DOI:
10.1039/D0RA01978J
(Paper)
RSC Adv., 2020,
10, 15614-15621
A PANI-Fe3O4@ZnO nanocomposite: a magnetically separable and applicable catalyst for the synthesis of chromeno-pyrido[d]pyrimidine derivatives†
Received
1st March 2020
, Accepted 31st March 2020
First published on 21st April 2020
Abstract
We report herein green, practical, PANI-Fe3O4@ZnO-nanocomposite-catalyzed cyclocondensation reactions involving 4-aminocoumarin, 1,3-dimethylbarbituric acid, and aromatic aldehydes in an aqueous medium at room temperature to synthesize 9,11-dimethyl-7-aryl-6H-chromeno[3′,4′:5,6]pyrido[2,3-d]pyrimidine-6,8,10(9H,11H)triones. This research aims to provide an applicable and high-yield protocol that follows the principles of green chemistry, with the use of water as an environmentally benign medium and the PANI-Fe3O4@ZnO nanocomposite as a magnetically recoverable catalyst.
1. Introduction
Current questions about the use and benefits of continuous flow chemistry,1 including the elaborate design and development of sequences for the synthesis of alternative structurally complex molecules with structural diversity and eco-congeniality, are of great interest to organic chemists.2 The use of multicomponent reactions (MCRs),3 which involve important contributions resulting from the combination of a minimum of three reactants (or a reactant with three reaction centers) in a single step to form a product that incorporates frameworks containing substantial elements from all the reactants,4,5 is a well-recognized tool in macrocyclization strategies, peptide cyclization, and the diversity-oriented derivatization of complex fused heterocycles.6–15
Magnetic nanoparticles (MNPs) have been attracted significant attention due to their wide range of various usages, such as in cancer treatment,16 drug release,17 and the simplification of catalyst separation. MNP-supported catalysts have also been extensively used in organic transformations.18–23 Recent intensive developments in the field of supported nanomaterials have mainly focused on polymer-bound nanostructures due to their essential applications in photocatalysis,24,25 superhydrophobic materials,26 organic conductors, electromagnetic interference shielding, etc.27 Polyaniline (PANI) is a highly promising polymer for developing novel catalyst supports owing to its energy band gap of 2.8 eV,28 good stability, corrosion protection, nontoxicity, great intrinsic redox potential, and facile and low-cost synthesis.29 The chromene nucleus is a well-known scaffold and an important feature of diverse natural products and medicinal agents.30 Compounds carrying the chromene motif have been reported to possess diverse biological properties, such as antibacterial,31,32 antimicrobial,33–36 antioxidant,37,38 antivascular, antitumor,39 hypotensive,40 anticancer,41–44 local anesthetic, and antiarrhythmic activities.45 Additionally, some chromene-bearing compounds have been previously found to have neurogenic and cognition-enhancing activities.46 Pyridine is a nitrogen-containing basic heterocycle found in various synthetic and naturally occurring compounds that have been shown to possess promising agrochemical and pharmaceutical properties.47
As a part of ongoing studies into the synthesis of biologically significant heterocycles catalyzed by various inorganic nanosized materials,48–50 we explored a low-cost and ecofriendly cyclocondensation reaction for the synthesis of 9,11-dimethyl-7-aryl-6H-chromeno[3′,4′:5,6]pyrido[2,3-d]pyrimidine-6,8,10(9H,11H)trione derivatives in the presence of water as a green solvent and a PANI-Fe3O4@ZnO nanocomposite as a applicable heterogeneous catalyst at room temperature. The synthetic pathway is presented in Scheme 1.
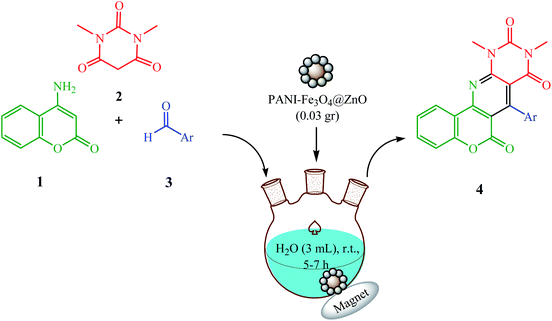 |
| Scheme 1 The synthesis of 9,11-dimethyl-7-aryl-6H-chromeno[3′,4′:5,6]pyrido[2,3-d]pyrimidine-6,8,10(9H,11H)trione derivatives. | |
2. Experimental
2.1. Materials and methods
All of the chemical materials used in this work were purchased from Merck and Fluka and were used without further purification. Melting points were determined using Electrothermal 9100 apparatus. IR spectra were obtained using an ABB FT-IR (FTLA 2000) spectrometer. 1H NMR and 13C NMR spectra were recorded using a Bruker DRX-500 AVANCE spectrometer at 500 and 125 MHz, respectively, using TMS as an internal standard and DMSO-d6 as a solvent. Elemental analyses were carried out using Foss-Heraeus CHN–O-rapid analyzer instruments. Powder X-ray diffraction data were obtained with a Rigaku D-max C III X-ray diffractometer using Cu Kα radiation (λ = 1.54 Å). The microscopic morphology of the catalyst was revealed using a scanning electron microscope (SEM, Philips, XL-30) equipped with energy dispersive X-ray analysis (EDX) apparatus. The microscopic morphology of the catalyst was studied using transmission electron microscopy (TEM) techniques on a Philips CM300 microscope operating with a 100 kV electron beam accelerating voltage.
