DOI:
10.1039/D1RA00926E
(Paper)
RSC Adv., 2021,
11, 30455-30464
Antithrombogenic properties of Tulbaghia violacea aqueous leaf extracts: assessment of platelet activation and whole blood clotting kinetics
Received
3rd February 2021
, Accepted 28th August 2021
First published on 13th September 2021
Abstract
Tulbaghia violacea plant extracts have been investigated for their potential therapeutic effects in the management of various ailments, among which are cardiovascular diseases, due to the wide range of phytocompounds that the plant possesses. One of the major challenges in clinical practice is the inability to control platelet activation and clotting caused by cardiovascular disease interventions. Current treatment methods to inhibit platelet aggregation and thromboxane formation have been associated with major undesirable side effects. This has led to increased research studies on the development of newer and more effective antiplatelet agents. In particular, there has been a growing interest on the potential antiplatelet activity of plant-derived extracts. Hence this study methodically evaluates the anticlotting and antiplatelet properties of T. violacea aqueous leaf extracts. The platelet activity of the plant extracts was assessed using total platelet adhesion, platelet morphology and whole blood clotting kinetics. The 0.1 mg ml−1 T. violacea extract mixed with blood plasma demonstrated the lowest platelet adhesion and activation and also reduced whole blood clotting kinetics. There was a reduction of about 70% in platelet adhesion for the 0.1 mg ml−1 treatment compared to the control in the first 15 min which was supported by morphological characterization under SEM. These observations suggest that T. violacea may be a potential antiplatelet therapeutic agent to inhibit the initial step of platelet adhesion and ultimately reduce the incidence of cardiovascular events.
1. Introduction
Platelets are small anucleate cell fragments produced from nucleated precursor cells known as megakaryocytes in the bone marrow. They are found in the bloodstream and play an important role in vascular integrity and hemostasis regulation.1,2 Platelet activity is oftentimes correlated with the initiation of coagulation cascades. Platelets have a role in the initial biological process of chronic inflammation associated with disease pathology,1 intercellular communication, and immunomodulatory activities.3 Platelets have the basic function of rapidly adhering to damaged blood vessels, and aggregating to form thrombi, thus preventing excessive bleeding. Activated platelets, on the other hand, aggregate at the site of an atherosclerotic plaque rupture or endothelial cell erosion, which stimulates thrombus formation and development of atherothrombotic disease.4,5 Besides thrombosis and haemostasis, platelets are also important in atherothrombotic processes and fibroproliferation, which have been linked to a number of cardiovascular diseases (CVDs).6–8 Cardiovascular diseases (CVDs) are the leading cause of morbidity and mortality worldwide and are responsible for over 17 million deaths annually worldwide.9,10 There are multiple factors that contribute to cardiovascular diseases and this includes the increased ability of platelets to aggregate.11 Platelet activation is a process that occurs when platelets adhere to the exposed collagen, laminin, and von Willebrand factor in the injured vessel. Platelets can also be activated by agonists such as adenosine-5-diphosphate (ADP), thrombin, collagen and thromboxane A2.11–13 Increased platelet activity has been associated with vascular disease and has a key role in haemostasis and thrombosis.14,15
One of the therapeutic strategies for the treatment of platelet-related cardiovascular diseases is interfering with the process of platelet aggregation.16 The activation of blood platelets on the surface of medical implants such as stents causes the devices to fail. Antiplatelet therapy is a procedure of great significance which has been used for treatment of coronary diseases17–19 and to also prevent the formation of clots throughout post-percutaneous coronary interventions (PCIs). Anti-platelet drugs such as acetylsalicylic acid/aspirin (thromboxane inhibitor),6 clopidogrel (adenosine diphosphate antagonists), abciximab (glycoprotein-IIb/IIIa antagonists), warfarin (vitamin K epoxide reductase inhibitor), and heparin (inhibitor of factor IIa) have been used to inhibit the uncontrollable activation of platelets.20
Despite the frequent use of these anti-platelet drugs, they may be unresponsive or cause side effects such as gastric erosions, gastric ulcers, which may result in anaemia and gastric haemorrhage.21,22 This has resulted in the search for other therapeutic antagonists. There is currently a growing interest in the development of newer and more effective antiplatelet agents. This has led to increased research in the evaluation of properties of plant extracts, and their antiplatelet activity with the aim of developing safe and effective antithrombotic drugs from plant-derived extracts.23
Over the years, the use of medicinal plants with a broad range of biologically active constituents has gained popularity in pharmacological research and drug development. This involves a direct approach as therapeutic agents, as templates from which the drugs are produced, or as models to access the active compounds that are present in the plant constituents.24 There is also an annual increase in the number of people that have turned to medicinal plants as they are believed to be free from undesirable side effects however, the appropriate dosage needs to be established to prevent toxic effects.25–28
Tulbaghia violacea is a drought-resistant medicinal plant that stretches from the Eastern Cape, KwaZulu-Natal, Northern Gauteng and Limpopo in South Africa to even as far north as Zimbabwe.29,30 The plant has been assumed to possess biological activities similar to garlic (Allium sativum) since both belong to the same Alliaceae family and have similar bioactive compounds.31–33 However, most of the biological activities demonstrated by garlic remain to be investigated in T. violacea.
There are only a few studies that have reported on the cardiovascular potential of T. violacea plant extracts. Ref. 34 and 35 reported that extracts from T. violacea demonstrated antithrombotic activity but this was dependent on their extraction methods as well as the season of extraction. The studies of ref. 36 and 37 reported that T. violacea reduces systemic arterial blood pressure in the Dahl rat as a result of a decrease in renal AT1 receptor gene expression. Recent studies have concentrated on the ability of a T. violacea extract to decrease the effect of hypertension.38 According to ref. 39, an organic bulb extract of T. violacea has advantageous effects on the cardiovascular system by reducing fibrinogen levels, extending the activated partial thromboplastin time (APTT) and regulation of platelet activation through the GPIIb receptor that are found on platelets. A recent study by ref. 40 demonstrated improved blood compatibility when polycaprolactone nanofibers fabricated with and without the addition of T. violacea plant extracts was used as the contact surfaces. 100 μg ml−1 and 1000 μg ml−1 of T. violacea plant extracts were then added to each nanofiber to assess the antiplatelet activity of the fabricated materials. The results indicated that when lower concentrations of T. violacea plant extracts were used, the overall platelet activity was significantly reduced for all fabricated scaffold.
In this study, the responses of platelets and red blood cells incorporated with T. violacea aqueous leaf extracts was evaluated using platelet adhesion, platelet morphology and whole blood clotting kinetics for better understanding of the effect of the plant extracts on platelet activity.
2. Materials and methods
2.1. Preparation of extracts
Tulbaghia violacea was collected from Vanderbijlpark, South Africa and identified (ST0008) as described by ref. 41. The leaves of T. violacea were gently washed with distilled water to remove the dust and cut into small pieces, frozen at −20 °C, lyophilized and lastly pulverized into a fine powder. The crude water extracts from the leaves was then prepared by adding 30 g of the pulverized plant material to 600 ml of distilled water and boiled at 100 °C for 10 min. The mixture was then allowed to cool and then filtered through a 0.45 μm Whatman® filter paper. Afterwards, the filtrate was frozen and lyophilized to form a powder. A percentage yield of 42.3% was obtained from the filtrate. A stock solution of 100 mg ml−1 in distilled water was then prepared and stored at −20 °C until use.
