to LTHFs in a reductive rate about 92%. For the scale-up, when we expand the experiment by 30 times, the reduction rate do not decrease significantly. Therefore, the method can be expected in industrial utility.
As comparison, several other metal chlorides (MClx) are also evaluated. Through the optimization studies, the optimal reaction conditions of NaBH4/MClx are consistent with NaBH4/SmCl3. As shown in Table 2, only NaBH4/SmCl3 gives excellent reductive rate of LTCFs (No. 1). It is concluded that the unique electronic structure and high coordination number of samarium ions provide a new method for efficient reduction of LTCFs and a potential scheme for the reduction of other carboxyl organic compounds.
Table 2 Comparison of reduction activity between NaBH4/MClx and NaBH4/SmCl3
Reduction system |
Ratio (mol) |
THF/diglyme (mL) |
Reductive rate (%) |
NaBH4/SmCl3 |
4/2 |
1/1 |
92 |
NaBH4/AlCl3 |
4/2 |
1/1 |
64 |
NaBH4/ZnCl2 |
4/2 |
1/1 |
72 |
NaBH4/CaCl2 |
4/2 |
1/1 |
44 |
NaBH4/CoCl2 |
4/2 |
1/1 |
20 |
3.4 Structure characterization
FTIR spectra of LTCFs is shown in Fig. 4(a). It can be seen from Fig. 4(a) that the absorption peaks at 1769, 1686, 1398, 1183, and 879 cm−1 ascribed to stretching vibration of –CF2COOH, –C
C–, –FCH2–, –CF2–, and –CF3, respectively.30,31 Fig. 4(b) shows the FTIR spectra of reduction product by single NaBH4. Comparing the Fig. 4(b) with Fig. 4(a), the absorption peak of –C
C– at 1686 cm−1 is weakened and the absorption peak of –CF2COOH is still obvious (1769 cm−1) indicating that the structure of –C
C– is reduced significantly but only little –CF2COOH is reduced via single NaBH4 without SmCl3. Fig. 4(c) shows the FTIR spectra of LTHFs reduced by NaBH4/SmCl3. From Fig. 4(c) can be seen that the absorption peaks of –C
C– and –CF2COOH are weakened ascribed to effective reduction of NaBH4/SmCl3. LTHFs exhibits absorption peaks at 1398, 1183 and 879 cm−1 ascribed to stretching vibration of –FCH2–, –CF2–, and –CF3, respectively, which clearly shows that LTHFs have the same backbone structure as LTCFs.
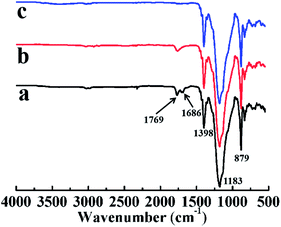 |
| Fig. 4 FTIR spectra of (a) LTCFs, (b) reduction product of single NaBH4 (No. 2) and (c) LTHFs (No. 6). | |
As seen from Fig. 5(a), in the 1H-NMR spectra of LTCFs the multiple peaks at 3.51–2.86 ppm, the peaks at 1.55 ppm, 4.68 ppm and 7.50–7.70 ppm are assigned to the structures of –CH2CF2–, –CF
C(CF3)CH2–, –(CF3)C
CH– and –CH
CF–,32,33 Respectively. Fig. 5(b) shows the disappearance of –C
C– peaks at 1.55 ppm, 4.68 ppm and 7.5–7.70 ppm, respectively, indicating that –C
C– converted to –C–C–. In general, single NaBH4 does not reduce –C
C– and it is commonly necessary to add Lewis acids to improve the reduction activity.34,35 However, –C
C– of LTCFs can be directly reduced by single NaBH4 ascribed to effect of F atom, which increases the activity of –C
C– through the electronic induction effect. Compared with the LTCFs, as seen from Fig. 5(c), LTHFs exhibit new peaks at 3.63 ppm and 3.75 ppm, which are assigned to the structure of –CH2OH,30 it clearly confirms the formation of hydroxyl groups.
