DOI:
10.1039/D1RA08710J
(Paper)
RSC Adv., 2022,
12, 9058-9068
Application of novel metal–organic framework [Zr-UiO-66-PDC-SO3H]FeCl4 in the synthesis of dihydrobenzo[g]pyrimido[4,5-b]quinoline derivatives†
Received
29th November 2021
, Accepted 8th March 2022
First published on 23rd March 2022
Abstract
In the current paper, we produce a new metal–organic framework (MOF) based on Zr metal, [Zr-UiO-66-PDC-SO3H]FeCl4, via an anion exchange method, which is fully characterized by FT-IR, SEM with elemental mapping and EDX, FE-SEM and TEM. Furthermore, the use of [Zr-UiO-66-PDC-SO3H]FeCl4 as a porous catalyst was examined for the one-pot synthesis of novel dihydrobenzo[g]pyrimido[4,5-b]quinoline derivatives by reaction of 6-amino-1,3-dimethylpyrimidine-2,4(1H,3H)-dione, 2-hydroxynaphthalene-1,4-dione and various aldehydes at 100 °C with good to excellent yields.
Introduction
Nowadays, porous materials such as metal–organic frameworks (MOFs) are of great interest to scientists.1,2 These crystalline materials comprise metal and organic compounds as the nucleus and ligands, respectively. MOFs are multifunctional materials that have been used as adsorbents for the storage and separation of gas, drug delivery, catalyst, proton conductivity and heavy metal adsorbents.3–9 Post- modification and synthesis of MOFs with acid functional groups and metal have been reported for the transportation of organic compounds, oxidation, and synthesis of biological compounds.10 Lillerud et al. reported the first MOFs based on Zr, such as Zr-UiO-66-PDC.11 Considering this, the new class of porous catalyst with sulfonic and phosphorus acid tag-MOFs have been applied in the preparation of pyrimido[4,5-b]quinolones and dicyanomethylene pyridine derivatives – N-amino-2-pyridone and pyrano [2,3-c]pyrazole derivatives.12–15
Anion exchange is an architectonic method for the preparation of ionic liquids (ILs) and/or molten salts (MSs) with several opposing ions that cannot be synthesized directly. Our research group has introduced MSs with N–S bonds as a new category of catalyst and reagent based on organic materials.16–24 Now, we combine the porous materials MOF Zr-UiO-66-PDC with ClSO3H, to prepare [Zr-UiO-66-PDC-SO3H]Cl as a novel porous catalyst for ILs.
Recently, N-heterocyclic scaffold compounds have been considered as candidates for the design and discovery of new biologically active compounds. It is very important to supply new and easy methods for the preparation of target compounds with unique features. In this regard, 1,4-dihydropyridine structures containing uracil and henna (2-hydroxynaphthalene-1,4-dione) moieties are suitable candidates for biological and pharmacological purposes.25–29 These molecules have been used as drugs in furnidipine and alogliptin (Fig. 1).30,31 Also, scaffolds with uracil moieties have been reported as having antitumour,32 cardiotonic,33 hepatoprotactive,34 antihypertensive,35 antibronchitic36 and antifungal activity.37 Therefore, the appearance of novel and simple organic synthetic approaches for the efficient preparation of this type of heterocycle is an interesting challenge. Since scaffolds with uracil and henna moieties are of biological interest,38,39 we synthesize 1,4-dihydropyridine with uracil and henna moieties.
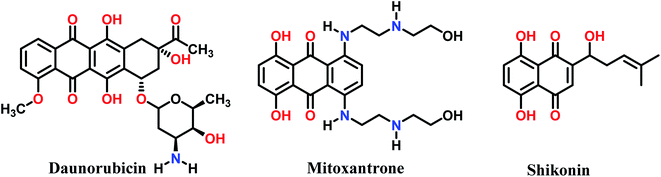 |
| Fig. 1 Biological compounds containing uracil, henna and dihydropyridine moieties in their structures. | |
In continuation of our investigation on the development and preparation of MOFs with sulfonic acid tags and organic molecules with henna moieties,40 in this paper, we prepare [Zr-UiO-66-PDC-SO3H]FeCl4 as an efficient and novel porous catalyst for new dihydrobenzo[g]pyrimido[4,5-b]quinoline derivatives by condensation reaction of 2-hydroxynaphthalene-1,4-dione, 6-amino-1,3-dimethylpyrimidine-2,4(1H,3H)-dione and various aldehydes (mono and bis) under solvent free conditions at 100 °C (Fig. 2).
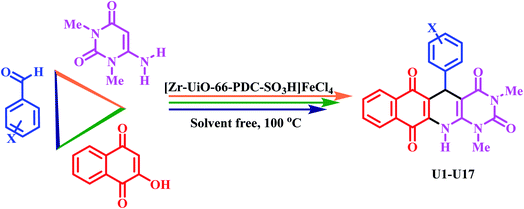 |
| Fig. 2 Preparation of dihydrobenzo[g]pyrimido[4,5-b]quinoline using [Zr-UiO-66-PDC-SO3H]FeCl4. | |
Experimental
Preparation of [Zr-UiO-66-PDC-SO3H]FeCl4
Initially, our MOFs [Zr-UiO-66-PDC] were synthesized according to a previously reported methodology.41 In a round-bottomed flask, 50 mL, a mixture of ClSO3H (2 mmol, 0.134 mL) and [Zr-UiO-66-PDC] (0.564 g) in dry CH2Cl2 (30 mL) at 0 °C was stirred for 2 hours. After this, a white precipitate appeared which was separated (by centrifugation) and dried under vacuum. Then, according to the anion exchange method, a mixture of [Zr-UiO-66-PDC-SO3H]Cl (2 g) and FeCl3 (5 mmol, 0.81 g) was stirred in a mortar at 50 °C for 2 hours. After completion of the reaction, the reaction mixture was cooled down to room temperature. Finally, acetone was used to purify the [Zr-UiO-66-PDC-SO3H]FeCl4 via trituration (Fig. 3).
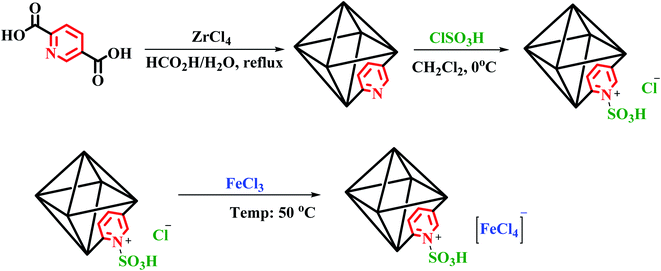 |
| Fig. 3 Preparation of [Zr-UiO-66-PDC-SO3H]FeCl4. | |
General procedure for the synthesis of dihydrobenzo[g]pyrimido[4,5-b]quinoline derivatives using [Zr-UiO-66-PDC-SO3H]FeCl4
In a 15 mL round-bottomed flask, a mixture of 2-hydroxynaphthalen-1,4-dione (henna, 1 mmol, 0.174 g), 6-amino-1,3-dimethylpyrimidine-2,4(1H,3H)-dione (1 mmol, 0.155 g) and aldehyde (1 mmol) in the presence of 10 mg of [Zr-UiO-66-PDC-SO3H]FeCl4 was stirred at 100 °C under solvent-free conditions. After the completion of the reactions which were monitored by the TLC technique (2
:
3 n-hexane
:
ethyl acetate). The described catalyst was separated from the reaction mixture by centrifugation (1000 rpm) after adding 10 mL of EtOH as solvent. Finally, after the evaporation of the solvent at room temperature, pure product was obtained by washing with hot ethanol and water (Fig. 2).