2.2. Quantitative phytochemical screening
Quantitative phytochemical analysis was conducted for total phenols, total tannins and total flavonoids content.42–44 For the quantification, three concentrations of 0.1, 1.0 and 10 mg ml−1 were prepared from the stock solution and used for the subsequent assays.
2.2.1. Determination of total phenolic content (TPC). The total phenolic acid content of the aqueous extracts was determined using the Folin–Ciocalteu reagent as described by ref. 43. Aliquots of 0.5 ml of the dilute water extracts (0.1, 1.0 and 10 mg ml−1) and gallic acid of standard 1 mg ml−1 were mixed with 5 ml of Folin–Ciocalteu reagent (1
:
10 diluted with distilled water) and 4 ml of aqueous 7.5% sodium carbonate (Na2CO3). The mixture was allowed to stand for 15 min and total phenolic acid content was determined spectrophotometrically at 760 nm using a Genesys 10S UV-Vis spectrophotometer. A standard curve was prepared using 0, 50, 100, 150, 200, and 250 mg ml−1 solutions of gallic acid in methanol. The total phenolic acid contents are expressed in milligrams of gallic acid equivalents per gram of dry material.
2.2.2. Determination of total tannins content (TTC). The tannin content in the leaf extracts of T. violacea was determined using Folin–Ciocalteu method as described by ref. 44. Aliquot extracts of 100 μl (0.1, 1.0 and 10 mg ml−1) were added to 750 μl of distilled water, 500 μl of Folin–Ciocateu reagent and 1 ml of 35% sodium carbonate (Na2CO3). The mixture was shaken vigorously after diluting to 10 ml of distilled water. The mixture was incubated for 30 min at room temperature and the absorbance was read at 725 nm. Distilled water was used as blank. Gallic acid standard solutions were prepared as described above for TPC. The total tannins content was expressed as mg GAE per g dry matter as calculated from the prepared standard curve with 0–100 mg per milliliter of GA.45
2.2.3. Determination of total flavonoid content (TFC). The total flavonoid content of the leaf extracts was determined using a colorimetric assay described by ref. 42. Aqueous extracts (0.5 ml) of 0.1, 1.0 and 10 mg ml−1 were mixed with 0.5 ml of aluminium chloride (1.2% w/v) and 0.5 ml potassium acetate (120 mM). The mixture was allowed to stand for 30 min at room temperature and then the absorbance was measured at 415 nm. Quercetin was used as a standard (0.5 g l−1). The flavonoid content was expressed in milligrams of quercetin equivalents per gram of dry material (mg QE per g).46
2.3. Antioxidant activity
2.3.1. DPPH free radical scavenging assay. The method given by ref. 47 was followed to determine the hydrogen donating capacity of different concentrations of T. violacea water extracts using 2,2′-diphenyl-1-picrylhydrazyl radical as a substrate. Tulbaghia violacea water extracts (1 ml) with concentration ranging from 0.01 to 10 mg ml−1, were mixed with 1 ml of a 0.12 mM DPPH solution. After shaking, the mixture was incubated at ambient temperature in the dark for 30 min, and then the absorbance was measured at 517 nm using a UV-160U spectrometer (PG instruments, Leicestershire, UK). Distilled water was used as a negative control and L-ascorbic acid was used as a positive control. All assays were done in triplicates. The radical scavenging activity was determined as the percentage of inhibition using eqn (1) below: |
% Scavenging activity = (Acontrol − Atest)/Acontrol × 100
| (1) |
where Acontrol is the absorbance of the control (DPPH solution without any sample) and Atest is the absorbance of the test sample (DPPH solution plus the sample). The inhibitory concentration (IC50) value is the concentration of the sample that is required to scavenge 50% of the DPPH free radicals.
2.3.2. 2,2-Azino-bis(3-ethylbenzthiazoline-6-sulfonic acid) (ABTS) assay. The aqueous plant extracts were prepared in distilled water with concentrations ranging from 0.01 mg ml−1 to 10 mg ml−1. L-Ascorbic acid was used as a positive control and distilled water was used as a negative control. A 7 mM stock solution (192 mg in 50 ml distilled water) of ABTS was prepared in double-distilled water. The ABTS radical cation was then prepared by adding 88 μl of 140 mM potassium persulfate to 5 ml of ABTS. This solution was stored in the dark for 12–16 h to stabilize it before use. Shortly before conducting the assay, the concentrated ABTS+ solution was placed in a cuvette and diluted with cold ethanol to a final absorbance of 0.70 ± 0.02 at 734 nm. The total scavenging capacities of the extracts were quantified by addition of 1 ml of ABTS+ to 50 μl of each plant extract. The solutions were heated on a heating block at 37 °C for 4 min, after which the absorbance was recorded at 734 nm using a spectrophotometer. All assays were performed in triplicates and the percentage of inhibition was calculated in the same manner as in Section 2.3.1. using eqn (1).48
2.4. Platelet adhesion and activation
Platelet adhesion and activation studies were conducted following a modified procedure described in ref. 41 and 49–51. Initially, blood was drawn from a healthy donor into standard 6 ml vacuum tubes that were coated with the anticoagulant EDTA. The first vial of drawn blood (about 6 ml) was disposed so as to avoid any skin plug and to remove the locally activated platelets produced by insertion of the needle. The subsequent blood vials were centrifuged at 150 g for 15 min to separate the plasma from the red blood cells. The plasma was then pooled together into fresh tubes and used immediately for adhesion and activation studies. Three sets of experiments were performed with aqueous leaf extracts of T. violacea: (i) plasma mixed with 0.1 mg ml−1 extract, (ii) plasma mixed with 1.0 mg ml−1 of extract and (iii) plasma without any plant extract (experimental control). The dilution factor of the plasma to the plant extract was a 10 fold.
The platelet adhesion and activation experiments were conducted onto tissue culture polystyrene (PS) discs (Greiner Bio-One GmbH, Frickenhausen, Germany). The PS discs were first sterilized by sonicating in 70% ethanol for 15 min, followed by washing twice with PBS buffer, air drying and finally exposed to UV light for 30 min. The PS discs were then incubated with 0.5 ml of freshly pooled plasma (control), plasma added at a concentration of 0.1 mg ml−1 and 1.0 mg ml−1 of extract in a 24-well plate at 37 °C and 5% CO2 on a horizontal shaker plate at 100 rpm for 2 h. After 2 h of incubation, the supernatant plasma was carefully removed by aspirating then gently rinsed twice with PBS buffer. Half of the PS discs were moved to a new 24-well plate into which 300 μl of 5 mM calcein-AM solution in PBS buffer was added and the plate was incubated for 20 min at room temperature. The PS discs were then rinsed with PBS and platelets were instantly imaged with the help of a fluorescence microscope containing high efficiency filter set 62 HE BP 474/28 (green) (Zeiss, Jena, Germany).
