DOI:
10.1039/D1RA07559D
(Paper)
RSC Adv., 2022,
12, 2399-2407
Synthesis of novel antibacterial and antifungal quinoxaline derivatives†
Received
13th October 2021
, Accepted 27th December 2021
First published on 18th January 2022
Abstract
A series of quinoxaline derivatives were designed, synthesized and evaluated as antimicrobial agents against plant pathogenic bacteria and fungi. Some of these compounds exhibited significant antibacterial and antifungal activities in vitro. Compound 5k displayed good antibacterial activity against Acidovorax citrulli (Ac). Compounds 5j and 5t exhibited the most potent anti-RS (Rhizoctonia solani) activity, with the corresponding EC50 values of 8.54 and 12.01 μg mL−1, respectively, which are superior to that of the commercial azoxystrobin (26.17 μg mL−1). Further, the scanning electron microscopy results proved that compound 5j had certain effects on the cell morphology of RS. Moreover, an in vivo bioassay also demonstrated that the anti-RS activity of compound 5j could effectively control rice sheath blight. These results indicate that quinoxaline derivatives could be promising agricultural bactericides and fungicides.
1. Introduction
A variety of diseases caused by plant pathogen infections seriously threaten the quality and yields of crops, and thereby also lead to a large number of economic losses in agricultural production.1,2 For example, rice sheath blight, bacterial fruit spot of melon and bacterial blight of rice, and so forth, are difficult to control in agricultural production.3–5 Rice sheath blight is one of the most widely distributed in rice and caused by RS infection. It mainly infects the leaf sheath and leaf of rice, and its symptom change from an early water damage disease spot to a moire shape. It is also one of the main reasons for the decline of rice yields and quality globally, and meanwhile has caused huge economic losses.6,7 The importance of rice is self-evident as one of the main food crops in the world.8 Bacterial fruit spot melon is caused by Ac, which could infect the leaf veins, extend along the leaf veins causing irregular spots of light brown to red on the leaves, and softening and rotting the fruits.5,9 At present, the main prevention and control methods are chemical drugs, such as bismerthiazol (BT), thiodiazole-copper (TC), azoxystrobin, etc.10 However, due to the ecological pollution caused by drug abuse and the emergence of drug resistance, many adverse effects have become increasingly prominent.11,12 Therefore, the discovery of new pesticides with low toxicity, high-efficiency and good environment compatibility is very critical to ensure agriculture production.
Quinoxaline derivatives, a class of N-containing heterocyclic compounds, which is an important chemical intermediate, have played an important role in the design and synthesis of new heterocyclic compounds,13 and are of great significance for drug discovery.14 Quinoxaline derivatives exhibit broad-spectrum bioactivities, such as antibacterial,15,16 antiviral,17,18 antitumor,19–21 anti-tuberculous,22 anticancer,23,24 and so on.25,26 Therefore, quinoxaline moieties widely exist in pesticides and medicines, and some commercialized pesticides used in agricultural production, including quintiofos, quizalofop-p-ethyl, chloramphenicol, olaquindox, and so forth (Fig. 1). Among them, quintiofos is an organophosphorus insecticide, developed by the Bayer company, that became a more widely used insecticide because of its low toxicity and replaced many highly toxic and harmful insecticides including parathion, methyl parathion, methamidophos, etc.27 Similarly, quizalofop-p-ethyl is also a low toxicity molecule, developed by the Nissan Chemical Company, and is widely used to control gramineous weeds.28 The above survey suggests that quinoxoline derivatives have huge application prospects in agrochemicals. However, few studies have been done on quinoxoline derivatives with strong antibacterial and antifungal activities.29 Therefore, the study of quinoxaline derivatives as antibacterial and antifungal agents has an important significance for the diversification of pesticide development.
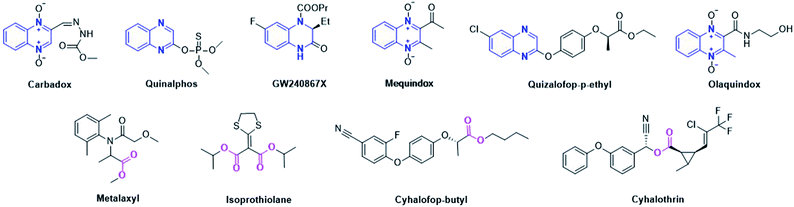 |
| Fig. 1 Structure of some commercial agents. | |
Based on these considerations, a class of quinoxaline derivatives was designed (Fig. 2), synthesized and evaluated for their anti-microbial activities. The bioassay results displayed that some of the target compounds exhibited obviously superior antifungal and antibacterial activities to those of the commercial agents bismerthiazol, thiodiazole copper and azoxystrobin. In addition, the mechanisms of action of these compounds were preliminarily studied, and an in vivo biological activity study of compound 5j against RS was performed.
