DOI:
10.1039/D1SC05404J
(Edge Article)
Chem. Sci., 2021,
12, 15727-15732
Dibenzocycloheptanones construction through a removable P-centered radical: synthesis of allocolchicine analogues†
Received
30th September 2021
, Accepted 8th November 2021
First published on 9th November 2021
Abstract
Dibenzocycloheptanones containing a tricyclic 6–7–6-system are present in numerous biologically active natural molecules. However, the simple and efficient preparation of derivatives containing a dibenzocycloheptanone scaffold remains difficult to date. Herein, we report a versatile strategy for the construction of these challenging seven-membered rings using a 7-endo-trig cyclization which is initiated by a phosphorus-centered radical. This approach provides a step-economical regime for the facile assembly of a wide range of phosphorylated dibenzocycloheptanones. Remarkably, we also have devised a traceless addition/exchange strategy for the preparation of dephosphorylated products at room temperature with excellent yields. Therefore, this protocol allows for the concise synthesis of biorelevant allocochicine derivatives.
Introduction
Seven-membered rings represent a class of structurally special compounds with a synthetically difficult scaffold.1 In particular, the 6–7–6 ring system has been found to be a privileged structure which is present in a number of marketed natural products and bioactive molecules, such as N-acetylcolchicinol methyl ether (NSC 51046), ZD6126 and other pharmaceutical compounds (Fig. 1, bottom).2,3 However, because of inherent transannular and entropic factors,4,5 the synthesis of functionalized 6–7–6 ring systems still represents an ongoing challenge compared to numerous efficient and modular procedures to access common six- or five-membered cyclic compounds through traditional substitution, condensation and coupling reactions.6
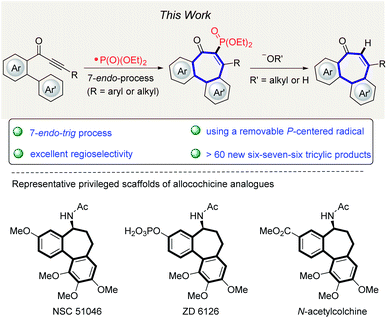 |
| Fig. 1 Dibenzocycloheptanones construction with reaction design, and selected examples of bioactive 6–7–6 tricyclic compounds. | |
During the last decades, various synthetic methods producing allocolchicine analogues have been developed. These examples include enyne ring-closing metathesis/Diels–Alder reaction7 or Diels–Alder reaction/aromatization approach,8 palladium-catalyzed intramolecular direct arylation,9 intramolecular Nicholas reaction,10 oxidative coupling,11 phenanthrol ring expansion,12 electrophilic iodocyclization13 and Suzuki–Miyaura coupling/Aldol condensation.14 Although those procedures definitely represent attractive strategies to access the challenging 6–7–6 ring system, the continuing investigation of direct and efficient approaches towards this core structure is still highly valuable. The construction of new chemical bonds by radical addition reactions represents a powerful tool for chemical transformations and has attracted considerable recent attention,15 as this approach usually features mild reaction conditions, predictable reaction outcome, a high functional group tolerance and the use of diverse radical precursors and initiation strategies. As 7-endo-cyclization reaction are considered to be favorable following the Baldwin rules and the extended ones from Alabugin,16 we questioned whether the efficiency noted in a removable radical formation could be translated into a practical formation strategy for 6–7–6 scaffolds. Although this direct radical annulation approach seems to be straightforward for the construction of the tricyclic core, key challenges remained to be addressed (Fig. 1, top).
