DOI:
10.1039/D0QI01130D
(Research Article)
Inorg. Chem. Front., 2021,
8, 164-172
Y2(Te4O10)(SO4): a new sulfate tellurite with a unique Te4O10 polyanion and large birefringence†
Received
18th September 2020
, Accepted 25th October 2020
First published on 26th October 2020
Abstract
Two new yttrium sulfate tellurites, namely, Y3(TeO3)2(SO4)2(OH)(H2O) and Y2(Te4O10)(SO4), were achieved in our exploration of new sulfate tellurites, with a large birefringence in the Y3+–Te4+–SO42− system. The two compounds exhibit two new three dimensional (3D) frameworks composed of different yttrium tellurite layers bridged by sulfate tetrahedra. The tellurite groups in Y2(Te4O10)(SO4) polymerized together into a unique (Te4O10)4− 1D double-strand with an eight-member polyhedral ring tunnel along the a-axis. Optical diffuse reflectance spectra showed that the two compounds present a wide energy band gap with Eg values of 4.4 and 4.1 eV respectively. The birefringence values of Y3(TeO3)2(SO4)2(OH)(H2O) and Y2(Te4O10)(SO4) were calculated to be 0.092 and 0.149 at 532 nm respectively. Furthermore, the anhydrous Y2(Te4O10)(SO4) features a large birefringence, wide energy band gap and high thermal stability. In addition, the IR spectrum, TG-DSC curve and theoretical calculations of the title compound were recorded in this paper.
Introduction
The Te(IV) cation with stereo-chemically active lone pair electrons can adopt three different coordination geometries: (i) TeO3 triangular pyramid, (ii) TeO4 disphenoid, and (iii) TeO5 tetragonal pyramid.1–14 In these configurations, the lone pair is located at one side of the central Te(IV), pushing the oxygen atoms to the other side. These tellurite groups can be polymerized further to multifarious clusters or even unlimited expanded architectures. So, the structural types of tellurite compounds are very rich. Recently, it is reported that the inorganic units with stereo-chemically active lone pair electrons can play a positive role in birefringence (Δn) because of their strong anisotropies.15–17 For example, the lone pair cation of Sn2+ was reported to increase the birefringence of phosphates, which is usually too low to be used because of the weak optical anisotropy of the PO4 tetrahedral group, to an appropriate level of 0.07 at 1064 nm.18 The birefringence of Bi4NbO3F3(SeO3)4 can reach 0.22 at 1064 nm owing to the stereo-chemically active lone pair electrons of Bi3+ cations and SeO3 groups.19 As a kind of active lone pair electron unit with plentiful configurations, research on the birefringence of tellurites is very rare.
Sulfates, similar to phosphates, composed of noncentrosymmetric tetrahedral groups, could be promising second harmonic generation materials because of their wide transparent range and good thermal stability.20–27 However, as the tetrahedral oxysalt, small birefringence is an unavoidable question, which usually means hard to achieve phase matching. To enhance the birefringence level of sulfates, active lone pair electron cations of Bi3+ and Sb3+ have been introduced into the structures. The birefringence of A2Bi2(SO4)2Cl4 (A = NH4, K, and Rb) is calculated to be around 0.05 at 1064 nm, which is sufficient for phase-matching in the SHG process. This value has been increased to 0.112 in CsSbF2SO4 due to the stereo-chemically active lone pair electron effect of (SbO2F2)3− anionic groups.28,29 As we described above, tellurites can undergo plentiful anionic group configurations. We believe that these active lone pair electron units could also improve the birefringence of sulfates effectively. However, such research hasn't been reported yet.
The literature on sulfate tellurites focused on lanthanide compounds and magnetic properties. Two structure types have been found in Ln2M(TeO3)2(SO4) (Ln = Y, Nd, and Sm–Lu; M = Co, Cu, and Zn) due to the lanthanide contraction. The structural types in the Ln–Te(IV)–SO4 (Ln = La–Nd and Sm–Lu) system are even more diverse.30–33 As we know, the Rare Earth cation Y3+ has a closed-shell electron configuration and the f–f transition is prohibited.34–43 To explore new sulfate tellurites with a large birefringence and wide transparent range, yttrium was chosen as the charge balance cation. Our efforts in the Y3+–Te4+–SO42− system result in two new Rare Earth sulfate tellurites, namely, Y3(TeO3)2(SO4)2(OH)(H2O) and Y2(Te4O10)(SO4). They exhibit different 3D frameworks composed of different yttrium tellurite layers bridged by SO4 tetrahedra. Y2(Te4O10)(SO4) contains a unique Te4O10 polyanionic chain formed by one TeO3 and three TeO4 groups with an eight-member polyhedral ring tunnel. Furthermore, the anhydrous Y2(Te4O10)(SO4) features a large birefringence (Δn = 0.149 @ 532 nm), wide energy band gap (Eg = 4.1 eV) and high thermal stability (>700 °C). Here we report their syntheses, crystal structures, and thermal and optical properties.
Experimental section
Reagents and instruments
All the chemicals were analytically pure from commercial sources and used without further purification: TeO2 (Aladdin, >99.99%), Y2O3 (Aladdin, 99.99%), and H2SO4 (Sinopharm, 95.0–98.0%). Powder X-ray diffraction patterns of the two compounds were collected on a Miniflex II powder X-ray diffractometer using Cu Kα radiation at room temperature in the angular range of 2θ = 5–70° with a scan step size of 0.02°. Infrared (IR) spectra were recorded on a Magna 750 FT-IR spectrometer using air as the background in the range of 4000–400 cm−1 with a resolution of 2 cm−1 at room temperature. The UV-vis-NIR spectra were obtained at 2000–200 nm with a PerkinElmer Lambda 900 spectrophotometer using BaSO4 as the reference, and the reflection spectra were converted into an absorption spectrum using the Kubelka–Munk function. Thermogravimetric analysis and differential scanning calorimetry (TG-DSC) were performed with a Netzsch STA 499C. The samples (about 3.0 mg) were placed in alumina crucibles and heated in 20–1200 °C at a rate of 15 °C min−1 under a N2 atmosphere.