Spectra data
1,3-Dimethyl-5-(p-tolyl)-5,12-dihydrobenzo[g]pyrimido[4,5-b]quinolone 2,4,6,11(1H,3H)-tetraone (U1). Red solid; Mp: 280 °C dec.; FT-IR (KBr) υ (cm−1) = 3401, 3245, 1702, 1575, 1510. 1H NMR (400 MHz, DMSO-d6) δ 7.89 (d, J = 7.5 Hz, 1H), 7.80 (d, J = 7.1 Hz, 1H), 7.69 (t, J = 8.0 Hz, 1H), 7.62 (s, 1H), 7.57 (t, J = 7.6 Hz, 1H), 6.95 (s, 4H), 6.30 (s, 1H), 3.31 (s, 3H), 3.21 (s, 3H), 2.26 (s, 3H). 13C NMR (101 MHz, DMSO) δ 180.3, 162.3, 150.6, 135.4, 133.4, 132.5, 131.2, 130.4, 127.9, 126.6, 125.7, 125.4, 125.2, 124.7, 87.0, 34.4, 29.6, 28.1, 20.5. MS: m/z (%) = 413.2.
1,3-Dimethyl-5-(4-nitrophenyl)-5,12-dihydrobenzo[g]pyrimido[4,5-b]quinoline 2,4,6,11(1H,3H)-tetraone (U2). Red solid; Mp: >300 °C; FT-IR (KBr) υ (cm−1) = 3396, 3230, 1694, 1656, 1605, 1576, 1342. 1H NMR (400 MHz, DMSO-d6) δ 7.86 (d, J = 7.6 Hz, 1H), 7.77 (d, J = 6.4 Hz, 1H), 7.66 (t, J = 5.6 Hz, 1H), 7.54 (s, 2H), 7.16 (d, J = 7.4 Hz, 2H), 7.03 (d, J = 6.9 Hz, 2H), 6.27 (s, 1H), 3.27 (s, 3H), 3.18 (s, 3H). 13C NMR (101 MHz, DMSO-d6) δ 180.0, 150.7, 135.4, 133.4, 131.3, 130.3, 128.6, 128.2, 127.6, 127.1, 125.4, 124.7, 119.1, 86.4, 34.5, 29.5, 28.1. MS: m/z (%) = 444.1.
5-(4-Chlorophenyl)-1,3-dimethyl-5,12-dihydrobenzo[g]pyrimido[4,5-b]quinoline-2,4,6,11(1H,3H)-tetraone (U3). Red solid; Mp: >300 °C; FT-IR (KBr) υ (cm−1) = 3456, 3336, 1693, 1596, 1508. 1H NMR (400 MHz, DMSO-d6) δ 8.00 (d, J = 8.7 Hz, 2H), 7.86 (d, J = 7.5 Hz, 1H), 7.77 (d, J = 7.3 Hz, 1H), 7.66 (t, J = 7.2 Hz, 1H), 7.53 (t, J = 7.3 Hz, 1H), 7.47 (s, 1H), 7.26 (d, J = 8.4 Hz, 2H), 6.39 (s, 1H), 3.26 (s, 3H), 3.17 (s, 3H). 13C NMR (101 MHz, DMSO) δ 185.8, 179.6, 161.7, 154.3, 152.6, 150.8, 144.4, 135.4, 133.4, 131.3, 130.3, 127.9, 127.8, 125.4, 124.7, 122.6, 118.6, 85.8, 35.8, 29.5, 27.9. MS: m/z (%) = 433.1[M], 435.2 [M + 2].
5-(3,4-Difluorophenyl)-1,3-dimethyl-5,12-dihydrobenzo[g]pyrimido[4,5-b]quinoline-2,4,6,11(1H,3H)-tetraone (U4). Red solid; Mp:>300 °C; FT-IR (KBr) υ (cm−1) = 3392, 3061, 1691,1669, 1591, 1510. 1H NMR (400 MHz, DMSO-d6) δ 7.88–7.85 (m, 1H), 7.77–7.74 (m, 1H), 7.68–7.64 (m, 1H), 7.54–7.50 (m, 1H), 7.44 (s, 1H), 7.16–7.09 (m, 1H), 6.90 (d J = 9.1 Hz, 1H), 6.83–6.77 (m, 1H), 6.27 (s, 1H), 3.25 (s, 3H), 3.16 (s, 3H). 13C NMR (100 MHz, DMSO) δ 163.9, 154.4, 152.1, 147.9, 140.9, 133.8, 132.5, 129.5, 124.0, 123.3, 121.2, 120.8, 116.9, 114.3, 93.4, 37.2, 30.4, 29.0. MS: m/z (%) = 435.2.
1,3-Dimethyl-5-(4-(trifluoromethyl)phenyl)-5,12-dihydrobenzo[g]pyrimido[4,5-b]quinoline-2,4,6,11(1H,3H)-tetraone (U5). Red solid; Mp: 290 °C dec.; FT-IR (KBr) υ (cm−1) = 3403, 3247, 1702, 1603, 1575, 1511. 1H NMR (400 MHz, DMSO-d6) δ 7.96 (d, J = 7.5 Hz, 1H), 7.87 (d, J = 7.4 Hz, 1H), 7.76 (t, J = 7.3 Hz, 1H), 7.66–7.60 (m, 2H), 7.56 (d, J = 8.1 Hz, 2H), 7.32 (d, J = 7.9 Hz, 2H), 6.45 (s, 1H), 3.36 (s, 3H), 3.27 (s, 3H). 13C NMR (101 MHz, DMSO-d6) δ 185.6, 162.0, 152.9, 150.7, 135.4, 133.4, 131.3, 130.4, 127.3, 126.2, 125.4, 124.7, 124.2, 118.9, 86.1, 35.2, 29.6, 28.1. MS: m/z (%) = 467.1.
5-(3,5-Difluorophenyl)-1,3-dimethyl-5,12-dihydrobenzo[g]pyrimido[4,5-b]quinoline-2,4,6,11(1H,3H)-tetraone (U6). Red solid; Mp: >300 °C; FT-IR (KBr) υ (cm−1) = 3393, 3238, 1691, 1659, 1594, 1509. 1H NMR (400 MHz, DMSO-d6) δ 8.00 (d, J = 7.4 Hz, 1H), 7.89 (d, J = 7.1 Hz, 1H), 7.81–7.77 (m, 1H), 7.66 (t, J = 7.4 Hz, 1H), 6.94 (s, 1H), 6.77 (s, 1H), 6.75 (s, 1H), 6.41 (s, 1H), 3.38 (s, 3H), 3.29 (s, 3H). 13C NMR (100 MHz, DMSO) δ 183.5, 150.7, 133.8, 133.2, 131.9, 130.9, 128.7, 128.4, 128.1, 125.7, 125.1, 123.3, 121.8, 117.7, 116.4, 115.8, 53.5, 32.5, 29.6, 28.0. MS: m/z (%) = 436.2.
5-(3-Hydroxyphenyl)-1,3-dimethyl-5,12-dihydrobenzo[g]pyrimido[4,5-b]quinoline-2,4,6,11(1H,3H)-tetraone (U7). Red solid; Mp:280 °C dec.; FT-IR (KBr) υ (cm−1) = 3397, 3250, 1674, 1609, 1578, 1512. 1H NMR (400 MHz, DMSO-d6) δ 8.83 (s, 1H), 7.92 (d, J = 7.6 Hz, 1H), 7.81 (d, J = 7.2 Hz, 1H), 7.71 (t, J = 7.2 Hz, 1H), 7.60 (s, 1H), 7.57 (d, J = 7.3 Hz, 1H), 6.95 (t, J = 7.7 Hz, 1H), 6.53 (d, J = 7.4 Hz, 2H), 6.45 (d, J = 7.8 Hz, 1H), 6.29 (s, 1H), 3.33 (s, 3H), 3.23 (s, 3H). 13C NMR (101 MHz, DMSO-d6) δ 182.1, 156.7, 135.7, 133.4, 131.3, 130.2, 127.9, 125.4, 124.5, 119.6, 117.7, 113.7, 110.8, 87.0, 34.8, 29.5, 28.0. MS: m/z (%) = 415.1.