2.5. Platelet morphology: scanning electron microscopy (SEM)
The platelet–leukocyte morphology was conducted with the aid of a scanning electron microscopy (SEM) (JEOL JSM-6500F, Peabody, MA, USA) to conjure up an image of the platelet–leukocyte interaction with the PS disc surfaces in the presence and absence of T. violacea extracts. The remaining half of the PS discs were then transferred to a clean Petri dish with the aim of fixing and dehydrating the platelets onto the surfaces. The platelets were fixed by incubating the PS discs in a solution of primary fixative (3% glutaraldehyde (Sigma), 0.1 M sodium cacodylate (Polysciences, Warrington, PA, USA), and 0.1 M sucrose (Sigma)) for 45 min. The discs were then moved to a solution of secondary fixative (primary fixative without glutaraldehyde) and incubated for 10 min. Thereafter, dehydration was carried out by incubating the discs for a period of 10 min each in consecutive solutions of increasing ethanol concentrations (35%, 50%, 70%, 95%, and 100%). The discs were then air dried overnight and stored in a desiccator before they can be imaged using SEM at 15 kV after coating with 10 nm layer of gold. A Denton-Vacuum Desk II Au sputter deposition system was used to coat the discs with gold, the default operating pressure used was 100 mTorr and the operating current was 30 mA. Standard routine SEM was conducted following the studies of ref. 40 and 52–56. Each experiment was conducted in triplicate with 3 different platelet populations (n = 3). For both fluorescence and SEM, 5 images were analysed per sample with a total of 15 images per population.
2.6. Whole blood clotting kinetics
The influence of the antithrombogenic properties of the T. violacea aqueous extracts on the whole blood clotting kinetics was investigated using whole human blood acquired from a non-smoking healthy volunteer and drawn using venepuncture following the protocol described by ref. 57 and 58. Whole blood was drawn into standard 3 ml sterile centrifuge tubes without any anti-coagulants then, 7 μl of this blood was immediately placed onto the PS disc and the droplet was allowed to clot for up to 60 min. For the Experimental section, 3 ml of this blood was mixed with freshly prepared T. violacea plant extract in deoxygenated DI water (net concentrations of 0.1 mg ml−1 and 1.0 mg ml−1 blood). Seven microliters of this mixture was instantly dropped onto the surface of sterilized PS disc placed in a 24-well plate. The blood was allowed to clot on the PS discs for up to 60 min and the free haemoglobin concentration was measured at regular intervals (0, 15, 30 and 60 minutes) and represented a measure of the extend of blood clotting. After the specific clotting periods, the discs were transferred into a different 24-well plate and immersed in 500 μl of DI water. The discs were then gently agitated for 30 s and left in the DI water for another 5 min to lyse the cells and release the free haemoglobin from red blood cells. About 300 μl of the blood sample was then transferred to a 96-well plate and the free haemoglobin content was measured at a wavelength of 540 nm using a plate reader. The value of absorbance is directly proportional to the amount of free haemoglobin in DI water which directly correlates to the level of blood clotting on the PS disc surfaces. The experiments were repeated on multiple days using the blood drawn from the same donor to assess reproducibility as well as reliability of the method and obtained results.
2.7. Statistical analysis
All samples experiments were assayed in triplicate n = 3. A two-way ANOVA was performed to determine the significance of platelet counts. Significance was set at p value < 0.05. All statistics are presented here as a mean ± standard deviation. Graphpad prism 8 and ImageJ software were used for analysis.
3. Results and discussion
3.1. Quantitative phytochemical screening
The total phenolic content (TPC), total tannin content (TTC) and total flavonoid content (TFC) of the T. violacea water extracts are shown in Table 1. In general, the total contents of phenolic acids, tannins and flavonoids increased as the concentration of plant material increased.
Table 1 Total phenolic content (TPC), total tannin content (TTC) and total flavonoid content (TFC) from Tulbaghia violacea water extractsa
Concentration (mg ml−1) |
TPC (mg of GAE per g of dry extract) ± SEM |
TTC (mg of GAE per g of dry extract) ± SEM |
TFC (mg of QE per g of dry extract) ± SEM |
Data represents the mean ± SD mg of gallic acid equivalent per gram of dry weight (mg GAE per g) and quercetin equivalent per gram of dry weight (mg QE per g) of the extracts, n = 3. * indicates statistically significant groups according to the t-test: two-sample assuming equal variance (p < 0.05). |
0.1 |
0.0646 ± 0.010* |
0.0010 ± 9.33 × 10−5* |
0.030825 ± 0.0021* |
1.0 |
0.2615 ± 0.011* |
0.0046 ± 0.0001* |
0.126665 ± 0.0021* |
10 |
2.003 ± 0.022* |
0.031 ± 0.0009* |
0.9116 ± 0.0979* |
3.1.1. Total phenolic content (TPC). This study showed that the leaf extracts of T. violacea have a higher content of phenolic compounds than those of tannins and flavonoids at every concentration of plant material that was used (Table 1). These results are similar to previous reports that showed a high percentage of phenolic compound in the leaves of T. violacea.59,60 Phenolic compounds have been reported to be the main source of natural antioxidants in fruits, vegetables, nuts, seeds, flowers and some herbs and are also responsible for their colour and sensory properties such as bitterness and astringency.61,62 According to ref. 63, phenolic compounds are strong antioxidants thus, preventing the influence of free radicals and ROS which is the basis of several chronic human infections. The presence of phenolic compounds in T. violacea indicates that it has potential use in pharmacology and needs to be investigated further.
3.1.2. Total tannin content (TTC). Tannins are water-soluble antioxidants with molecular weight of 500–3000 g mol−1 and mainly present in plants. Tannins have the ability to condense proteins and alkaloids.64,65 Tannins isolated from various plant sources have been reported to inhibit a number of digestive enzymes such as trypsin, α-amylase and lipase in vitro.66 The presence and quantity of tannic compounds in T. violacea leaves is significant in confirming the antioxidative properties of the leaves. To the best of our knowledge, this is the first study that has evaluated the total tannic content of the water extracts of T. violacea.