First, the regioselectivity between 5-exo, 6-exo and 7-endo cyclization needs to be well controlled by adjusting the influence of substituents on the Ar′-moiety. Compared to five- or six-membered ring formation, the use of the 7-endo-trig approach is rare to construct seven-membered rings via radical cyclization.17 Second, similar to common directing groups in C–H activation, almost all radical sources will also leave behind undesired chemical “foot prints”,18 thereby requiring further synthetic derivatization and restricting the method to the desired skeleton of the products. Third, only few radical cascade reactions initiated by the addition of a phosphonyl radical to alkynes were reported, presumably due to slow kinetics of radical [(RO)2P(O)˙] addition step.19,20 Thus, the development of efficient P-radical tandem procedures and step-economical routes for the construction of various seven-membered rings is still desirable. Within our ongoing interest in radical addition reactions to the alkynones,20a,21 we herein report an unprecedented cascade cyclization of biaylynones, which can be performed with commercially available, inexpensive reagents in water-tolerant radical reaction conditions. Notable features of our strategy include (a) the first phosphonyl radical addition to internal alkynes resulting in a 7-endo-trig process, (b) the P-centered radical motif can be removed traceless under operationally-simple conditions, (c) excellent regioselectivity, and (d) ample substrate scope.
Results and discussion
Optimization of reaction condition
We initiated our studies by probing various reaction conditions for the envisioned cascade cyclization of 1-(3′,5′-dimethoxy-[1,1′-biphenyl]-2-yl)-3-phenylprop-2-yn-1-one (1a) as the model substrate (Tables 1 and S2 in the ESI†), using the inexpensive HPO(OEt)2 (2) as the phosphonyl radical source. Gratifyingly, the desired 6–7–6 tricyclic product 3 was isolated in 60% yield, when the reaction was performed in the presence of AgNO3/K2S2O8 in a 1,4-dioxane/H2O solvent system at 70 °C for 8 h. Different reaction media were explored, but fell short in efficiently delivering the desired products (2–8). Furthermore, a series of chemical oxidants was tested but showed not to be beneficial (entries 9–12). Either decreasing or increasing the reaction temperature failed to improve the yield of 3 (entry 13). Not surprisingly, substituents on the Ar'-ring have a decisive influence on the formation of the seven-membered ring. Therefore, a substitution effect was first examined under the optimized conditions (entry 11 in Table S2†). Indeed, the type and position of substituents on the arene moiety determined the efficacy of the 7-endo-trig cyclization process. We were pleased to find that 4-(tert-butyl)phenyl alkynone 1i gave the best result and the desired product 11 was thereby obtained in 67% yield (Fig. 2).
Table 1 Optimization of the cascade cyclization/phosphorylationa
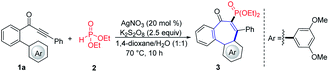
|
Entry |
Deviation from standard conditions |
Yield/% |
Standard conditions: 1a (0.2 mmol, 1.0 equiv), 2 (0.5 mmol, 2.5 equiv.), K2S2O8 (0.5 mmol, 2.5 equiv.), AgNO3 (20 mol%), 1,4-dioxane/H2O (1 : 1, 2 mL), under N2, 70 °C, 10 h. Yield of the isolated product.