2.2. Preparation of Fe3O4 nanoparticles
Fe3O4 magnetic nanoparticles (Fe3O4 MNPs) were prepared under solvothermal conditions. Firstly, a mixture of iron(III) chloride (0.6 g), ammonia (7 mL), and glycerol (25 mL) was magnetically stirred at 20 °C for 15 min. A reaction mixture color change from orange to deep brown occurred. Then, the resultant mixture was irradiated ultrasonically for 10 min. Then, the mixture was removed from the ultrasonic bath and magnetically stirred for 30 min. The obtained brown suspension was then placed into an autoclave and heated at 185 °C for 8 h. Then, the autoclave was cooled down gradually to room temperature. The synthesized Fe3O4 MNPs were separated using an external magnet, washed several times with distilled water, and dried under an air atmosphere for two days.
2.3. Preparation of the Fe3O4–ZnO nanocomposite
The synthesis of a core–shell nanostructured Fe3O4–ZnO nanocomposite with an iron oxide (Fe3O4) core and zinc oxide (ZnO) shell was carried out via a solvothermal process. First, a precursor solution was obtained from dissolving 4 g of Fe3O4 MNPs in 50 mL of distilled water, and this was magnetically stirred at room temperature for 20 min. Next, a mixture of polyvinyl pyrrolidone (PVP) (0.5 g), urea (3.8 g), and zinc nitrate (3 g) was added to the above solution under strong magnetic stirring. The obtained mixture was magnetically stirred at 90 °C for 1 h. The produced dark brown composite was then separated using an external magnet, washed with distilled water and ethanol several times, and dried in an oven at 80 °C for one day. The Fe3O4–ZnO nanocomposite powder was finally calcined for 2 h at 500 °C with a heating rate of 10 °C min−1.
2.4. Preparation of the PANI-Fe3O4@ZnO nanocomposite
The as-prepared Fe3O4–ZnO nanocomposite powder (1 g) was added to 30 mL of 1 M HCl, and this was magnetically stirred at room temperature for 30 min. To the above suspension, 3 mL of aniline, 15 mL of 1 M HCl, and a solution of ammonium persulfate (APS) (0.03 g) in distilled water (15 mL) were then added dropwise at 5 °C, respectively. Then, the resultant mixture was magnetically stirred at room temperature for 5 h. The PANI-Fe3O4@ZnO nanocomposite was collected as a black precipitate using an external magnet, washed with distilled water and ethanol several times, and finally dried in an oven at 60 °C for one day.
2.5. General procedure for the synthesis of compounds 4a–h
A mixture of 4-aminocoumarin (1, 1 mmol), 1,3-dimethylbarbituric acid (2, 1 mmol), aromatic aldehyde 3 (1 mmol), and the PANI-Fe3O4@ZnO nanocomposite (0.03 g) in H2O (3 mL) was stirred at ambient temperature for 5–7 h (see Table 2). After the completion of the reaction, as indicated via TLC (ethyl acetate
:
petroleum ether = 3
:
1), the catalyst was recovered simply from the reaction mixture using an external magnet. For reuse, the catalyst was washed with EtOH and dried in air at ambient temperature for several hours. Then the resultant mixture was filtered and the collected solid was dissolved in 5 mL of hot ethanol. The pure product was obtained via re-crystallization from this solution.
2.6. Reusability of the catalyst
For this purpose, and according to the above general procedure, the model reaction for the synthesis of 4c was selected, and it was consecutively carried out over a number of cycles using the recovered catalyst under the same conditions. After the completion of each run of the reaction, the catalyst was separated easily from the reaction mixture with an external magnet and washed with hot ethanol, and it could be reused at least five times without considerable loss of catalytic activity (Fig. 1).
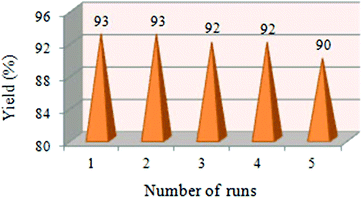 |
| Fig. 1 The reusability of the catalyst. | |
2.7. Spectroscopic data
9,11-Dimethyl-7-phenyl-6H-chromeno[3′,4′:5,6]pyrido[2,3-d]pyrimidine-6,8,10(9H,11H)trione (4a). White solid; yield: 0.354 g (92%); mp > 300 °C. IR (KBr) (νmax/cm−1): 2845, 2732, 1706, 1658, 1618, 1571, 1510, 1450, 1356, 767. 1H-NMR: δ = 3.26 (s, 3H, NCH3), 3.49 (s, 3H, NCH3), 7.47 (d, 3H, J = 7.2 Hz, H–Ar), 7.58 (d, 2H, J = 7.2 Hz, H–Ar), 7.68 (t, 1H, J = 7.2 Hz, H–Ar), 7.75 (d, 1H, J = 8.2 Hz, H–Ar), 7.97 (t, 1H, J = 7.6 Hz, H–Ar), 8.16 (d, 1H, J = 7.6 Hz, H–Ar) ppm. 13C-NMR: δ = 27.1, 29.0, 105.7, 112.5, 113.9, 117.8, 121.4, 124.6, 126.6, 127.2, 129.0, 131.3, 134.6, 135.9, 140.8, 142.9, 150.9, 157.0, 169.0, 173.1 ppm. Anal. calc. for C22H15N3O4 (385.38): C 68.57, H 3.92, N 10.90; found: C 68.69, H 3.82, N 10.81.