Synthesis
The two compounds were obtained by mild hydrothermal reactions. The component parts are as follows: TeO2 (0.319 g, 2 mmol), Y2O3 (0.339 g, 1.5 mmol) and H2SO4 (0.2 mL) for Y3(TeO3)2(SO4)2(OH)(H2O); TeO2 (0.319 g, 2 mmol), Y2O3 (0.226 g, 1.0 mmol) and H2SO4 (0.25 mL) for Y2(Te4O10)(SO4). The mixtures were dispersed in 3 mL deionized water, sealed in an autoclave equipped with a Teflon liner (23 mL), heated at 230 °C for 4 days and then cooled down to 50 °C at a rate of 3 °C h−1. The two products were washed with alcohol and dried in air at room temperature. Transparent crystals of Y3(TeO3)2(SO4)2(OH)(H2O) and Y2(Te4O10)(SO4) were obtained in yields of 32% and 47% (based on Te) respectively. Powder X-ray diffraction of the polycrystalline samples was in good agreement with the patterns generated from the single crystal structures.
Single-crystal X-ray diffraction
Data collections for the two compounds were performed on an Agilent Technologies SuperNova Dual Wavelength CCD diffractometer with graphite-monochromated Mo-Kα radiation (λ = 0.71073 Å). The structures were solved by direct methods and refined by full-matrix least-squares fitting on F2 using the SHELXTL-97 crystallographic software package.44 All non-hydrogen atoms were refined with anisotropic thermal parameters and finally converged for F02 ≥ 2σ(F02). Hydrogen atoms were located at geometrically calculated positions and refined with isotropic thermal parameters. The structural data were also checked for possible missing symmetry with the program PLATON, and no higher symmetry was found.45 The crystallographic data and refinement details for the compounds are given in Table 1. The selected bond lengths are listed in Table S1.†
Table 1 Summary of crystal data and structural refinements for the two compounds
R
1 = ∑||Fo| − |Fc||/∑|Fo| and wR2 = {∑w[(Fo)2 − (Fc)2]2/∑w[(Fo)2]2}1/2.
|
Molecular formula |
Y2(Te4O10)(SO4) |
Y3(TeO3)2(SO4)2(OH)(H2O) |
Formula weight |
944.28 |
845.07 |
Crystal system |
Triclinic |
Monoclinic |
Space group |
P![[1 with combining macron]](https://www.rsc.org/images/entities/char_0031_0304.gif) |
P21/m |
Temperature (K) |
293(2) |
293(2) |
F(000) |
828 |
768 |
a/Å |
6.8140(3) |
5.4419(6) |
b/Å |
9.3229(3) |
15.288(2) |
c/Å |
9.8640(4) |
7.9709(9) |
α (deg) |
89.835(3) |
90 |
β (deg) |
72.490(3) |
99.198(10) |
γ (deg) |
88.200(3) |
90 |
V/Å3 |
597.28(4) |
654.64(14) |
Z
|
2 |
2 |
D
c (g cm−3) |
5.251 |
4.287 |
GOF on F2 |
0.986 |
1.102 |
R
1, wR2[I > 2σ(I)]a |
0.0497, 0.1300 |
0.0386, 0.0833 |
R
1, wR2 (all data)a |
0.0551, 0.1359 |
0.0482, 0.0932 |
Results and discussion
Crystal structure of Y3(TeO3)2(SO4)2(OH)(H2O)
Y3(TeO3)2(SO4)2(OH)(H2O) crystallized in the centrosymmetric space group of P21/m (No. 11). Its asymmetric unit contains two yttrium, one sulfur, one tellurium, and nine oxygen, totaling thirteen unique atoms with Y(1), O(4) and O(5) situated at the m symmetry. O(4) is protonated and O(5) is treated as a coordination water. Y(1) and Y(2) are coordinated with eight oxygen atoms in square face capped trigonal prism and square antiprism geometries respectively. The Y–O distances are in the range of 2.267(5)–2.449(5) Å, which are consistent with those of the reported yttrium tellurites. Te(1) is connected with three oxygen atoms in a triangular pyramid geometry with Te–O bond lengths in the range of 1.847(5)–1.874(4) Å. The S(1) atom is linked with four oxygen atoms to a SO4 tetrahedron with the S–O bond lengths ranging from 1.441(5) to 1.487(6) Å, which are comparable with those of metal sulfates. Bond valence calculations revealed that the valence state of Y, Te, and S atoms should be +3, +4, and +6, respectively. The detailed total bond valences for Y(1), Y(2), Te(1), and S(1) are 3.300, 3.242, 4.118 and 6.102, respectively.
Y3(TeO3)2(SO4)2(OH)(H2O) exhibits a novel 3D framework consisting of 2D yttrium tellurite wave-like layers bridged by a SO4 tetrahedron. As shown in Fig. 1a, Y(2)O8 polyhedra are interconnected into a 1D chain via corner- and edge-sharing. Y(1)O8 is capped on the Y(2) chain via face-sharing, forming a curved yttrium oxide chain along the b-axis. Te(1) is a pentadentate anion group, chelating bidentately with one Y(2) atom and bridging with two Y(1) and two Y(2) atoms (Fig. S3†). The TeO3 groups connected the yttrium oxide chains to a 2D wave-like layer parallel to the ab plane. The discrete SO4 tetrahedra bridged the yttrium tellurite layers to a 3D structure (Fig. 1b and c).