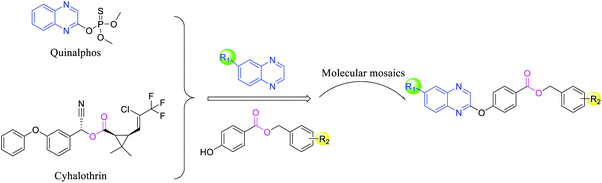 |
| Fig. 2 Design strategy of target compounds. | |
2. Experimental
2.1 Instruments and chemicals
The melting points were measured on an XT-4 binocular microscope (Beijing Tech. Instrument Co., China) and left uncorrected. 1H NMR, 13C NMR, and 19F NMR spectra were completed in a chloroform-d solution on an ASCEND 400 NMR (Swiss Bruker). High-resolution mass spectrometry (HRMS) was conducted using a Thermo Scientific Q Exactive (Thermo Scientific, Missouri, USA). All of the reactions were monitored by TLC. All the chemical materials and reagents involved in the reactions were purchased from commercial suppliers and the reagents were chemically or analytically pure. The plant pathogenic fungi were provided by the Environment and Plant Protection Institute, Chinese Academy of Tropical Agricultural Science.
2.2 General procedures for preparing intermediates 1–4 and the target compounds 5a–5t
Compounds 5a–5t were synthesized according to the designed route shown in Scheme 1. Intermediates 1, 2, 3, and 4 were synthesized by literature methods.30,31 The intermediate 4 and K2CO3 were heated in acetonitrile for 30 min, then 2 was dissolved in acetonitrile and added dropwise, to give compounds 5a–5t after the complete reaction. The reaction was monitored using TLC. The target compounds 5a–5t were purified by column chromatography (V/V, petroleum ether
:
ethyl acetate = 20
:
1 to 12
:
1).
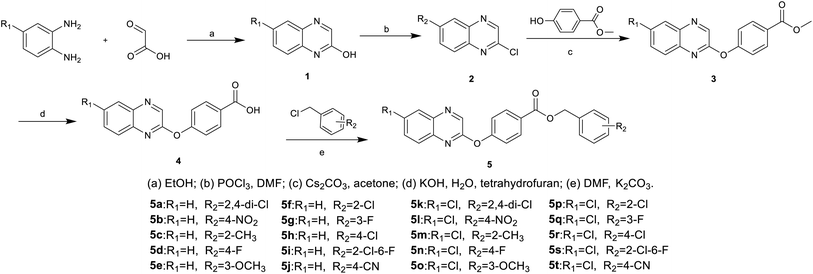 |
| Scheme 1 Synthetic route of the target compounds 5a–5t. | |
2.3. Biological activities tests
2.3.1 In vitro antibacterial activity tests. The in vitro antibacterial activities of target compounds 5a–5t against the five plant pathogenic bacteria Xanthomonas oryzae pv. Oryzae (Xoo), Xanthomonas campestris pv. Mangiferae indicae (Xcm), Pectobacterium carotovorum subsp. Brasiliense (Pcb), Ralstonia salanocearum (Rs) and Acidovorax citrulli (Ac) were evaluated by a slightly modified 96-well plate method.32 Bismerthiazol (BT) and thiodiazole-copper (TC) were used as positive control agents. The details are listed in the ESI.†
2.3.2 In vitro antifungal tests. The in vitro antifungal effects of the target compounds against Alternaria brassicae (AB), Fusarium fujikuroi (FF), Fusarium oxysporum f. sp. cucumerinum (FO), Colletotrichum truncatum (CT), Phytophthora capsici (PC), Colletotrichum gloeosporioides (CG), Rhizoctonia solani (RS), Fusarium graminearum (FG), Phytophthora sojae (PS), Phytophthora palmivora (PP), Botrytis cinerea (BC), and Phytophthora litchii (PL) were evaluated by a mycelial growth rate method.33–35 The experimental details for the twelve fungi are presented in the ESI.†
2.3.3 In vivo antifungal tests. The in vivo biological activity of compound 5j against rice sheath blight was carried out by a reported detached leaf assay and greenhouse experiment36–38 with slight modifications, and the variety of rice was Fengyouxiangzhan.