3.1.3. Total flavonoid content (TFC). For the total flavonoid content (Table 1), a lower quantity was observed when compared to the total phenolics in the T. violacea leaves which is in agreement with previous reports.59,60 This may be due to the fact that polar flavonoids such as flavones and isoflavones are more soluble in ether and chloroform44 compared to water which was used as a solvent in this study. Flavonoids are polyphenolic compounds present in most plants and are composed of a number of hydroxyl groups attached to aromatic ring structures that determines its antioxidative properties. They are the most common and widely distributed group of phenolic compounds and are known to prevent free radicals associated damage through direct scavenging of the free radicals and by inhibiting enzymes involved in free radical production.67
3.2. Antioxidant activity
There has been a growing interest for natural antioxidants and their health benefits.68 Antioxidant-based drug formulations are used for the prevention and treatment of many complex diseases. Plants are a major source of natural antioxidants and produce a wide range of secondary metabolites with antioxidative activities that have therapeutic potential.69 Polyphenols have been identified as the most abundant secondary metabolite in plants.70 The antioxidant activity of polyphenols is attributed to their redox properties which establishes their activity as reducing agents, hydrogen donors, singlet oxygen quenchers, metal chelators and reductants of ferryl.71 Medicinal plants are commonly rich in phenolic compounds such as flavonoids, phenolic acids, stilbenes, tannins, coumarins, lignans and lignins. These compounds have multiple biological effects including antioxidant activity.72,73 The presence of antioxidants in plant material has been suggested to have potential in the prevention of several chronic diseases, namely, cancer, diabetes, cardiovascular disease74 and osteoporosis.75 Tulbaghia violacea possesses a wide range of compounds including flavonoids, phenolics, tannins and coumarins.59
The percentage DPPH and ABTS activity is illustrated in Fig. 1 and 2, respectively. There was a concentration dependent increase in the antioxidant activity in both the DPPH and ABTS assays. This may be attributed to the presence of phenols, tannins and flavonoids in the plant extracts (Table 1). In the DPPH method, the percentage inhibition of the water extract was in the range of 55.28–86.31% (Fig. 1). The highest concentration of plant extract at 10 mg ml−1 showed the highest percentage inhibition value (86.31%) as shown in Fig. 1.
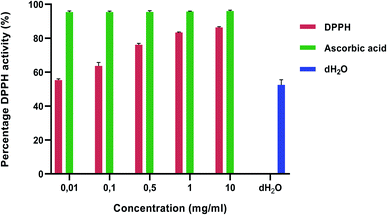 |
| Fig. 1 DPPH scavenging activities of Tulbaghia violacea water extracts. Values are expressed as mean ± SD (n = 3). Ascorbic acid and distilled water was used as a standard and control, respectively. | |
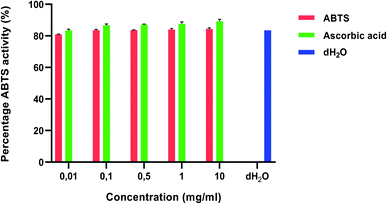 |
| Fig. 2 ABTS scavenging activities of Tulbaghia violacea water extracts. Values are expressed as mean ± SD (n = 3). Ascorbic acid and distilled water was used as a standard and control, respectively. | |
With the ABTS activity (Fig. 2), the percentage inhibition of the water extracts was in the range of 80.90–84.38% and the highest concentration of 10 mg ml−1 showed the highest percentage inhibition value (84.38%). These values were low when compared to the ascorbic acid control. These results are similar to those of ref. 76 and 60 who reported on a dose dependent increase in antioxidant activity which was lower than the ascorbic acid control. According to ref. 76, the antioxidant activity is mainly due to the potential of the T. violacea essential oils to prevent the generation of free radicals through Fe2+ chelation as opposed to pre-existing free radical scavengers.
Oxidative stress has been identified as the major cause for the development and progression of a number of life-threatening diseases, among which are neurodenerative and cardiovascular diseases. The presence of antioxidants in plant material suggests that the plant has the ability to decrease ROS-induced oxidative damage77,78 and will enable the plant to play a role in the prevention of heart diseases, cancer, DNA degeneration, pulmonary disease, and neurological disorder.79
The concentration of the samples required to scavenge 50% of the peroxide radicals, the IC50, was used to examine the antioxidant effectiveness of the samples (Table 2). A low IC50 confirms that the plant extract is a strong scavenger of free radicals.80 This means that the lower the IC50, the greater the overall effectiveness of the suspected antioxidant sample in question. The results revealed that the water extracts of T. violacea showed significant antioxidant effectiveness with IC50 values of 0.49 and 0.24 mg ml−1 for DPPH and ABTS, respectively. L-Ascorbic acid produced the lowest IC50 values of 0.22 and 0.27 mg ml−1 for DPPH and ABTS respectively (Table 2). Antioxidant activity was reported to be higher in the ABTS than in the DPPH assay.
Table 2 IC50 values of the crude water extracts of Tulbaghia violacea
Sample |
DPPH (mg ml−1) |
ABTS (mg ml−1) |
log IC50 |
1/log IC50 |
log IC50 |
1/log IC50 |
Tulbaghia violacea |
0.49 |
2.04 |
0.24 |
4.12 |
L-Ascorbic acid |
0.22 |
4.55 |
0.27 |
3.70 |
The results of this study are different from those recorded by ref. 60 who recorded lower IC50 values of 0.08 and 0.003 mg ml−1 for DPPH and ABTS, respectively using crude acetone extracts. The higher antioxidant activity reported by ref. 60 may be attributed to the ability of acetone to extract more phenolic compounds compared to that of water.81,82 Ref. 83 and 76 also reported higher antioxidant activity compared to the results of this study and this may be due to the fact that crude extracts of T. violacea were used instead of pure compounds isolated from the plant extracts which may have degraded some plant-derived compounds that are most effective when pure.76,83
3.3. Platelet adhesion and activation
The term thrombogenicity is still not yet clearly defined however, it includes the activation, secretion, adherence and aggregation of platelets.84 One of the major reasons for the failure of blood-contacting medical devices such as vascular grafts, stents, and heart valves is thrombosis.85,86 Thrombosis can be shown/demonstrated by the level of platelet adhesion which is the initial step in blood coagulation.58
For this study, freshly pooled plasma isolated from whole human blood was treated with 0.1 mg ml−1 and 1.0 mg ml−1 of T. violacea leaf aqueous extracts. The research investigated the adhesion of platelets within the treated and untreated plasma onto polystyrene surfaces after 2 h of contact time. The platelets were then stained with calcein-AM and platelet adhesion was evaluated by examining surfaces under a fluorescence microscope. Fluorescence microscopy was evaluated at 20× magnification and the cell coverage on surfaces was analysed using ImageJ software (Fig. 3A–C). This study found that there was a significant reduction in the number of platelets that adhered on the polystyrene surfaces treated with plasma mixed with 0.1 mg ml−1 plant extract (Fig. 3B) compared to the untreated group (Fig. 3A). On the contrary, treatment with 1.0 mg ml−1 T. violacea extract (Fig. 3C) showed a great increase in the number of platelets that adhered to polystyrene surfaces and this was even higher than the untreated sample (Fig. 3A).