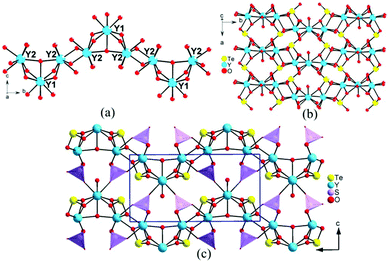 |
| Fig. 1 Yttrium oxide chain (a), 2D yttrium tellurite layer (b) and 3D structure (c) of Y3(TeO3)2(SO4)2(OH)(H2O) (hydrogen atoms are omitted for clarity and SO4 groups are shown in purple tetrahedra). | |
Crystal structure of Y2(Te4O10)(SO4)
Y2(Te4O10)(SO4) crystallized in a centrosymmetric space group of P
(No. 2). There are two yttrium, one sulfur, four tellurium and fourteen oxygen, totaling 21 atoms in its asymmetric unit. Y(1) is linked with eight oxygen atoms in a dodecahedral geometry, while Y(2) is seven-coordinated into a pentagonal bipyramid with Y–O bond distances in the range of 2.148(8)–2.473(7) Å. The four tellurium present two different coordination modes: Te(1) is connected with three oxygen in a triangular pyramid while Te(2)–Te(4) are four-coordinated in TeO4 disphenoids with lone pair electrons occupied at the open sides of the central atoms. The Te–O distances are falling in the range of 1.821(8)–2.495(8) Å. S(1) is linked with four oxygen atoms in a tetrahedral geometry with the S–O distances in the range of 1.448(9)–1.487(8) Å. The oxidation state of Y, Te, and S atoms was proved to be +3, +4, and +6, respectively. The calculated total bond valences for Y(1), Y(2), Te(1), Te(2), Te(3), Te(4) and S(1) are 2.962, 3.101, 3.984, 4.134, 4.021, 4.128 and 6.108, respectively.
Y2(Te4O10)(SO4) features a novel 3D network composed of 2D yttrium tellurite layers bridged by sulfate tetrahedra (Fig. S6†). Te(1)O3 and Te(4)O4 groups are corner-shared into 1D tellurium chains, while Te(2)O4 and Te(3)O4 are interconnected to a Te2O7 dimer. The dimers bridged two of the tellurium chains into an unprecedented Te4O10 1D double-strand with an eight-member polyhedral ring (MPR) tunnel along the a-axis (Fig. 2). There are three different (Te4O10)4− 1D polyanionic chains in the literature. The zigzag Te4O10 chain in Ba2(VO3)[Te4O9(OH)] was formed by one TeO3 and two TeO4 groups, with Te(1)O3 hanging on one side of the chain, while two TeO3 and two TeO4 in HoCl(Te2O5) are linked together into a stair-shaped Te4O10 polyline.46,47 In Nd2(MoO4)(Te4O10), the Te(3)O4 and Te(4)O3 groups are connected into a 1D chain, with Te2O6 dimers suspended on one side (Fig. S5†).48 All the (Te4O10)4− polyanions reported are formed in 1D chains unlike the (Te4O9)2− polymers, which can present 1D chains, 2D layers and even 3D networks.
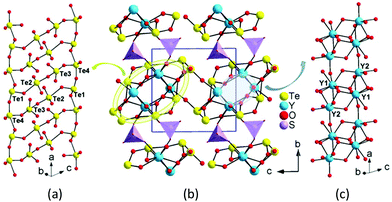 |
| Fig. 2 Te4O10 1D polyanionic double-strand (a), 3D structure (b) and 1D yttrium oxide chain (c) of Y2(Te4O10)(SO4) (SO4 groups are shown in purple tetrahedra). | |
The Y(1)O8 and two Y(2)O7 polyhedra were joined together via corner- and edge-sharing to an yttrium oxide 1D chain along the a-axis. The yttrium 1D chain is located at the 8-MPR tunnel of the Te4O10 double-strand, forming a complicated 1D yttrium tellurite chain along the a-axis. Neighboring yttrium tellurite chains joined together to a 2D yttrium tellurite layer parallel to the ac plane by Te–O–Y bonds. The discrete SO4 tetrahedra bridged the yttrium tellurite layers into a 3D framework by S–O–Y bonds with eight-MPR tunnels along the c-axis. The lone pair electrons of tellurite groups pointed to the voids of the tunnels (Fig. 2).
Thermal analysis
TG-DSC analyses of the two compounds have been performed in the temperature range of 20–1200 °C (Fig. 3). For Y3(TeO3)2(SO4)2(OH)(H2O), it exhibits three steps of weight losses. The first loss occurring at 434–696 °C corresponds to the release of 1.5 molecules of coordination water. The second step between 757 and 1080 °C is equivalent to the loss of two molecules of SO3. The last one can be attributed to the incomplete evaporation of two molecules of TeO2. The anhydrous Y2(Te4O10)(SO4) shows two steps of weight losses. The first loss at 700–852 °C belongs to the volatilization of one molecule of SO3 and the second step from 852 °C to 1200 °C corresponds to the incomplete loss of four molecules of TeO2. These thermal analyses are in accordance with those reported before.49–52
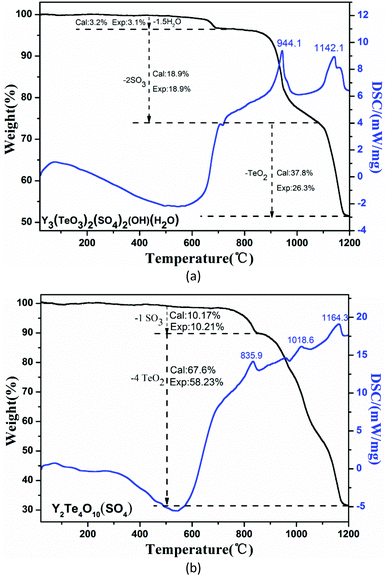 |
| Fig. 3 Thermal analysis curves of Y3(TeO3)2(SO4)2(OH)(H2O) (a) and Y2(Te4O10)(SO4) (b). | |
IR and UV–Vis–NIR spectra
The IR spectra for the two compounds are shown in Fig. S7.† The absorption peaks between 1150 and 1010 cm−1 can be attributed to the stretching vibrations of SO4 tetrahedra and their bending vibrations appear around 620 cm−1. The stretching vibrations of TeO3 triangular pyramids can be seen in the range of 750–700 cm−1. For Y3(TeO3)2(SO4)2(OH)(H2O), the absorption peaks at 1600 cm−1 can be assigned to the bending vibrations of coordination water. These assignments show good agreement with those reported previously.53–56