2.3.4 Sclerotia germination inhibition tests. The sclerotia of RS were obtained after 21 days of culture in PDA (potato dextrose agar) medium, and medium with concentrations of 0, 10, 50 and 100 μg mL−1 containing 5j were prepared. Fifteen sclerotia were placed in a Petri dish with three replicates for each concentration. Azoxystrobin was used as a positive control. All the treatments were incubated at 25 °C for 24 h, and the inhibition rate was calculated.39
2.4 Scanning electron microscopy (SEM)
Scanning electron microscopy (SEM) experiments were performed according to a reported method.40–43
3 Results and discussion
3.1 Chemistry
Compounds 5a–5t were synthesized according to the design route in Scheme 1. All the target compounds were characterized by 1H NMR, 13C NMR, 19F NMR and HRMS, and the specific data are presented in the ESI.†
3.2 Biological activities test
3.2.1 In vitro antibacterial test. As can be seen in Table S1,† some compounds exhibited good inhibitory activities against the pathogenic bacteria at concentrations of 200 μg mL−1. Compound 5k (86.28%) displayed a good inhibitory effect against Ac, and its inhibitory rate was significantly better than those of TC (57.67%) and BT (41.07%). Meanwhile, compound 5o (72.64%) showed a moderate inhibitory activity on Pcb, which was better than those of TC and BT (51.09 and 49.61%, respectively). Moreover, the inhibition rates of 5o and 5p to Xoo were 72.84 and 76.15%, respectively, higher than those of TC (60.11%) and BT (52.13%). In addition, the antibacterial activity of 5b (71.33%) and 5c (70.45%) against Rs in vitro was similar to that of TC (66.01%) and slightly better than that of BT (42.33%), and the inhibition rates of 5p (75.17%) and 5q (74.23%) against Xcm were higher than those of TC (67.82%) and BT (46.82%).Further, EC50 tests were carried out and the toxicity curve and EC50 values were calculated (Table 1). The results display that compound 5k had a good anti-Ac ability with an EC50 value of 35.18 μg mL−1, which was significantly better than those of TC (198.51 μg mL−1) and BT (295.15 μg mL−1). Meanwhile, the EC50 value of compound 5p for Xoo was 72.21 μg mL−1, superior to those of TC (182.85 μg mL−1) and BT (230.23 μg mL−1). Furthermore, the EC50 value for compound 5p (86.88 μg mL−1) was analogous to that of TC (80.14 μg mL−1) against Xcm, which was better than that of BT (232.82 μg mL−1). According to Table 1, all the compounds tested had certain antibacterial effects, and 5k had the best antibacterial activity against Ac.
Table 1 Virulence curves and EC50 values of the target compounds against five kinds of bacteriaa,b
Pathogen |
Chemical |
Toxic regression equation |
r |
EC50 (μg mL−1) |
The experiments were repeated three times. Commercial bactericides bismerthiazol (BT) and thiodiazole-copper (TC) were used as positive control agents. |
Ac |
5k |
y = 1.1818x + 3.1726 |
0.9570 |
35.18 |
TC |
y = 1.4286x + 1.7174 |
0.9724 |
198.51 |
BT |
y = 1.6492x + 0.9264 |
0.9935 |
295.15 |
Pcb |
5o |
y = 1.6514x + 1.6444 |
0.9801 |
107.64 |
TC |
y = 1.8284x + 0.7780 |
0.9899 |
203.76 |
BT |
y = 1.3964x + 1.7517 |
0.9966 |
211.93 |
Xoo |
5o |
y = 1.5922x+1.7321 |
0.9749 |
112.83 |
5p |
y = 1.1848x + 2.8051 |
0.9698 |
72.211 |
TC |
y = 1.4216x + 1.7842 |
0.9675 |
182.85 |
BT |
y = 1.8337x + 0.6685 |
0.9853 |
230.23 |
Rs |
5b |
y = 0.7773x + 3.6717 |
0.9804 |
51.15 |
5c |
y = 1.3088x + 2.3014 |
0.9551 |
115.31 |
TC |
y = 1.0061x + 3.1290 |
0.9975 |
72.38 |
BT |
y = 1.7183x + 0.8514 |
0.9966 |
259.63 |
Xcm |
5p |
y = 1.2743x + 2.5292 |
0.9618 |
86.88 |
5q |
y = 1.5969x + 1.7239 |
0.9654 |
112.59 |
TC |
y = 1.0849x + 2.9345 |
0.9976 |
80.14 |
BT |
y = 1.7293x + 0.9067 |
0.9859 |
232.82 |
Some compounds had a good inhibitory activity against Xoo. When R1 was the electron-donating group H, the inhibition rates of compounds 5a, 5e, 5g and 5i against Xoo were 62.53, 66.81, 65.49 and 61.35%, respectively, and 5p (R2 = 2-Cl) > 5o (R2 = 3-OCH3) > 5n (R2 = 2-F) > 5l (R2 = 4-NO2), which indicates that R1 and R2 are electron-donating groups, which were beneficial to improve the inhibitory activity of compounds against Xoo. When the R1 group was the electron withdrawing group Cl, the inhibition rates of compounds 5l, 5n, 5o and 5p against Xoo were 67.25, 67.28, 72.84 and 76.15%, respectively, with 5p (R2 = 2-Cl) > 5o (R2 = 3-OCH3) > 5n (R2 = 2-F) > 5l (R2 = 4-NO2), and it is speculated that when the R2 group was an electron withdrawing group, the weaker the electron withdrawing ability, the stronger the compound's ability to inhibit Xoo. When R2 = 3-OCH3, the activity against Xoo with R1 an electron withdrawing group was better than that with R1 being an electron donating group, for example, 5o (R1 = Cl) > 5e (R1 = H).