|
1 |
No change |
60 |
2 |
MeCN |
41 |
3 |
1,4-Dioxane |
27 |
4 |
MeCN/H2O (1 : 1) |
Trace |
5 |
DMSO/H2O (1 : 1) |
Trace |
6 |
DMF/H2O (1 : 1) |
31 |
7 |
MeOH/H2O (1 : 1) |
Trace |
8 |
THF/H2O (1 : 1) |
40 |
9 |
Cu(OTf)2 and THBP in MeCN |
Trace |
10 |
Mn(OAc)3 (2.5 equiv.) in MeCN |
15 |
11 |
AgNO3 (2.0 equiv.) |
48 |
12 |
Na2S2O8 instead of K2S2O8 |
54 |
13 |
Reaction at 60 °C |
52 |
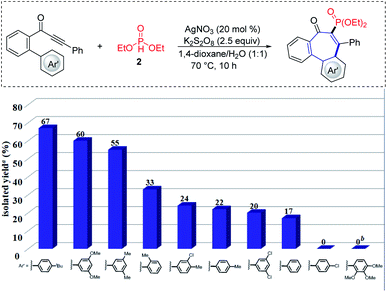 |
| Fig. 2 Substituent impact on 7-endo radical cyclization process.a aStandard conditions: 1a (0.2 mmol, 1.0 equiv.), 2 (0.5 mmol, 2.5 equiv.), K2S2O8 (0.5 mmol, 2.5 equiv.), AgNO3 (20 mol%), 1,4-dioxane/H2O (1 : 1, 2 mL), under N2, 70 °C, 10 h. Yield of the isolated products. b6-Exo product 5 formed in 60% yield. | |
Robustness
With the optimized conditions in hand, we became interested in investigating the substrate scope of this radical cyclization and we tested a diverse range of biarylyone substrates with different aromatic rings Ar and R (Scheme 1). The reaction tolerated a variety of H-phosphonates and substituents with diverse electronic properties in all positions of the arene (12–21), with the mass balance largely accounting for unreacted starting material. We were pleased to find that ortho- and meta-substituted arenes R underwent this transformation efficiently despite a possible steric repulsion (18–21), even for polysubstituted substrates. Furthermore, heterocyclic substrates bearing thiophene, pyridine and benzothiophene as well as naphthyl substituents were also tolerated in this transformation (22–25). After having demonstrated the broad applicability with respect to the arene R, substitutions on the phenone scaffold were examined as well. Substrates decorated with both electron-withdrawing and electron-donating groups on the aryl ring Ar did not significantly alter the reaction efficacy and the tricyclic compounds were efficiently obtained (26–38). As depicted, the 7-endo- approach was compatible with valuable sensitive functional groups, such as chloro-, bromo-, ether-, nitro-, cyano- and ester-functionalities. A heterocyclic substrate was also applicable in this transformation to afford the corresponding product 39 in good yield. Noteworthy, also alkyl alkynes were applicable and gave minor amounts of the corresponding products (40–41). The connectivity of product 31 was unambiguously confirmed by single-crystal X-ray analysis (Scheme 1).22
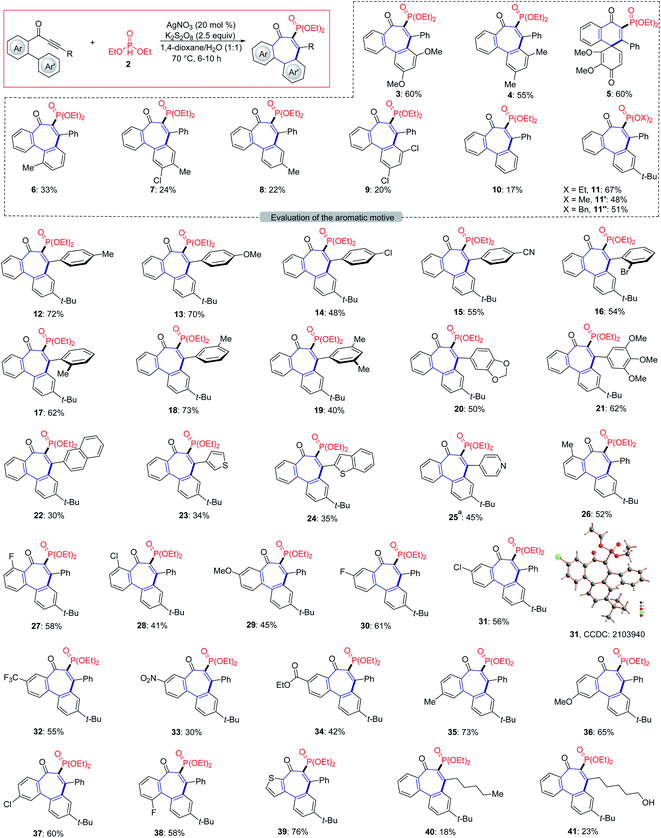 |
| Scheme 1 Scope of 7-endo-trig cyclization process. Reaction conditions from Table 1, entry 1, alkynone (1, 0.3 mmol), 6–10 h. aAfter being stirred at 70 °C for 10 h, the mixture was neutralized with NaHCO3 until pH 7–8 and extracted with ethyl acetate. | |