UV-vis-NIR absorption spectra revealed that the ultraviolet absorption edges of Y3(TeO3)2(SO4)2(OH)(H2O) and Y2(Te4O10)(SO4) can extend to 230 nm and 251 nm, respectively. For Y3(TeO3)2(SO4)2(OH)(H2O), the absorption band around 1452 and 1960 nm should be ascribed to the aqua ligands. The anhydrous Y2(Te4O10)(SO4) shows a wide transparent range and no absorptions can be found between 505 and 2000 nm. The inset optical diffuse-reflectance spectra showed that Y3(TeO3)2(SO4)2(OH)(H2O) and Y2(Te4O10)(SO4) exhibit broad optical bandgaps of 4.4 and 4.1 eV, respectively. From Table S2† we can find that introduction of sulfate is beneficial for the enhancement of the band gaps of yttrium tellurites.57–62 Furthermore, their band gaps are positively related to the S/Te ratios if the elemental compositions of the compounds are similar, which is consistent with our previous report on indium sulfate tellurites [In2(SO4)(TeO3)(OH)2(H2O) (S/Te = 1, 4.86 eV) and In3(SO4)(TeO3)2F3(H2O) (S/Te = 0.5, 4.10 eV)] (Fig. 4).50
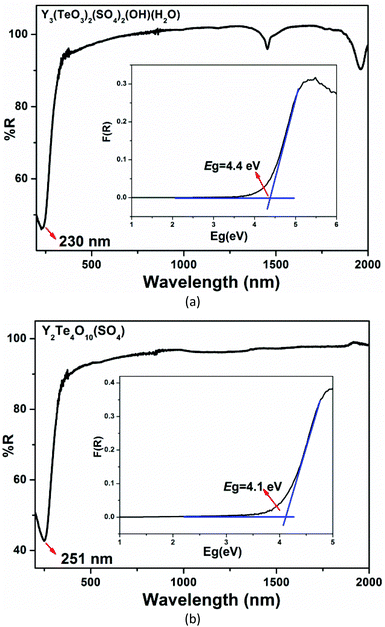 |
| Fig. 4 UV-vis-NIR diffuse reflectance spectra of Y3(TeO3)2(SO4)2(OH)(H2O) (a) and Y2(Te4O10)(SO4) (b). | |
Theoretical calculations
To explore the electronic properties of Y3(TeO3)2(SO4)2(OH)(H2O) and Y2(Te4O10)(SO4), theoretical calculations based on DFT methods were performed. The state energies of the lowest conduction band and the highest valence band are listed in Table S3.†Fig. 5 shows their band structures along the high symmetry points of the first Brillouin zone. The two compounds are indirect band gap structures with band gaps of 1.71 eV and 3.66 eV, respectively. The calculated band gaps are smaller than their experimental values because of the limitation of the GGA methods. So the optical property calculations below are based on the experimental gaps.63
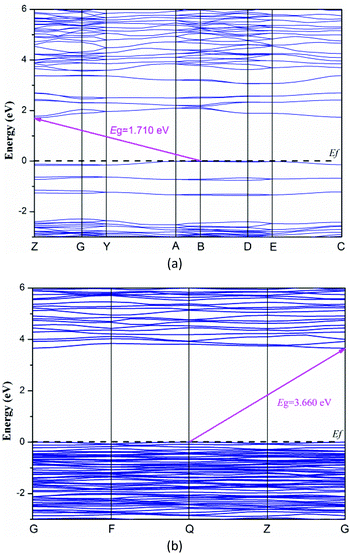 |
| Fig. 5 Band structures of Y3(TeO3)2(SO4)2(OH)(H2O) (a) and Y2(Te4O10)(SO4) (b). | |
The bands can be assigned on the basis of the total and partial density of states as shown in Fig. 6. For convenience, the valence band (VB) and conduction band (CB) of the compounds were divided into 3 and 2 regions respectively. The curves of the two compounds look alike excluding the H 1s state in Y3(TeO3)2(SO4)2(OH)(H2O). VB-3 in the lower energy from −25 to −16 eV originates from O 2s, S 3p, Te 5s5p and H 1s in Y3(TeO3)2(SO4)2(OH)(H2O). VB-2 is mainly constituted by the Te 5s state. The CB-2 in the higher energy is composed of Te 5s and Y 4p4d primarily. To understand more about the atoms’ interaction, we concentrate our attention on VB-1 and CB-1 close to the Fermi level, which account for the majority of the bonding character. In these areas, we can find that the O 2p states match perfectly with Te 5p and S 3p states, which points out the definite Te–O and S–O covalent bonding. Furthermore, the partial density of states of the two compounds disclosed that the tops of the VBs are dominated by O 2p nonbonding states and the bottoms of the CBs are mainly from the empty Te 5p states. Consequently, the band gaps of the two compounds are determined by Te and O atoms.
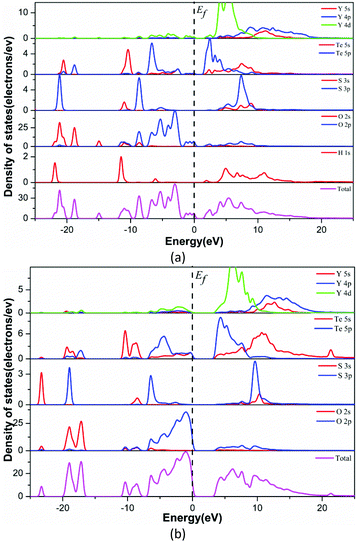 |
| Fig. 6 Total and partial density of states of Y3(TeO3)2(SO4)2(OH)(H2O) (a) and Y2(Te4O10)(SO4) (b). | |
The linear optical response properties were calculated by the complex dielectric function ε(ω) = ε1(ω) + iε2(ω). The dispersion curves of refractive indices based on the formula n2(ω) = ε(ω) displayed strong anisotropy. The frequency dependent refractive indices and birefringences for compounds 1–2 are plotted in Fig. 7. For Y3(TeO3)2(SO4)2(OH)(H2O), the refractive indices follow the order of ny > nx > nz. The birefringences (Δn) are 0.092 and 0.080 at 532 nm and 1064 nm respectively. For Y2(Te4O10)(SO4), the refractive indices are in a different order nx > nz > ny. The birefringences (Δn) are higher, 0.149 and 0.124 at 532 nm and 1064 nm respectively. The Δn values of some recently reported sulfates are listed in Table 2. From the table we can find that the birefringence of Y2(Te4O10)(SO4) is even larger than that of CsSbF2SO4 at 1064 nm.28,29
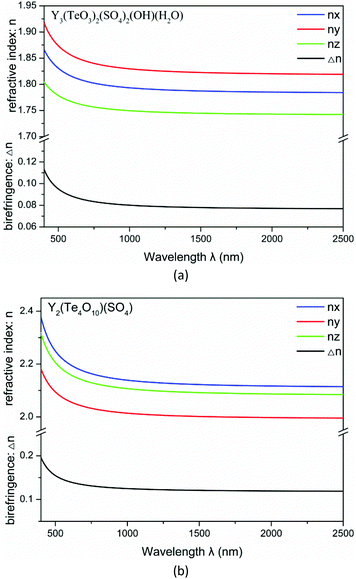 |
| Fig. 7 Calculated refractive indices and birefringence of Y3(TeO3)2(SO4)2(OH)(H2O) (a) and Y2(Te4O10)(SO4) (b). | |
Table 2 Birefringence of some recently reported sulfates
Compounds |
Space group |
Δn @1064 nm |
E
g (eV) |
Ref. |
This work.