In terms of the inhibitory activity of the target compounds against Rs, the inhibition rate of R1 = H was generally better than that of R2 = Cl; for example, 5b (R1 = H, R2 = 4-NO2) > 5l (R1 = Cl, R2 = 4-NO2), 5c (R1 = H, R2 = 2-CH3) > 5m (R1 = Cl, R2 = 2- CH3), 5e (R1 = H, R2 = 3-OCH3) > 5o (R1 = Cl, R2 = 3-OCH3), indicating that an R1 group electron donor group conducive for the target compound to inhibit the growth of Rs. When R1 = H, 5b (R2 = 4-NO2) has the best inhibitory activity against Rs; when R1 = Cl, 5l (R2 = 4-NO2) has a good inhibitory activity against Rs, 5b > 5l. It is speculated that when the R1 group is an electron donor group and R2 is an electron strong group, the inhibitory activity of the target compounds against Rs could be improved.
3.2.2 In vitro antifungal test. The results shown in Table S2† indicate that some compounds exhibited good antifungal activities. Among them, compounds 5j and 5t showed a good control effect on RS, with inhibition rates of 89.56 and 95.17%, respectively, which were significantly better than that of azoxystrobin (76.43%). Meanwhile, the inhibitory rates of compound 5k on FF, PS and PP were 89.38, 89.92 and 89.38%, respectively, which were better than those of commercial azoxystrobin (51.34, 55.70 and 77.19%, respectively). Furthermore, the toxicological curves and EC50 values shown in Table 2 and Fig. 4 indicate that compounds 5j and 5t had an exceptionally significant antifungal activity against RS with EC50 values of 8.54 and 12.01 μg mL−1, respectively, which were better than that of azoxystrobin (26.17 μg mL−1). The structure of compound 5j shown in Fig. 3.
Table 2 Virulence curves and EC50 values of the target compounds against four fungal pathogensa
Pathogen |
Chemical |
Toxic regression equation |
r |
EC50 (μg mL−1) |
The experiments were repeated three times. |
FF |
5k |
y = 2.5936x + 0.7048 |
0.9747 |
45.29 |
Azoxystrobin |
y = 1.0678x + 3.2400 |
0.9828 |
44.48 |
PS |
5k |
y = 2.20480 + 1.2841 |
0.9505 |
48.45 |
Azoxystrobin |
y = 1.6159x + 2.1092 |
0.9781 |
61.51 |
PP |
5k |
y = 2.3750x + 1.0053 |
0.9517 |
48.08 |
Azoxystrobin |
y = 1.3961x + 2.9045 |
0.9978 |
31.69 |
RS |
5j |
y = 0.7190x + 4.3299 |
0.9989 |
8.54 |
5t |
y = 1.8452x + 3.0077 |
0.9850 |
12.01 |
Azoxystrobin |
y = 1.2996x + 3.1574 |
0.9885 |
26.17 |
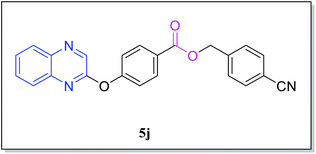 |
| Fig. 3 Structures of compound 5j. | |
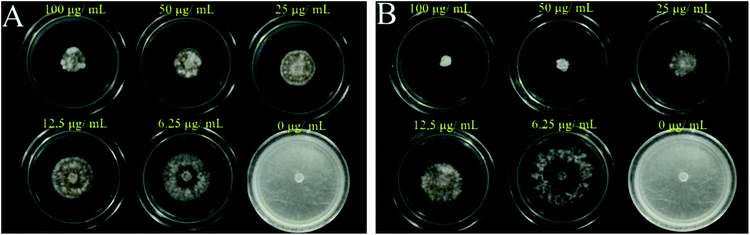 |
| Fig. 4 In vitro anti-RS activity of 5j(A) and 5t(B). | |
On the basis of in vitro antifungal bioassay shown in Table S2†, the preliminary analysis of structure activity relationships could be generalized, as below. Obviously, comparing compounds 5a–5j with 5k–5t, the in vitro inhibitory activity against FF, RS of most compounds at 100 μg mL−1 with R1 = Cl was better than of R1 = H. For example, 5k (89.38%, R1 = Cl) > 5a (22.12%, R1 = H), 5t (95.17%, R1 = Cl) > 5j (89.56%, R1 = H).
3.2.3 In vivo antifungal test. In vitro antifungal activity suggested that compound 5j had remarkable effects against RS. Further, compound 5j was evaluated for its activity in vivo, and the results were in Table 3 and Fig. 5. Compound 5j displayed a good protective activity in vivo on detached leaves at 5 days after inoculating with a control efficacy of 66.17% at 100 μg mL−1 and 93.3% at 200 μg mL−1, superior to those of carbendazim at 100 and 200 μg mL−1 (41.67 and 55.43%, respectively). The control efficacy reached 100% when the concentration was increased to 500 μg mL−1. The control efficacy of 5j (93.30%) at 200 μg mL−1 was equivalent to that of azoxystrobin (94.28%) at 100 μg mL−1.