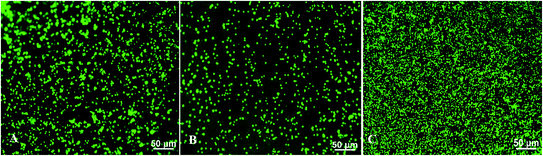 |
| Fig. 3 Representative fluorescence microscopy images of adhered platelets on polystyrene stained with calcein-AM as shown in the control (A), 0.1 mg ml−1 (B) and 1.0 mg ml−1 (C) of aqueous leaf extracts of T. violacea. | |
The platelets coverage analysed using the ImageJ software from fluorescence images in Fig. 4 showed a nearly 69% decrease in cell coverage with plasma mixed with 0.1 mg ml−1 compared to the untreated platelets. One of the reasons for the decrease in platelets coverage may be that the 0.1 mg ml−1 T. violacea leaf extract hindered platelet aggregation and adhesion to the polystyrene surfaces. The increase of nearly 58% in platelet adherence when the plasma was mixed with 1.0 mg ml−1 of extract may be due to platelet cytotoxicity which may be cause by oxidative stress among other factors. Under high shear stress conditions platelets are well-known to become activated and enter a state in which they are sensitive to endogenous agonists, chemicals, or damage,87–90 indicating possible direct platelet cytotoxicity of T. violacea plant extracts at higher concentrations. The cytotoxicity of T. violacea plant extracts at higher concentrations was confirmed by ref. 54. The results obtained in this study are not congruent with the observations reported by ref. 39 who reported a lower platelet count for a concentration of 1.0 mg ml−1 leaf aqueous extract treated plasma when compared to the untreated group and their lowest concentration of 250 μg ml−1. The discrepancies in the results between the study done by ref. 39 and this study may be due to the variation in the preparation of the extracts which may have resulted in the extraction of different compounds thus producing different biological activities.91,92
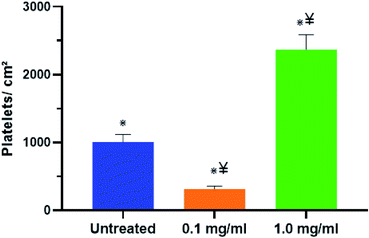 |
| Fig. 4 Quantity of adhered platelets stained with calcein-AM on polystyrene with control, 0.1 mg ml−1 and 1.0 mg ml−1 T. violacea aqueous leaf extracts treatments. There was a significant difference between the untreated group and the two treatment groups of 0.1 mg ml−1, and 1.0 mg ml−1. There was also a significant difference between the 0.1 mg ml−1, and 1.0 mg ml−1 treatment groups. Error bars indicate standard deviation. ⋇ indicates significance with untreated group and ¥ indicates significance with the 0.1 mg ml−1 group (p < 0.05). | |
3.4. Platelet morphology: scanning electron microscopy (SEM)
SEM imaging was then used to study the morphological changes produced by the T. violacea plant extracts on cellular functionality.93 Platelet activation and blood coagulation are mutually dependent processes in haemostasis and thrombosis.93 This may in turn cause arterial thrombosis and acute coronary syndromes due to the formation of atherosclerotic plaques.7,94 In particular, platelets play a key role as inflammatory mediators by delivering activating signals to target cells including endothelial cells and myelomonocytic cells which may lead to amplification of inflammation.90,95,96 There is thus a need to develop materials that can prevent initial platelet activation with the ultimate aim of inhibiting thrombotic events. The SEM study was undertaken to evaluate the morphology of platelet activation after 2 h of surface contact time (Fig. 5A–F). At a lower magnification of 500× (Fig. 5A–C), the platelets appeared more clustered with the 1.0 mg ml−1 (Fig. 5C) producing an almost complete surface coverage whereas with the 0.1 mg ml−1 (Fig. 5B) there was minimal surface coverage of the adhered platelet to the PS surfaces. These results may be attributed to the ability of the higher concentration of T. violacea plant extract to support high platelet adhesion which may in turn result in thrombosis. Similar to the fluorescence microscopy results, there was a higher number of adhered platelets with the 1.0 mg ml−1 treatment compared to the control while the 0.1 mg ml−1 treatment had the lowest number of adhered platelets. At a higher magnification of 2000× (Fig. 5D–F), more detailed morphology of the adhered platelets was visible. The 0.1 mg ml−1 (Fig. 5E) showed formation of a few small pseudopodia but no signs of modification of the pseudopodia or the platelet shape was visible suggesting that the 0.1 mg ml−1 treatment prevented platelet activation and maintained the platelet morphology during the contact period. For the 1.0 mg ml−1 (Fig. 5F) a loss of the discoid platelet shape and formation of long pseudopodia connecting the platelets was observed with more cells covering the surface area indicating platelet activation. Similar to the results observed by ref. 40, when the lower concentration of 0.1 mg ml−1 of T. violacea plant extracts was used, the platelet activity was significantly lower compared to the 1.0 mg ml−1 and the untreated groups. This suggests that the plant possesses antithrombogenic properties, particularly at lower concentrations irrespective of the surface material as seen in this study. Polyphenols have been reported to have an effect on platelet activation, adhesion, degranulation, and aggregation by targeting specific thrombogenic pathways such as P2Y1/P2Y12–ADP and glycoprotein VI (GPVI)–collagen among many others.97 Cardiac glycosides which have also been reported by several studies to be present in T. violacea plant extracts59,60 cause changes in intracellular calcium concentration by inhibiting Na/K-ATPase. An increase in intracellular calcium plays a crucial part in platelet activation, and studies have reported that high concentrations of cardiac glycosides activate platelets in vitro.98,99
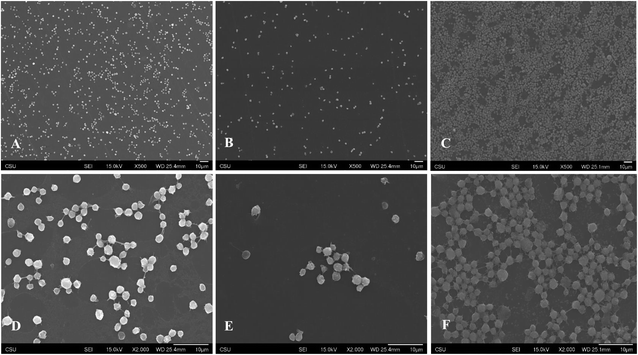 |
| Fig. 5 Representative SEM images of adhered and activated platelets on polystyrene control ((A) 500×, (D) 2000×), 0.1 mg ml−1 ((B) 500×, (E) 2000×) and 1.0 mg ml−1 ((C) 500×, (F) 2000×). | |
3.5. Whole blood clotting kinetics