1,3-Dimethyl-5-phenyl-5,12-dihydrobenzo[g]pyrimido[4,5-b]quinoline-2,4,6,11(1H,3H)-tetraone (U8). Red solid; Mp: 255–256 °C; FT-IR (KBr) υ (cm−1) = 3407, 3244, 1699, 1605, 1578, 1509. 1H NMR (400 MHz, DMSO-d6) δ 13.21 (s, 1H), 8.03 (d, J = 7.4 Hz, 1H), 8.00 (d, J = 8.5 Hz, 1H), 7.87–7.80 (m, 2H), 7.22 (dt, J = 16.9, 5.6 Hz, 5H), 5.86 (s, 1H), 3.38 (s, 3H), 3.15 (s, 3H). 13C NMR (101 MHz, DMSO-d6) δ 181.1, 163.6, 154.3, 150.1, 138.4, 134.3, 133.9, 133.5, 132.0, 131.7, 130.5, 128.0, 127.7, 126.7, 126.0, 125.7, 125.1, 124.8, 123.4, 85.6, 34.7, 30.4, 28.2. MS: m/z (%) = 399.2.
5-(2-Methoxyphenyl)-1,3-dimethyl-5,12-dihydrobenzo[g]pyrimido[4,5-b]quinoline-2,4,6,11(1H,3H)-tetraone (U9). Red solid; Mp: 288 °C dec.; FT-IR (KBr) υ(cm−1) = 3442, 3355, 1696, 1633, 1657, 1504. 1H NMR (400 MHz, DMSO-d6) δ 9.02 (s, 1H), 8.06 (s, 1H), 7.99–7.71 (m, 3H), 7.25 (d, J = 100.1 Hz, 2H), 6.96–6.75 (m, 2H), 5.31 (s, 1H), 3.70 (s, 3H), 3.61 (s, 3H), 3.09 (s, 3H). 13C NMR (101 MHz, DMSO-d6) δ 181.8, 160.4, 150.6, 139.4, 135.0, 133.5, 131.6, 128.1, 125.9, 125.8, 125.8, 122.5, 119.7, 111.8, 88.3, 55.4, 33.8, 29.7, 27.6. MS: m/z (%) = 429.2.
5-(2,4-Dichlorophenyl)-1,3-dimethyl-5,12-dihydrobenzo[g]pyrimido[4,5-b]quinoline-2,4,6,11(1H,3H)-tetraone (U10). Red solid; Mp: >300 °C; FT-IR (KBr) υ (cm−1) = 3456, 3336, 1693, 1596, 1508. 1H NMR (400 MHz, DMSO-d6) δ 7.96 (s, 2H), 7.89 (s, 1H), 7.77 (s, 1H), 7.69 (s, 1H), 7.41 (s, 1H), 7.35 (s, 1H), 7.27 (s, 1H), 6.51 (s, 1H), 2.90 (s, 3H), 2.74 (s, 3H).13C NMR (101 MHz, DMSO-d6) δ 177.9, 176.9, 158.9, 158.6, 156.4, 153.0, 151.8, 150.5, 150.4, 136.6, 136.6, 135.4, 134.9, 132.6, 132.4, 132.3, 132.1, 131.9, 128.8, 128.2, 127.8, 127.7, 127.2, 127.0, 126.8, 121.3, 104.2, 79.3, 31.3, 30.0, 28.0. MS: m/z (%) = 466.8 [M], 469 [M + 2].
5-(4-Methoxyphenyl)-1,3-dimethyl-5,12-dihydrobenzo[g]pyrimido[4,5-b]quinoline-2,4,6,11(1H,3H)-tetraone (U11). Red solid; Mp: 232–234 °C; FT-IR (KBr) υ (cm−1) = 3398, 3238, 1702, 1607, 1580, 1511.1H NMR (400 MHz, DMSO-d6) δ 8.04–7.99 (m, 1H), 7.98 (d, J = 7.3 Hz, 1H), 7.86–7.78 (m, 2H), 7.20 (s, 1H), 7.09 (d, J = 8.3 Hz, 2H), 6.79 (d, J = 8.7 Hz, 2H), 5.80 (s, -1H), 3.71 (s, 3H), 3.36 (s, 3H), 3.14 (s, 3H). 13C NMR (101 MHz, DMSO-d6) δ 163.4, 157.2, 154.2, 150.2, 134.3, 133.8, 133.3, 130.5, 127.7, 126.0, 125.6, 113.4, 85.9, 54.9, 34.0, 30.3, 28.1. MS: m/z (%) = 429.1.
5-(4-(Dimethylamino)phenyl)-1,3-dimethyl-5,12-dihydrobenzo[g]pyrimido[4,5-b]quinoline-2,4,6,11(1H,3H)-tetraone (U12). Red solid; Mp: 230 °C dec.; FT-IR (KBr) υ (cm−1) = 3402, 3357, 1671, 1597, 1574, 1515. 1H NMR (400 MHz, DMSO-d6) δ 7.92 (d, J = 7.7 Hz, 1H), 7.82 (d, J = 7.3 Hz, 1H), 7.75–7.68 (m, 1H), 7.61–7.56 (m, 1H), 6.90 (d, J = 8.4 Hz, 1H), 6.86 (s, 2H), 6.62 (d, J = 8.5 Hz, 1H), 4.77 (s, 1H), 3.31 (s, 3H), 3.24 (s, 3H), 3.14 (s, 3H), 2.87 (s, 3H). 13C NMR (101 MHz, DMSO-d6) δ 185.4, 180.6, 163.1, 158.0, 153.9, 149.7, 145.1, 135.1, 133.9, 133.0, 131.2, 130.1, 126.1, 125.5, 125.2, 123.2, 85.2, 40.6, 34.0, 30.0, 27.7. MS: m/z (%) = 441.2.
5-(4-Isopropylphenyl)-1,3-dimethyl-5,12-dihydrobenzo[g]pyrimido[4,5-b]quinoline-2,4,6,11(1H,3H)-tetraone (U13). Red solid; Mp: >300 °C; FT-IR (KBr) υ (cm−1) = 3408, 3130, 2925, 1668, 1590, 1511. 1H NMR (400 MHz, DMSO-d6) δ 7.85 (d, J = 7.5 Hz, 1H), 7.73 (d, J = 7.6 Hz, 1H), 7.64 (t, J = 7.4 Hz, 1H), 7.53–7.47 (m, 2H), 6.96 (d, J = 8.1 Hz, 2H), 6.90 (d, J = 7.9 Hz, 2H), 6.24 (s, 1H), 3.25 (s, 3H), 3.15 (s, 3H), 2.82–2.74 (m, 1H), 1.17 (d, J = 6.8 Hz, 6H). 13C NMR (101 MHz, DMSO-d6) δ 185.9, 181.1, 163.6, 158.5, 154.4, 150.1, 145.5, 135.6, 134.3, 133.5, 131.7, 130.6, 126.6, 126.1, 126.0, 125.7, 123.7, 85.6, 34.4, 32.9, 30.4, 28.2, 24.0, 23.9. MS: m/z (%) = 441.2.