Table 3 Anti-RS protective activity data of compound 5j on detached rice leavesa
Chemical |
Treatment (μg mL−1) |
Lesion length (cm) |
Control efficacy (%) |
Values are the average of 15 replicates. |
5j |
100 |
2.07 ± 0.31 |
66.17 |
200 |
0.41 ± 0.16 |
93.30 |
500 |
0.00 ± 0.00 |
100.00 |
Azoxystrobin |
100 |
0.35 ± 0.12 |
94.28 |
200 |
0.18 ± 0.11 |
97.06 |
Carbendazim |
100 |
3.57 ± 0.19 |
41.67 |
200 |
2.85 ± 0.22 |
53.43 |
Negative control |
— |
6.12 ± 0.58 |
— |
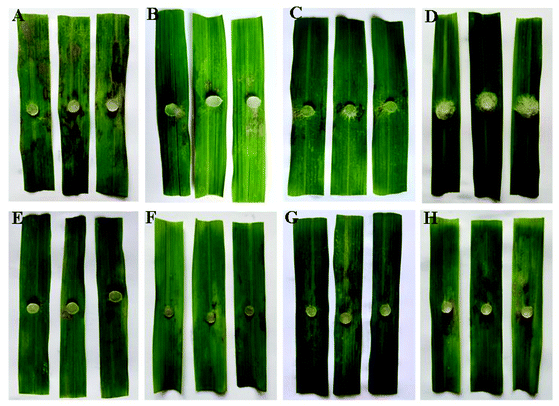 |
| Fig. 5 Anti-RS protection efficacy photographs of compound 5j on detached rice leaves. (A) Negative control; (B) 5j at 100 μg mL−1; (C) 5j at 200 μg mL−1; (D) 5j at 500 μg mL−1; (E) azoxystrobin at 100 μg mL−1; (F) azoxystrobin at 200 μg mL−1; (G) carbendazim at 100 μg mL−1; (H) carbendazim at 200 μg mL−1. | |
Moreover, for the anti-RS results of compound 5j in the greenhouse experiment shown in Table 4 and Fig. 6, treatment with compound 5j at 100 μg mL−1 proved that the protective efficacy was 85.99% in the greenhouse experiment, similar to that of azoxystrobin (84.75%) at 200 μg mL−1. In contrast, the curative activity of compound 5j at the concentrations of 200 and 100 μg mL−1 were 68.43 and 49.72%, respectively, which were better than those of azoxystrobin (65.64 and 46.50%, respectively).
Table 4 In vivo control efficacy of compound 5j against rice sheath blight under greenhouse conditionsa
Chemical |
Treatment (μg mL−1) |
Protective activity |
Curative activity |
Lesion length (cm) |
Control efficacy (%) |
Lesion length (cm) |
Control efficacy (%) |
Values are the average of 15 replicates. |
5j |
200 |
1.13 ± 0.28 |
85.99 |
2.26 ± 0.11 |
68.43 |
100 |
2.33 ± 0.17 |
71.12 |
3.60 ± 0.43 |
49.72 |
Azoxystrobin |
200 |
0.80 ± 0.31 |
90.08 |
2.46 ± 0.55 |
65.64 |
100 |
1.23 ± 0.59 |
84.75 |
3.83 ± 0.27 |
46.50 |
Negative control |
|
8.07 ± 0.13 |
— |
7.16 ± 0.25 |
— |
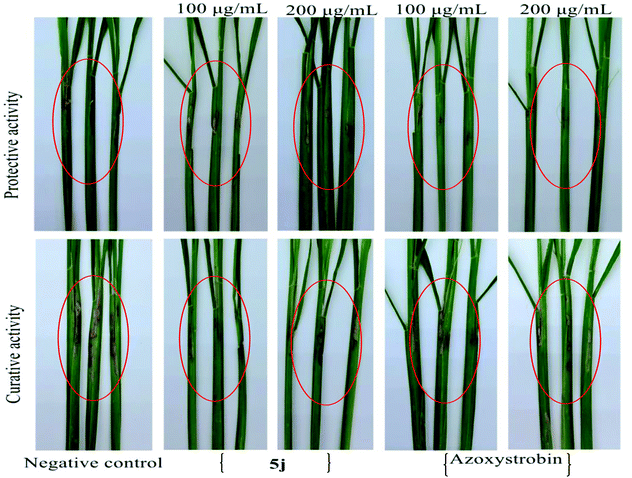 |
| Fig. 6 Anti-RS efficacy photographs of compound 5j in greenhouse experiment. | |
3.2.4 Sclerotia germination inhibition of 5j. As shown in Fig. 7, compound 5j had a certain inhibitory effect on the germination of RS sclerotia in a dose-dependent manner. At a concentration of 100 μg mL−1, the inhibitory rate of compound 5j could reach 40.00%, which was better than that of azoxystrobin (28.89%). As can be seen, a high concentration of azoxystrobin has a good inhibitory effect on the outward growth of mycelia, the mycelia growth circle was small, and the growth trend of mycelia was similar to that of the negative control. At high and low concentrations of 5j, the edge of the sclerotia hyphae growth is close to the circle, but the hyphae grow totally differently to those with the negative control, with the negative control sclerotium near the hyphae appearing more transparent, and with a medium growth of mycelial attachment, whereas the 5j processed sclerotium hyphae were whiter in color, and the hyphae gathered to grow.