9,11-Dimethyl-7-(4-bromophenyl)-6H-chromeno[3′,4′:5,6]pyrido[2,3-d]pyrimidine-6,8,10(9H,11H)trione (4b). White solid; yield: 0.441 g (95%); mp > 300 °C. IR (KBr) (νmax/cm−1): 2937, 2760, 1700, 1666, 1618, 1572, 1508, 1449, 1351, 762. 1H-NMR: δ = 3.25 (s, 3H, NCH3), 3.49 (s, 3H, NCH3), 7.34 (d, 2H, J = 8.2 Hz, H–Ar), 7.59 (d, 1H, J = 7.8 Hz, H–Ar), 7.62 (d, 2H, J = 8.2 Hz, H–Ar), 7.65 (d, 1H, J = 8.7 Hz, H–Ar), 7.88 (t, 1H, J = 7.8 Hz, H–Ar), 8.05 (d, 1H, J = 7.8 Hz, H–Ar) ppm. 13C-NMR: δ = 27.7, 29.1, 105.3, 112.4, 113.1, 117.8, 122.9, 124.6, 125.0, 127.1, 128.3, 129.2, 131.7, 138.9, 140.2, 142.9, 150.7, 157.5, 170.4, 172.9 ppm. Anal. calc. for C22H14BrN3O4 (464.27): C 56.92, H 3.04, N 9.05; found: C 57.05, H 3.21, N 9.15.
9,11-Dimethyl-7-(4-chlorophenyl)-6H-chromeno[3′,4′:5,6]pyrido[2,3-d]pyrimidine-6,8,10(9H,11H)trione (4c). White solid; yield: 0.390 g (93%); mp > 300 °C. IR (KBr) (νmax/cm−1): 2952, 2871, 1689, 1668, 1619, 1570, 1511, 1443, 1355, 752. 1H-NMR: δ = 3.27 (s, 3H, NCH3), 3.50 (s, 3H, NCH3), 7.45 (d, 2H, J = 8.1 Hz, H–Ar), 7.54 (d, 2H, J = 8.1 Hz, H–Ar), 7.63 (t, 1H, J = 7.5 Hz, H–Ar), 7.70 (d, 1H, J = 8.3 Hz, H–Ar), 7.92 (t, 1H, J = 7.6 Hz, H–Ar), 8.11 (d, 1H, J = 7.6 Hz, H–Ar) ppm. 13C-NMR: δ = 27.1, 29.1, 105.4, 112.6, 114.0, 117.8, 124.6, 125.2, 127.4, 129.3, 131.2, 133.4, 134.1, 138.4, 140.8, 142.7, 150.9, 156.9, 168.8, 172.5 ppm. Anal. calc. for C22H14ClN3O4 (419.82): C 62.94, H 3.36, N 10.01; found: C 63.05, H 3.46, N 10.13.
9,11-Dimethyl-7-(4-cyanophenyl)-6H-chromeno[3′,4′:5,6]pyrido[2,3-d]pyrimidine-6,8,10(9H,11H)trione (4d). Pale yellow solid; yield: 0.394 g (96%); mp > 300 °C. IR (KBr) (νmax/cm−1): 2965, 2883, 2223, 1705, 1667, 1619, 1572, 1505, 1450, 1345, 759. 1H-NMR: δ = 3.26 (s, 3H, NCH3), 3.50 (s, 3H, NCH3), 7.73 (d, 2H, J = 8.2 Hz, H–Ar), 7.76 (d, 2H, J = 8.2 Hz, H–Ar), 7.81 (d, 1H, J = 8.3 Hz, H–Ar), 8.05 (m, 2H, H–Ar), 8.21 (d, 1H, J = 7.9 Hz, H–Ar) ppm. 13C-NMR: δ = 27.2, 29.1, 105.2, 110.4, 112.6, 114.5, 117.8, 119.8, 124.0, 125.0, 128.2, 129.4, 134.5, 135.3, 139.1, 140.7, 142.6, 150.9, 156.2, 169.2, 173.0 ppm. Anal. calc. for C23H14N4O4 (410.39): C 67.31, H 3.44, N 13.65; found: C 67.17, H 3.52, N 13.49.
9,11-Dimethyl-7-(3-hydroxyphenyl)-6H-chromeno[3′,4′:5,6]pyrido[2,3-d]pyrimidine-6,8,10(9H,11H)trione (4e). Pale yellow solid; yield: 0.377 g (94%); mp > 300 °C. IR (KBr) (νmax/cm−1): 3436, 2923, 2758, 1678, 1651, 1620, 1570, 1510, 1445, 1353, 756. 1H-NMR: δ = 3.25 (s, 3H, NCH3), 3.51 (s, 3H, NCH3), 7.52 (m, 2H, H–Ar), 7.65 (br s, 2H, H–Ar), 7.73 (t, 1H, J = 7.5 Hz, H–Ar), 7.79 (d, 1H, J = 8.2 Hz, H–Ar), 8.01 (t, 1H, J = 7.8 Hz, H–Ar), 8.21 (d, 1H, J = 7.8 Hz, H–Ar), 9.44 (s, 1H, OH) ppm. 13C-NMR: δ = 27.0, 29.1, 105.5, 112.7, 113.6, 114.1, 114.5, 117.8, 124.4, 124.7, 127.3, 130.0, 133.3, 135.9, 138.4, 140.7, 143.0, 151.0, 155.9, 166.6, 169.2, 173.1 ppm. Anal. calc. for C22H15N3O5 (401.38): C 65.83, H 3.77, N 10.47; found: C 65.68, H 3.59, N 10.30.