In order to increase the potential synthetic elaboration, we also explored an access to other classes of allocolchicine analogues. To our delight, we found that the incorporated phosphonyl motif could be easily removed in the presence of alkoxide or hydroxide bases. Next, the substrate scope of this traceless bond-constructing strategy was explored (Scheme 2). As expected, this mild anion addition/exchange approach was found to be generally applicable for the dephosphorylation (43–61). The structure of product 59 was unambiguously verified by X-ray crystallographic analysis.22b
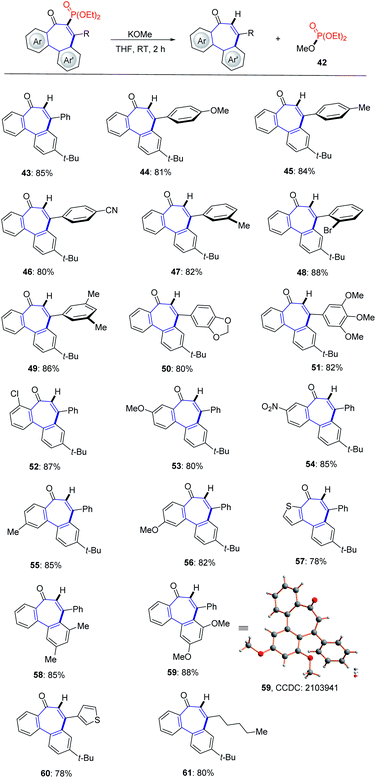 |
| Scheme 2 Scope of dephosphorylation process. | |
To further demonstrate the synthetic utility, the developed strategy was exploited for the preparation of bioactive NSC 51046 analogues (Scheme 3). NSC 51046 is a natural product which displays potent anticancer activity by inhibition of the tubulin polymerization.2c Due to the modularity of the our synthesis, this approach could be beneficial for preparing diverse NSC 51046 analogues from 3-phenyl-1-(3′,4,5′-trimethoxy-[1,1′-biphenyl]-2-yl)prop-2-yn-1-one 1n′ which could be easily prepared from 2-bromo-5-methoxybenzaldehyde via cross-coupling reaction. Under the standard reaction conditions, the phosphorylation product 62 was obtained in gram-scale. Subsequently, phosphonate 62 was converted to the dephosphorylation product enone 63 following our defunctionalization procedure. Reduction of alkene 63 led to the formation of intermediate 64, which provided the allocolchicine 66 after reduction followed by acetylation. Thus, this strategy opens a new avenue to a divergent synthesis of allocolchicine analogues with readily available starting materials and high efficacy.
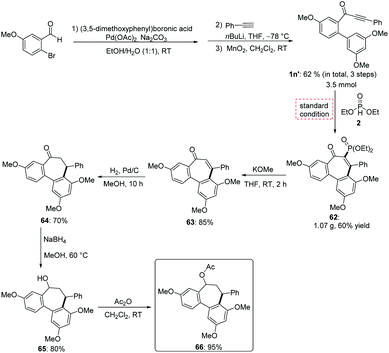 |
| Scheme 3 Gram-scale reaction and NSC 51046 analogue synthesis. | |
Considering that this conversion process includes a radical addition step, a control experiment was conducted thereafter. The key radical intermediate A could be trapped by 2,2,6,6-tetramethyl-1-piperidinyloxy (TEMPO) to form the TEMPO-adduct 67 (Fig. 3a). Based on these experimental result and related findings,23 a plausible mechanism involving a radical-type catalytic cycle is proposed as depicted in Fig. 3. First, the P-radical is generated from diethyl H-phosphonate (2) initiated by AgNO3. Selective radical addition of the P-radical to the α-position of the carbonyl in 1i affords the vinyl radical A, which undergoes 7-endo-trig cyclization resulting in intermediate B. Then, intermediate B undergoes further SET oxidation to form the product 11. In addition, we also found by pH measurement that after the reaction between 1i and 2 was performed under standard conditions, the reaction mixture became clearly acidic. Finally, enone 43 could be obtained after dephosphorylation involving an alkoxy anion addition/exchange process, generating 42 as a byproduct that can be detected by HRMS and 31P-NMR (see the ESI†).