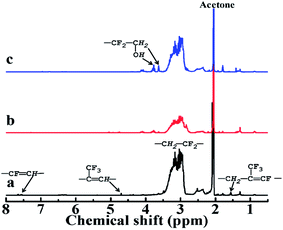 |
| Fig. 5 1H-NMR spectra of (a) LTCFs, (b) reduction product of single NaBH4 reductant (No. 2) and (c) LTHFs (No. 6). | |
19F-NMR spectra were used further to characterize. 19F-NMR spectra of (a) LTCFs, (b) reduction product of single NaBH4 reductant (No. 2) and (c) LTHFs (No. 6) are shown in Fig. 6. The assignments of peaks are listed in Table 3. As seen from Fig. 6(a), a peak at 63.67 ppm is ascribed to –CF2COOH. The peaks at −73.71 ppm, 80.66 ppm and 81.30 ppm are ascribed to the fluorine atoms on the structure of –(CF3)C
CH–, –CH
CF– and –CF
C(CF3)CH2– for LTCFs36–38 respectively. Fig. 6(b) shows that –C
C– peaks at −73.71 ppm, 80.66 ppm and 81.30 ppm disappear by reductive transformation using NaBH4, this result is similar to Fig. 5(b). Fig. 6(c) clearly shows new peak at −104.9 ppm, which is assigned to –CF2CH2OH.30 Therefore, the above results of 19F-NMR spectra, 1H-NMR and FTIR spectra consistent with each other and LTHFs is prepared successfully. 19F-NMR spectra of LTHFs in different reaction times is shown in Fig. 7. It can be seen from Fig. 7 that no byproducts are detected in all times.
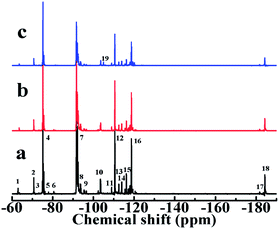 |
| Fig. 6 19F-NMR spectra of (a) LTCFs, (b) reduction product of single NaBH4 reductant (No. 2) and (c) LTHFs (No. 6). | |
Table 3 Assignments of 19F-NMR peaks in liquid fluoroelastomers
No |
δ (ppm) |
Assignment |
No |
δ (ppm) |
Assignment |
1 |
−63.46 |
–CF2CF2COOH |
11 |
−108.96 |
–CF(CF3)CH2CF2CF(CF3)CF2– |
2 |
−70.67 |
–CH2CF2CF(CF3)CF2CH2– |
12 |
−110.51 |
–CF2CH2CF2CF2CF(CF3)– |
3 |
−73.71 |
–CF2CH C(CF3)CF2– |
13 |
−112.53 |
–CF(CF3)CH2CF2CF2CH2– |
4 |
−75.19 |
–CF2CH2CF(CF3)CF2CF2– |
14 |
−113.95 |
–CF2CH2CF2CF2CH2– |
5 |
−80.66 |
–CH CFCF(CF3)– |
15 |
−116.24 |
–CH2CH2CF2CF2CF(CF3)– |
6 |
−81.30 |
–CF CHCF(CF3)CF2– |
16 |
−118.72 |
–CH2CF2CF2CF(CF3)CH2– |
7 |
−91.62 |
–CF2CH2CF2CH2CF2– |
17 |
−181.74 |
–CH2CF2CF(CF3)CF2CH2– |
8 |
−93.56 |
–CF2CH2CF2CH2CF(CF3)– |
18 |
−184.33 |
–CF2CF2CF(CF3)CH2CF2– |
9 |
−95.64 |
–CH2CH2CF2CH2CF2– |
19 |
−104.90 |
–CF2CH2OH |
10 |
−103.62 |
–CF2CH2CF2CF(CF3)CF2– |
|
|
|
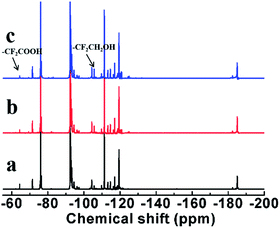 |
| Fig. 7 19F-NMR spectra of LTHFs in different reaction times ((a) 1 h, (b) 3 h, (c) 5 h). | |
3.5 Reduction mechanism
The reported literatures on reduction mechanism of NaBH4 and Lewis acid has predominantly considered that borane (BH3) was formed by the reaction of NaBH4 with Lewis acid initially, and then BH3 reduced the substrates.21,39 To investigate the applicability of this reduction mechanism in our experiment for LTCFs, the sequence of reagent addition is investigated first (Table 4). When NaBH4, SmCl3 and LTCFs are added into the reactor simultaneously, the reduction rate of LTCFs is 33% (No. 1). When SmCl3 and LTCFs are first added into the reactor