DOI:
10.1039/D0SC02516J
(Edge Article)
Chem. Sci., 2020,
11, 8295-8300
N- and O-arylation of pyridin-2-ones with diaryliodonium salts: base-dependent orthogonal selectivity under metal-free conditions†
Received
4th May 2020
, Accepted 21st July 2020
First published on 21st July 2020
Abstract
Metal-free N- and O-arylation reactions of pyridin-2-ones as ambident nucleophiles have been achieved with diaryliodonium salts on the basis of base-dependent chemoselectivity. In the presence of N,N-diethylaniline in fluorobenzene, pyridin-2-ones were very selectively converted to N-arylated products in high yields. On the other hand, the O-arylation reactions smoothly proceeded with the use of quinoline in chlorobenzene, leading to high yields and selectivities. In these methods, a variety of pyridin-2-ones in addition to pyridin-4-one and a set of diaryliodonium salts were accepted as suitable reaction partners.
Introduction
Carbon–heteroatom bond forming reactions are one of the fundamental transformations in organic synthesis.1 Especially, N- and O-arylation reactions have been pursued with great vigor because of their wide utilization in pharmaceutical research and process development.2,3 Pyridin-2-ones are an ambident nucleophile containing an amide moiety and their arylated products such as N-aryl pyridin-2-ones and O-aryl 2-hydroxypyridines are important substructures in bioactive compounds.4,5 Therefore, N- and O-arylation reactions of pyridin-2-ones have been studied under catalytic and non-catalytic conditions.6–8 In the transition metal-catalyzed methods,6 the O-arylation was realized by using 6-substituted pyridin-2-ones to suppress the formation of N-arylated products. As a metal-free process, Mukaiyama achieved the N-arylation with organobismuth reagents.7a While Gaunt reported the preparation of 2-phenoxypyridine with the use of diphenyliodonium fluoride,7c Mo developed the metal-free O-arylation of 6-substituted pyridin-2-ones.7b The utilization of appropriate catalysts and reagents is a promising approach to control chemoselectivity for ambident nucleophiles,9,10 and the orthogonal selectivity in arylation reactions of N,O-nucleophiles has been realized with transition-metal catalysts to give simple and useful methods.11 For example, the chemoselectivity in the formation of arylated aminophenols largely depended on the kind of catalyst metals,11a while bidentate ligands for a copper catalyst played key roles in the selective arylation of aminoalcohols (Scheme 1a).11b Buchwald found that copper catalysts had the ability to switch the selectivity for the N- and O-arylation of pyridin-2-one with aryl iodides (Scheme 1b).12 However, this type of selectivity-switchable arylation via cross-coupling for N,O-nucleophiles including aminoalcohols, aminophenols, and tautomerizable amides has not been developed under metal-free conditions in spite of its attractive potential in terms of green chemistry.13 Among hypervalent halogen compounds,14 diaryliodonium salts have recently received much attention as mild, less-toxic, and easily accessible arylating agents.15 By leveraging their beneficial features, transition metal-free carbon–heteroatom bond forming reactions have been actively pursued.16,17 Herein, we report metal-free N- and O-arylation of pyridin-2-ones with diaryliodonium salts based on base-dependent chemoselectivity (Scheme 1c).
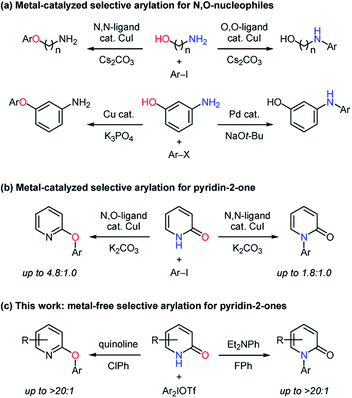 |
| Scheme 1 Selectivity-switchable arylation for N,O-nucleophiles. | |
Results and discussion
At the outset, the effects of organic and inorganic bases were investigated in the phenylation of pyridin-2-one (1a) with diphenyliodonium triflate (2a) (Table 1). In the absence of bases, the N-phenylated product 3aa and O-phenylated product 4aa were obtained in low yields with no selectivity (entry 1). The examination of organic bases proved that the reaction conditions with pyridine gave the O-phenylated product 4aa with a high selectivity despite a low yield, while the highly selective formation of the N-phenylated product 3aa was observed with a high yield in the presence of DIPEA (entries 2–3). The use of DABCO and DBU afforded the mixtures of 3aa and 4aa with low selectivities (entries 4–5). In the screening of inorganic bases, the N-phenylated product 3aa was obtained as a major product in moderate to good yields with low to moderate selectivities (entries 6–12). Sodium bicarbonate conduced to a better selectivity than the other inorganic bases only to give 3aa and 4aa in the ratio of 78
:
22 (entry 9).
