DOI:
10.1039/D2RA00380E
(Paper)
RSC Adv., 2022,
12, 14154-14166
An innovative electrochemical immuno-platform towards ultra-sensitive monitoring of 2-arachidonoyl glycerol in samples from rats with sleep deprivation: bioanalysis of endogenous cannabinoids using biosensor technology†
Received
18th January 2022
, Accepted 3rd May 2022
First published on 11th May 2022
Abstract
The endocannabinoid system (ECS) is a complex of neurotransmitters in the central nervous system and plays a key role in regulating cognitive and physiological processes. 2-Arachidonoylglycerol (2-AG) is one of the imperative endocannabinoids that play key roles in the central nervous system. It acts as a signaling lipid and activates the cannabinoid CB1 receptor. In addition, 2-AG is involved in a variety of physiological functions such as energy balance, emotion, pain sensation, cognition, and neuroinflammation. So, rapid and specific diagnosis of 2-AG is of great importance in medical neuroscience. The development of new methods in this area has been one of the most important research areas in recent years. Herein, an innovative immunosensor is developed for quantification of 2-AG. For this means, gold nanostars (GNS) were synthesized and conjugated with a specific biotinylated antibody against 2-AG. The resultant bioconjugate, a bioreceptor with GNS, was immobilized on the surface of a gold electrode and used for the detection of the antigen based on the immunocomplex formation followed by analysis using different electrochemical techniques. For the first time, 2-AG protein was measured with an excellent linear range of 0.48–1 ng mL−1 and lower limit of quantification of 0.48 ng L−1 by the electroanalysis method. The engineered immunosensor showed high sensitivity and specificity in the presence of interfering antigens, proving its utility in neurological disorder detection. This immunosensor is the first sandwich type immunoassay for the detection of 2-AG in real samples and the first innovation of designing a novel sandwich type immunosensor for this analyte. Also, excellent analytical results are other advantages of this biosensor for the detection of 2-AG in human plasma samples and serum samples of rats under sleep deprivation. So, this is the first report of an immunosensor of 2-AG using a sandwich type immunosensor.
1. Introduction
The endocannabinoid system (ECS) is a complex of neurotransmitters in the central nervous system and plays a key role in regulating cognitive and physiological processes. It is one of the most important physiological systems involved in organizing and maintaining human health and its subsets include endogenous cannabinoids, endocannabinoid receptors and enzymes responsible for synthesis and degradation.1,2 The ECS and its receptors are found in the brain, organs, tissues, glands and immune cells and throughout the body. By understanding this system, one can discover a mechanism that can link brain activity and physical health status and disease.2 Other important and essential roles of ECs are in physiological processes including neuroprotection,3 pregnancy,4 stress and anxiety, mood and memory, pain sensation, fertility, and appetite,5 neuroinflammation6 and energy balance.7 It is also involved in the pathophysiology of many diseases and brain disorders such as attention-deficit hyperactivity disorder8 and spinal cord injury.9 There are three types of cannabinoids: (I) cannabinoids are produced by the body itself such as anandamide or arachidonyl ethanolamide (AEA) and di-arachidonoyl glycerol (2-AG); (II) phytocannabinoids (cannabinoids that occur naturally in the cannabis plant) such as Δ9-tetrahydrocannabinol (Δ9-THC) and cannabinol,10 and (III) man-made cannabinoids that are hallucinogenic chemicals.11 The 2-AG is a signaling lipid that has an important role in regulating neurotransmitter release within the central nervous system (CNS). In fact, the main activator of the CB1 receptor is endocannabinoid 2-AG.12 It is the agonist of CB1 and CB2 receptors which modulated by Δ9-THC, the main psychoactive substance of cannabis sativa,13 if used. The CB1 receptor is expressed throughout the body, but is widely present in the CNS, where G protein-coupled receptor is most expressed,12 while CB2 receptor appears to be expressed only on the basis of various injuries in the CNS and is associated with the immune system.2 It is proven that 2-AG is synthesized “on demand” and relatively high levels in the central nervous system, with cannabinoid neuro-modulatory effects.14 A study about sleep deprivation reported that there is a considerable relation between effect of activation of the ECS and the effect of sleep deprivation. Sleep deprivation stimulates the EC system, especially 2-AG.15 Besides the availability of competitive enzyme linked immunosorbent (ELISA) assay from various companies, to date, most of the researchers have used high performance liquid chromatography (HPLC),16–18 ultra-high performance liquid chromatography tandem mass spectrometry (UHPLC-MS/MS) (UHPLC-MS/MS),19,20 LC-MS/MS,21 liquid chromatography electrospray ionization-mass