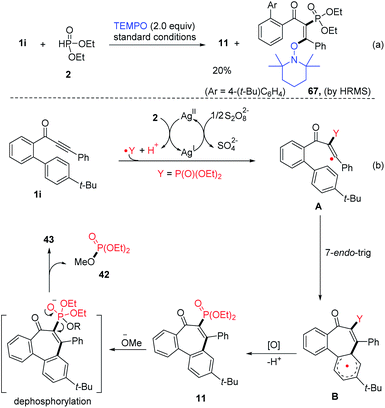 |
| Fig. 3 Mechanistic investigation on the radical annulation and dephosphorylation process (a) radical trapping experiment, (b) proposed mechanism. | |
Conclusions
In summary, we have developed an efficient approach for the construction of dibenzocycloheptanones through a cascade radical addition of biarylynones with diethylphosphite as the radical source. This versatile strategy showed high regioselectivity, ample scope and good functional group compatibility. It is worth mentioning that the introduced radical fragment of the phosphorylated products was easily removed in the presence of bases to give the corresponding allocolchicine analogues. The developed approach represents one of the rare 7-endo-trig radical cyclization processes and is a useful method for the concise construction of a variety of novel and potentially useful drug-type, and natural product-like molecules bearing valuable 6–7–6-scaffolds.
Data availability
All experimental data, procedures for data analysis and pertinent data sets are provided in the ESI.†
Author contributions
Y. Z. and L. A. conceived the project. Y. Z., Z. C., C. M. W. Z., X. L. and M. X. performed the experiments, Y. Z., Z.C. and C. M. and J. S. analyzed and interpreted the experimental data. Y. Z. and J. S. drafted the paper. Y. Z. and L. A. supervised the project. All of the authors discussed the results and contributed to the preparation of the final manuscript.
Conflicts of interest
There are no conflicts to declare.
Acknowledgements
Generous support by the National Natural Science Foundation of China (No. 21702188) and by the DFG (Gottfried-Wilhelm-Leibniz award to LA) is gratefully acknowledged.
Notes and references
-
(a) I. Shiina, Chem. Rev., 2007, 107, 239–273 CrossRef CAS PubMed;
(b) T. P. Majhi, B. Achari and P. Chattopadhyay, Heterocycles, 2007, 71, 1011–1052 CrossRef CAS;
(c) M. Inoue, Chem. Rev., 2005, 105, 4379–4405 CrossRef CAS PubMed.
-
(a) T. Graening and H.-G. Schmalz, Angew. Chem., Int. Ed., 2004, 43, 3230–3256 CrossRef CAS PubMed;
(b) B. Pérez-Ramírez, M. J. Gorbunoff and S. N. Timasheff, Biochemistry, 1998, 37, 1646–1661 CrossRef PubMed;
(c) A. Brossi, J. Med. Chem., 1990, 33, 2311–2319 CrossRef CAS PubMed.
-
(a) F. Büttner, S. Bergemann, D. Guénard, R. Gust, G. Seitz and S. Thoret, Bioorg. Med. Chem., 2005, 13, 3497–3511 CrossRef PubMed;
(b) K. Nakagawa-Goto, M. K. Jung, E. Hamel, C.-C. Wu, K. F. Bastow, A. Brossi, S. Ohta and K.-H. Lee, Heterocycles, 2005, 65, 541–550 CrossRef CAS;
(c) Q. Shi, K. Chen, X. Chen, A. Brossi, P. Verdier-Pinard, E. Hamel, A. T. McPhail, A. Tropsha and K.-H. Lee, J. Org. Chem., 1998, 63, 4018–4025 CrossRef CAS.