9,11-Dimethyl-7-(4-methoxyphenyl)-6H-chromeno[3′,4′:5,6]pyrido[2,3-d]pyrimidine-6,8,10(9H,11H)trione (4f). Yellow solid; yield: 0.395 g (95%); mp > 300 °C. IR (KBr) (νmax/cm−1): 2923, 2874, 1703, 1675, 1619, 1571, 1507, 1440, 1356, 768. 1H-NMR: δ = 3.26 (s, 3H, NCH3), 3.49 (s, 3H, NCH3), 3.68 (s, 3H, OCH3), 7.01 (d, 2H, J = 8.0 Hz, H–Ar), 7.27 (d, 2H, J = 8.02 Hz, H–Ar), 7.59 (t, 1H, J = 7.5 Hz, H–Ar), 7.66 (d, 1H, J = 8.2 Hz, H–Ar), 7.87 (t, 1H, J = 7.8 Hz, H–Ar), 8.06 (d, 1H, J = 7.8 Hz, H–Ar) ppm. 13C-NMR: δ = 27.2, 29.1, 55.7, 105.8, 112.4, 114.0, 117.8, 123.1, 124.6, 125.2, 128.3, 129.5, 133.7, 135.3, 141.0, 142.8, 150.9, 155.9, 158.2, 167.7, 172.9 ppm. Anal. calc. for C23H17N3O5 (415.40): C 66.50, H 4.12, N 10.12; found: C 66.39, H 3.98, N 10.22.
9,11-Dimethyl-7-(4-methylphenyl)-6H-chromeno[3′,4′:5,6]pyrido[2,3-d]pyrimidine-6,8,10(9H,11H)trione (4g). White solid; yield: 0.371 g (93%); mp > 300 °C. IR (KBr) (νmax/cm−1): 3019, 2952, 1701, 1673, 1623, 1571, 1509, 1446, 1356, 767. 1H-NMR: δ = 2.24 (s, 3H, CH3), 3.25 (s, 3H, NCH3), 3.49 (s, 3H, NCH3), 7.28 (br s, 4H, H–Ar), 7.60 (t, 1H, J = 7.4 Hz, H–Ar), 7.68 (d, 1H, J = 8.0 Hz, H–Ar), 7.90 (t, 1H, J = 7.0 Hz, H–Ar), 8.09 (d, 1H, J = 7.0 Hz, H–Ar) ppm. 13C-NMR: δ = 21.4, 27.1, 29.4, 105.6, 113.2, 114.0, 117.8, 122.1, 124.6, 127.1, 129.3, 132.1, 133.3, 136.5, 137.2, 140.9, 142.8, 151.2, 156.0, 168.7, 172.9 ppm. Anal. calc. for C23H17N3O4 (399.40): C 69.17, H 4.29, N 10.52; found: C 69.35, H 4.44, N 10.38.
9,11-Dimethyl-7-(3-nitrophenyl)-6H-chromeno[3′,4′:5,6]pyrido[2,3-d]pyrimidine-6,8,10(9H,11H)trione (4h). Pale yellow solid; yield: 0.413 g (96%); mp > 300 °C. IR (KBr) (νmax/cm−1): 3008, 2984, 1704, 1676, 1610, 1572, 1520, 1485, 1346, 758. 1H-NMR: δ = 3.27 (s, 3H, NCH3), 3.53 (s, 3H, NCH3), 7.69 (m, 1H, H–Ar), 7.75 (d, 1H, J = 7.6 Hz, H–Ar), 7.85 (t, 1H, J = 8.0 Hz, H–Ar), 7.97 (m, 2H, H–Ar), 8.15 (d, 1H, J = 7.7 Hz, H–Ar), 8.24 (br s, 1H, H–Ar), 8.35 (d, 1H, J = 8.1 Hz, H–Ar) ppm. 13C-NMR: δ = 27.2, 29.3, 105.2, 112.1, 113.5, 114.6, 116.1, 117.7, 124.3, 125.6, 128.4, 129.5, 137.5, 138.6, 140.9, 141.2, 142.6, 151.1, 155.4, 156.8, 168.4, 174.1 ppm. Anal. calc. for C22H14N4O6 (430.37): C 61.40, H 3.28, N 13.02; found: C 61.48, H 3.17, N 12.88.
3. Results and discussion
The present synthesis involved the cyclocondensation reaction of 4-aminocoumarin (1), 1,3-dimethylbarbituric acid (2), and aromatic aldehydes (3) for the synthesis of 9,11-dimethyl-7-aryl-6H-chromeno[3′,4′:5,6]pyrido[2,3-d]pyrimidine-6,8,10(9H,11H)triones (4a–h) in the presence of a catalytic amount of the PANI-Fe3O4@ZnO nanocomposite in aqueous media at room temperature (Scheme 1). For the first run of experiments, the PANI-Fe3O4@ZnO nanocomposite was prepared according to the general procedure described in the Experimental section. The XRD pattern of the synthesized nanocomposite shows relatively weak and broad diffraction peaks from the lattice structure of ZnO and the spinel structure of Fe3O4. The broad peak at around 2θ = 20° is ascribed to periodic parallel and perpendicular PANI chains in the semi-crystalline structure (Fig. 2). In the XRD pattern, the peak intensities of ZnO are stronger than those of Fe3O4, confirming the ZnO shell of the composite.