spectrometry (LC-ESI-MS)22,23 for identifying and monitoring 2-AG (Table 1).28–32 Although the conventional methods have some advantages such as considerable sensitivity and specificity in the identification of 2-AG but they suffer from some disadvantages, for example time-consuming procedures, necessity for advanced equipment and experts. Since 2-AG is susceptible and vulnerable against enzymatic degradations, in order to its detection it is essential to develop novel rapid methods. Up to now, other strategies have been developed to remedy the drawbacks of the routine methods. Among them, biosensors are analytical devices that benefit from numerous advantages including simplicity, cost effectiveness, sensitivity and high specificity. Recently immense studies have been accomplished to develop biosensors for distinguishing biomarkers. Moreover, investigators need cost effective detection tools for medical uses.24 Researchers mostly employ these methods in medicine for prediagnosis and pretreatment of diseases and different illnesses. Meanwhile, nanoparticles are being used in the electrochemical immunosensors for modifying of the transducer surfaces, forming nano-structural surfaces with better electrochemical response coming either from the enhancement of the electron transfer rate or the improved performance of biomolecules immobilization.25–27 Due to electrochemically inactive nature of 2-AG, we have recently used ferricyanide/ferrocyanide as a highly sensitive electrochemical redox couple along with a gold electrode modified by gold nano-flowers to develop an electrochemical immune-platform for sensitive detection of 2-AG. Herein, we report an ultra-sensitive immunosensor to identify endocannabinoids' 2-AG in human plasma and rat serum samples using gold nanostars conjugated antibodies immobilized on gold electrode beside the ferricyanide/ferrocyanide system. In this research, gold nano stars (GNS) were synthesized and characterized by field emission scanning electron microscopy (FE-SEM), dynamic light scattering (DLS) and zeta potential analysis and then conjugated with a biotinylated antibody against 2-AG (analyte). GNSs were used to expand the desired surface area for easily locating nanocomposite on it. Bioconjugated GNS composite was immobilized on the surface of a gold electrode and used for detection of antigen based on the immune-complex formation. In addition, we utilized sensitive electrochemical techniques including differential pulse voltammetry (DPV) and square wave voltammetry (SWV) to quantify target concentrations based on the increase in the current of redox couple by increasing the concentration of target. Based on obtained analytical results in real sample, the created immunosensor might be an appropriate platform for the monitoring of 2-AG in immunomodulatory activity and neuroprotection. Also, this study showed that sleep deprivation activates the endocannabinoid system and thus increases 2-AG in the body fluids.
Table 1 Different methods for detection of 2-AG
Method |
Advantages |
Disadvantages |
Ref. |
HPLC |
Efficiency and relatively accuracy |
Cost and complexity |
28 |
UHPLC-MS/MS |
Time consuming, expensive |
29 |
LC-MS/MS |
Requires advanced tools |
30 |
LC-ESI-MS |
Expensive, need for an expert |
31 |
ELISA |
Detect antigens at the picto-gram level in a specific manner |
Insufficient level of sensitivity in bio-recognition |
32 |
Immunoassay/immunosensor |
Easley and fast operation |
Low repeatability |
24 |
No need for advanced tools |
Sensitivity and specificity |
Easley and fast operation |
This work |
No need for advanced tools |
High sensitivity and specificity |
The engineered immunosensor showed high sensitivity and specificity in the presence of interfering antigens, proving its utility in neurological disorders detection. This immunosensor is the first sandwich type immunoassay for the detection of 2-AG in real samples. So, the first innovation of designing of the novel sandwich type immunosensor for this analyte. Also, excellent analytical results are other advantages of this biosensor for the detection of 2-AG in human plasma samples and serum samples of rat under sleep deprivation. So, this is the first report in the immunosensor of 2-AG by sandwich type immunosensor.
2. Materials and methods
2.1. Chemicals and reagents
A 2-AG kit consisted of biotinylated antibodies and various concentrations of the antigen was purchased from ZellBio GmbH (Germany) by Padgin Teb Company. K4Fe(CN)6, K3Fe(CN)6 and KCl were obtained from Merck. Hydroxysuccinimide (NHS)/N-1-ethyl-3-(3-dimethylaminopropyl)carbodiimide (EDC), hydrogen tetrachloroaurate(III) hydrate (HAuCl4·3H2O), sodium citrate tribasic (Na3C6H5O7), silver nitrate (AgNO3), sodium borohydride (NaBH4), ascorbic acid and cetyltrimethylammonium bromide (CTAB) were all obtained from Sigma-Aldrich (Ontario, Canada). The fresh frozen plasma samples were gained from the Iranian Blood Transfusion Research Center (Tabriz, Iran). Sample donors signed a written cosecant form approved by the ethics committee of Tabriz University of Medical Science (IR.NIMAD.REC.1400.059).