|
Rb2Bi2(SO4)2Cl4 |
P212121 |
0.047 |
3.93 |
28
|
(NH4)2Bi2(SO4)2Cl4 |
P212121 |
0.055 |
3.96 |
28
|
K2Bi2(SO4)2Cl4 |
P212121 |
0.056 |
3.92 |
28
|
Y3(TeO3)2(SO4)2(OH)(H2O) |
P21/m |
0.080 |
4.40 |
|
CsSbF2SO4 |
Pna21 |
0.112 |
4.76 |
29
|
Y2(Te4O10)(SO4) |
P![[1 with combining macron]](https://www.rsc.org/images/entities/char_0031_0304.gif) |
0.124 |
4.10 |
|
Conclusions
In summary, two novel yttrium sulfate tellurites, namely, Y3(TeO3)2(SO4)2(OH)(H2O) and Y2(Te4O10)(SO4), have been synthesized by the hydrothermal method successfully. They exhibit two new different 3D structures composed of 2D yttrium tellurite layers bridged by isolated sulfate tetrahedra. Interestingly, the (Te4O10)4− polyanion in Y2(Te4O10)(SO4) features a unique 1D double-strand chain with an eight-member polyhedral ring tunnel along the a-axis. They present a wide energy band gap with Eg values of 4.4 and 4.1 eV respectively. Their birefringences were calculated to be 0.092 and 0.149 at 532 nm respectively. What's more, the anhydrous Y2(Te4O10)(SO4) can remain stable below 700 °C. Therefore, Y2(Te4O10)(SO4) features a large birefringence, wide energy band gap and high thermal stability, which make it a potential candidate as a birefringent material.
Conflicts of interest
There are no conflicts to declare.
Acknowledgements
This work was supported by the National Natural Science Foundation of China (Grant Nos: 91963105, 21773244, 21875248, 21921001 and 22031009), the Strategic Priority Research Program of the Chinese Academy of Sciences (Grant No: XDB20000000), and the NSF of Fujian Province (Grant No: 2018J01025).
References
- W. Q. Lu, Z. L. Gao, X. L. Du, X. X. Tian, Q. Wu, C. G. Li, Y. X. Sun, Y. Liu and X. T. Tao, Shedding Light on the Intrinsic Characteristics of 3D Distorted Fluorite-Type Zirconium Tellurite Single Crystals, Inorg. Chem., 2019, 58, 7794–7802 CrossRef.
- S. Lee, H. Jo and K. M. Ok, Bi2Te2O6(NO3)2(OH)2(H2O): A layered bismuth tellurium nitrate hydroxide with multiple noncentrosymmetric chromophores, J. Solid State Chem., 2019, 271, 298–302 CrossRef CAS.
- K. Stolze, T. Kong, F. O. von Rohr and R. J. Cava, Crystal structure and anisotropic magnetic properties of CaCo4(TeO3)4Cl2, J. Solid State Chem., 2018, 263, 141–147 CrossRef CAS.
- J. W. Purcell, D. L. Long, E. C. Lee, L. Cronin and H. N. Miras, Spontaneous formation of a chiral (Mo2O2S2)2+-based cluster driven by dimeric {Te2O6}-based templates, Dalton Trans., 2018, 47, 6283–6287 RSC.
- W. Q. Lu, Z. L. Gao, Q. Wu, X. X. Tian, Y. X. Sun, Y. Liu and X. T. Tao, Tailored fabrication of a prospective acousto-optic crystal TiTe3O8 endowed with high performance, J. Mater. Chem. C, 2018, 6, 2443–2451 RSC.
- M. F. Lu, H. Jo, S. J. Oh, S. Lee, K. Y. Choi, Y. Yu and K. M. Ok, Syntheses, Structures, and Characterization of Quaternary Tellurites, Li3MTe4O11 (M = Al, Ga, and Fe), Inorg. Chem., 2017, 56, 5873–5879 CrossRef CAS.
- Y. G. Chen, N. Yang, X. X. Jiang, Y. Guo and X. M. Zhang, Pb@Pb8 Basket-like-Cluster-Based Lead Tellurate−Nitrate Kleinman-Forbidden Nonlinear-Optical Crystal: Pb9Te2O13(OH)(NO3)3, Inorg. Chem., 2017, 56, 7900–7906 CrossRef CAS.
- H. Yu, J. Young, H. Wu, W. Zhang, J. M. Rondinelli and P. S. Halasyamani, Electronic, Crystal Chemistry, and Nonlinear Optical Property Relationships in the Dugganite A3B3CD2O14 Family, J. Am. Chem. Soc., 2016, 138, 4984–4989 CrossRef CAS.