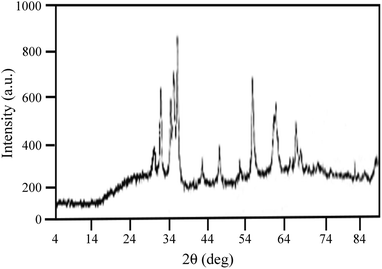 |
| Fig. 2 The XRD pattern of the synthesized PANI-Fe3O4@ZnO nanocomposite. | |
SEM (Fig. 3) and TEM (Fig. 4) imaging of the catalyst show that the microspheres of the PANI-Fe3O4@ZnO nanocomposite have an average diameter of about 75 nm and typical core–shell structure with uniform spherical morphology in which ZnO nanoparticles are dispersed uniformly around PANI.
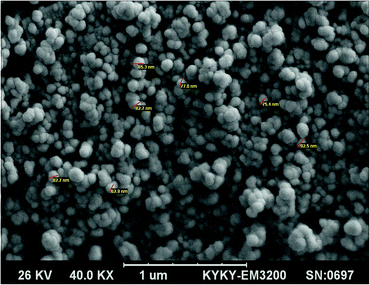 |
| Fig. 3 An SEM image of the synthesized PANI-Fe3O4@ZnO nanocomposite. | |
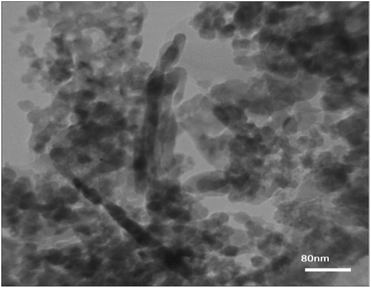 |
| Fig. 4 A TEM image of the synthesized PANI-Fe3O4@ZnO nanocomposite. | |
The EDX analysis results reveal the presence of C, O, Fe, and Zn elements in the structure of the nanocomposite (Fig. 5).
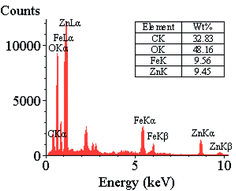 |
| Fig. 5 The EDX spectrum of the synthesized PANI-Fe3O4@ZnO nanocomposite. | |
In a typical experimental procedure, the reaction between 4-aminocoumarin (1), 1,3-dimethylbarbituric acid (2), and 4-chlorobenzaldehyde (3c) was chosen and investigated under various reaction conditions (Scheme 2 and Table 1). First, we studied the model reaction in refluxing water without any catalyst. The reaction was incomplete, with a yield of 61% even after 7 h (Table 1, entry 1). Next, we studied the use of various catalysts, including p-TsOH, DAHP, commercial Fe3O4 NPs (≤50 nm), and ZnO NPs (≤40 nm), in comparison to the PANI-Fe3O4@ZnO nanocomposite for the synthesis of the desired product 4c. Among these, the PANI-Fe3O4@ZnO nanocomposite (0.03 g) was found to be the superior catalyst (Table 1, entries 2–8). The effect of the reaction temperature was also examined. It is noteworthy that there was no distinct difference in the yield of this conversion under reflux heating; only a reduction in the reaction time was observed (Table 1, entries 7 and 9). To prove the effective involvement of water as a reaction media, we carried out the above reaction in several solvents, including EtOH, CH3CN, CH2Cl2, and DMF. The results showed that the highest yield (93%) was obtained in the presence of water (Table 1, entries 7 and 9–13).
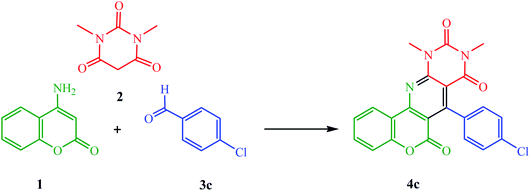 |
| Scheme 2 The synthesis of 9,11-dimethyl-7-(4-chlorophenyl)-6H-chromeno[3′,4′:5,6]pyrido[2,3-d]pyrimidine-6,8,10(9H,11H)trione (4c). | |
Table 1 The synthesis of 9,11-dimethyl-7-(4-chlorophenyl)-6H-chromeno[3′,4′:5,6]pyrido[2,3-d]pyrimidine-6,8,10(9H,11H)trione (4c) under various reaction conditions
Entry |
Catalyst |
Solvent |
Temp. (°C) |
Time (h) |
Yieldd,e (%) |
p-TsOH: p-toluenesulfonic acid. DAHP: diammonium hydrogen phosphate. DMF: dimethylformamide. Isolated yield. Reaction conditions: a mixture of 4-aminocoumarin (1, 1 mmol), 1,3-dimethylbarbituric acid (2, 1 mmol), and 4-chlorobenzaldehyde (3c, 1 mmol) was stirred under various reaction conditions. |
1 |
None |
H2O |
Reflux |
7 |
61 |
2 |
p-TsOHa (0.03 g) |
H2O |
r.t. |
5 |
84 |
3 |
DAHPb (0.03 g) |
H2O |
r.t. |
7 |
79 |
4 |
ZnO NPs (0.03 g) |
H2O |
r.t. |
6 |
86 |
5 |
Fe3O4 MNPs (0.03 g) |
H2O |
r.t. |
6 |
88 |
6 |
PANI-Fe3O4@ZnO (0.02 g) |
H2O |
r.t. |
6 |
72 |
7 |
PANI-Fe3O4@ZnO (0.03 g) |
H2O |
r.t. |
5 |
93 |
8 |
PANI-Fe3O4@ZnO (0.04 g) |
H2O |
r.t. |
5 |
93 |
9 |
PANI-Fe3O4@ZnO (0.03 g) |
H2O |
Reflux |
3 |
94 |
10 |
PANI-Fe3O4@ZnO (0.02 g) |
EtOH |
r.t. |
2.5 |
71 |
11 |
PANI-Fe3O4@ZnO (0.02 g) |
CH3CN |
r.t. |
3 |
63 |
12 |
PANI-Fe3O4@ZnO (0.02 g) |
CH2Cl2 |
r.t. |
3 |
45 |
13 |
PANI-Fe3O4@ZnO (0.02 g) |
DMFc |
r.t. |
2 |
76 |
Various aromatic aldehydes were condensed with 4-aminocoumarin and 1,3-dimethylbarbituric acid under the optimized reaction conditions to provide the corresponding products 4a–h in high yields (Table 2). It is noticeable that the aromatic ring substituent did not produce any special effects in terms of the yields under these reaction conditions. This may be due to the speed of the first step of the reaction in the formation of the alkene. The structures of compounds 4a–h were confirmed via IR, 1H NMR and 13C NMR spectroscopic studies, and also via elemental analyses. Spectroscopic data are given in the Experimental section. The synthesized catalyst was fully characterized via XRD, SEM, TEM, and EDX techniques.