Table 1 Initial screening of basesa
The findings of the initial screening encouraged us, and the optimization of conditions for the selective N-arylation was conducted at a higher concentration (Table 2). In the examination of tertiary amines, the N-phenylated product 3aa was obtained in high yields and selectivities when using trialkyl amines such as DIPEA and n-Bu3N (entries 1–2). N,N-Diethylaniline led to a slightly better yield of 3aa, while a decrease in yield was observed in the presence of N,N-dimethylaniline or N-methyldiphenylamine (entries 3–5). Then, a series of solvents were investigated with N,N-diethylaniline. More polar solvents exhibited a tendency to give 3aa in lower yields (entries 3 and 6–8). The use of fluorobenzene resulted in 90% yield of 3aa with an excellent selectivity, although 3aa was formed in chlorobenzene with a slightly reduced yield (entries 9–10). DIPEA and n-Bu3N were also examined in fluorobenzene only to afford lower yields of 3aa as compared to N,N-diethylaniline (entries 11–12).
Table 2 Optimization for N-arylationa
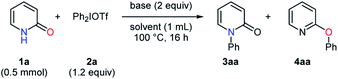
|
Entry |
Base |
Solvent |
3aa (%) |
4aa (%) |
Reaction conditions: 1a (0.5 mmol), Ph2IOTf (1.2 equiv.), base (2 equiv.), solvent (1 mL), 100 °C, 16 h.
85 °C.
|
1 |
DIPEA |
Toluene |
86 |
6 |
2 |
n-Bu3N |
Toluene |
85 |
4 |
3 |
Et2NPh |
Toluene |
88 |
6 |
4 |
Me2NPh |
Toluene |
77 |
3 |
5 |
MeNPh2 |
Toluene |
30 |
7 |
6 |
Et2NPh |
Dioxane |
83 |
4 |
7 |
Et2NPh |
DMA |
73 |
8 |
8 |
Et2NPh |
DMSO |
43 |
4 |
9 |
Et2NPh |
FPh |
90(90)b |
Trace(trace)b |
10 |
Et2NPh |
ClPh |
85 |
6 |
11 |
DIPEA |
FPh |
81 |
4 |
12 |
n-Bu3N |
FPh |
75 |
6 |
Subsequently, the reaction conditions for the selective O-arylation were optimized at a higher temperature (Table 3). The influences of pyridine and related compounds18 were tested in toluene. The use of pyridine and 2,6-lutidine gave the O-phenylated product 4aa in moderate yields with good selectivites, and 2,6-lutidine led to a slightly better result (entries 1–2). Pyrazine caused a significant decrease in yield, while isoquinoline provided almost the same result as pyridine (entries 3–4). In the presence of quinoline, 4aa was obtained with a high selectivity despite a slightly lower yield as compared to 2,6-lutidine (entry 5). Then, the examination of solvents was conducted with quinoline. More polar solvents such as dioxane, DMA, and DMSO conduced to decreased yields of 4aa (entries 6–8). Although fluorobenzene was not suitable for the O-phenylation of pyridin-2-one, chlorobenzene gave 4aa in high yields with excellent selectivities especially at a higher temperature (entries 9–10).19
Table 3 Optimization for O-arylationa
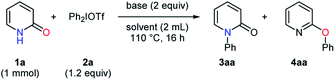
|
Entry |
Base |
Solvent |
3aa (%) |
4aa (%) |
Reaction conditions: 1a (1 mmol), Ph2IOTf (1.2 equiv.), base (2 equiv.), solvent (2 mL), 110 °C, 16 h.