2.2. Apparatus
The electrochemical measurements were assessed by a standard three electrode cell (from Metrohm), containing a Pt wire as a counter electrode, gold disk as a working electrode (d = 2 mm), and Ag/AgCl-saturated KCl as a reference electrode. The assembly was powered by PalmSens electrochemical system with PSTrance 5.3 software as a running program (PS4.F1.05, Palm instruments, Utrecht, The Netherlands). The used AC voltage amplitude was 10 mV, and the equilibrium time was 5 s. In this research, cyclic voltammetry (CV) technique was utilized for the cleaning of the gold electrode and also for the primary analysis of electrode interface where Tequilibration: 0 s, Ebegin: −1.0 V, Evertex1: 1.0 V, Evertex2: −1.0 V, Estep: 0.01 V, scan rate: 0.1 V s−1. The other two techniques used for quantification that include square wave voltammetry (SWV) with Tequilibration: 2 s, Ebegin: −1.0 V, Eend: 1.0 V, Estep: 0.005 V, amplitude: 0.02 V, frequency: 10 Hz, and differential pulse voltammetry (DPV) with Tequilibration: 2 s, Ebegin: −1.0 V, Eend: 1.0 V, Estep: 0.05 V, Epulse: 0.1 V, tpulse: 0.01 s, scan rate: 0.1 V s−1. For the dynamic light scattering (DLS) analysis and zeta potential evaluation, Zetasizer Ver. 7.11 (Malvern Instruments Ltd, MAL1032660, England) was used. These apparatuses have three main segments: laser, sample and light indicator. UV-vis spectrophotometer achieved by Shimadzu UV-1800 UV-vis spectrophotometer with a resolution of 1 nm. Verification of the surface immobilization and size examination of GNS were demonstrated further via the atomic force microscope (AFM) analysis by Nanosurf (AG Gräubernstrasse 124410 Liestal Switzerland) in tapping mode. Field-emission scanning electron microscopy (FE-SEM, Hitachi-Su8020, Czech) was used for analyzing morphology of GNS and the electrode surface, and the chemical elements on the electrode surface after modification were analyzed using energy-dispersive spectroscopy (EDS).
2.3. Synthesis of gold nano-stars
2.3.1. Synthesis of Ag-seeds. In order to synthesize Ag-seeds, 156 μL of 0.0163 M AgNO3 solution was added to 10 mL of deionized water, then, 250 μL of 0.055 M sodium citrate tribasic solution was added to it and stirred completely (solution A). In the next step, 400 μL of 0.04 M sodium borohydride solution was added to the mixture and stirred. Subsequently, the solution color changed to yellow. After 5 minutes of stirring, the Ag seeds were obtained and stored at room temperature in a foil-covered vial for 2 h to let hydrogen to egress.33
2.3.2. Gold nano-stars (GNS) synthesis. To synthesize GNS, 0.364 g of cetyltrimethylammonium bromide (CTAB, C19H42BrN) was dissolved in 20 mL deionized water by heating at 30 °C (solution B). Then, 60 μL of 0.0163 M AgNO3 solution was added to it. After 1 minute stirring, 100 μL of 50 mM gold chloride was added to it and stirred for another minute. By adding gold, the solution color turned to yellow. Then, 100 μL of 0.08 M ascorbic acid solution was added to the mixture, which resulted in discoloring of the solution. After 20 seconds of stirring, 50 μL of the synthesized silver seeds (solution A) was added to the solution and stirred for next 15 minutes, making a color change from blue to brownish. The color of solution turned brown after 1 hour of storage in dark place. After 24 hours of storage at room temperature in dark place, crystals from CTAB were observable inside the solution. In order to separate the crystals, the solution was primarily stirred for 2 minutes at 30 °C. Then, it was centrifuged for 5 minutes at 2500 rpm and 12 minutes at 3000 rpm. The colorless supernatant that contains surfactant was isolated. The GNS was then dispersed in deionized water and stored within a dark bottle at 4 °C.33
2.3.3. Gold electrode preparation and cleaning approach. In this research, a very common electrochemical cleaning technique applied for activating and polishing gold electrode surfaces, by cycling the electrode potential from −400 to 1400 mV vs. Ag/AgCl at a scan rate of 100 mV s−1 in a 50 mM sulfuric acid solution until the CVs become stable (about 12 cycles).34,35
2.3.4. Preparation of 2-AG immunosensor. Firstly, the conventional and robust EDC/NHS approach was used for conjugation of GNS to antibodies.36 For this means, 5 μL human 2-AG biotinylated antibody was activated by 10 μL of EDC/NHS (in 1
:
2 ratio). Then, 15 μL of synthesized GNS was added to it and mixed and let to remain at 4 °C for 30 min, then, it was centrifuged and re-suspended in deionized water. After carefully cleaning and washing of gold electrodes as mentioned in Section 2.3.2, 5 μL of GNS conjugated with antibodies were dropped on gold electrode surface and the modified electrode was sustained at 4 °C for 4 h to complete immobilization process. In the next step to eliminate possible contaminations, modified electrodes were washed with washing solution. For blocking step, 5 μL of 1% bovine serum albumin (BSA) solution dropped on the modified electrode surface and incubated for 30 minutes. Thereafter, 2-AG antigen was dropped on the electrode surface and incubated for 2 h in refrigerator (Scheme 1).