5-(4-Hydroxyphenyl)-1,3-dimethyl-5,12-dihydrobenzo[g]pyrimido[4,5-b]quinoline-2,4,6,11(1H,3H)-tetraone (U14). Red solid; Mp: 220 °C dec.; FT-IR (KBr) υ (cm−1) = 3393, 3229, 1671, 1654, 1509. 1H NMR (400 MHz, DMSO-d6) δ 8.49 (s, 1H), 7.86 (d, J = 7.7 Hz, 1H), 7.75 (d, J = 7.6 Hz, 1H), 7.65 (t, J = 7.5 Hz, 2H), 7.52 (d, J = 7.5 Hz, 2H), 7.43 (s, 1H), 7.17–7.07 (m, 2H), 6.90 (d, J = 7.8 Hz, 2H), 6.26 (s, 1H), 3.24 (s, 3H), 3.16 (s, 3H). 13C NMR (101 MHz, DMSO-d6) δ 180.0, 150.7, 142.9, 135.4, 133.4, 131.3, 130.3, 128.6, 128.2, 127.1, 125.4, 124.7, 86.4, 34.5, 29.5, 28.1. MS: m/z (%) = 415.2.
1,3-Dimethyl-5-(3-nitrophenyl)-5,12-dihydrobenzo[g]pyrimido[4,5-b]quinoline-2,4,6,11(1H,3H)-tetraone (U15). Red solid; Mp: 300 °C dec.; FT-IR (KBr) υ (cm−1) = 3334, 3508, 1717, 1682, 1562, 1529. 1H NMR (400 MHz, DMSO-d6) δ 7.90 (d, J = 7.9 Hz, 1H), 7.87 (d, J = 8.2 Hz, 1H), 7.78 (s, 1H), 7.67 (t, J = 7.1 Hz, 1H), 7.56–7.51 (m, 1H), 7.49–7.39 (m, 3H), 6.39 (s, 1H), 3.27 (s, 3H), 3.17 (s, 3H). 13C NMR (101 MHz, DMSO-d6) δ 185.8, 179.8, 161.7, 152.7, 150.8, 147.6, 147.3, 135.4, 133.8, 133.4, 131.4, 130.3, 128.7, 125.4, 124.7, 121.2, 119.1, 118.4, 85.8, 35.2, 29.5, 28.0. MS: m/z (%) = 444.1.
5,5’-(1,3-Phenylene)bis(1,3-dimethyl-5,12-dihydrobenzo[g]pyrimido[4,5-b]quinoline-2,4,6,11(1H,3H)-tetraone) (U16). Red solid; Mp: >300 °C; FT-IR (KBr) υ (cm−1) = 3396, 3200, 1682, 1608, 1579, 1508.1H NMR (400 MHz, DMSO-d6) δ 8.00 (d, J = 7.9 Hz, 2H), 7.97 (d, J = 8.2 Hz, 2H), 7.88 (s, 2H), 7.77 (t, J = 7.1 Hz, 2H), 7.66–7.61 (m, 2H), 7.57 (d, J = 7.2 Hz, 2H), 7.55–7.50 (m, 2H), 6.49 (s, 2H), 3.37 (s, 6H), 3.27 (s, 6H). 13C NMR (101 MHz, DMSO-d6) δ 193.8, 161.7, 152.6, 150.9, 145.8, 135.8, 135.6, 133.4, 131.4, 130.2, 128.1, 127.9, 125.6, 125.4, 124.6, 118.9, 86.2, 35.0, 29.5, 27.9. MS: m/z (%) = 718.1.
5,5’-(1,4-Phenylene)bis(1,3-dimethyl-5,12-dihydrobenzo[g]pyrimido[4,5-b]quinoline-2,4,6,11(1H,3H)-tetraone) (U17). Red solid; Mp: 289 °C dec.; FT-IR (KBr) υ (cm−1) = 3396, 3200, 1682, 1608, 1579, 1508. 1H NMR (400 MHz, DMSO-d6) δ 1H NMR (400 MHz, DMSO-d6) δ 9.97 (s, 2H), 7.93 (d, J = 7.5 Hz, 2H), 7.85 (d, J = 7.4 Hz, 2H), 7.74 (d, J = 7.8 Hz, 4H), 7.31 (d, J = 7.7 Hz, 4H), 6.45 (s, 2H), 3.35 (s, 6H), 3.25 (s, 6H). 13C NMR (101 MHz, DMSO-d6) δ 185.8, 153.7, 144.6, 142.9, 137.8, 127.7, 127.5, 125.3, 123.4, 122.1, 120.1, 119.9, 117.5, 117.3, 116.5, 110.8, 78.2, 26.9, 21.5, 19.9. MS: m/z (%) = 718.1.
Results and discussion
To improve the catalytic application of MOFs, we have designed and synthesized [Zr-UiO-66-PDC-SO3H]Cl. Post-functionalization of [Zr-UiO-66-PDC] occurred by preparing [Zr-UiO-66-PDC-SO3H]FeCl4 using FeCl3 in a mortar at room temperature (Fig. 3). [Zr-UiO-66-PDC-SO3H]FeCl4 has a dual role as a Brønsted-Lewis acid catalyst for the preparation of biological compounds. For more detail, full characterization of [Zr-UiO-66-PDC-SO3H]FeCl4 as a catalyst was conducted by FT-IR, VSM, EDX, FE-SEM, elemental mapping, SEM and TEM techniques.
Synthesis and characterization of [Zr-UiO-66-PDC-SO3H]FeCl4 as a new metal–organic framework (MOF)
The FT-IR analysis of ZrCl4, [Zr-UiO-66-PDC], [Zr-UiO-66-PDC-SO3H]Cl and [Zr-UiO-66-PDC-SO3H]FeCl4 is shown in Fig. 4. The broad peak at 2700–3500 cm−1 is related to the OH of SO3H functional group. The aromatic C–H and C
C stretching bands are respectively at 2924 and 1626 cm−1. The absorption bands at 1042 and 1136 cm−1 are related to N–S and O–S bond stretching. Furthermore, the absorption bands at 587 cm−1 are linked to the stretching vibrational modes of Fe–Cl groups in FeCl4. The FT-IR spectrum difference between starting materials and [Zr-UiO-66-PDC-SO3H]FeCl4 verified the structure of the catalyst.
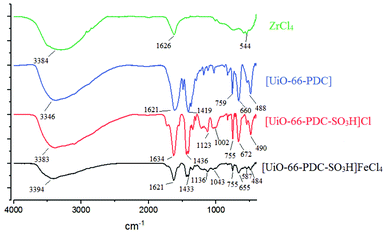 |
| Fig. 4 FT-IR spectra of ZrCl4, [Zr-UiO-66-PDC], [Zr-UiO-66-PDC-SO3H]Cl and [Zr-UiO-66-PDC-SO3H]FeCl4. | |
The materials in the structure of [Zr-UiO-66-PDC-SO3H]FeCl4 were characterized by energy dispersive X-ray spectroscopy (EDX) (Fig. 5). The [Zr-UiO-66-PDC-SO3H]FeCl4 confirmed the existence of Zr, C, O, S, Cl, N and Fe atoms. Furthermore, [Zr-UiO-66-PDC-SO3H]Cl as a well-dispersed material, was determined and verified by SEM-elemental mapping (Fig. 5).
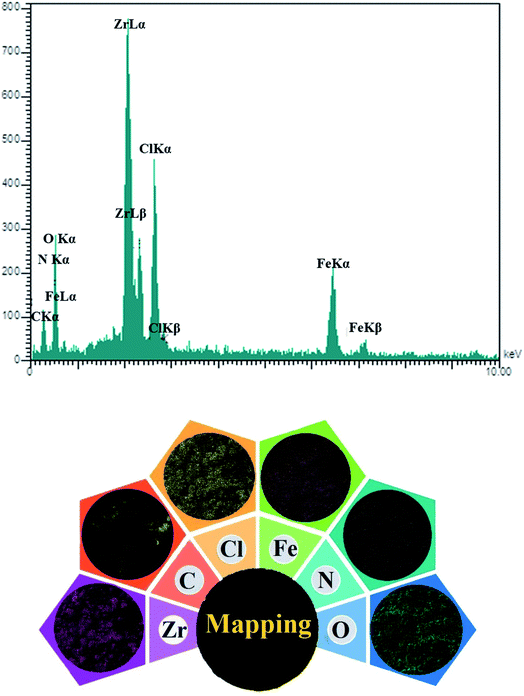 |
| Fig. 5 Upper: energy dispersive X-ray spectroscopy (EDX) of [Zr-UiO-66-PDC-SO3H]FeCl4. Lower: elemental mapping analysis of [Zr-UiO-66-PDC-SO3H]FeCl4. | |
Also, SEM images of [Zr-UiO-66-PDC-SO3H]FeCl4 were recorded to investigate the morphology (Fig. 6). The obtained images show the face centred cubic (fcu) structure. In addition, the topography of [Zr-UiO-66-PDC-SO3H]FeCl4 was studied more closely using transmission electron microscopy (TEM) as shown in Fig. 7. We can see that [Zr-UiO-66-PDC-SO3H]FeCl4 is a fcu topological network with 12-connected Zr clusters.