under stirring for 1 h, then, NaBH4 is added and the reduction rate is 48% (No. 2). The above two addition sequence experiments can ensure the reaction of NaBH4 with SmCl3 firstly and have low reduction rate indicating the mechanism is not suitable for LTCFs. Nevertheless, when NaBH4 and LTCFs are first added into the reactor under stirring for 1 h, then SmCl3 is added (No. 3), the reduction rate increase dramatically to 92%. Hence, according to our experimental results and literatures,20,40 the reduction mechanism of LTCFs is proposed, as shown in Scheme 1. Firstly, –C
C– of LTCFs is directly reduced by NaBH4 and R0COOH react with NaBH4 to form (R1COO)4BNa and release H2. Secondly, the complexation of Sm3+ with carbonyl oxygen of LTCFs makes the electrons of carbonyl group move to oxygen and increase the electrophilicity of carbonyl carbon. Electrophilicity of carbonyl carbon is facilely attacked by the borohydride anion (BH4−) of NaBH4.40 Thirdly, with the departure of Sm3+ and the other oxygen atom, aldehyde group is formed.20 Aldehyde group is then reduced by NaBH4/SmCl3 and the LTCFs is successfully reduced to the final formation of LTHFs. In contrast to carboxyl group, aldehyde group is readily reactive. The generated aldehyde group in the reaction could be immediately reduced by the strong reduction system of NaBH4/SmCl3. Therefore, no byproduct of liquid terminated-aldehyde fluoroelastomers is generated.
Table 4 Effect of charging sequence on the reduction of LTCFsa
No. |
Step 1a |
Step 2b |
Reductive rate (%) |
First, chemical reagent of Step 1a were added into the reactor and reacted 1 h. Then, chemical reagent of Step 2b was added for reaction. |
1 |
NaBH4, SmCl3, LTCFs |
— |
33 |
2 |
SmCl3, LTCFs |
NaBH4 |
48 |
3 |
NaBH4, LTCFs |
SmCl3 |
92 |
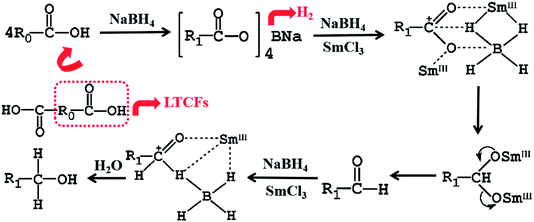 |
| Scheme 1 Scheme of reduction mechanism of LTCFs (R0 is composed of –CH2CF2–, –CF2CF(CF3)–, –CFCHCHF(CF3)–, –CF2C(CF3)CHCF2–, and –CFCH–; R1 is composed of –CH2CF2– and –CF2CF(CF3)–). | |
4. Conclusion
In summary, the combination of affordable and readily available NaBH4/SmCl3 provided a method for the selective reduction of LTCFs in THF/diglyme and the 1/4/2 molar ratio of R0COOH/NaBH4/SmCl3 was suitable for LTCFs reduction at 90 °C. LTCFs were reduced to their corresponding LTHFs in excellent reduction rate (92%). FTIR spectra and NMR spectra analysis showed that –C
C– and –CF2COOH of LTCFs were effectively reduced to –C–C– and –CF2CH2OH. We have proposed the reduction mechanism that Sm3+ complex with the carbonyl group increase the electroaffinity of the carbon of carbonyl and make carbon of carbonyl more receptive to the hydride moiety transfer from the borohydride anion. Application of this method to reduction of other carboxylic acid derivatives will be presented in our future work.