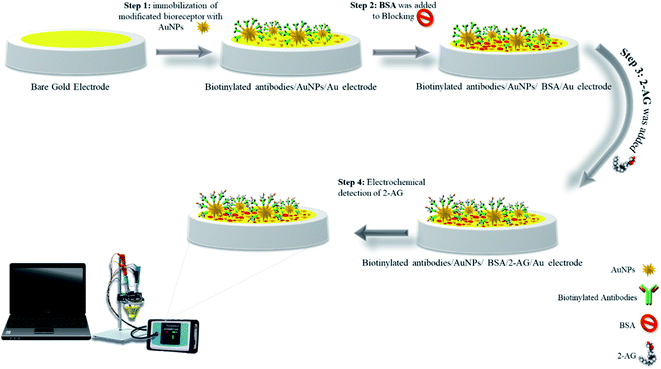 |
| Scheme 1 Schematic illustration of developed immunosensor for detection of 2-AG. | |
3. Results and discussion
3.1. Synthesis and characterization studies
GNPs has been synthesized and characterized according to our previous report.33
3.2. Electrochemical study of synthesized nanocomposite
The SWV and DPV techniques were used to investigate the electrochemical properties of the synthesized nanocomposite (bulk, modified) (Fig. 1). All three techniques were noted in the presence of K4Fe(CN)6/K3Fe(CN)6 solution as electrochemical mediator at the scan rate of 100 mV s−1. First, the SWV of bare electrode was recorded, which it's Ip and Ep values are 11 μA and 0.23 V, respectively (Fig. 1A). Next, the SWVs of the modified electrode by GNS-antibody nano-bioconjugate were noted, where Ip and Ep reached to 20 μA and 0.18 V, respectively. The enhancement in the conductivity and surface area of electrode by immobilizing GNS-antibody conjugates resulted in an increase in peak current and a bit reduction in redox potential. Finally, after the formation of antibody-antigen immunocomplex, peak current was dropped to 16 μA and potential was even reduced to 0.15 V. According to the obtained results, the modified electrode shows different electrochemical behaviors at each step.