Table 2 The PANI-Fe3O4@ZnO nanocomposite catalyzed synthesis of the 9,11-dimethyl-7-aryl-6H-chromeno[3′,4′:5,6]pyrido[2,3-d]pyrimidine-6,8,10(9H,11H)triones 4a–h
Product |
Ar |
Time (h) |
Yielda,b (%) |
Mp (°C) |
Yield refers to the pure isolated product characterized via IR, 1H NMR and 13C NMR spectral data and via elemental analyses. Reaction conditions: a mixture of 4-aminocoumarin (1, 1 mmol), 1,3-dimethylbarbituric acid (2, 1 mmol), aromatic aldehyde (3, 1 mmol), and PANI-Fe3O4@ZnO nanocomposite (0.03 g) in H2O (3 mL) was stirred at ambient temperature for an appropriate time. |
4a |
C6H5 |
5 |
92 |
>300 |
4b |
4-Br–C6H4 |
5 |
95 |
>300 |
4c |
4-Cl–C6H4 |
5.5 |
93 |
>300 |
4d |
4-CN–C6H4 |
5.5 |
96 |
>300 |
4e |
3-OH–C6H4 |
5 |
94 |
>300 |
4f |
4-OCH3–C6H4 |
7 |
95 |
>300 |
4g |
4-CH3–C6H4 |
6.5 |
93 |
>300 |
4h |
3-NO2–C6H4 |
6 |
96 |
>300 |
A suggested mechanism for the reaction is outlined in Scheme 3. Initially, the PANI-Fe3O4@ZnO nanocomposite is involved in the formation of the alkene (7) via Knoevenagel condensation between 1,3-dimethylbarbituric acid (2) and the aromatic aldehyde (3), via intermediates 5 and 6. Afterward, 4-aminocoumarin (1) is added to the alkene (7) generating the Michael adduct (8), which undergoes intermolecular cyclization and subsequent aromatization to produce the product (4). In order to demonstrate the proposed mechanism, we tried to divide the model reaction into two separate steps. In the first step, in the absence of 4-aminocoumarin (1), the formation of the alkene 7c occurred from the reaction between 1,3-dimethylbarbituric acid (2) and 4-chlorobenzaldehyde (3c). When we used the preformed alkene 7c in the reaction with 4-aminocoumarin (1) in a second step, it was shown that the product 4c resulted. The crude product from the first step (the alkene 7c) without further purification was isolated and used as a control spot in TLC testing of the three-component reaction. It was found that in the three-component reaction, alkene 7c as a first intermediate product is formed on the TLC plate about 0.5 hour after the start of the reaction, which is presumed to come from the reaction of 1,3-dimethylbarbituric acid (2) with 4-chlorobenzaldehyde (3c), and then the final product is obtained from the reaction of this intermediate with 4-aminocoumarin (1) after a specific period of time.
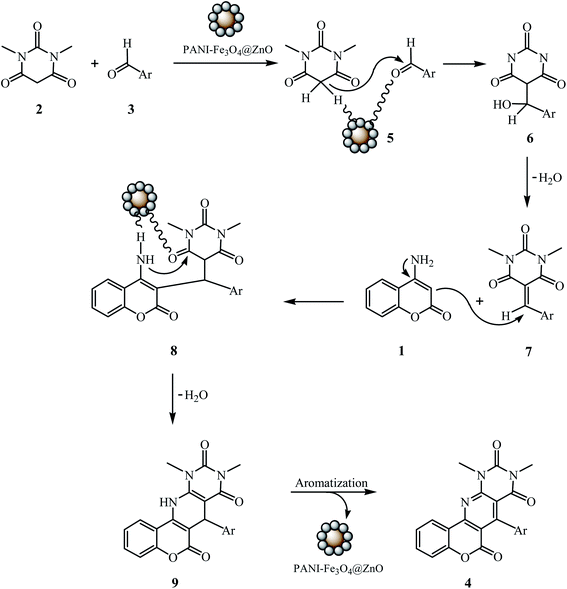 |
| Scheme 3 The proposed mechanism for the formation of 4. | |
4. Conclusions
In summary, a PANI-Fe3O4@ZnO nanocomposite was synthesized through a new strategy, characterized and applied as a suitable heterogenous catalyst for the environmentally friendly and economic synthesis of 9,11-dimethyl-7-aryl-6H-chromeno[3′,4′:5,6]pyrido[2,3-d]pyrimidine-6,8,10(9H,11H)triones via cyclocondensation reactions between 4-aminocoumarin, 1,3-dimethylbarbituric acid, and aromatic aldehydes. The appearance of relevant absorption peaks in the XRD pattern confirmed the successful synthesis of the nanocatalyst. In the EDX spectrum of the nanocatalyst, the expected elements were displayed. The average size and morphology of the nanocatalyst were determined from SEM and TEM images. The structures of compounds 4a–h were fully confirmed via IR, 1H NMR and 13C NMR spectroscopic studies, and also from elemental analyses. High yields of products, the use of a green solvent, the easy work-up procedure, the short reaction times, and the easily separable catalyst are the main advantages of this protocol. The magnetically recovered catalyst could be reused appropriately many times with suitable catalyst activity.