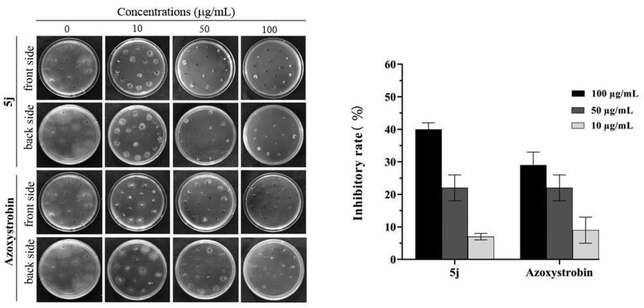 |
| Fig. 7 Inhibitory activity of compound 5j on the germination of the sclerotia of RS. | |
3.3 Scanning electron microscopy (SEM)
The effect of compound 5k on the morphology of Ac cells was observed by SEM as illustrated in Fig. 8. The image shows that the degree of cell membrane damage and the concentration of compound 5k in a dose-dependent manner. To be specific, the cell membrane changed from a simple deformation to depression and rupture when the concentration ranged from 100 to 200 μg mL−1. In contrast, the control group was intact and full. Through the analysis of the SEM results, the antibacterial mechanism of compound 5k for Ac might be that it destroyed the cell membrane and led to cell death, thus achieving the antibacterial effect.
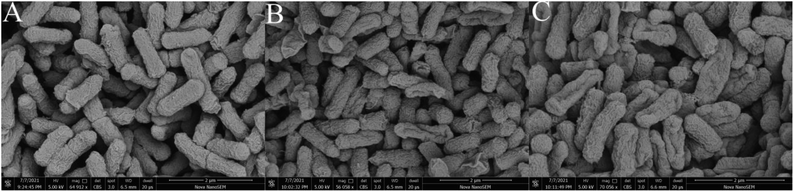 |
| Fig. 8 SEM images for Ac. after incubation in different concentrations of compound 5k. (A) 0 μg mL−1; (B) 100 μg mL−1 and (C) 200 μgmL−1. Scale bar for is 2 μm. | |
The morphological changes of RS hyphae were observed by SEM, as shown in Fig. 9. The untreated mycelial surface was smooth and presents a full cylindrical shape, while the mycelial growth was abnormal after treatment with compound 5j for 48 h. A large number of folds were generated on the mycelial surface, with many short folds that shrink inward, but a cylindrical shape could still be seen when the concentration was 50 μg mL−1. There were a few long fold marks on the smooth mycelial surface when the concentration increased to 100 μg mL−1, while the hyphae were of a flake shape. We speculate that the initial antifungal mechanism of 5j on RS was that with the increase of drug concentration, the mycelial epidermis gradually concave inward, squeezing the internal material of mycelium and inactivating it, leading to the mycelium changes from a full columnar to sheet, so as to inhibit the growth of RS.
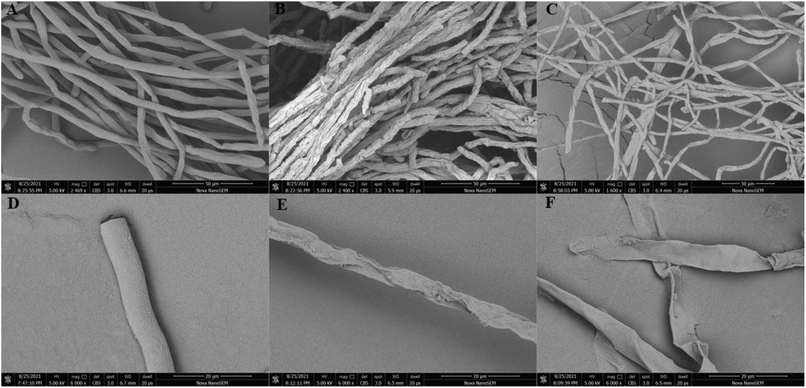 |
| Fig. 9 Scanning electron micrographs of the hyphae of RS grown on PDA plates with 5j at 28 °C. (A and D) control (DMSO); (B and E) 5j (50 μg mL−1) and (C and F) 5j (100 μg mL−1). | |
4. Conclusions
In summary, a series of quinoxaline derivatives was designed, synthesized, and evaluated for their biological activities (five bacteria and twelve fungi). The preliminary experiment showed that some of the designed compounds were identified with an excellent antimicrobial competence. Antibacterial assays discovered that compound 5k had better activities than those of BT and TC against Ac. It is worth noting that 5j showed a highlighted fungicidal activity against RS, and an in vivo assay further proved that it could effectively control rice sheath blight. Moreover, the SEM result confirmed that this series of compounds had the competence to change and destroy the bacteria and fungi cell morphologies. By and large, all the findings suggest that quinoxaline derivatives are of research value for agricultural bactericides and fungicides, which could be used to develop potential agrochemicals in the future.
Conflicts of interest
The authors declare no competing financial interest.