- S. W. Bae, J. Yoo, S. Lee, K. Y. Choi and K. M. Ok, Synthesis and Characterization of Three New Layered Vanadium Tellurites, MVTe2O8 (M = Al, Ga, and Mn): Three-Dimensional (3-D) Antiferromagnetic Behavior of MnVTe2O8 with a Zigzag S = 2 Spin Chain, Inorg. Chem., 2016, 55, 1347–1353 CrossRef CAS.
- J. G. Mao, H. L. Jiang and F. Kong, Structures and Properties of Functional Metal Selenites and Tellurites, Inorg. Chem., 2008, 47, 8498–8510 CrossRef CAS.
- T. Wang, Y. G. Chen, Y. Guo, F. Wang, Q. Song, Y. J. Jia and X. M. Zhang, BaLiTe2O5X (X = Cl, Br): mixed alkali/alkaline-earth metal tellurite halides with [Te2O5]∞ chains, Dalton Trans., 2020, 49, 4914–4919 RSC.
- C. Wu, L. H. Li, L. Lin, Z. P. Huang, M. G. Humphrey and C. Zhang, Enhancement of Second-Order Optical Nonlinearity in a Lutetium Selenite by Monodentate Anion Partial Substitution, Chem. Mater., 2020, 32, 3043–3053 CrossRef CAS.
- F. Kong and J. G. Mao, Recent Progress in Selenite and Tellurite Based SHG Materials, Dalton Trans., 2020, 49, 8433–8437 RSC.
- G. H. Zou and K. M. Ok, Novel ultraviolet (UV) nonlinear optical (NLO) materials discovered by chemical substitution-oriented design, Chem. Sci., 2020, 11, 5404–5409 RSC.
- S. J. Han, C. M. Huang, A. Tudi, S. S. Hu, Z. H. Yang and S. L. Pan, β-CsB9O14: A Triple-Layered Borate with Edge-Sharing BO4 Tetrahedra Exhibiting a Short Cutoff Edge and a Large Birefringence, Chem. – Eur. J., 2019, 25, 11614–11619 CrossRef CAS.
- H. X. Fan, G. Peng, C. S. Lin, K. C. Chen, S. D. Yang and N. Ye, Ba(IO3)F: An Alkaline-Earth-Metal Iodate Fluoride Crystal with Large Band Gap and Birefringence, Inorg. Chem., 2020, 59, 7376–7379 CrossRef CAS.
- Y. Wang, B. B. Zhang, Z. H. Yang and S. L. Pan, Cation-Tuned Synthesis of Fluorooxoborates: Towards Optimal Deep-Ultraviolet Nonlinear Optical Materials, Angew. Chem., Int. Ed., 2018, 57, 2150–2154 CrossRef CAS.
- Y. Yang, Y. Qiu, P. F. Gong, L. Kang, G. M. Song, X. M. Liu, J. L. Sun and Z. S. Lin, Lone-Pair Enhanced Birefringence in an Alkaline-Earth Metal Tin(II) Phosphate BaSn2(PO4)2, Chem. – Eur. J., 2019, 25, 5648–5651 CrossRef CAS.
- Y. P. Gong, C. L. Hu, F. Kong and J. G. Mao, Exploration of New Birefringent Crystals in Bismuth d0 Transition Metal Selenites, Chem. – Eur. J., 2019, 25, 3685–3694 CrossRef CAS.
- B. P. Yang, X. H. Zhang, J. Chen, C. L. Hu, Z. Fang, Z. J. Wang and J. G. Mao, A New Iodate-Phosphate Pb2(IO3)(PO4) Achieving Great Improvement in Birefringence Activated by (IO3)− Groups, Chem. Commun., 2019, 56, 635–638 Search PubMed.
- F. F. He, Y. L. Deng, X. Y. Zhao, L. Huang, D. J. Gao, J. Bi, X. Wang and G. H. Zou, RbSbSO4Cl2: an excellent sulfate nonlinear optical material generated due to the synergistic effect of three asymmetric chromophores, J. Mater. Chem. C, 2019, 7, 5748–5754 RSC.
- Y. Q. Li, F. Liang, S. G. Zhao, L. N. Li, Z. Y. Wu, Q. R. Ding, S. Liu, Z. S. Lin, M. C. Hong and J. H. Luo, Two Non-π-Conjugated Deep-UV Nonlinear Optical Sulfates, J. Am. Chem. Soc., 2019, 141, 3833–3837 CrossRef CAS.
- M. Q. Gai, Y. Wang, T. H. Tong, Z. H. Yang and S. L. Pan, ZnIO3F: Zinc Iodate Fluoride with Large Birefringence and Wide Band Gap, Inorg. Chem., 2020, 59, 4172–4175 CrossRef CAS.
- Y. X. Ma, Y. P. Gong, C. L. Hu, J. G. Mao and F. Kong, Three new d10 transition metal selenites containing PO4 tetrahedron: Cd7(HPO4)2(PO4)2(SeO3)2, Cd6(PO4)1.34(SeO3)4.66 and Zn3(HPO4)(SeO3)2(H2O), J. Solid State Chem., 2018, 262, 320–326 CrossRef CAS.
- F. J. Guo, J. Han, J. N. Cheng, Z. H. Yang, T. Abudouwufu, H. W. Yu and S. L. Pan, Cs2Zn5(PO4)4 and Cs3La(PO4)2: Two Cs-Containing Phosphates with Three-Dimensional Frameworks, Eur. J. Inorg. Chem., 2019, 19, 2462–2467 CrossRef.
- Z. Miao, Y. Yang, Z. L. Wei, Z. H. Yang, S. J. Yu and S. L. Pan, NaCa5BO3(SiO4)2 with Interesting Isolated [BO3] and [SiO4] Units in Alkali- and Alkaline-Earth-Metal Borosilicates, Inorg. Chem., 2019, 58, 3937–3943 CrossRef CAS.
- B. L. Wu, C. L. Hu, F. F. Mao, R. L. Tang and J. G. Mao, Highly Polarizable Hg2+ Induced a Strong Second Harmonic Generation Signal and Large Birefringence in LiHgPO4, J. Am. Chem. Soc., 2019, 141, 10188–10192 CrossRef CAS.