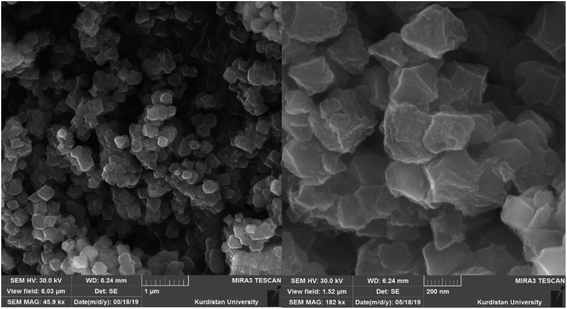 |
| Fig. 6 FE-SEM images of [Zr-UiO-66-PDC-SO3H]FeCl4. | |
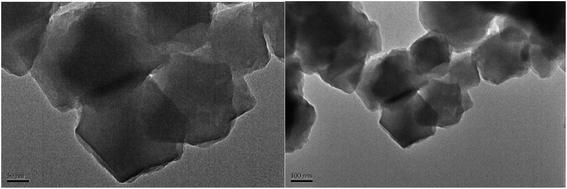 |
| Fig. 7 TEM of [Zr-UiO-66-PDC-SO3H]FeCl4. | |
After the preparation of [Zr-UiO-66-PDC-SO3H]FeCl4 via the anion exchange method, it was tested as a catalyst for the synthesis of new dihydrobenzo[g]pyrimido[4,5-b]quinoline derivatives with uracil and henna (2-hydroxynaphthalene-1,4-dione) moieties. The above-mentioned products were obtained by reaction of 4-methoxy benzaldehyde (1.0 mmol, 0.136 g), 2-hydroxynaphthalen-1,4-dione (1 mmol, 0.174 g), and 6-amino-1,3-dimethylpyrimidine-2,4(1H,3H)-dione (1 mmol, 0.155 g) as a model for the optimization of the reaction conditions. The optimized data is listed in Table 1. As shown in Table 1, the best choice for the synthesis of 5-(4-methoxyphenyl)-1,3-dimethyl-5,12-dihydrobenzo[g]pyrimido[4,5-b]quinoline-2,4,6,11(1H,3H)-tetraone was achieved in the presence of 10 mg [Zr-UiO-66-PDC-SO3H]FeCl4 under solvent-free conditions (entry 4, Table 1). The model reaction was also studied under different temperatures and several solvents – H2O, EtOH, DMF, n-hexane, EtOAc, CH3CN (5 mL) – in the presence of 10 mg of [Zr-UiO-66-PDC-SO3H]FeCl4. As is shown, the results of the reaction did not improve (Table 1, entries 10–15).
Table 1 Effect of different amounts of catalyst, solvent and different temperatures, on the synthesis of 5-(4-methoxyphenyl)-1,3-dimethyl-5,12-dihydrobenzo[g]pyrimido[4,5-b]quinoline-2,4,6,11(1H,3H)-tetraone
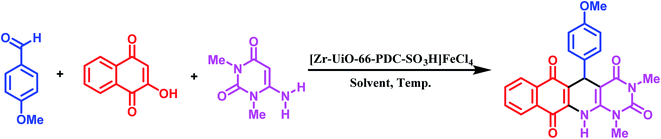
|
Entry |
Amount of catalyst (mg) |
Temp. (o C) |
Solvent (10 mL) |
Time (min) |
Yield (%) |
1 |
— |
100 |
— |
50 |
Trace |
2 |
5 |
100 |
— |
50 |
45 |
3 |
7 |
100 |
— |
50 |
50 |
4 |
10 |
100 |
— |
50 |
90 |
5 |
15 |
100 |
— |
50 |
90 |
6 |
10 |
r.t. |
— |
50 |
75 |
7 |
10 |
50 |
— |
50 |
70 |
8 |
10 |
70 |
— |
50 |
20 |
9 |
15 |
120 |
— |
50 |
90 |
10 |
10 |
Reflux |
H2O |
50 |
85 |
11 |
10 |
Reflux |
EtOH |
50 |
70 |
12 |
10 |
100 |
DMF |
50 |
40 |
13 |
10 |
Reflux |
n-Hexane |
50 |
Trace |
14 |
10 |
Reflux |
EtOAc |
50 |
Trace |
15 |
10 |
Reflux |
CH3CN |
50 |
45 |
After optimizing the reaction conditions, [Zr-UiO-66-PDC-SO3H]FeCl4 (10 mg) is applied to synthesize a range of novel biological and pharmacological candidate compounds using various aromatic aldehydes such as trephetaldehyde, iso-trephetaldehyde, bearing electron-donating and electron-withdrawing groups, 2-hydroxynaphthalen-1,4-dione and 6-amino-1,3-dimethylpyrimidine-2,4(1H,3H)-dione. As shown in Table 2, the obtained results indicated that [Zr-UiO-66-PDC-SO3H]FeCl4 is appropriate for the preparation of target molecules in high to excellent yield (70–90%) with relatively short reaction times (70–120 min).
Table 2 Synthesis of dihydrobenzo[g]pyrimido[4,5-b]quinoline derivatives using [Zr-UiO-66-PDC-SO3H]FeCl4
In the proposed mechanism, the [Zr-UiO-66-PDC-SO3H]FeCl4 catalyst activates the carbonyl functional group of aldehyde. To investigate the activation of the aldehyde, 4-methoxy benzaldehyde was reacted with [Zr-UiO-66-PDC-SO3H]FeCl4 at room temperature. The FT-IR spectra of the subsequent reaction mixtures were examined.15,42,43 The absorption bond of C
O of the 4-methoxy benzaldehyde at 1704 cm−1, was changed to 1704, 1705 or 1711 cm−1 by [Zr-UiO-66-PDC], [Zr-UiO-66-PDC-SO3H]Cl and [Zr-UiO-66-PDC-SO3H]FeCl4 (Fig. 8). Then, the henna (2-hydroxynaphthalen-1,4-dione) moiety reacts with the carbonyl of the aldehyde by removing one H2O molecule, to give intermediate (I) (Fig. 9). In the next step, 6-amino-1,3-dimethylpyrimidine-2,4(1H,3H)-dione reacts with intermediate (I) to give intermediate (II). In the next two steps, intermediate (II) gives the desired product after intramolecular cyclization and the loss of another molecule of H2O.