Acknowledgements
The authors gratefully acknowledge the National Nature Science Foundation of China (No. 31701821), the Science Foundation of Guizhou Province (No. 20192452), the Natural Science research project of Guizhou Education Department (No. 2018009), Frontiers Science Centre for Asymmetric Synthesis and Medicinal Molecules, Department of Education, Guizhou Province (No. 2020004), and Program of Introducing Talents of Discipline to Universities of China (111 Program, D20023).
References
- X. B. Wang, X. C. Fu, J. H. Yan, A. Wang, M. Q. Wang, M. Chen, C. L. Yang and Y. M. Song, Mol. Diversity, 2019, 23, 573–583 CrossRef CAS PubMed.
- S. C. Jiang, X. Tang, M. Chen, J. He, S. J. Su, L. W. Liu, M. He and W. Xue, Pest Manage. Sci., 2020, 76, 853–860 CrossRef CAS PubMed.
- J. Shi, M. H. Ding, N. Luo, S. R. Wan, P. J. Li, J. H. Li and X. P. Bao, J. Agric. Food Chem., 2020, 68, 9613–9623 CrossRef CAS PubMed.
- Q. Q. Tao, L. W. Liu, P. Y. Wang, Q. S. Long, Y. L. Zhao, L. H. Jin, W. M. Xu, Y. Chen, Z. Li and S. Yang, J. Agric. Food Chem., 2019, 67, 7626–7639 CrossRef CAS PubMed.
- B. Saul and W. Ron, Mol. Plant Pathol., 2012, 13, 805–815 CrossRef PubMed.
- X. F. Shang, L. X. Dai, Z. J. Zhang, C. J. Yang, S. S. Du, T. L. Wu, Y. H. He, J. K. Zhu, Y. Q. Liu, Y. F. Yan, X. L. Miao and J. Y. Zhang, J. Agric. Food Chem., 2021, 69, 6455–6464 CrossRef CAS PubMed.
- N. A. Slaton, R. D. Cartwright, J. Meng, E. E. Gbur and R. J. Norman, Agron. J., 2003, 95, 1489–1496 CrossRef CAS.
- D. Durgadevi, S. Harish, R. Manikandan, S. R. Prabhukarthikeyan, D. Alice and T. Raguchander, Physiol. Mol. Plant Pathol., 2021, 115, 101669 CrossRef CAS.
- S. Horuz, J. Plant Pathol., 2021, 103, 751–758 CrossRef.
- X. Tang, S. J. Su, M. Chen, J. He, R. J. Xia, T. Guo, Y. Chen, C. Zhang, J. Wang and W. Xue, RSC Adv., 2019, 9, 6011–6020 RSC.
- X. B. Wang, X. C. Fu, M. Chen, A. Wang, J. H. Yan, Y. D. Mei, M. Q. Wang and C. L. Yang, Chin. Chem. Lett., 2019, 30, 1419–1422 CrossRef CAS.
- T. Guo, R. J. Xia, M. Chen, S. J. Su, J. He, M. He, H. Wang and W. Xue, RSC Adv., 2019, 9, 24942–24950 RSC.
- A. Keivanloo, S. Abbaspour, S. Sepehri and M. Bakherad, Polycyclic Aromat. Compd., 2020, 1–14 CrossRef.
- H. Behzadi, P. Roonasi, K. A. taghipour, D. V. Spoel and S. Manzetti, J. Mol. Struct., 2015, 1091, 196–202 CrossRef CAS.
- A. Soozani, A. Keivanloo and M. Bakherad, ChemistrySelect, 2017, 2, 9701–9705 CrossRef CAS.
- S. Alavi, M. H. Mosslemin, R. Mohebat and A. R. Massah, Res. Chem. Intermed., 2017, 43, 4549–4559 CrossRef CAS.
- R. J. Xia, T. Guo, M. Chen, S. J. Su, J. He, X. Tang, S. C. Jiang and W. Xue, New J. Chem., 2019, 43, 16461–16467 RSC.
- X. Y. Li, Y. H. Wang, K. Chen, D. Gao, D. M. Wang and W. Xue, Pestic. Biochem. Physiol., 2019, 155, 45–50 CrossRef CAS PubMed.
- X. M. Zhao, C. C. Xia, X. D. Wang, H. Wang, M. Xin, Y. L. Xin and Y. L. Liang, Front. Pharmacol., 2018, 9, 126–136 CrossRef PubMed.
- A. G. Giuglio-Tonolo, C. Curti, T. Terme and P. Vanelle, Molecules, 2020, 25, 5922 CrossRef CAS PubMed.
- B. Galina, S. Alexander, K. Alexander and D. Pavel, Curr. Org. Synth., 2020, 17, 29–39 CrossRef PubMed.
- R. S. Keri, S. S. Pandule, S. Budagumpi and B. M. Nagaraja, Arch. Pharm., 2018, 351, e1700325 CrossRef PubMed.
- M. A. Sibiya, L. Raphoko, D. Mangokoana, R. Makola, W. Nxumalo and T. M. Matsebatlela, Molecules, 2019, 24, 407–423 CrossRef PubMed.