Conflicts of interest
The authors declare no competing financial interests.
Acknowledgements
The authors gratefully acknowledge the financial support of National Natural Science Foundation of China (Grant No. 51873026).
References
- P. Ferrandez, Fluoroelastomers, FKM and FEPM, in Handbook of Specialty Elastomers, ed. C. K. Robert, Taylor & Francis Group, New York, 2008, pp. 133–154 Search PubMed.
- B. Ameduri, Controlled radical (co)polymerization of fluoromonomers, Macromolecules, 2010, 43, 10163–10184 CrossRef CAS.
- B. Ameduri, From vinylidene fluoride (VDF) to the applications of VDF-containing polymers and copolymers: recent developments and future trends, Chem. Rev., 2009, 109, 6632–6686 CrossRef CAS PubMed.
- B. Ameduri and B. Boutevin, Well-architectured fluoropolymers: synthesis, properties and applications, Elsevier, Amsterdam, 2004, pp. 187–230 Search PubMed.
- B. Ameduri, B. Boutevin and G. Kostov, Fluoroelastomers: synthesis, properties and applications, Prog. Polym. Sci., 2001, 26, 105–187 CrossRef CAS.
- D. H. Li and M. Y. Liao, Dehydrofluorination mechanism, structure and thermal stability of pure fluoroelastomer (poly(VDF-ter-HFP-ter-TFE) terpolymer) in alkaline environment, J. Fluorine Chem., 2017, 201, 55–67 CrossRef CAS.
- D. H. Li and M. Y. Liao, Study on the dehydrofluorination of vinylidene fluoride (VDF) and hexafluoropropylene (HFP) copolymer, Polym. Degrad. Stab., 2018, 152, 116–125 CrossRef CAS.
- J. Li, Y. Lu, Y. Liu, Y. Li, X. Zhang and S. Qi, Synthesis, characterization, curing and properties of carboxyl-terminated liquid fluoropolymers, Polym.-Plast. Technol. Eng., 2014, 53, 46–53 CrossRef CAS.
- N. Findlay, S. R. Park, F. Schoenebeck, E. Cahard, S. Zhou, L. E. A. Berlouis, M. D. Spicer, T. Tuttle and J. A. Murphy, Reductions of challenging organic substrates by a nickel complex of a noninnocent crown carbene ligand, J. Am. Chem. Soc., 2010, 132, 15462–15464 CrossRef CAS PubMed.
- G. Pelletier, W. S. Bechara and A. B. Charette, Controlled and chemoselective reduction of secondary amides, J. Am. Chem. Soc., 2010, 132, 12817–12819 CrossRef CAS PubMed.
- S. Das, D. Addis, S. Zhou, K. Junge and M. Beller, Zinc-catalyzed reduction of amides: unprecedented selectivity and functional group tolerance, J. Am. Chem. Soc., 2010, 132, 1770–1771 CrossRef CAS PubMed.
- S. Das, K. Möller, K. Junge and M. Beller, Zinc-Catalyzed Chemoselective Reduction of Esters to Alcohols, Chem.–Eur. J., 2011, 17, 7414–7417 CrossRef CAS PubMed.
- J. Collins, U. Rinner, M. Moser, T. Hudlicky, I. Ghiviriga, A. E. Romero, A. Kornienko, D. Ma, C. Griffin and S. Pandey, Chemoenzymatic Synthesis of Amaryllidaceae Constituents and Biological Evaluation of their C-1 Analogues. The Next Generation Synthesis of 7-Deoxypancratistatin and trans-Dihydrolycoricidine, J. Org. Chem., 2010, 75, 3069–3084 CrossRef CAS PubMed.