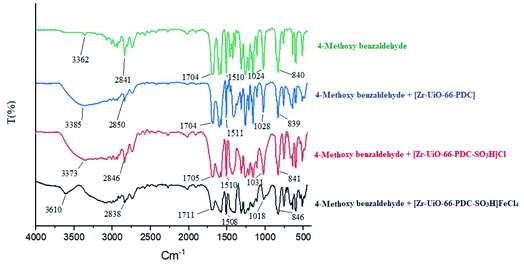 |
| Fig. 8 FT-IR spectra of 4-methoxy benzaldehyde in percent of [Zr-UiO-66-PDC], [Zr-UiO-66-PDC-SO3H]Cl and [Zr-UiO-66-PDC-SO3H]FeCl4. | |
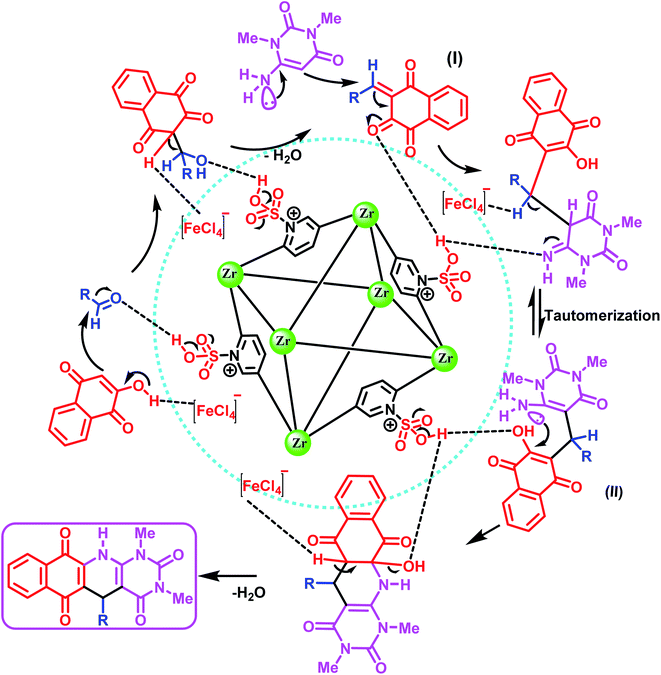 |
| Fig. 9 Proposed mechanism for the synthesis of dihydrobenzo[g]pyrimido[4,5-b]quinoline derivatives using [Zr-UiO-66-PDC-SO3H]FeCl4. | |
To evaluate the performance of [Zr-UiO-66-PDC-SO3H]FeCl4 as a catalyst for the synthesis of dihydrobenzo[g]pyrimido[4,5-b]quinoline derivatives, we tested various acid catalysts (organic and inorganic) in the reaction of 4-methoxy benzaldehyde (1.0 mmol, 0.136 g), 2-hydroxynaphthalen-1,4-dione (1 mmol, 0.174 g), 6-amino-1,3-dimethylpyrimidine-2,4(1H,3H)-dione (1 mmol, 0.155 g) as evaluated in Table 3. The obtained results which are collected in Table 3 show that, [Zr-UiO-66-PDC-SO3H]FeCl4 is the best catalyst for the synthesis of novel dihydrobenzo[g]pyrimido[4,5-b]quinoline derivatives. The obtained results of catalytic activity and reusability of [Zr-UiO-66-PDC-SO3H]FeCl4 are shown in Fig. 10. As mentioned above, [Zr-UiO-66-PDC-SO3H]FeCl4 was separated by centrifugation and reused without significant reduction in its catalytic activity. Recyclability of the catalyst was also studied using the one-pot reaction of 4-methoxy benzaldehyde (1.0 mmol, 0.136 g), 2-hydroxynaphthalen-1,4-dione (1 mmol, 0.174 g), 6-amino-1,3-dimethylpyrimidine-2,4(1H,3H)-dione (1 mmol, 0.155 g) as a model under the above-mentioned optimized reaction conditions. We found that [Zr-UiO-66-PDC-SO3H]FeCl4 can be reused up to four times without noticeable changes in its catalytic activity.
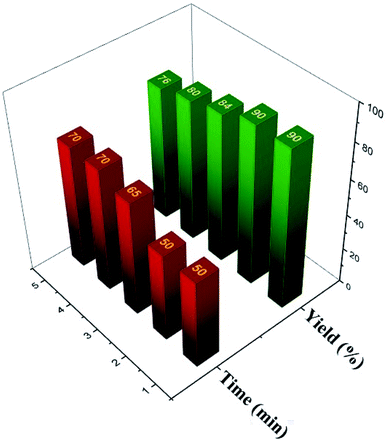 |
| Fig. 10 Recyclability of [Zr-UiO-66-PDC-SO3H]FeCl4 in the synthesis of dihydrobenzo[g]pyrimido[4,5-b]quinoline derivatives. | |
Table 3 Evaluation of various catalysts for the synthesis of 5-(4-methoxyphenyl)-1,3-dimethyl-5,12-dihydrobenzo[g]pyrimido[4,5-b]quinoline-2,4,6,11(1H,3H)-tetraone with [Zr-UiO-66-PDC-SO3H]FeCl4
Entry |
Catalyst |
Amount of catalyst (mol%) |
Time (min) |
Yield (%) |
1 |
NaOH |
10 |
60 |
25 |
2 |
Et3N |
10 |
65 |
— |
3 |
K2CO3 |
10 |
70 |
Trace |
4 |
P-TSA |
10 |
90 |
— |
5 |
SSA 44,45 |
10 mg |
120 |
Trace |
6 |
GTBSA 46 |
10 mg |
80 |
45 |
7 |
[PVI-SO3H]FeCl4 19 |
10 mg |
70 |
70 |
8 |
MIL-100(Cr)–NHEtN(CH2PO3H2)2 15 |
10 mg |
60 |
65 |
9 |
Fe3O4@Co(BDC-NH2) 47 |
10 mg |
50 |
35 |
10 |
CQDs-N(CH2PO3H2)2 48 |
10 mg |
80 |
43 |
11 |
TTPA49 |
10 |
100 |
55 |
12 |
MIL-101(Cr)–N(CH2PO3H2)2 12 |
10 mg |
60 |
80 |
13 |
FeCl3 |
10 |
120 |
50 |
14 |
ZrCl4 |
10 |
120 |
55 |
15 |
Zr-UiO-66-PDC |
10 mg |
120 |
40 |
16 |
[Zr-UiO-66-PDC-SO3H]Cl |
10 mg |
65 |
75 |
17 |
[Zr-UiO-66-PDC-SO3H]FeCl4 |
10 mg |
50 |
90 |
Conclusion
In this study, we have designed, synthesized and introduced [Zr-UiO-66-PDC-SO3H]FeCl4 as a novel mesoporous catalyst, which was fully characterized using various techniques. To the best of our knowledge, this catalyst is the first MOF that was synthesized via the anion exchange method. [Zr-UiO-66-PDC-SO3H]FeCl4 is an efficient catalyst. It was tested for the preparation of new dihydrobenzo[g]pyrimido[4,5-b]quinoline derivatives with henna and uracil moieties which have biological interest.
Conflicts of interest
There are no conflicts to declare.
Acknowledgements
We thank Bu-Ali Sina University and the Iran National Science Foundation (INSF) (Grant number: 98001912) for the financial support of our research group.