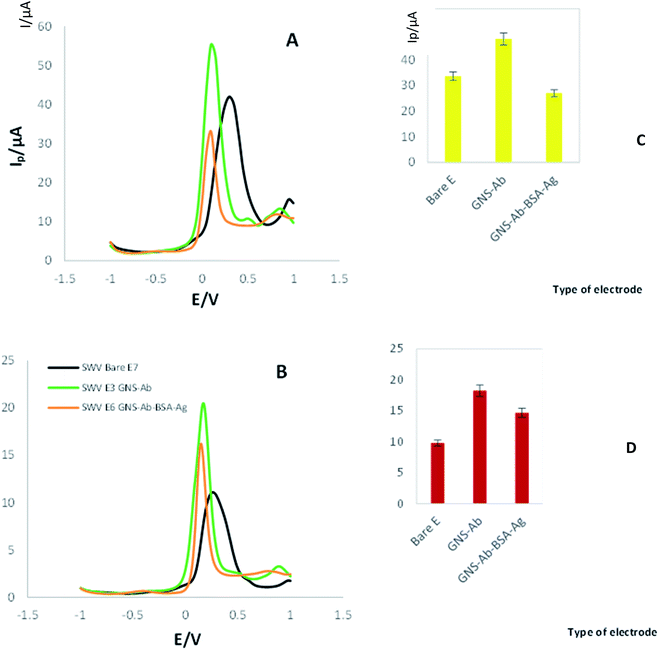 |
| Fig. 1 (A–D) DPV and SWVs of immunosensor fabrication steps (Au electrode, Au-(GNS-biotinylated Ab), Au-(GNS-biotinylated Ab)-2-AG). Information of SWV; Ebegin = −1.0 V, Eend = 1.0 V, Estep = 0.005 V, amplitude = 0.02 V, frequency = 10 Hz. Information of DPV; Ebegin: −1.0 V, Eend: 1.0 V, Estep: 0.05 V, Epulse: 0.1 V, Tpulse: 0.01 s, scan rate: 0.1 V s−1. Supporting electrolyte; K4Fe(CN)6/K3Fe(CN)6 containing KCl. (E and F) Histogram of peak current versus type of electrode by DPV and SWV, respectively. (RSD = 1.84%, n = 3). | |
DPVs of bare and modified electrodes were recorded too. According to Fig. 1B, the Ip and Ep of bare electrode is 41 μA and 0.3 V, respectively. Then AuNPs-biotinylated Ab modified electrode's DPV was recorded, and the results showed there is an increasing in the current intensity with Ip = 55 μA and reduction in Ep = 0.099 V. Finally, after the addition of 2-AG antigen on modified electrode with GNS-antibody, peak current was appeared to be 33 μA with a redox potential located at 0.099 V.
3.3. Morphological study of developed immunosensor
For investigation of Au-GNSs-biotinylated Ab, FE-SEM technique was used appropriately in different magnifications. Today, FE-SEM has been widely used for the imagining of applied nanocomposite in biosensors. FESEM was used in the current work for the morphological study of synthesized AuNPs (Fig. 2) and conjugated AuNPs with Ab and Ag. As seen in the Fig. 3, biotinylated Ab have changed the star-shaped morphology of AuNPs.
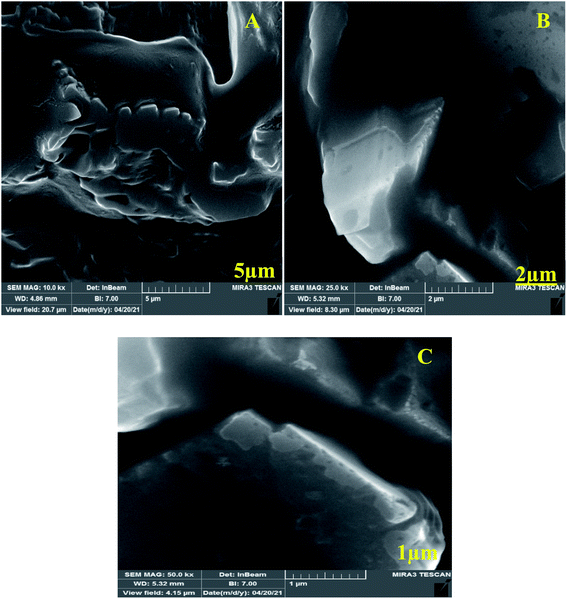 |
| Fig. 2 (A–C) Field emission scanning electron microscopy (FE-SEM) images of Au-GNSs-biotinylated antibody in different magnification. | |
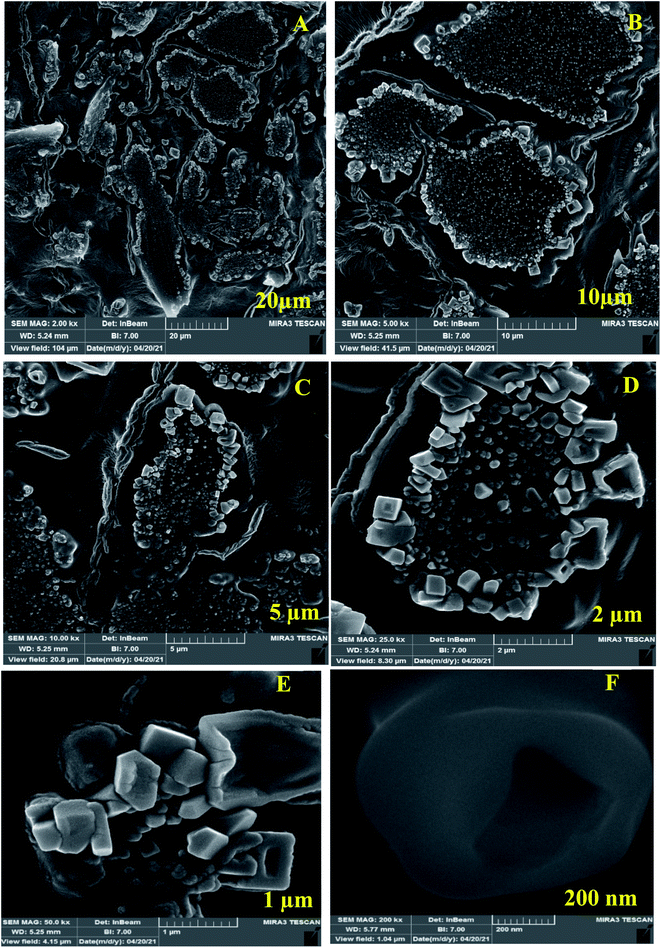 |
| Fig. 3 (A–F) FE-SEM images of Au-GNSs-biotinylated Ab-antigen (2-AG) in different magnification. | |
Also, FE-SEM, was used for determination of Au-GNSs-biotinylated Ab-antigen (2-AG) in different magnifications. As it is appeared, the conjugated 2-AG antigen with gold nano-stars-Ab has exhibited different geometric and mostly square shapes (Fig. 3).