-
(a) R. L. Reyes, T. Iwai and M. Sawamura, Chem. Rev., 2021, 121, 8926–8947 CrossRef CAS PubMed;
(b) L. Yet, Chem. Rev., 2000, 100, 2963–3007 CrossRef CAS PubMed.
- G. Illuminati and L. Mandolini, Acc. Chem. Res., 1981, 14, 95–102 CrossRef CAS.
-
(a) R. S. Doerksen, T. Hodík, G. Hu, N. O. Huynh, W. G. Shuler and M. J. Krische, Chem. Rev., 2021, 121, 4045–4083 CrossRef CAS PubMed;
(b) J.-R. Chen, X.-Q. Hu, L.-Q. Lu and W.-J. Xiao, Chem. Rev., 2015, 115, 5301–5365 CrossRef CAS PubMed.
- F.-D. Boyer and I. Hanna, Org. Lett., 2009, 9, 715–718 CrossRef PubMed.
- A. V. Vorogushin, A. V. Predeus, W. D. Wulff and H.-J. Hansen, J. Org. Chem., 2003, 68, 5826–5831 CrossRef CAS PubMed.
- M. Leblanc and K. Fagnou, Org. Lett., 2005, 7, 2849–2852 CrossRef CAS PubMed.
-
(a) S. Djurdjevic, F. Yang and J. R. Green, J. Org. Chem., 2010, 75, 8241–8251 CrossRef CAS PubMed;
(b) S. Djurdjevic and J. R. Green, Org. Lett., 2007, 9, 5505–5508 CrossRef CAS PubMed.
- G. Besong, K. Jarowicki, P. J. Kocienski, E. Sliwinski and F. T. Boyle, Org. Biomol. Chem., 2006, 4, 2193–2207 RSC.
- W. M. Seganish and P. DeShong, Org. Lett., 2006, 8, 3951–3954 CrossRef CAS PubMed.
- Y. Chen, C. Huang, X. Liu, E. Perl, Z. Chen, J. Namgung, G. Subramaniam, G. Zhang and W. H. Hersh, J. Org. Chem., 2014, 79, 3452–3464 CrossRef CAS PubMed.
- Y. L. Choi, C.-M. Yu, B. T. Kim and J.-N. Heo, J. Org. Chem., 2009, 74, 3948–3951 CrossRef CAS PubMed.
- For reviews on radical chemistry, see:
(a) H. Xiao, Z. Zhang, Y. Fang, L. Zhu and C. Li, Chem. Soc. Rev., 2021, 50, 6308–6319 RSC;
(b) H. Zhou, Z.-L. Li, Q.-S. Gu and X.-Y. Liu, ACS Catal., 2021, 11, 7978–7986 CrossRef CAS;
(c) M. Latrache and N. Hoffmann, Chem. Soc. Rev., 2021, 50, 7418–7435 RSC;
(d) D. Leifert and A. Studer, Angew. Chem., Int. Ed., 2020, 59, 74–108 CrossRef CAS PubMed;
(e) Q.-Q. Zhou, Y.-Q. Zou, L.-Q. Lu and W.-J. Xiao, Angew. Chem., Int. Ed., 2019, 58, 1586–1604 CrossRef CAS PubMed;
(f) Y. Wei, P. Hu, M. Zhang and W. Su, Chem. Rev., 2017, 117, 8864–8907 CrossRef CAS PubMed;
(g) A. Studer and D. P. Curran, Angew. Chem., Int. Ed., 2016, 55, 58–102 CrossRef CAS PubMed;
(h) M.-C. Belhomme, T. Besset, T. Poisson and X. Pannecoucke, Chem. - Eur. J., 2015, 21, 12836–12865 CrossRef CAS PubMed;
(i) E. Merino and C. Nevado, Chem. Soc. Rev., 2014, 114, 2587–2693 CrossRef PubMed;
(j) F. Dénès, M. Pichowicz, G. Povie and P. Renaud, Chem. Rev., 2014, 114, 2587–2693 CrossRef PubMed;
(k) U. Wille, Chem. Rev., 2013, 113, 813–853 CrossRef CAS PubMed;
(l) P. Chen and G. Liu, Synthesis, 2013, 2919–2939 CAS.