References
- H. C. Zhou, J. R. Long and O. M. Yaghi, Introduction to metal–organic frameworks, Chem. Rev., 2012, 112, 673–674 CrossRef CAS
. - B. Zornoza, C. Tellez, J. Coronas, J. Gascon and F. Kapteijn, Metal organic framework based mixed matrix membranes: an increasingly important field of research with a large application potential, Microporous Mesoporous Mater., 2013, 166, 67–78 CrossRef CAS
. - R. Ricco, L. Malfatti, M. Takahashi, A. J. Hill and P. Falcaro, Applications of magnetic metal–organic framework composites, J. Mater. Chem. A, 2013, 1, 13033–13045 RSC
. - J. Lee, O. K. Farha, J. Roberts, K. A. Scheidt, S. T. Nguyen and J. T. Hupp, Metal–organic framework materials as catalysts, Chem. Soc. Rev., 2009, 38, 1450–1459 RSC
. - S. Wang, C. M. McGuirk, A. d’Aquino, J. A. Mason and C. A. Mirkin, Metal–organic framework nanoparticles, Adv. Mater., 2018, 30, 1800202–1800216 CrossRef
. - J. Cao, X. Li and H. Tian, Metal–organic framework (MOF)-based drug delivery, Curr. Med. Chem., 2020, 27, 5949–5969 CrossRef CAS PubMed
. - Y. S. Wei, M. Zhang, R. Zou and Q. Xu, Metal–organic framework-based catalysts with single metal sites, Chem. Rev., 2020, 120, 12089–12174 CrossRef CAS PubMed
. - S. Chen, Y. Li and L. Mi, Porous carbon derived from metal organic framework for gas storage and separation: the size effect, Inorg. Chem. Commun., 2020, 118, 107999–108006 CrossRef CAS
. - H. Sepehrmansourie, Metal Organic Frameworks (MOFs): as multi-purpose catalysts, Iran. J. Catal., 2021, 11, 207–215 CAS
. - Y. Yang, H. F. Yao, F. G. Xi and E. Q. Gao, J. Amino-functionalized Zr (IV) metal–organic framework as bifunctional acid-base catalyst for Knoevenagel condensation, J. Mol. Catal. A: Chem., 2014, 390, 198–205 CrossRef CAS
. - J. H. Cavka, S. Jakobsen, U. Olsbye, N. Guillou, C. Lamberti, S. Bordiga and K. P. Lillerud, A new zirconium inorganic building brick forming metal organic frameworks with exceptional stability, J. Am. Chem. Soc., 2008, 130, 13850–13851 CrossRef PubMed
. - S. Babaee, M. Zarei, H. Sepehrmansourie, M. A. Zolfigol and S. Rostamnia, Synthesis of metal–organic frameworks MIL-101(Cr)-NH2 containing phosphorous acid functional groups: application for the synthesis of N-Amino-2-pyridone and pyrano [2, 3-c] pyrazole derivatives via a cooperative vinylogous anomeric-based oxidation, ACS Omega, 2020, 5, 6240–6249 CrossRef CAS PubMed
. - S. Babaee, M. Zarei, M. A. Zolfigol, S. Khazalpour, M. Hasani, U. Rinner, R. Schirhagl, N. Norouzi and S. Rostamnia, Synthesis of biological based hennotannic acid-based salts over porous bismuth coordination polymer with phosphorous acid tags, RSC Adv., 2021, 11, 2141–2157 RSC
. - A. M. Naseri, M. Zarei, S. Alizadeh, S. Babaee, M. A. Zolfigol, D. Nematollahi, J. Arjomandi and H. Shi, Synthesis and application of [Zr-UiO-66-PDC-SO3H]Cl MOFs to the preparation of dicyanomethylene pyridines via chemical and electrochemical methods, Sci. Rep., 2021, 11, 1–19 CrossRef PubMed
. - H. Sepehrmansouri, M. Zarei, M. A. Zolfigol, A. R. Moosavi-Zare, S. Rostamnia and S. Moradi, Multilinker phosphorous acid anchored En/MIL-100(Cr) as a novel nanoporous catalyst for the synthesis of new N-heterocyclic pyrimido [4, 5-b] quinolines, Mol. Catal., 2020, 481, 110303–110320 CrossRef CAS
. - M. A. Zolfigol, A. Khazaei, A. R. Moosavi-Zare, A. Zare and V. Khakyzadeh, Rapid synthesis of 1-amidoalkyl-2-naphthols over sulfonic acid functionalized imidazolium salts, Appl. Catal., A, 2011, 400, 70–81 CrossRef CAS
. - S. Babaee, M.
A. Zolfigol, M. Zarei, M. Abbasi and Z. Najafi, Synthesis of pyridinium-based salts: catalytic application at the synthesis of six membered O-heterocycles, Mol. Catal., 2019, 475, 110403–110417 CrossRef CAS
. - M. A. Zolfigol, A. Khazaei, A. R. Moosavi-Zare and A. Zare, Ionic liquid 3-Methyl-1-sulfonic acid imidazolium chloride as a novel and highly efficient catalyst for the very rapid synthesis of bis(indolyl)methanes under solvent-free conditions, Org. Prep. Proced. Int., 2010, 42, 95–102 CrossRef CAS
. - H. Sepehrmansourie, M. Zarei, R. Taghavi and M. A. Zolfigol, Mesoporous ionically tagged cross-linked poly(vinyl imidazole)s as novel and reusable catalysts for the preparation of N-heterocycle spiropyrans, ACS Omega, 2019, 4, 17379–17392 CrossRef CAS PubMed
. - H. Sepehrmansourie, M. Zarei, M. A. Zolfigol, A. Mehrzad and H. R. Hafizi-Atabak, Application of [PVI-SO3H]NO3 as a novel polymeric nitrating agent with ionic tags in preparation of high-energetic materials, RSC Adv., 2021, 11, 8367–8374 RSC
. - M. Zarei, E. Noroozizadeh, A. R. Moosavi-Zare and M. A. Zolfigol, Synthesis of nitroolefins and nitroarenes under mild conditions, J. Org. Chem., 2018, 83, 3645–3650 CrossRef CAS PubMed
. - W. Zhu, Y. Pei, Y. Liu, J. Zhang, Y. Qin, Y. Yin and M. D. Guiver, Mass transfer in a Co/N/C catalyst layer for the anion exchange membrane fuel cell, ACS Appl. Mater. Interfaces, 2020, 12, 32842–32850 CrossRef CAS PubMed
. - M. A. Zolfigol, A. Khazaei, A. R. Moosavi-Zare, A. Zare, H. G. Kruger, Z. Asgari, V. Khakyzadeh and M. Kazem-Rostami, Design of ionic liquid 3-methyl-1-sulfonic acid imidazolium nitrate as reagent for the nitration of aromatic compounds by in situ generation of NO2 in acidic Media, J. Org. Chem., 2012, 77, 3640–3645 CrossRef CAS PubMed
. - H. Sepehrmansourie, Magnetic ionic liquids: as multi-purpose catalysts, Iran. J. Catal., 2020, 10, 337–341 Search PubMed
. - H. R. Hudson, N. J. Wardle, S. WA Bligh, I. Greiner, A. Grun and G. Keglevich, N-heterocyclic dronic acids: applications and synthesis, Mini-Rev. Med. Chem., 2012, 12, 313–325 CrossRef CAS PubMed
. - M. De Luca, G. Ioele and G. Ragno, 1, 4-dihydropyridine antihypertensive drugs: Recent advances in photostabilization strategies, Pharmaceutics, 2019, 11, 85–98 CrossRef CAS
. - G. Ragno, C. Vetuschi, A. Risoli and G. Ioele, Application of a classical least-squares regression method to the assay of 1, 4-dihydropyridine antihypertensives and their photoproducts, Talanta, 2003, 59, 375–382 CrossRef CAS
. - S. Moradi, M. A. Zolfigol, M. Zarei, D. A. Alonso and A. Khoshnood, An efficient catalytic method for the synthesis of pyrido[2,3-d]pyrimidines as biologically drug candidates by using novel magnetic nanoparticles as a reusable catalyst, Appl. Organomet. Chem., 2018, 32, 4043–4061 CrossRef
. - F. Jalili, M. Zarei, M. A. Zolfigol, S. Rostamnia and A. R. Moosavi-Zare, SBA-15/PrN(CH2PO3H2)2 as a novel and efficient mesoporous solid acid catalyst with phosphorous acid tags and its application on the synthesis of new pyrimido[4,5-b]quinolones and pyrido[2,3-d]pyrimidines via anomeric based oxidation, Microporous Mesoporous Mater., 2020, 294, 109865 CrossRef
. - W. Yu and C. Li, Regioselective one-pot C–N coupling of substituted naphthoquinones: selective intramolecular ring fusion of sulfonamides, Tetrahedron, 2014, 70, 459–464 CrossRef CAS
. - J. Tyleckova, R. Hrabakova, K. Mairychova, P. Halada, L. Radova, P. Dzubak, M. Hajduch, S. J. Gadher and H. Kovarova, Cancer cell response to anthracyclines effects: mysteries of the hidden proteins associated with these drugs, Int. J. Mol. Sci., 2012, 13, 15536–15564 CrossRef CAS PubMed
. - S. ElKalyoubi and E. Fayed, Synthesis and evaluation of antitumour activities of novel fused tri-and tetracyclic uracil derivatives, J. Chem. Res., 2016, 40, 771–777 CrossRef
. - I. Nizhenkovska, Standardization of citrullus colocynthis (L.) Shrad.