3.4. Electroanalytical behavior
Toward investigation of analytical performance of developed immunosensor, DPV of electrode in the presence of different concentration of 2-AG (0.0312, 0.0625, 0.125, 0.5, and 0.25 ng L−1) were recorded and the respective signals were measured.
According to Fig. 4A, an inverse and meaningful relation between Ip and the concentration of 2-AG was obtained. With increasing concentration of 2-AG, the electrode showed gradual decreasing in DPVs' peak currents. This means that the developed immunosensor has detected down to 0.0312 ng L−1 of target very well.
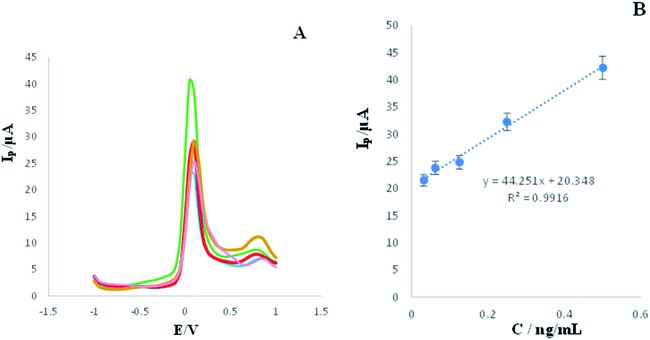 |
| Fig. 4 (A) DPVs of engineered immunosensor in the presence of high concentrations (0.5, 0.25, 0.125, 0.0625, and 0.0312 ng L−1) of 2-AG, measuring conditions is similar to legend of Fig. 1. (RSD = 1.26%, n = 3). (B) Calibration Curve. | |
Similarly, DPV technique was used for determination of 2-AG in low concentrations.
As shown in Fig. 5A, increment of concentration lead to enhancement of peak current. Therefore, there is a linear relation between concentration and the current decreased related to the concentration of 0.0156 ng L−1 is 30.4 μA, while the current intensity related to 0.00097 ng L−1 is 51.6 μA. This means that the engineered immunosensor is capable of detecting 2-AG in very low concentrations properly.
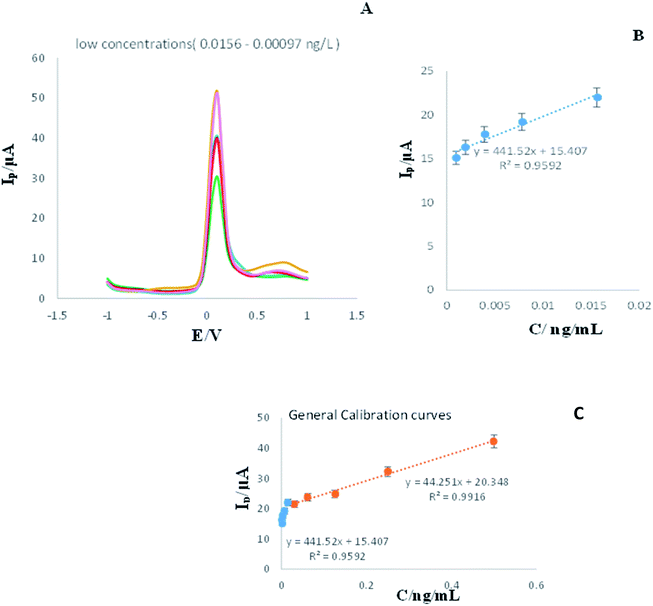 |
| Fig. 5 (A and B) DPVs of engineered immunosensor in the presence of low concentrations of analyte (0.0156, 0.00781, 0.0039, 0.00195, and 0.00097 ng L−1), measuring conditions is similar to legend of Fig. 1. (RSD = 2.4%, n = 3). (C) General calibration curve for low and high concentration of analyte. | |
3.5. Real samples analysis
In this study various concentration (1, 0.5, 0.25, 0.0625, and 0.0312 ng L) of antigen was added to plasma samples and analyzed by the developed immunosensor using DPV and SWV techniques.