Conflicts of interest
There are no conflicts to declare.
Acknowledgements
Shahrzad Abdolmohammadi is grateful to the Research Council of East Tehran Branch, Islamic Azad University for financial support.
References
- I. Horvath and P. Anastas, Chem. Rev., 2007, 107, 2167–2168 CrossRef CAS PubMed.
- S. L. Schreiber, Nature, 2009, 457, 153–154 CrossRef CAS PubMed.
- J. Zhu and H. Bienayme, Multicomponent Reactions, Wiley-VCH, Weinheim, 2005 Search PubMed.
- B. M. Trost, Acc. Chem. Res., 2002, 35, 695–705 CrossRef CAS PubMed.
- P. A. Wender, V. A. Verma, T. J. Paxton and T. H. Pillow, Acc. Chem. Res., 2008, 41, 40–49 CrossRef CAS PubMed.
- S. Farshbaf, L. Sreerama, T. Khodayari and E. Vessally, Chem. Rev. Lett., 2018, 1, 56–67 Search PubMed.
- F. Behmagham, Z. Asadi and Y. J. Sadeghi, Chem. Rev. Lett., 2018, 1, 68–76 Search PubMed.
- S. Zhi, X. Ma and W. Zhang, Org. Biomol. Chem., 2019, 17, 7632–7650 RSC.
- H. G. O. Alvim, J. R. Correa, J. A. F. Assumpção, W. A. da Silva, M. O. Rodrigues, J. L. de Macedo, M. Fioramonte, F. C. Gozzo, C. C. Gatto and B. A. D. Neto, J. Org. Chem., 2018, 83, 4044–4053 CrossRef CAS PubMed.
- A. V. Vasco, Y. Méndez, A. Porzel, J. Balbach, L. A. Wessjohann and D. G. Rivera, Bioconjugate Chem., 2019, 30, 253–259 CrossRef CAS PubMed.
- L. S. da Silveira Pinto, M. R. C. Couri and M. V. N. de Souza, Curr. Org. Synth., 2018, 15, 21–37 CrossRef CAS.
- A. C. Boukis, K. Reiter, M. Frölich, D. Hofheinz and M. A. R. Meier, Nat. Commun., 2018, 9, 1439 CrossRef PubMed.
- M. Nikpassand and L. ZareFekri, Chem. Rev. Lett., 2019, 2, 7–12 Search PubMed.
- F. Valinia, N. Shojaei and P. Ojaghloo, Chem. Rev. Lett., 2019, 2, 90–97 Search PubMed.
- E. Jafari, P. Farajzadeh, N. Akbari and A. Karbakhshzadeh, Chem. Rev. Lett., 2019, 2, 123–129 Search PubMed.
- Y. M. Huh, Y. W. Jun, H. T. Song, S. Kim, J. S. Choi, J. H. Lee, S. Yoon, K. S. Kim, J. S. Shin, J. S. Suh and J. Cheon, J. Am. Chem. Soc., 2005, 127, 12387–12391 CrossRef CAS PubMed.
- P. Sharma, S. Rana, K. C. Barick, C. Kumar, H. G. Salunked and P. A. Hassan, New J. Chem., 2014, 38, 5500–5508 RSC.
- J. Deng, L. P. Mo, F. Y. Zhao, L. L. Hou, L. Yang and Z. H. Zhang, Green Chem., 2011, 13, 2576–2584 RSC.
- R. K. Sharma, S. Dutta, S. Sharma, R. Zboril, R. S. Varma and M. B. Gawande, Green Chem., 2016, 18, 3184–3209 RSC.
- L. Javadian and J. Safari, Iran. J. Catal., 2016, 6, 57–64 Search PubMed.
- R. Gupta, M. Yadav, R. Gaur, G. Arora, P. Rana, P. Yadav, A. Adholeya and R. K. Sharma, ACS Omega, 2019, 4, 21529–21539 CrossRef CAS PubMed.
- S. Fakheri-Vayeghan, S. Abdolmohammadi and R. Kia-Kojoori, Z. Naturforsch., B: Chem. Sci., 2018, 73, 545–551 CAS.
- D. Girija, H. S. B. Naik, B. V. Kumar, C. N. Sudhamani and K. N. Harish, Arabian J. Chem., 2019, 12, 420–428 CrossRef CAS.
- N. M. Dimitrijevic, S. Tepavcevic, Y. Liu, T. Rajh, S. C. Silver and D. M. Tiede, J. Phys. Chem. C, 2013, 117, 15540–15544 CrossRef CAS.
- C. W. Peng, K. C. Chang, C. J. Weng, M. C. Lai, C. H. Hsu, S. C. Hsu, Y. Y. Hsu, W. I. Hung, Y. Wei and J. M. Yeh, Electrochim. Acta, 2013, 95, 192–199 CrossRef CAS.