- K. Gilmore and I. V. Alabugin, Chem. Rev., 2011, 111, 6513–6556 CrossRef CAS PubMed.
-
(a) P. Xiong, H.-H. Xu, J. Song and H.-C. Xu, J. Am. Chem. Soc., 2018, 140, 2460–2464 CrossRef CAS PubMed;
(b) Y. Li and J.-H. Li, Org. Lett., 2018, 20, 5323–5326 CrossRef CAS PubMed.
- Y. Lu, D.-H. Wang, K. M. Engle and J.-Q. Yu, J. Am. Chem. Soc., 2010, 132, 5916–5921 CrossRef CAS PubMed.
- For reviews on phosphorous-centered radical reactions, see:
(a) X.-Q. Pan, J.-P. Zou, W.-B. Yi and W. Zhang, Tetrahedron, 2015, 71, 7481–7529 CrossRef CAS;
(b) D. Leca, L. Fensterbank, E. Lacôte and M. Malacria, Chem. Soc. Rev., 2005, 34, 858–865 RSC;
(c) W. G. Bentrude, Acc. Chem. Res., 1982, 15, 117–125 CrossRef CAS.
- For phosphonyl radical addition to alkynes, see:
(a) Y. Zhang, J. Zhang, B. Hu, M. Ji, S. Ye and G. Zhu, Org. Lett., 2018, 20, 2988–2992 CrossRef CAS PubMed;
(b) Z.-D. Liu, W.-Z. Bi, Y.-Y. Xia, H.-T. Wu and Y.-F. Zhao, Chem. Commun., 2015, 51, 3846–3849 RSC;
(c) L.-J. Wang, A.-Q. Wang, Y. Xia, X.-X. Wu, X.-Y. Liu and Y.-M. Liang, Chem. Commun., 2014, 50, 13998–14001 RSC;
(d) X. Mi, C. Wang, M. Huang, J. Zhang, Y. Wu and Y. Wu, Org. Lett., 2014, 16, 3356–3359 CrossRef CAS PubMed;
(e) X.-Q. Pan, J.-P. Zou, G.-L. Zhang and W. Zhang, Chem. Commun., 2010, 46, 1721–1723 RSC.
-
(a) Y. Zhang, C. Ma, J. Struwe, J. Feng, G. Zhu and L. Ackermann, Chem. Sci., 2021, 12, 10092–10096 RSC;
(b) Y. Zhang, D. Guo, S. Ye, Z. Liu and G. Zhu, Org. Lett., 2017, 19, 1302–1305 CrossRef CAS PubMed;
(c) W. Ma and L. Ackermann, Synthesis, 2014, 2297–2304 CAS.
-
(a) CCDC 2103940;†;
(b) CCDC 2103941.†.
-
(a) X. Tan, Z. Liu, H. Shen, P. Zhang, Z. Zhang and C. Li, J. Am. Chem. Soc., 2017, 139, 12430–12433 CrossRef CAS PubMed;
(b) Z.-Z. Zhou, X.-B. Yan, X.-Y. Liu and Y.-M. Liang, Org. Lett., 2014, 16, 5616–5619 CrossRef CAS PubMed.
Footnote |
† Electronic supplementary information (ESI) available: Experimental details and characterization of all new compounds and details for DFT calculations. CCDC 2103940 and 2103941. For ESI and crystallographic data in CIF or other electronic format see DOI: 10.1039/d1sc05404j |
|
This journal is © The Royal Society of Chemistry 2021 |
Click here to see how this site uses Cookies. View our privacy policy here.