130 °C.
|
1 |
Pyridine |
Toluene |
5 |
41 |
2 |
2,6-Lutidine |
Toluene |
6 |
51 |
3 |
Pyrazine |
Toluene |
2 |
18 |
4 |
Isoquinoline |
Toluene |
5 |
40 |
5 |
Quinoline |
Toluene |
2 |
47 |
6 |
Quinoline |
Dioxane |
1 |
18 |
7 |
Quinoline |
DMA |
9 |
12 |
8 |
Quinoline |
DMSO |
4 |
4 |
9 |
Quinoline |
FPh |
2 |
20 |
10 |
Quinoline |
ClPh |
Trace(trace)b |
79(96)b |
The influence of varying pyridin-2-ones and diaryliodonium salts on the selective N-arylation was studied (Table 4). The transformation of 5-methylpyridin-2-one (1b) proceeded smoothly to give the desired product in a high yield (3ba).20 Pyridin-2-ones with an electron-withdrawing group at the 5-position were converted with no problem, and an ester moiety proved to be tolerated under the conditions (3ca–ea). In the examination of 3-substituted substrates, electron-donating and -withdrawing groups gave no significant decrease in yield (3fa–ha).21 Whereas a high yield was observed in the N-phenylation of 4-chloropyridin-2-one, 6-chloropyridin-2-one afforded only the N-phenylated product in 33% yield (3ia–ja). On the other hand, pyridin-4-one was found to be a good reaction partner for this transformation (3ka). In addition, the investigation of diaryliodonium salts was carried out. A diaryliodonium triflate and tetrafluoroborate bearing an electron-donating group led to high yields of the N-arylated products (3ab–ac). Electron-withdrawing substituents such as fluoro, chloro, and ester groups caused no serious difficulty (3ad–af). In the presence of steric hindrance close to a reactive site, the desired product was obtained in 75% yield with a relatively lower selectivity (3ag). In most cases, quite high selectivities (>20
:
1) were observed except for the selective formation of 3ag.
Table 4 Selective N-arylation of pyridin-2-ones with diaryliodonium salts
1 mmol scale.
Et2NPh (2.5 equiv.).
100 °C.
DIPEA (2.5 equiv.), Ph2IBF4 (1.2 equiv.).
DIPEA (3 equiv.), 2-fluorotoluene.
ClPh, 130 °C.
Ph2IOTf (1 equiv.).
(4-MeOPh)2IBF4 (1.2 equiv.).
(3-EtO2CPh)2IBF4 (1.2 equiv.).
(2-MeOPh)2IBF4 (1.2 equiv.).
|
|
The examination of pyridin-2-ones and diaryliodonium salts was conducted in the selective O-arylation (Table 5). Most of the 5-substituted substrates bearing an electron-donating or -withdrawing group gave high yields (4ba–ca and 4ea), although a slight decrease in yield was observed in the presence of a trifluoromethyl group (4da). Even under the electronic and steric influences of substituents at the 3-position, the O-arylated products 4fa–ha were also obtained uneventfully. The transformation of 6-methoxypyridin-2-one as well as 4-chloropyridin-2-one was carried out with favorable results (4ia and 4la). Moreover, pyridin-4-one proved to be a suitable substrate for the selective O-arylation (4ka). Subsequently, a set of diaryliodonium triflates were investigated. Aryl moieties with a substituent such as alkyl, trifluoromethyl, and halogen groups were efficiently transferred to an oxygen atom of pyridin-2-one (4ah–ai and 4ad–ae). Neither an ester group nor ortho-methyl group led to harmful effects, affording 4aj and 4ak in high yields with almost no side product. In all cases, the O-arylated products were formed with excellent selectivities (> 20
:
1).
Table 5 Selective O-arylation of pyridin-2-ones with diaryliodonium salts
1,2-Cl2Ph, 140 °C.
Ph2IOTf (1.5 equiv.).
8 h.
Ph2IOTf (2.5 equiv.).
0.25 mmol scale.
1 h.
|
|
To explore the influences of substituents on aryl group transfer preference, unsymmetrical diaryliodonium salts were tested in the selective N-arylation (Scheme 2a). When a diaryliodonium triflate with an electron-donating and -withdrawing group was employed, the N-arylated products 3ac and 3ai were obtained in 35% and 44% yields, respectively. In the examination of steric effects in an arylating agent, the diaryliodonium triflate 2m favorably transferred 4-methylphenyl group to pyridin-2-one, providing a 3.2
:
1 ratio of 3ab
:
3ak. While the anti-ortho effect was observed, the electronic effect was not pronounced unlike the reported N-arylation of amides.22 A similar investigation was conducted in the selective O-arylation (Scheme 2b). The use of the diaryliodonium triflate 2l caused the selective formation of the CF3-containing product in a high yield with a 1
:
27 ratio of 4ac
:
4ai. A preferential transfer of 2-methylphenyl group from the aryl source 2m was observed to give 4ab and 4ak in 9% and 84% yields, respectively. These results had a similar tendency to those in the reported arylation of phenols.23 Besides, the scalability of these transformations was studied in a 10 mmol scale, and the desired products were obtained with high yields in both the selective N- and O-phenylation of pyridin-2-one (Scheme 3).