- X. Wang, Y. Shen, A. Xie, L. Qiu, S. Li and Y. Wang, J. Mater. Chem., 2011, 21, 9641–9646 RSC.
- L. Liang, J. Liu, C. F. W. Indisch Jr, G. J. Exarhos and Y. Lin, Angew. Chem., Int. Ed., 2002, 41, 3665–3668 CrossRef CAS PubMed.
- S. Xiong, Q. Wang and H. Xia, Synth. Met., 2004, 146, 37–42 CrossRef CAS.
- D. W. Hatchett and M. Josowicz, Chem. Rev., 2008, 108, 746–769 CrossRef CAS PubMed.
- R. Pratap and V. J. Ram, Chem. Rev., 2014, 114, 10476–10526 CrossRef CAS PubMed.
- M. Kidwai, S. Saxena, M. K. Rahman Khan and S. S. Thukral, Bioorg. Med. Chem. Lett., 2005, 15, 4295–4298 CrossRef CAS PubMed.
- R. R. Kumar, S. Perumal, P. Senthilkumar, P. Yogeeswari and D. Sriram, Bioorg. Med. Chem. Lett., 2007, 17, 6459–6462 CrossRef CAS PubMed.
- I. O. Donkor, C. L. Klein, L. Liang, N. Zhu, E. Bradley and A. M. Clark, J. Pharm. Sci., 1995, 84, 661–664 CrossRef CAS PubMed.
- M. M. Khafagy, A. H. Abd el-Wahab, F. A. Eid and A. M. el-Agrody, Farmaco, 2002, 57, 715–722 CrossRef CAS PubMed.
- L. Alvey, S. Prado, V. Huteau, B. Saint-Joanis, S. Michel, M. Koch, S. T. Cole, F. Tillequin and Y. L. Janin, Bioorg. Med. Chem., 2008, 16, 8264–8272 CrossRef CAS PubMed.
- A. H. Bedair, N. A. El-Hady, A. El-Latif, A. H. Fakery and A. M. El-Agrody, Farmaco, 2000, 55, 708–714 CrossRef CAS PubMed.
- M. Mladenović, M. Mihailović, D. Bogojević, S. Matić, N. Nićiforović, V. Mihailović, N. Vuković, S. Sukdolak and S. I. Solujić, Int. J. Mol. Sci., 2011, 12, 2822–2841 CrossRef PubMed.
- T. Symeonidis, M. Chamilos, D. J. Hadjipavlou-Litina, M. Kallitsakis and K. E. Litinas, Bioorg. Med. Chem. Lett., 2009, 19, 1139–1142 CrossRef CAS PubMed.
- H. Gourdeau, L. Leblond, B. Hamelin, C. Desputeau, K. Dong, I. Kianicka, D. Custeau, C. Boudreau, L. Geerts, S. X. Cai, J. Drewe, D. Labrecque, S. Kasibhatla and B. Tseng, Mol. Cancer Ther., 2004, 3, 1375–1384 CAS.
- V. K. Tandon, M. Vaish, S. Jain, D. S. Bhakuni and R. C. Srimal, Indian J. Pharm. Sci., 1991, 53, 22–23 CAS.
- J. L. Wang, D. Liu, Z. J. Zhang, S. Shan, X. Han, S. M. Srinivasula, C. M. Croce, E. S. Alnemri and Z. Huang, Proc. Natl. Acad. Sci. U. S. A., 2000, 97, 7124–7129 CrossRef CAS PubMed.
- F. Cheng, A. Ishikawa, Y. Ono, T. Arrheniusa and A. Nadzana, Bioorg. Med. Chem. Lett., 2003, 13, 3647–3650 CrossRef PubMed.
- D. Grée, S. Vorin, V. L. Manthati, F. Caijo, G. Viault, F. Manero, P. Juin and R. Grée, Tetrahedron Lett., 2008, 49, 3276–3278 CrossRef.
- W. Kemnitzer, S. Jiang, H. Zhang, S. Kasibhatla, C. Crogan-Grundy, C. Blais, G. Attardo, R. Denis, S. Lamothe, H. Gourdeau, B. Tseng, J. Drewe and X. Cai, Bioorg. Med. Chem. Lett., 2008, 18, 5571–5575 CrossRef CAS PubMed.
- M. Longobardi, A. Bargagna, E. Mariani, P. Schenone, S. Vitagliano, L. Stella, A. Di Sarno and E. Marmo, Farmaco, 1990, 45, 399–401 CAS.
- T. A. Bayer, S. Schäfer, H. Breyhan, O. Wirths, C. Treiber and G. Multhaup, Clin. Neuropathol., 2006, 25, 163–171 CAS.
- G. D. Henry, Tetrahedron, 2004, 60, 6043–6061 CrossRef CAS.
- S. Abdolmohammadi, Comb. Chem. High Throughput Screening, 2018, 21, 594–601 CrossRef CAS PubMed.
- S. Abdolmohammadi, B. Mirza and E. Vessally, RSC Adv., 2019, 9, 41868–41876 RSC.
- S. Abdolmohammadi, S. R. Rasouli Nasrabadi, M. R. Dabiri and S. M. Banihashemi Jozdani, Appl. Organomet. Chem., 2020, 34, e5462 CrossRef CAS.
Footnote |
† Electronic supplementary information (ESI) available. See DOI: 10.1039/d0ra01978j |
|
This journal is © The Royal Society of Chemistry 2020 |
Click here to see how this site uses Cookies. View our privacy policy here.