- K. C. Chen, Y. Yang, G. Peng, S. D. Yang, T. Yan, H. X. Fan, Z. S. Lin and N. Ye, A2Bi2(SO4)2Cl4 (A = NH4, K, Rb): achieving a subtle balance of the large second harmonic generation effect and sufficient birefringence in sulfate nonlinear optical materials, J. Mater. Chem. C, 2019, 7, 9900–9907 RSC.
- X. H. Dong, L. Huang, C. F. Hu, H. M. Zeng, Z. E. Lin, X. Wang, K. M. Ok and G. H. Zou, CsSbF2SO4: An Excellent Ultraviolet
Nonlinear Optical Sulfate with a KTiOPO4 (KTP)-type Structure, Angew. Chem., Int. Ed., 2019, 58, 6528–6534 CrossRef CAS.
- T. N. Poe, F. D. White, V. Proust, E. M. Villa and M. J. Polinski, [Ag2M(Te2O5)2]SO4 (M = CeIV or ThIV): A New Purely Inorganic d/f Heterometallic Cationic Material, Inorg. Chem., 2018, 57, 4816–4819 CrossRef CAS.
- J. Lin, K. Diefenbach, M. A. Silver, N. S. Dalal and T. E. Albrecht-Schmitt, Structure−Property Correlations in the Heterobimetallic 4f/3d Materials Ln2M(TeO3)2(SO4) (Ln = Y, Nd, Sm, Eu, Gd, Tb, Dy, Ho, Er, Tm, Yb, or Lu; M = Co or Zn), Cryst. Growth Des., 2015, 15, 4606–4615 CrossRef CAS.
- J. Lin, P. Chai, K. Diefenbach, M. Shatruk and T. E. Albrecht-Schmitt, Challenges in the Search for Magnetic Coupling in 3d/4f Materials: Syntheses, Structures, and Magnetic Properties of the Lanthanide Copper Heterobimetallic Compounds, RE2Cu(TeO3)2(SO4)2, Chem. Mater., 2014, 26, 2187–2194 CrossRef CAS.
- J. Lin, K. Diefenbach, J. N. Cross, J. M. Babo and T. E. Albrecht-Schmitt, Thermochromism, the Alexandrite Effect, and Dynamic Jahn−Teller Distortions in Ho2Cu(TeO3)2(SO4)2, Inorg. Chem., 2013, 52, 13278–13281 CrossRef CAS.
- Z. T. Lu, W. J. Fan, Z. Q. Wang, N. Gu, Z. H. Yue, H. G. Xue and S. P. Guo, Second-Order Nonlinear-Optical-Active Selenide Borate YSeBO2: Featuring a [YSeBO2]n Planar Belt, Inorg. Chem., 2020, 59, 7905–7909 CrossRef CAS.
- O. A. Lipina, L. L. Surat, A. Y. Chufarov, A. P. Tyutyunnik, A. N. Enyashin, I. V. Baklanova, K. G. Belova, Y. V. Baklanova and V. G. Zubkov, Structural, electronic, and optical studies of BaRE2Ge3O10 (RE = Y, Sc, Gd–Lu) germanates with a special focus on the [Ge3O10]8− geometry, CrystEngComm, 2019, 21, 6491–6502 RSC.
- Y. F. Li, F. Liang, H. M. Song, W. Liu, Z. S. Lin, G. C. Zhang and Y. C. Wu, Rb7SrY2(B5O10)3: A Rare-Earth Pentaborate with Moderate Second-Harmonic Response and Interesting Phase-Matching Behavior, Inorg. Chem., 2019, 58, 8943–8947 CrossRef CAS.
- Z. Z. Zhang, Y. Wang, H. Li, Z. H. Yang and S. L. Pan, BaB8O12F2: a promising deep-UV birefringent material, Inorg. Chem. Front., 2019, 6, 546–549 RSC.
- Z. Jia, X. X. Jiang, Z. S. Lin and M. J. Xia, PbTeGeO6: polar rosiaite-type germanate featuring a two dimensional layered structure, Dalton Trans., 2018, 47, 16388–16392 RSC.
- Y. H. Kim, T. T. Tran, P. S. Halasyamani and K. M. Ok, Macroscopic polarity control with alkali metal cation size and coordination environment in a series of tin iodates, Inorg. Chem. Front., 2015, 2, 361–368 RSC.
- X. B. Pan, H. P. Wu, S. C. Cheng and Z. P. Wang, Pb3Ba3Zn6(BO3)8 and α-BaZn2(BO3)2:new members of the zincoborates containing two different dimensional Zn–O units, Inorg. Chem. Front., 2020, 7, 101–107 RSC.
- X. X. Wang, X. B. Li, C. L. Hu, F. Kong and J. G. Mao, Ag4Hg(SeO3)2(SeO4): a novel SHG material created in mixed valent selenium oxides by in situ synthesis, Sci. China Mater., 2019, 62, 1821–1830 CrossRef.
- C. Wu, L. H. Li, L. Lin, Z. P. Huang, M. G. Humphrey and C. Zhang, Gd(NO3)(Se2O5)·3H2O: a nitrate–selenite nonlinear optical material with a short ultraviolet cutoff edge, Dalton Trans., 2020, 49, 3253–3259 RSC.
- C. Wu, X. X. Jiang, L. Lin, Z. S. Lin, Z. P. Huang, M. G. Humphrey and C. Zhang, AGa3F6(SeO3)2 (A = Rb, Cs): A New Type of Phase-Matchable Hexagonal Tungsten Oxide Material with Strong
Second-Harmonic Generation Responses, Chem. Mater., 2020, 32, 6906–6915 CrossRef CAS.
-
Rigaku, CrystalStructure Version 3.6.0, Rigaku Corporation, 2002 Search PubMed;
CrystalClear, version 1.3.5, Rigaku Corp., Woodlands, TX, 1999 Search PubMed;
G. M. Sheldrick, SHELXTL, version 5.1, Bruker-AXS, Madison, WI, 1998 Search PubMed.
-
A. L. Spek, PLATON, Utrecht University, Utrecht, The Netherlands, 2001 Search PubMed.
- S. Y. Zhang, C. L. Hu, C. F. Sun and J. G. Mao, Syntheses and Crystal Structures of a Series of Alkaline Earth Vanadium Selenites and Tellurites, Inorg. Chem., 2010, 49, 11627–11636 CrossRef CAS.