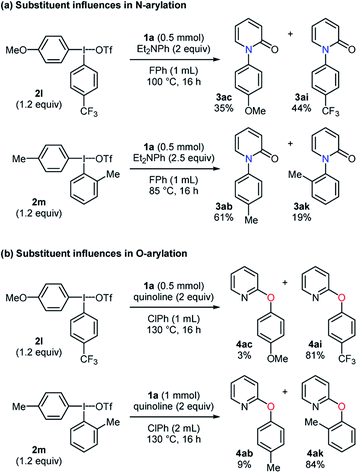 |
| Scheme 2 Substituent influences of diaryliodonium triflates on aryl group transfer preference in N-arylation and O-arylation. | |
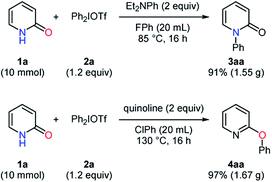 |
| Scheme 3 Examination of the scalability for N- and O-arylation. | |
To obtain further information on these methods, 2,6-di-tert-butyl-4-methylphenol (BHT), 9,10-dihydroanthracene (DHA), and 1,1-diphenylethylene (DPE) were employed as radical scavengers (Scheme 4). The N- and O-phenylation of the substrate 1b smoothly proceeded with high selectivities even in the presence of these radical trapping reagents, which suggested that single electron transfer processes might not be included.22,23 Because the reaction mechanism for the carbon–heteroatom bond formation with diaryliodonium salts has been proposed on the basis of the T-shaped intermediate A in the literature (Scheme 5a),16,17 the ligand exchange at the iodine center of 2a using the sodium salt of the pyridin-2-one 1d was carried out to afford the iodonium salt 5 (Scheme 5b).24 According to X-ray analysis, the compound 5 basically possesses the T-shaped structure with the N–I bond length of 2.740 Å and the N–I–C bond angle of 168.93°. The carbon–oxygen distance of 1.286 Å is closer to the C
O bond length.21 The small deviation of the N–I–C bond angle from linearity and slightly longer C
O bond length might result from a partial electron delocalization in the amidate moiety. The iodonium salt 5 was subjected to the N-arylation conditions to give the N-phenylated product 3da in a high yield with a good selectivity, and N,N-diethylaniline showed no significant influence (Scheme 5c). Under the O-arylation conditions, 3da was obtained from 5 as a major product with a lower selectivity despite the presence or absence of quinoline. The interaction between the amidate moiety and iodine center could play a key role in the selective C–N bond formation, but the decreased selectivity could suggest more than one pathway.25 Meanwhile, the iodonium salt 5 might not be directly relevant to the selective O-arylation.
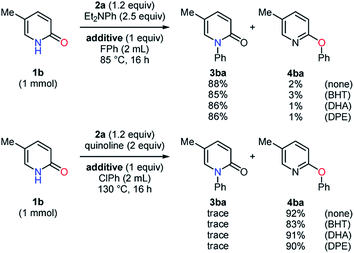 |
| Scheme 4
N- and O-arylation in the presence of radical scavengers. | |
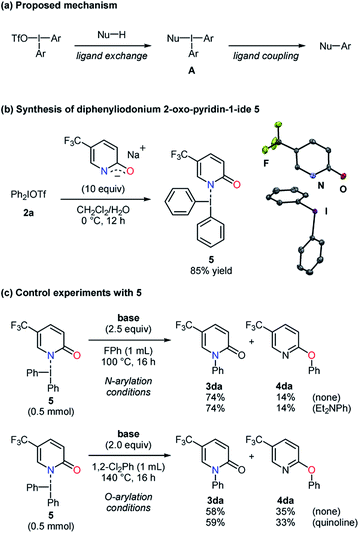 |
| Scheme 5 Synthesis of diaryliodonium salt 5 and control experiments. | |
Conclusions
In summary, a complementary set of the selective N- and O-arylation for pyridin-2-ones with diaryliodonium salts has been developed under metal-free conditions. While N-arylated products were obtained in high yields with high selectivities by using N,N-diethylaniline in fluorobenzene, the reaction conditions with quinoline in chlorobenzene led to the highly selective formation of O-arylated products in high yields. These methods were applicable to various substituted pyridin-2-ones as well as pyridin-4-one, and a series of diaryliodonium salts proved to be good reaction partners. Further studies on the detailed reaction mechanism including selectivity and application towards synthesis of bioactive compounds are ongoing in our laboratory.