fruitsdry extract for futher study of its antidiabetic activity, Am. J. Clin. Exp. Immunol., 2015, 3, 162 CAS
. - T. Mirsaev, Experimental study of hepatoprotective activity of hydroxymethyluracil, Bull. Exp. Biol. Med., 2007, 143, 575–576 CrossRef CAS PubMed
. - M. Eltze, Investigations on the mode of action of a new antihypertensive drug, urapidil, in the isolated rat vas deferens, Eur. J. Pharmacol., 1979, 59, 1–9 CrossRef CAS PubMed
. - J. I. Bardagi and R. A. Rossi, Advances in the synthesis of 5-and 6-substituted uracil derivatives, Org. Prep. Proced. Int., 2009, 41, 479–514 CrossRef CAS
. - A. Mai, D. Rotili, S. Massa, G. Brosch, G. Simonetti, C. Passariello and A. T. Palamara, Discovery of uracil-based histone deacetylase inhibitors able to reduce acquired antifungal resistance and trailing growth in candida albicans, Bioorg. Med. Chem. Lett., 2007, 17, 1221–1225 CrossRef CAS PubMed
. - T. Tomašić and L. Peterlin Mašič, Rhodanine as a scaffold in drug discovery: a critical review of its biological activities and mechanisms of target modulation, Expert Opin. Drug Discovery, 2012, 7, 549–560 CrossRef
. - I. Yousefi, M. Pakravan, H. Rahimi, A. Bahador, Z. Farshadzadeh and I. Haririan, An investigation of electro spun Henna leaves extract-loaded chitosan based nanofibrous mats for skin tissue engineering, Mater. Sci. Eng., C, 2017, 75, 433–444 CrossRef CAS PubMed
. -
(a) M. A. Zolfigol, A. Khazaei, S. Alaie and S. Baghery, Synthesis of tricyanomethanesulfonic acid as a novel nanostructured and recyclable solid acid: application at the synthesis of biological henna-based chromenes, Can. J. Chem., 2017, 95, 560–570 CrossRef CAS
;
(b) M. Zarei, M. A. Zolfigol, A. R. Moosavi-Zare and E. Noroozizadeh, Trityl bromide versus nano-magnetic catalyst in the synthesis of henna-based xanthenes and bis-coumarins, J. Iran. Chem. Soc., 2017, 14, 2187–2198 CrossRef CAS
;
(c) M. Yarie, M. A. Zolfigol, S. Babaee, S. Baghery, D. A. Alonso and A. Khoshnood, Catalytic application of a nano-molten salt catalyst in the synthesis of biological naphthoquinone-based compounds, Res. Chem. Intermed., 2018, 44, 2839–2852 CrossRef CAS
;
(d) S. Moradi, M. A. Zolfigol, M. Zarei, D. A. Alonso and A. Khoshnood, Synthesis of a biological-based glycoluril with phosphorous acid tags as a new nanostructured catalyst: application for the synthesis of novel natural Henna-based compounds, ChemistrySelect, 2018, 3, 3042 CrossRef CAS
;
(e) M. Dashteh, S. Baghery, M. A. Zolfigol, A. Khazaei, S. Makhdoomi, M. Safaiee, J. Rakhtshah, Y. Bayat and A. Asgari, Design and synthesis of nickel tetra-2,3-pyridiniumporphyrazinato trinitromethanide as an influential catalyst and its application in the synthesis of 1,2,4-triazolo based compounds, J. Phys. Chem. Solids, 2022, 160, 110322 CrossRef CAS
;
(f) M. Dashteh, M. Safaiee, S. Baghery and M. A. Zolfigol, Application of cobalt phthalocyanine as a nanostructured catalyst in the synthesis of biological henna-based compounds, Appl. Organomet. Chem., 2019, 33, e4690 CrossRef
. - S. Waitschat, D. Fröhlich, H. Reinsch, H. Terraschke, K. Lomachenko, C. Lamberti, H. Kummer, T. Helling, M. Baumgartner and S. Henninger, Synthesis of M-UiO-66 (M= Zr, Ce or Hf) employing 2,5-pyridinedicarboxylic acid as a linker: defect chemistry, framework hydrophilization and sorption properties, Dalton Trans., 2018, 47, 1062–1070 RSC
. - S. Babaee, M. A. Zolfigol, M. Zarei and J. Zamanian, 1,10-Phenanthroline-Based Molten Salt as a Bifunctional Sulfonic Acid Catalyst: Application to the Synthesis of N-Heterocycle Compounds via Anomeric Based Oxidation, ChemistrySelect, 2018, 3, 8947–8954 CrossRef CAS
. - S. E. John, S. Gulati and N. Shankaraiah, Recent advances in multi-component reactions and their mechanistic insights: A triennium review, Org. Chem. Front., 2021, 8, 4237–4287 RSC
. - M. A. Zolfigol, Silica sulfuric acid/NaNO2 as a novel heterogeneous system for production of thionitrites and disulfides under mild conditions, Tetrahedron, 2001, 57, 9509–9511 CrossRef CAS
. - H. Sepehrmansourie, Silica sulfuric acid (SSA): as a multipurpose catalyst, Iran. J. Catal., 2020, 10, 175–179 CAS
. - M. Zarei, H. Sepehrmansourie, M. A. Zolfigol, R. Karamian and S. H. M. Farida, Novel nano-size and crab-like biological-based glycoluril with sulfonic acid tags as a reusable catalyst: its application to the synthesis of new mono-and bis-spiropyrans and their in vitro biological studies, New J. Chem., 2018, 42, 14308–14317 RSC
. - H. Sepehrmansourie, M. Zarei, M. A. Zolfigol, S. Babaee and S. Rostamnia, Application of novel nanomagnetic metal–organic frameworks as a catalyst for the synthesis of new pyridines and 1,4-dihydropyridines via a cooperative vinylogous anomeric based oxidation, Sci. Rep., 2021, 11, 1–15 CrossRef PubMed
. - M. M. Rasooll, M. Zarei, M. A. Zolfigol, H. Sepehrmansourie, A. Omidi, M. Hasani and Y. Gu, Novel nano-architectured carbon quantum dots (CQDs) with phosphorous acid tags as an efficient catalyst for the synthesis of multisubstituted 4H-pyran with indole moieties under mild conditions, RSC Adv., 2021, 11, 25995–26007 RSC
. - S. Kalhor, M. Zarei, H. Sepehrmansourie, M. A. Zolfigol, H. Shi, J. Wang, J. Arjomandi, M. Hasani and R. Schirhagl, Novel uric acid-based nano organocatalyst with phosphorous acid tags: application for synthesis of new biologically-interest pyridines with indole moieties via a cooperative vinylogous anomeric based oxidation, Mol. Catal., 2021, 507, 111549 CrossRef CAS
.
Footnote |
† Electronic supplementary information (ESI) available. See DOI: 10.1039/d1ra08710j |
|
This journal is © The Royal Society of Chemistry 2022 |
Click here to see how this site uses Cookies. View our privacy policy here.