- S. Chandrasekhar and A. Shrinidhi, Synthetic Communications: An International Journal for Rapid Communication of Synthetic Organic Chemistry, Synth. Commun., 2014, 44, 2051–2056 CrossRef CAS.
- C. P. Prasanth, E. Joseph, A. Abhijith, D. S. Nair, I. Ibnusaud, J. Raskatov and B. Singaram, Stabilization of NaBH4 in Methanol Using a Catalytic Amount of NaOMe. Reduction of Esters and Lactones at Room Temperature without Solvent-Induced Loss of Hydride, J. Org. Chem., 2018, 83, 1431–1440 CrossRef CAS PubMed.
- B. S. Bodnar and P. F. Vogt, An Improved Bouveault-Blanc Ester Reduction with Stabilized Alkali Metals, J. Org. Chem., 2009, 74, 2598–2600 CrossRef CAS PubMed.
- M. S. Ganewatta, W. Ding, M. A. Rahman, L. Yuan, Z. Wang, N. Hamidi, M. L. Robertson and C. Tang, Biobased plastics and elastomers from renewable rosin via “living” ring-opening metathesis polymerization, Macromolecules, 2016, 49, 7155–7164 CrossRef CAS.
- Y. Kamochi and T. Kudo, Novel reduction of carboxylic acids, esters, amides and nitriles using samarium diiodide in the presence of water, Chem. Lett., 1993, 22, 1495–1498 CrossRef.
- Y. Kamochi and T. Kudo, Lanthanoids in Organic Synthesis. I. The Novel Reduction of Carboxylic Acids with Samarium Diiodide-Base System, Bull. Chem. Soc. Jpn., 1992, 65, 3049–3054 CrossRef CAS.
- M. Szostak, M. Spain and D. Procter, Electron Transfer Reduction of Carboxylic Acids Using SmI2-H2O-Et3N, J. Org. Lett., 2012, 14, 840–843 CrossRef CAS PubMed.
- P. A. S. Bhanu, J. V. Bhaskar and M. Periasamy, Convenient Methods for the Reduction of Amides, Nitriles, Carboxylic Esters, Acids and Hydroboration of Alkenes Using NaBH4/I2 System, Tetrahedron, 1992, 48, 4623–4628 CrossRef.
- S. Narasimhan, S. Madhavan and K. G. Prasad, Facile reduction of carboxylic acids to alcohols by zinc borohydride, J. Org. Chem., 1995, 60, 5314–5315 CrossRef CAS.
- H. J. Zhu and C. U. Pittman Jr, Reductions of Carboxylic Acids and Esters with NaBH4 in Diglyme at 162 °C, Synth. Commun., 2003, 33, 1733–1750 CrossRef CAS.
- T. Hida, S. Mitsumori, T. Honma, Y. Hiramatsu, H. Hashizume, T. Okada, M. Kakinuma, K. Kawata, K. Oda, A. Hasegawa, T. Masui and H. Nogusa, Practical Diastereoselective Synthesis and Scale-up Study of (+)-2-((1 R, 2 R, 3 R, 5 S)-2-Amino-6, 6-dimethylbicyclo [3. 1. 1] hept-3-yl) ethanol: A Key Intermediate of the Novel Prostaglandin D2 Receptor Antagonist S-5751, Org. Process Res. Dev., 2009, 13, 1413–1418 CrossRef CAS.
- M. Ashot, T. Rafael, G. Sahak, A. Marina, P. Henry, M. Vahan and S. Raymond, Synthesis of 6-imino-5-tetrahydro-1H-2-pyrrolylidenhexahydro-2, 4-pyrimidinediones as intermediates for the synthesis of C-azanucleosides, Tetrahedron Lett., 2010, 51, 231–233 CrossRef.
- S. C. Qi, J. Li, Y. F. Lu, X. A. Zhang, J. D. Wang, Y. P. Li, Y. Li, S. L. Jiang and R. P. Yuan, CN103193919B, 2013.