Conflicts of interest
There are no conflicts to declare.
Acknowledgements
This research was supported by JSPS KAKENHI (18K06582, 19K16317, and 19K05459) and Research Grant for Pharmaceutical Sciences from Takeda Science Foundation. We are grateful to Prof. T. Dohi of Ritsumeikan University for his helpful advice. The data collection was conducted with the research equipment shared in MEXT Project for promoting public utilization of advanced research infrastructure (program for supporting introduction of the new sharing system: JPMXS0422500320).
Notes and references
-
(a)
Arene Chemistry: Reaction Mechanisms and Methods for Aromatic Compounds, ed. J. Mortier, Wiley, Hoboken, 2016 Search PubMed;
(b) J. X. Qiao and P. Y. S. Lam, Synthesis, 2011, 43, 829–856 CrossRef;
(c) I. P. Beletskaya and A. V. Cheprakov, Coord. Chem. Rev., 2004, 248, 2337–2364 CrossRef CAS.
-
(a) G. Evano, J. Wang and A. Nitelet, Org. Chem. Front., 2017, 4, 2480–2499 RSC;
(b) P. Ruiz-Castillo and S. L. Buchwald, Chem. Rev., 2016, 116, 12564–12649 CrossRef CAS PubMed;
(c) B. Schlummer and U. Scholz, Adv. Synth. Catal., 2004, 346, 1599–1626 CrossRef CAS.
-
(a) S. Bhunia, G. G. Pawar, S. V. Kumar, Y. Jiang and D. Ma, Angew. Chem. Int. Ed, 2017, 56, 16136–16179 CrossRef CAS PubMed;
(b) F. Monnier and M. Taillefer, Angew. Chem. Int. Ed, 2009, 48, 6954–6971 CrossRef CAS PubMed;
(c) S. V. Ley and A. W. Thomas, Angew. Chem. Int. Ed, 2003, 42, 5400–5449 CrossRef CAS PubMed.
-
(a) J. L. Engers, K. A. Bollinger, R. L. Weiner, A. L. Rodriguez, M. F. Long, M. M. Breiner, S. Chang, S. R. Bollinger, M. Bubser, C. K. Jones, R. D. Morrison, T. M. Bridges, A. L. Blobaum, C. M. Niswender, P. J. Conn, K. A. Emmitte and C. W. Lindsley, ACS Med. Chem. Lett., 2017, 8, 925–930 CrossRef CAS PubMed;
(b) J. Yang, G. Su, Y. Ren and Y. Chen, Eur. J. Med. Chem., 2015, 101, 41–51 CrossRef CAS PubMed;
(c) Z. Ma, Y. Pan, W. Huang, Y. Yang, Z. Wang, Q. Li, Y. Zhao, X. Zhang and Z. Shen, Bioorg. Med. Chem. Lett., 2014, 24, 220–223 CrossRef CAS PubMed.
-
(a) A. S. Carlson, H. Cui, A. Divakaran, J. A. Johnson, R. M. Brunner, W. C. K. Pomerantz and J. J. Topczewski, ACS Med. Chem. Lett., 2019, 10, 1296–1301 CrossRef CAS PubMed;
(b) T. Ogiyama, M. Yamaguchi, N. Kurikawa, S. Honzumi, Y. Yamamoto, D. Sugiyama, H. Takakusa and S. Inoue, Bioorg. Med. Chem., 2017, 25, 2234–2243 CrossRef CAS PubMed;
(c) C. M. G. Azevedo, K. R. Watterson, E. T. Wargent, S. V. F. Hansen, B. D. Hudson, M. A. Kępczyńska, J. Dunlop, B. Shimpukade, E. Christiansen, G. Milligan, C. J. Stocker and T. Ulven, J. Med. Chem., 2016, 59, 8868–8878 CrossRef CAS PubMed.