Blood clotting is a complex process that involves the activation of the clotting cascade.100 When examining a blood-dilating material as a potential precursor for antithrombogenic therapies, the whole blood clotting kinetics should be evaluated as it plays a role in the success or rejection of the material.58 In this study, the absorbance of the free haemoglobin concentration was measured in the control, 0.1 mg ml−1 and 1.0 mg ml−1 treatments for a period of 60 minutes. The results indicated that blood clotting was delayed significantly from time 0 to time 15 min with the 0.1 mg ml−1 treatment when compared to the control and 1.0 mg ml−1 treatments (Fig. 6). The untreated whole blood showed a nearly 70% reduction in the free haemoglobin within the initial 15 min suggesting that there was a significant amount of clotting within that period. This time frame is supported by ref. 58 and 101 who indicated that the maximum rate of thrombin production in untreated whole blood can be observed at time points beyond 7 minutes. However, with the 0.1 mg ml−1 extract treatment, there was a reduction in the free haemoglobin of about 55% in the initial 15 min when compared to the control. After a 60 min clotting period there was an 84% reduction in the free haemoglobin, which was significantly higher than that observed with the untreated and 1.0 mg ml−1 treatments. The experiment was repeated three times using blood from the same donor and a similar trend of results was observed. These results showed delayed clotting kinetics with the 0.1 mg ml−1 treatment thus confirming the anticlotting properties of T. violacea aqueous leaf extracts when used at lower concentrations. This observation may be attributed to the presence of polyphenols reported in this study and by ref. 59. Plant polyphenols have been identified as a natural source of antiplatelet and antithrombotic agents. Aside from their well-known anti-oxidative properties, current research studies have indicated that polyphenols have the ability to inhibit enzymes by regulating different pathways underlying CVD, as well as extracellular matrix degradation, inflammatory response and cell death.102–104
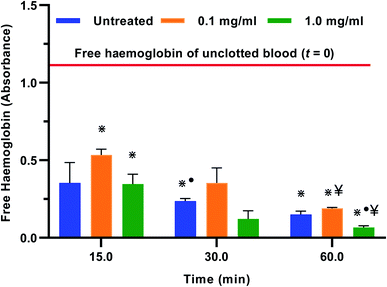 |
| Fig. 6 Measurements of free haemoglobin concentration for the control, 0.1 mg ml−1 and 1.0 mg ml−1 T. violacea aqueous leaf extracts treatments for 60 min. At 15 min there were significant differences between 0.1 mg ml−1 and 1.0 mg ml−1 treatments. The 0.1 mg ml−1 group at 15 min was also significant to the untreated group after 30 min, and all treatment groups after 60 min. There was a significant difference between the untreated group at 30 min and the 1.0 mg ml−1 group at 60 min. There was also a significant difference between the 0.1 mg ml−1 and the 1.0 mg ml−1 group after the 60 min time point. ⋇ indicates significance with the 0.1 mg mg−1 at 15 min, ● indicates significance with the 1.0 mg ml−1 group at 30 min and ¥ indicates significance with the 0.1 mg mg−1 at 60 min (p < 0.05). | |
4. Conclusion
This study was undertaken to assess the potential of aqueous extracts of T. violacea as an antiplatelet and anticlotting agent. The biological activities reported in this study may be attributed to the presence of the quantified polyphenols as well as the rich phytochemical profile that T. violacea possesses. Tulbaghia violacea water extracts showed strong antioxidant activity against DPPH and ABTS radicals. The high percentage of antioxidant activity suggests that T. violacea plant extracts have the ability to be used as antioxidant agents to eliminate free radical intermediates which are responsible for the progression of a variety of ailments including cardiovascular diseases. The results of the fluorescence microscopy and SEM indicated that at 0.1 mg ml−1 of T. violacea aqueous leaf extracts, platelet adhesion and activation decreased significantly. With the whole blood clotting kinetics, there was a significant increase in free haemoglobin concentration for the 0.1 mg ml−1 when compared to the control and the 1.0 mg ml−1 groups. Because T. violacea is frequently used in traditional South African medicine, this study sheds light on the preliminary cardiovascular potential of water extracts of the plant, laying the groundwork for future research comparable to that done on garlic. Future work on the antiplatelet therapeutic potential of T. violacea should focus on the extraction of polyphenols and cardiac glycoside identified in the plant extract and how these influence the biological activity observed.
Ethical statement
The procedure for blood drawing from healthy donors was approved by Colorado State University Institutional Review Board and performed by a trained phlebotomist at the Colorado State University Health Center. Informed consent from all the donors was obtained.
Author contributions
Prof. Popat Ketul conceptualized the project and was the project leader; Dr Lerato Madike analysed the samples, undertook the data analysis and wrote the manuscript; Prof. Michael Pillay was responsible for student supervision, data curation and revisions.
Conflicts of interest
The authors report no conflicts of interest with respect to the research, authorship, and/or publication of this article.
Acknowledgements
The author(s) disclosed receipt of the following financial support for the research, authorship, and/or publication of this article: we acknowledge the Bioengineering and Chemistry Department at Colorado State University, United States of America, the Research Directorate at Vaal University of Technology, South Africa and the National Research Foundation of South Africa (Grant number 116322) for their financial support.
Notes and references
- K. Ghoshal and M. Bhattacharyya, Sci. World J., 2014, 2014, 781857 CrossRef PubMed.
- R. Grozovsky, S. Giannini, H. Falet and K. M. Hoffmeister, Blood, 2015, 126, 1877–1884 CrossRef CAS PubMed.
- S.-H. Yun, E.-H. Sim, R.-Y. Goh, J.-I. Park and J.-Y. Han, BioMed Res. Int., 2016, 9060143 Search PubMed.
- L. K. Jennings, Thromb. Haemostasis, 2009, 102, 248–257 CrossRef CAS PubMed.
- M. R. Thomas and R. F. Storey, Thromb. Haemostasis, 2015, 114, 449–458 CrossRef PubMed.
- J. Vane and R. Botting, Thromb. Res., 2003, 110, 255–258 CrossRef CAS PubMed.
- G. Davì and C. Patrono, N. Engl. J. Med., 2007, 357, 2482–2494 CrossRef PubMed.
- Z. S. Kaplan and S. P. Jackson, ASH Education Program Book, 2011, vol. 2011, pp. 51–61 Search PubMed.
- G. A. Roth, C. Johnson, A. Abajobir, F. Abd-Allah, S. F. Abera, G. Abyu, M. Ahmed, B. Aksut, T. Alam and K. Alam, J. Am. Coll. Cardiol., 2017, 70, 1–25 CrossRef PubMed.
- World Health Organisation, World Health Organization Fact sheet, 2017 Search PubMed.
- G. L. Allison, G. M. Lowe and K. Rahman, J. Nutr., 2006, 136, 789S–792S CrossRef CAS PubMed.
- K. Rahman, Ageing Res. Rev., 2003, 2, 39–56 CrossRef PubMed.
- S. R. Steinhubl, in Platelets, Elsevier, 2007, pp. 509–518 Search PubMed.
- L. Pang, M. J. Weiss and M. Poncz, J. Clin. Invest., 2005, 115, 3332–3338 CrossRef CAS PubMed.
- I. Jagroop, A. Kakafika and D. Mikhailidis, Curr. Pharm. Des., 2007, 13, 1669 CrossRef CAS PubMed.
- Z. Lorigooini, S. A. Ayatollahi, S. Amidi and F. Kobarfard, Iran. J. Pharm. Res., 2015, 14, 1225–1231 CAS.
- M. M. Bednar and C. E. Gross, Stroke, 1999, 30, 887–893 CrossRef CAS PubMed.
- M. S. Sabatine, Cleveland Clin. Q., 2009, 76, S8–S15 Search PubMed.
- A. Schäfer, J. Bauersachs and M. Eigenthaler, Vasc. Dis. Prev., 2009, 6, 75–84 CrossRef.
- L. Badimon and G. Vilahur, Rev. Esp. Cardiol., 2008, 61, 501–513 CrossRef.
- M. Cattaneo and G. M. Podda, Intern. Emerg. Med., 2010, 5, 385–391 CrossRef PubMed.