- A. M. Newahie, Y. M. Nissan, N. M. Ismail, D. A. Ella, S. M. Khojah and K. A. Abouzid, Molecules, 2019, 24, 1175–1197 CrossRef PubMed.
- M. K. Ibrahim, I. H. Eissa, A. E. Abdallah, A. M. Metwaly, M. M. Radwan and M. A. ElSohly, Bioorg. Med. Chem., 2017, 25, 1496–1513 CrossRef CAS PubMed.
- S. Tariq, O. Alam and M. Amir, Bioorg. Chem., 2017, 76, 343–358 CrossRef PubMed.
- C. Gonçalves, A. Dimou, V. Sakkas, M. F. Alpendurada and T. A. Albanis, Chemosphere, 2006, 64, 1375–1382 CrossRef PubMed.
- X. Y. Yu, C. Huang, L. Wu, S. J. Hua, J. Ye, L. Meng and C. Xu, Sci. Total Environ., 2021, 776, 146036 CrossRef CAS.
- R. J. Xia, T. Guo, J. He, M. Chen, S. J. Su, S. C. Jiang, X. Tang, Y. Chen and W. Xue, Monatsh. Chem., 2019, 150, 1325–1334 CrossRef CAS.
- M. I. Shahin, D. E. Ella, N. M. Ismail and K. A. Abouzid, Bioorg. Chem., 2014, 56, 16–26 CrossRef CAS PubMed.
- B. T. Fahr, T. O'Brien, P. Pham, N. D. Waal, S. Baskaran, B. C. Raimundo, J. W. Lam, M. M. Sopko, H. E. Purkey and M. J. Romanowski, Bioorg. Med. Chem. Lett., 2005, 16, 559–562 CrossRef PubMed.
- M. Moghaddam, M. R. Alymanesh, L. Mehdizadeh, H. Mirzaei and A. G. Pirbalouti, Ind. Crops Prod., 2014, 59, 144–148 CrossRef CAS.
- X. B. Wang, A. Wang, L. L. Qiu, M. Chen, A. M. Lu, G. H. Li, C. L. Yang and W. Xue, J. Agric. Food Chem., 2020, 68, 14426–14437 CrossRef CAS PubMed.
- M. Zhang, M. L. Dou, Y. Y. Xia, Z. Hu, B. J. Zhang, Y. X. Bai, J. Xie, Q. F. Liu, C. P. Xie, D. D. Lu, S. Hou, J. G. He, J. Tao and R. F Sun, J. Agric. Food Chem., 2021, 69, 5435–5445 CrossRef CAS PubMed.
- Y. H. Ye, L. Ma, Z. C. Dai, Y. Xiao, Y. Y. Zhang, D. D. Li, J. X. Wang and H. L. Zhu, J. Agric. Food Chem., 2014, 62, 4063–4071 CrossRef CAS PubMed.
- Z. J. Zhang, Y. Zeng, Z. Y. Jiang, B. S. Shu, V. Sethuraman and G. H. Zhong, Pest Manage. Sci., 2018, 74, 1736–1746 CrossRef CAS PubMed.
- X. Wang, Z. C. Dai, Y. F. Chen, L. L. Cao, W. Yan, S. K. Li, J. X. Wang, Z. G. Zhang and Y. H. Ye, Eur. J. Med. Chem., 2017, 126, 171–182 CrossRef CAS PubMed.
- D. Peng, S. D. Li, J. X. Wang, C. J. Chen and M. G. Zhou, Pest Manage. Sci., 2014, 70, 258–263 CrossRef CAS PubMed.
- Z. J. Zhang, Z. Y. Jiang, Q. Zhu and G. H. Zhong, J. Agric. Food Chem., 2018, 66, 9598–9607 CrossRef CAS PubMed.
- S. C Jiang, S. J. Su, M. Chen, F. Peng, Q. Zhou, T. T. Liu, L. W. Liu and W. Xue, J. Agric. Food Chem., 2020, 68, 5641–5647 CrossRef PubMed.
- M. Chen, X. M. Tang, T. T. Liu, F. Peng, Q. Zhou, H. Luo, M. He and W. Xue, Fitoterapia, 2021, 149, 104804 CrossRef CAS PubMed.
- J. Zhang, L. T. Yan, E. L. Yuan, H. X. Ding, H. C. Ye, Z. K. Zhang, C. Yan, Y. Q. Liu and G. Feng, J. Agric. Food Chem., 2014, 62, 4905–4910 CrossRef CAS PubMed.
- L. Y. Feng, F. W. Wu, J. Li, Y. M. Jiang and X. W. Duan, Postharvest Biol. Technol., 2011, 61, 160–164 CrossRef CAS.
Footnotes |
† Electronic supplementary information (ESI) available. See DOI: 10.1039/d1ra07559d |
‡ These authors contributed equally to this work. |
|
This journal is © The Royal Society of Chemistry 2022 |
Click here to see how this site uses Cookies. View our privacy policy here.