-
N-Arylation (catalytic):
(a) S.-H. Jung, D.-B. Sung, C.-H. Park and W.-S. Kim, J. Org. Chem., 2016, 81, 7717–7724 CrossRef CAS PubMed;
(b) K. A. Kumar, P. Kannaboina, C. K. Jaladanki, P. V. Bharatam and P. Das, ChemistrySelect, 2016, 1, 601–607 CrossRef CAS;
(c) C. S. Li and D. D. Dixon, Tetrahedron Lett., 2004, 45, 4257–4260. CrossRef CAS
O-Arylation (catalytic):;
(d) T. Chen, Q. Huang, Y. Luo, Y. Hu and W. Lu, Tetrahedron Lett., 2013, 54, 1401–1404 CrossRef CAS.
-
N-Arylation (non-catalytic):
(a) K. Ikegai and T. Mukaiyama, Chem. Lett., 2005, 34, 1496–1497. CrossRef CAS
O-Arylation (non-catalytic):;
(b) X.-H. Li, A.-H. Ye, C. Liang and D.-L. Mo, Synthesis, 2018, 50, 1699–1710 CrossRef CAS;
(c) L. Chan, A. McNally, Q. Y. Toh, A. Mendoza and M. J. Gaunt, Chem. Sci., 2015, 6, 1277–1281 RSC.
- The metal-free arylation for quinolin-4-ones depending on steric effects was reported: M. K. Mehra, M. P. Tantak, V. Arun, I. Kumar and D. Kumar, Org. Biomol. Chem., 2017, 15, 4956–4961 RSC.
- N. A. Afagh and A. K. Yudin, Angew. Chem. Int. Ed, 2010, 49, 262–310 CrossRef CAS PubMed.
-
(a) Y. Yamane, K. Miyazaki and T. Nishikata, ACS Catal., 2016, 6, 7418–7425 CrossRef CAS;
(b) W.-H. Rao, X.-S. Yin and B.-F. Shi, Org. Lett., 2015, 17, 3758–3761 CrossRef CAS;
(c) T. Xu and H. Alper, J. Am. Chem. Soc., 2014, 136, 16970–16973 CrossRef CAS;
(d) S. Ueda and S. L. Buchwald, Angew. Chem. Int. Ed, 2012, 51, 10364–10367 CrossRef CAS PubMed.
-
(a) D. Maiti and S. L. Buchwald, J. Am. Chem. Soc., 2009, 131, 17423–17429 CrossRef CAS PubMed;
(b) A. Shafir, P. A. Lichtor and S. L. Buchwald, J. Am. Chem. Soc., 2007, 129, 3490–3491 CrossRef CAS PubMed;
(c) G. E. Job and S. L. Buchwald, Org. Lett., 2002, 4, 3703–3706 CrossRef CAS PubMed.
- R. A. Altman and S. L. Buchwald, Org. Lett., 2007, 9, 643–646 CrossRef CAS PubMed.
-
Green and Sustainable Medicinal Chemistry: Methods, Tools and Strategies for the 21st Century Pharmaceutical Industry, ed. L. Summerton, H. F. Sneddon, L. C. Jones and J. H. Clark, Royal Society of Chemistry, Cambridge, 2016 Search PubMed.
-
(a)
The Chemistry of Hypervalent Halogen Compounds, ed. B. Olofsson, I. Marek and Z. Rappoport, Weily, Chichester, 2019 Search PubMed;
(b) M. Nakajima, K. Miyamoto, K. Hirano and M. Uchiyama, J. Am. Chem. Soc., 2019, 141, 6499–6503 CrossRef CAS PubMed;
(c) U. Farooq, A. A. Shah and T. Wirth, Angew. Chem. Int. Ed, 2009, 48, 1018–1020 CrossRef CAS PubMed.
-
(a) D. R. Stuart, Chem. –Eur. J., 2017, 23, 15852–15863 CrossRef CAS PubMed;
(b) K. Aradi, B. L. Tóth, G. L. Tolnai and Z. Novák, Synlett, 2016, 27, 1456–1485 CrossRef CAS;
(c) E. A. Merritt and B. Olofsson, Angew. Chem. Int. Ed, 2009, 48, 9052–9070 CrossRef CAS.