Based on the results obtained in this study (Fig. 6C and D), a suitable linearity for different concentrations was recorded and is acceptable.
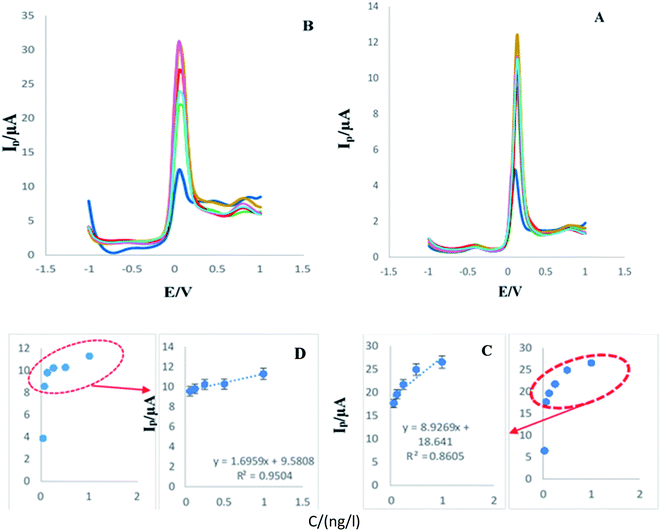 |
| Fig. 6 (A and B) DPVs and SWVs of engineered immunosensor in the presence of various concentration (1, 0.5, 0.25, 0.0625, and 0.0312 ng L) of analyte in human plasma samples. (C and D) Calibration curves ((C) RSD = 1.49%, n = 5) ((D) RSD = 0.57% n = 3). Experimental condition is similar to Fig. 1. (RSD = 2.05%, n = 3). | |
In the following, another series of different concentrations including (0.0156, 0.00781, 0.0039, 0.00195, and 0.000976 ng L−1) were prepared. As in the previous step, DPV and SWV techniques were used.
After assessment of the analytical study in the K4Fe(CN)6/K3Fe(CN)6 solution, similar conditions, techniques and concentrations were regulated for the analytical study in presence of human plasma samples. SWVs and DPVs of the immunosensor in the presence of 2-AG with different concentrations were recorded and same outcomes were achieved. According to Fig. 6 and 7, a linear relation between current intensity and the concentration of 2-AG, even in presence of human plasma, was obtained. These similar results confirmed that the created immunosensor is very accurate and relatively flawless. In addition, in relation to calibration curve, based on the results obtained in this study (Fig. 6C and D) a suitable linearity for different concentrations was recorded.