- E. Murad and D. I. Hildenbrand, Dissociation energies of GdO, HoO, ErO, TmO, and LuO; correlation of results for the lanthanide monoxide series, J. Chem. Phys., 1980, 73, 4005–4011 CrossRef CAS.
- W. A. Herrmann, R. Anwander and W. Seherer, Lanthanoiden-Komplexe, V [1]. Strukturchemie ein- und zweikerniger Seltenerdalkoxide, Chem. Ber., 1993, 126, 1533–1539 CrossRef CAS.
- S. Kobayashi, M. Moriwaki and I. Hachiya, The catalytic Fries rearrangement of acyloxy naphthalenes using scandium trifluoromethanesulfonate as a catalyst, Chem. Commun., 1995, 15, 1527–1528 RSC.
- M. Pianca, E. Barchiesi, G. Esposto and S. Radice, End groups in fluoropolymers, J. Fluorine Chem., 1999, 95, 71–84 CrossRef CAS.
- L. R. Saint, A. Manseri, B. Ameduri, B. Lebret and P. Vignane, Synthesis and properties of novel fluorotelechelic macrodiols containing vinylidene fluoride, hexafluoropropene and chlorotrifluoroethylene, Macromol, 2002, 35, 1524–1536 CrossRef.
- G. J. Ross, J. F. Wattsa, M. P. Hill and P. Morrissey, Surface modification of poly (vinylidene fluoride) by alkaline treatment1. The degradation mechanism, Polym, 2000, 41, 1685–1696 CrossRef CAS.
- S. R. Gondi, A. P. Vogt and B. S. Sumerlin, Versatile pathway to functional telechelics via RAFT polymerization and click chemistry, Macromol, 2007, 40, 474–481 CrossRef CAS.
- B. C. Ranu and S. Samanta, Remarkably Selective Reduction of the α, β-Carbon-Carbon Double Bond in Highly Activated α, β, γ, δ-Unsaturated Alkenes by the InCl3-NaBH4 Reagent System, J. Org. Chem., 2003, 68, 7130–7132 CrossRef CAS PubMed.
- J. M. Khurana and P. B. Sharma, Chemoselective reduction of α, β-unsaturated aldehydes, ketones, carboxylic acids, and esters with nickel boride in methanol-water, Chem. Soc. Jpn., 2004, 77, 549–552 CrossRef CAS.
- P. Wormald, B. Ameduri, R. K. Harris and P. Hazendonk, High-resolution 19F and 1H NMR of a vinylidenefluoride telomer, Polymer, 2008, 49, 3629–3638 CrossRef CAS.
- R. Souzy, B. Ameduri, S. V. Ahsen, H. Willner and G. A. Arguöello, Use of bis (trifluoromethyl) peroxy dicarbonate as initiator in the radical homopolymerisation of vinylidene fluoride (VDF) and copolymerisation of VDF with hexafluoropropylene, J. Fluorine Chem., 2003, 123, 85–93 CrossRef CAS.
- E. M. James, Polymer Data Handbook, New York, Oxford University Press, 1999 Search PubMed.
- J. Z. Saavedra, A. Resendez, A. Rovira, S. Eagon, D. Haddenham and B. Singaram, Reaction of InCl3 with various reducing agents: InCl3-NaBH4-mediated reduction of aromatic and aliphatic nitriles to primary amines, J. Org. Chem., 2012, 77, 221–228 CrossRef CAS PubMed.
- H. C. Brown, S. Narasimhan and Y. M. Choi, Selective Reductions. 30. Effect of Cation and Solvent on the Reactivity of Saline Borohydrides for Reduction of Carboxylic Esters. Improved Procedures for the Conversion of Esters to Alcohols by Metal Borohydrides, J. Org. Chem., 1982, 47, 4702–4708 CrossRef CAS.
|
This journal is © The Royal Society of Chemistry 2020 |
Click here to see how this site uses Cookies. View our privacy policy here.