-
(a) S. Roshandel, M. J. Lunn, G. Rasul, D. S. M. Ravinson, S. C. Suri and G. K. S. Prakash, Org. Lett., 2019, 21, 6255–6258 CrossRef CAS;
(b) N. Purkait, G. Kervefors, E. Linde and B. Olofsson, Angew. Chem. Int. Ed, 2018, 57, 11427–11431 CrossRef CAS PubMed;
(c) A. H. Sandtorv and D. R. Stuart, Angew. Chem. Int. Ed, 2016, 55, 15812–15815 CrossRef CAS PubMed;
(d) N. Lucchetti, M. Scalone, S. Fantasia and K. Muñiz, Angew. Chem. Int. Ed, 2016, 55, 13335–13339 CrossRef CAS PubMed;
(e) M. A. Carroll and R. A. Wood, Tetrahedron, 2007, 63, 11349–11354 CrossRef CAS.
-
(a) H. Chen, J. Han and L. Wang, Angew. Chem. Int. Ed, 2018, 57, 12313–12317 CrossRef CAS PubMed;
(b) E. Stridfeldt, E. Lindstedt, M. Reitti, J. Blid, P.-O. Norrby and B. Olofsson, Chem. –Eur. J., 2017, 23, 13249–13258 CrossRef CAS PubMed;
(c) T. Dohi, D. Koseki, K. Sumida, K. Okada, S. Mizuno, A. Kato, K. Morimoto and Y. Kita, Adv. Synth. Catal., 2017, 359, 3503–3508 CrossRef CAS;
(d) G. L. Tolnai, U. J. Nilsson and B. Olofsson, Angew. Chem. Int. Ed, 2016, 55, 11226–11230 CrossRef CAS PubMed;
(e) E. Lindstedt, E. Stridfeldt and B. Olofsson, Org. Lett., 2016, 18, 4234–4237 CrossRef CAS PubMed;
(f) K. Matsuzaki, K. Okuyama, E. Tokunaga, N. Saito, M. Shiro and N. Shibata, Org. Lett., 2015, 17, 3038–3041 CrossRef CAS;
(g) N. Jalalian, E. E. Ishikawa, L. F. Silva Jr and B. Olofsson, Org. Lett., 2011, 13, 1552–1555 CrossRef CAS PubMed.
- Ochiai reported that pyridine acted as a ligand for a diphenyliodonium salt: T. Suefuji, M. Shiro, K. Yamaguchi and M. Ochiai, Heterocycles, 2006, 67, 391–397 CrossRef CAS.
- The comparison between the effects of FPh and ClPh is included in the ESI.†.
- The compound 3ba is known as Pirfenidone, a medication used for idiopathic pulmonary fibrosis: E. S. Kim and G. M. Keating, Drugs, 2015, 75, 219–230 CrossRef CAS PubMed.
- The X-ray analysis for 3ga and 4ga was carried out (3ga: CCDC 1999363, 4ga: CCDC 1999364). The carbon–oxygen distances: 1.234 Å (3ga) and 1.377 Å (4ga). The data is included in the ESI.†.
- F. Tinnis, E. Stridfeldt, H. Lundberg, H. Adolfsson and B. Olofsson, Org. Lett., 2015, 17, 2688–2691 CrossRef CAS PubMed.
- J. Malmgren, S. Santoro, N. Jalalian, F. Himo and B. Olofsson, Chem. –Eur. J., 2013, 19, 10334–10342 CrossRef CAS PubMed.
- The examples of diaryliodonium salts bearing a nucleophile were reported in the ref. 16d and 17c, and the following: D. I. Bugaenko, M. A. Yurovskaya and A. V. Karchava, Org. Lett., 2018, 20, 6389–6393 CrossRef CAS PubMed.
- Olofsson proposed the two possible pathways in the metal-free arylation of amides such as a [1,2]-rearrangement and [2,3]-rearrangement in the ref. 22.
Footnote |
† Electronic supplementary information (ESI) available: Experimental details and spectroscopic data. CCDC 1999363 (3ga), 1999364 (4ga) and 1999366 (5). For ESI and crystallographic data in CIF or other electronic format see DOI: 10.1039/d0sc02516j |
|
This journal is © The Royal Society of Chemistry 2020 |
Click here to see how this site uses Cookies. View our privacy policy here.