- P. Gresele and G. Agnelli, Trends Pharmacol. Sci., 2002, 23, 25–32 CrossRef CAS PubMed.
- I. Jagroop, Purinergic Signalling, 2013, 10, 233–239 CrossRef PubMed.
- E. Levetin and K. McMahon, Plants and Society, WCB/McGraw-Hill, Boston, 1999 Search PubMed.
- M. Kazemipoor, C. Wan Mohamed Radzi, G. A. Cordell and I. Yaze, Int. J. Chem. Eng. Appl., 2012, 3, 288–292 CAS.
- H. Nasri, J. Renal Inj. Prev., 2013, 2, 27 Search PubMed.
- A. Karimi, M. Majlesi and M. Rafieian-Kopaei, J. Nephropharmacol., 2015, 4, 27–30 CAS.
- J. Zhang, I. J. Onakpoya, P. Posadzki and M. Eddouks, J. Evidence-Based Complementary Altern. Med., 2015, 2015, 316706 Search PubMed.
- A. Dyson, Discovering indigenous healing plants of the herb and fragrance gardens at kirstenbosch national botanical garden, National Botanical Institute, South Africa, 1998 Search PubMed.
- C. G. Vosa, Caryologia, 2000, 53, 83–112 CrossRef.
- B.-E. Van Wyk, B. v. Oudtshoorn and N. Gericke, Medicinal Plants of South Africa, Briza Publications, Hatfield, Pretoria, 1997 Search PubMed.
- B.-E. Van Wyk and N. Gericke, People's plants: A guide to useful plants of Southern Africa, Briza Publications, Hatfield, Pretoria, 2000 Search PubMed.
- S. George, S. V. Bhalerao, E. A. Lidstone, I. S. Ahmad, A. Abbasi, B. T. Cunningham and K. L. Watkin, BMC Complementary Altern. Med., 2010, 10, 52 CrossRef PubMed.
- L. Bungu, M. van de Venter and C. Frost, Afr. J. Biotechnol., 2008, 7, 681–688 Search PubMed.
- N. L. A. Kee, N. Mnonopi, H. Davids, R. J. Naudé and C. L. Frost, Afr. J. Biotechnol., 2008, 7, 217–223 Search PubMed.
- A. C. Duncan, A. K. Jäger and J. van Staden, J. Ethnopharmacol., 1999, 68, 63–70 CrossRef CAS PubMed.
- I. Mackraj, S. Ramesar, M. Singh, T. Govender, H. Baijnath, R. Singh and P. Gathiram, J. Ethnopharmacol., 2008, 117, 263–269 CrossRef PubMed.
- I. A. Raji, P. Mugabo and K. Obikeze, J. Ethnopharmacol., 2012, 140, 98–106 CrossRef PubMed.
- C. Davison, R. Levendal and C. Frost, J. Med. Plants Res., 2012, 6, 4815–4824 Search PubMed.
- L. N. Madike, M. Pillay and K. C. Popat, J. Bioact. Compat. Polym., 2020, 35, 102–116 CrossRef CAS.
- L. N. Madike, S. Takaidza, C. C. SSemakalu and M. Pillay, S. Afr. J. Sci., 2019, 115, 35–40 Search PubMed.
- S. T. Chang, J. H. Wu, S. Y. Wang, P. L. Kang, N. S. Yang and L. F. Shyur, J. Agric. Food Chem., 2001, 49, 3420–3424 CrossRef CAS PubMed.
- S. McDonald, P. D. Prenzler, M. A. Antolovich and K. Robards, Food Chem., 2001, 73, 73–84 CrossRef CAS.
- S. Mohammed and F. A. Manan, J. Chem. Pharm. Res., 2015, 7, 132–135 CAS.
- N. Tamilselvi, P. Krishnamoorthy, R. Dhamotharan, P. Arumugam and E. Sagadevan, J. Chem. Pharm. Res., 2012, 4, 3259–3262 CAS.
- F. Kallel, D. Driss, F. Chaari, L. Belghith, F. Bouaziz, R. Ghorbel and S. E. Chaabouni, Ind. Crops Prod., 2014, 62, 34–41 CrossRef CAS.
- M. Blois, Nature, 1958, 26, 1199–1200 CrossRef.
- R. Re, N. Pellegrini, A. Proteggente, A. Pannala, M. Yang and C. Rice-Evans, Free Radicals Biol. Med., 1999, 26, 1231–1237 CrossRef CAS PubMed.
- B. S. Smith, S. Yoriya, L. Grissom, C. A. Grimes and K. C. Popat, J. Biomed. Mater. Res., Part A, 2010, 95, 350–360 CrossRef PubMed.
- B. S. Smith and K. C. Popat, J. Biomed. Nanotechnol., 2012, 8, 642–658 CrossRef CAS PubMed.
- V. Leszczak, B. S. Smith and K. C. Popat, J. Biomater. Sci., Polym. Ed., 2013, 24, 1529–1548 CrossRef CAS PubMed.
- L. Y. Madruga, K. C. Popat, R. D. C. Balaban and M. J. Kipper, Carbohydr. Polym., 2021, 273, 118541 CrossRef CAS.
- Z Montgomerie and K. C. Popat, Mater.
Sci. Eng. Carbon, 2021, 119, 111503 CrossRef CAS PubMed.
- L. N. Madike, S. Takaidza, C. C. Ssemakalu and M. Pillay, S. Afr. J. Bot., 2020, 130, 185–197 CrossRef CAS.
- T. B. Wigmosta, K. C. Popat and M. J. Kipper, J. Biomed. Mater. Res., 2021, 109, 1173–1182 CrossRef CAS PubMed.
- R. M. Sabino, G. Mondini, M. J. Kipper, A. F. Martins and K. C. Popat, Carbohydr. Polym., 2021, 251, 117079 CrossRef CAS PubMed.
- R. M. Sabino, K. Kauk, S. Movafaghi, A. Kota and K. C. Popat, Nanomedicine, 2019, 21, 102046–102046 CrossRef CAS PubMed.
- V. B. Damodaran, V. Leszczak, K. A. Wold, S. M. Lantvit, K. C. Popat and M. M Reynolds, RSC Adv., 2013, 3, 24406–24414. RSC.
- L. N. Madike, S. Takaidza and M. Pillay, Int. J. Pharmacogn. Phytochem. Res., 2017, 9, 1300–1308 Search PubMed.
- S. Takaidza, F. Mtunzi and M. Pillay, J. Tradit. Chin. Med., 2018, 38, 272–279 CrossRef.
- J.-J. Macheix, A. Fleuriet and J. Billot, Fruits phenolics, CRC Press, Boca Raton, FL, 1990, pp. 509–516 Search PubMed.
- C Proestos, I. S. Boziaris, G. J. E. Nychas and M Komaitis, Food Chemistry, 2006, 95, 664–671 CrossRef CAS.
- J. Perret, J. Wilson and S. Haley, J. Agric. Food Chem., 2003, 51, 1566–1570 CrossRef PubMed.