- S. F. Meier and T. Schleid, HoClTe2O5: Ein tellurdioxidreiches Holmium(III)-Chlorid-Oxotellurat(IV), Z. Anorg. Allg. Chem., 2003, 629, 1575–1580 CrossRef CAS.
- H. L. Jiang, En. Ma and J. G. Mao, New Luminescent Solids in the Ln−W(Mo)−Te−O−(Cl) Systems, Inorg. Chem., 2007, 46, 7012–7023 CrossRef CAS.
- P. F. Li, F. Kong and J. G. Mao, MII2M3IIIF3(Te6F2O16) (MII=Pb, Ba; MIII=Al, Ga): New mixed anionic tellurites with isolated Te6 coplanar rings, J. Solid State Chem., 2020, 286, 121288 CrossRef CAS.
- Y. P. Gong, Y. X. Ma, S. M. Ying, J. G. Mao and F. Kong, Two Indium Sulfate Tellurites: Centrosymmetric In2(SO4)(TeO3)(OH)2(H2O) and Non-centrosymmetric In3(SO4)(TeO3)2F3(H2O), Inorg. Chem., 2019, 58, 11155–11163 CrossRef CAS.
- F. Kong, S. P. Huang, Z. M. Sun, J. G. Mao and W. D. Cheng, Se2(B2O7): A New Type of Second-Order NLO Material, J. Am. Chem. Soc., 2006, 128, 7750–7751 CrossRef CAS.
- E. P. Lee, S. Y. Song, D. W. Lee and K. M. Ok, New Bismuth Selenium Oxides: Syntheses, Structures, and Characterizations of Centrosymmetric Bi2(SeO3)2(SeO4) and Bi2(TeO3)2(SeO4) and Noncentrosymmetric Bi(SeO3)(HSeO3), Inorg. Chem., 2013, 52, 4097–4103 CrossRef CAS.
- J. J. Zhou, H. P. Wu, H. W. Yu, S. T. Jiang, Z. G. Hu, J. Y. Wang, Y. C. Wu and P. S. Halasyamani, BaF2TeF2(OH)2: A UV Nonlinear Optical Fluorotellurite Material Designed by Band-Gap Engineering, J. Am. Chem. Soc., 2020, 142, 4616–4620 CrossRef CAS.
- F. G. You, F. Liang, Q. Huang, Z. G. Hu, Y. C. Wu and Z. S. Lin, Pb2GaF2(SeO3)2Cl: Band Engineering Strategy by Aliovalent Substitution for Enlarging Bandgap while Keeping Strong Second Harmonic Generation Response, J. Am. Chem. Soc., 2019, 141, 748–752 CrossRef CAS.
- H. X. Tang, Y. X. Zhang, C. Zhuo, R. B. Fu, H. Lin, Z. J. Ma and X. T. Wu, A Niobium Oxyiodate Sulfate with a Strong Second-Harmonic-Generation Response Built by Rational Multi-Component Design, Angew. Chem., Int. Ed., 2019, 58, 3824–3828 CrossRef CAS.
- Q. Wu, H. M. Liu, L. Kang, Z. S. Lin, X. G. Meng, X. G. Chen and J. G. Qin, Rb2SeOCl4·H2O: a polar material among the alkali metal selenite halides with a strong SHG response, Dalton Trans., 2016, 45, 17723–17728 RSC.
- Y. H. Kim, D. W. Lee and K. M. Ok, Noncentrosymmetric YVSe2O8 and Centrosymmetric YVTe2O8: Macroscopic Centricities Influenced by the Size of Lone Pair Cation Linkers, Inorg. Chem., 2014, 53, 1250–1256 CrossRef CAS.
- Y. T. Zhang, Y. Long, X. H. Dong, L. Wang, L. Huang, H. M. Zeng, Z. E. Lin, X. Wang and G. H. Zou, Y8O(OH)15(CO3)3Cl: an excellent short-wave UV nonlinear optical material exhibiting an infrequent three-dimensional inorganic cationic framework, Chem. Commun., 2019, 55, 4538–4541 RSC.
- M. Wen, H. P. Wu, C. Hu, Z. H. Yang and S. L. Pan, Experiment and First-Principles Calculations of A2Mg2TeB2O10 (A=Pb, Ba): Influences of the Cosubstitution on the Structure Transformation and Optical Properties, Inorg. Chem., 2019, 58, 11127–11132 CrossRef CAS.
- X. L. Cao, F. Kong, C. L. Hu and J. G. Mao, A series of novel mercury(I) selenites and tellurites containing SOJT Mo6+ cations, Inorg. Chem. Front., 2014, 1, 761–768 RSC.
- B. L. Wu, C. L. Hu, R. L. Tang, F. F. Mao, J. H. Feng and J. G. Mao, Fluoroborophosphates: a Family of Potential Deep Ultraviolet NLO Materials, Inorg. Chem. Front., 2019, 6, 723–730 RSC.
- Y. P. Gong, C. L. Hu, Y. X. Ma, J. G. Mao and F. Kong, Pb2Cd(SeO3)2X2 (X=Cl, Br): Two Halogenated Selenites with Phase Matchable Second Harmonic Generation, Inorg. Chem. Front., 2019, 11, 3133–3139 RSC.
- D. Vanderbilt, Soft self-consistent pseudopotentials in a generalized eigenvalue formalism, Phys. Rev. B: Condens. Matter Mater. Phys., 1990, 41, 7892–7895 CrossRef.
Footnote |
† Electronic supplementary information (ESI) available: Powder X-ray diffraction patterns, crystal data, infrared spectra, and computational method. CCDC 2032280 and 2032281. For ESI and crystallographic data in CIF or other electronic format see DOI: 10.1039/d0qi01130d |
|
This journal is © the Partner Organisations 2021 |
Click here to see how this site uses Cookies. View our privacy policy here.