- A. E. Hagerman, Y. Zhao and S. Johnson, Antinutrients and Phytochemicals in Food, 1997, vol. 662, pp. 209–222 Search PubMed.
- R. Amarowicz, Eur. J. Lipid. Sci. Technol., 2007, 109, 549–551 CrossRef CAS.
- D. W. Griffiths, Adv. Exp. Med. Biol., 1986, 199, 509–516 CrossRef CAS PubMed.
- H.K. Sandhar, B. Kumar, S. Prasher, P. Tiwari, M. Salhan and P. Sharma, Int. Pharm. Sci., 2011, 1, 25–41 CAS.
- E. Heim, A. Tagliaferro and D Bobilya, J. Nutr. Biochem., 2002, 13, 572–584 CrossRef.
- M. Baier and K.-J. Dietz, J. Exp. Bot., 2005, 56, 1449–1462 CrossRef CAS PubMed.
- J. Dai and R. J. Mumper, Molecules, 2010, 15, 7313–7352 CrossRef CAS PubMed.
- A. Kumaran and R. J. Karunakaran, Food Chem. Toxicol., 2006, 97, 109–114 CrossRef CAS.
- J. H. Chen and C. T. Ho, J. Agric. Food Chem., 1997, 45, 2374–2378 CrossRef CAS.
- L. Rubió, M. J. Motilva and P. Romero, Crit. Rev. Food Sci. Nutr., 2013, 53, 943–953 CrossRef PubMed.
- Y. Cai, Q. Luo, M. Sun and H. Corke, Life Sci., 2004, 74, 2157–2184 CrossRef CAS PubMed.
- L. Rao and A. Rao, Phytochemicals—Isolation, Characterisation and Role in Human Health, InTech, Rijeka, 2015, pp. 247–260 Search PubMed.
- O. S. Soyingbe, A. O. Ayedeji, A. K. Basson, M. Singh and A. R. Opoku, Afr. J. Microbiol. Res., 2013, 7, 1787–1793 CrossRef CAS.
- J. Lee, N. Koo and D. Min, Compr. Rev. Food Sci. Food Saf., 2004, 3, 21–33 CrossRef CAS PubMed.
- D. M. Kasote, M. V. Hegde and S. S. Katyare, BioFactors, 2013, 39, 392–406 CrossRef CAS PubMed.
- R. A. Jacobs, Nutr. Res., 1995, 15, 755–766 CrossRef.
- B. Kumawat, M. Gupta and S. Y. Tarachand, Asian J. Plant Sci., 2012, 2, 323–329 Search PubMed.
- J. N. Eloff, J. Ethnopharmacol., 1998, 60, 1–8 CrossRef CAS.
- P. Tiwari, B. Kumar, M. Kaur, G. Kaur and H. Kaur, Int. Pharm. Sci., 2011, 1, 98–106 Search PubMed.
- S. Gololo, P. Masoko, L. Masoko, V. Mbazima, L. Shai, V. Bagla, J. Eloff and L. Mampuru, J. Altern. Complementary Med., 2013, 2013, 1–6 Search PubMed.
- R. Franke and F. Jung, Ser. Biomech., 2012, 27, 51–58 Search PubMed.
- M. B. Gorbet and M. V. Sefton, The Biomaterials: Silver Jubilee Compendium, Elsevier, 2004, pp. 219–241 Search PubMed.
- H. I. Jaffer, J. C. Fredenburgh, J. Hirsh and J. I. Weitz, J. Thromb. Haemost., 2015, 13, 72–81 CrossRef PubMed.
- Y. Miyazaki, S. Nomura, T. Miyake, H. Kagawa, C. Kitada, T. Taniguchi, Y. Komiyama, Y. Fujimura, Y. Ikeda and S. Fukuhara, Blood, 1996, 88, 3456–3464 CrossRef CAS PubMed.
- G. Leoncini, M. G. Signorello, A. Piana, M. Carrubba and U. Armani, Thromb. Res., 1997, 86, 153–160 CrossRef CAS PubMed.
- M. Kuwahara, M. Sugimoto, S. Tsuji, H. Matsui, T. Mizuno, S. Miyata and A. Yoshioka, Atertio. Thromb. Vasc. Biol., 2002, 22, 329–334 CrossRef CAS PubMed.
- D. D. Wagner and P. C. Burger, Atertio. Thromb. Vasc. Biol., 2003, 23, 2131–2137 CrossRef CAS PubMed.
- R. Alder, S. Lookinland, J. A. Berry and M. Williams, J. Am. Assoc. Nurse. Pract., 2003, 15, 120–129 CrossRef PubMed.
- U. Terblanche, C. C. SSemakalu, F. Mtunzi and M. Pillay, Int. J. Pharmacogn. Phytochem., 2017, 9, 303–312 Search PubMed.
- J. W. Heemskerk, E. M. Bevers and T. Lindhout, Thromb. Haemostasis, 2002, 88, 186–193 CrossRef CAS.
- D. M. Monroe, M. Hoffman and H. R. Roberts, Atertio. Thromb. Vasc. Biol., 2002, 22, 1381–1389 CrossRef CAS PubMed.
- V. Henn, J. R. Slupsky, M. Gräfe, I. Anagnostopoulos, R. Förster, G. Müller-Berghaus and R. A. Kroczek, Nature, 1998, 391, 591–594 CrossRef CAS PubMed.
- M. Gawaz, H. Langer and A. E. May, J. Clin. Invest., 2005, 115, 3378–3384 CrossRef CAS PubMed.
- B. E. Nignpense, K. A. Chinkwo, C. L. Blanchard and A. B. Santhakumar, Int. J. Mol. Sci., 2019, 21, 146 CrossRef PubMed.
- K. Prodouz, B. Poindexter and J. Fratantoni, Thromb. Res., 1987, 46, 337–346 CrossRef CAS PubMed.
- T. L. Andersson and E. Vinge, Pharmacol. Toxicol., 1988, 62, 172–176 CrossRef CAS PubMed.
- C. E. Sing, Blood Clotting Inspired Polymer Physics, PhD thesis, Massachusetts Institute of Technology, 2012.
- M. D. Rand, J. B. Lock, C. Van’t Veer, D. P. Gaffney and K. G. Maan, Blood, 1996, 88, 3432–3445 CrossRef CAS PubMed.
- H. H. Cho, D.-H. Han, K. Matsumura, S. Tsutsumi and S.-H. Hyon, Biomaterials, 2008, 29, 884–893 CrossRef CAS PubMed.
- M. Crescente, G. Jessen, S. Momi, H.-D. Höltje, P. Gresele, C. Cerletti and G. De Gaetano, Thromb. Haemostasis, 2009, 102, 336–346 CrossRef CAS PubMed.
- H.-J. Cho, H.-J. Kang, Y.-J. Kim, D.-H. Lee, H.-W. Kwon, Y.-Y. Kim and H.-J. Park, Blood Coagulation Fibrinolysis, 2012, 23, 629–635 CrossRef CAS PubMed.
|
This journal is © The Royal Society of Chemistry 2021 |
Click here to see how this site uses Cookies. View our privacy policy here.