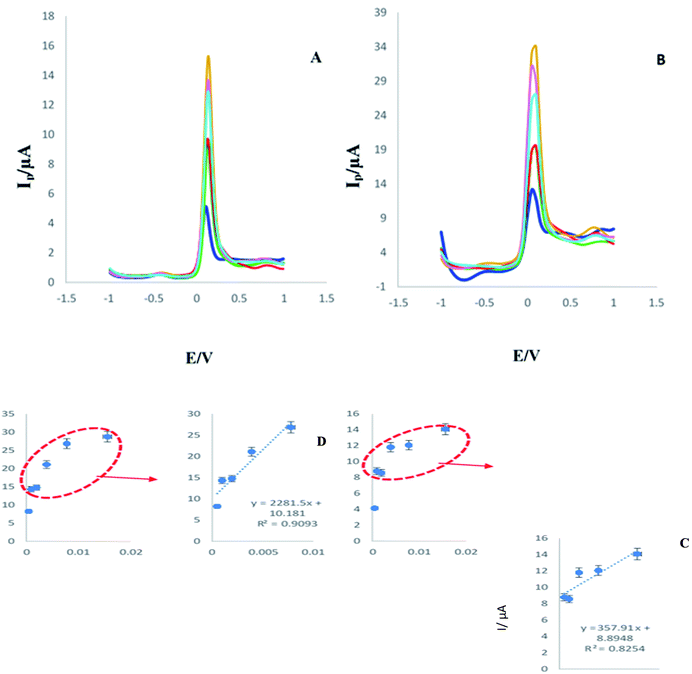 |
| Fig. 7 (A and B) DPV and SWV of engineered immunosensor in the presence of various concentration (0.0156, 0.00781, 0.0039, 0.00195, 0.000976, 0.000488, ng L) of analyte in human plasma samples. (C and D) Calibration curves ((C) RSD = 1.89%, n = 5) ((D) RSD = 3.68%, n = 5). Experimental condition is similar to Fig. 1. | |
3.6. Analytical comparison
The analytical results obtained in this study are evaluated in comparison with other methods. In spite of the simple and inexpensive structure of the created platform, it has suitable sensitivity and specificity. As explained in the previous sections, routine methods of 2-AG detection are difficult and require advanced and expensive tools. On the other hand, interpreting the results of routine methods requires experienced people, while the results obtained in methods based on biosensors are simple and fast. Table 2 summarizes the common diagnostic methods for arachidonic glycerol from a comparative perspective.37–44
Table 2 Analytical figure of merits of different methods for detection of 2-AG
Methods |
Linear rang |
LOD |
Advantages and limitation |
Ref. |
HPLC |
0/05–50 ng mL |
50 pg mL−1 |
Applicable for real samples and in vivo |
37 |
3.37–0.73 pmol g−1 |
15 fmol |
Applicable for real samples and in vivo, hard to work |
38 |
Liquid biosensor |
100–0.001 μM |
— |
Rapid, efficient, user friendly and inexpensive quantitation |
39 |
ESI-MS/MS |
— |
2 pg mL−1 |
Used for urine samples, hard to work |
40 |
Gas chromatography/MS |
5–500 ng mL−1 |
1 ng mL−1 |
Used for salvia samples, acceptable analytical results |
41 |
Biosensor |
100 pg mL−1–100 ng L−1 |
100 pg mL−1 |
Rapid identification, portable, usable for salvia samples |
42 |
25–1.26 ng L−1 |
1.5 μM |
Acceptable sensitivity and selectivity |
43 |
0.18–0.02 ng L−1 |
0.32 ng mL−1 |
Low-cost, good linearity and stability |
44 |
Immunosensor |
0.00024–0.0078 ng L−1 |
0.00024 ng L−1 |
Good sensitivity, wide linearity, acceptable selectivity |
24 |
0.00048–1 ng L−1 |
0.00048 ng L−1 |
Acceptable sensitivity and stability, wide linearity, usable for real samples |
This work |
Biosensors are more up-to-date devices that have been welcomed by researchers. Biosensors are simple, inexpensive tools that are highly sensitive and specific to some of conventional methods. The analytical features of the prepared platform in this study are not only better than routine methods but also more suitable than the previous biosensors. In general, the analytical results of biosensors are more appropriate than the old/traditional methods.
3.7. Application of developed sensor for detection of 2-AG in serum samples of rat before and after SD (sleep deprivation)
Repeatability refers to the agreement between successive measurements of the same sample in the same conditions.45 At this stage, the electrochemical behavior of the electrodes modified with Au/GNS-Ab-BSA-SD (sleep deprivation rat serum samples) and Au/GNS-Ab-BSA-C (rat control serum) under the same conditions were evaluated using the CV technique. The same behavior was really expected from different electrodes.
To test repeatability of the above immunosensor with real samples, CV technique was applied with Ebegin = −1.0 V and scan rate = 0.1 V s−1. Real rat serum samples immobilized on AuNPs-Ab modified electrode instead of the antigen inside the purchased kit. The samples used in this research include: blood serum of sleep deprivation group and control group. As shown in the Fig. S1 (see ESI†), the engineered immunosensor detected the 2-AG in the serum of the sleep deprivation group very well. Accordingly, the peak current intensity has reached to 15 μA and Ep is 0.2 V. However, in the control group, the peak current intensity has increased to 24 μA and Ep is 0.34, which means that the desired substance has not been identified in this group. These conditions appear to be almost the same on all electrodes, which indicates excellent repeatability.
3.8. Evaluation of the stability
3.8.1. Inter-day stability. Evaluation of the stability of the designed biosensors is one of the important parts in the development of biosensors. To investigate long-term surface stability of engineered biosensor during the first, second and third days was evaluated. In this part, SWV, CV, and DPV techniques were applied.As it is clear in the Fig. S2 (see ESI†), the stability of the biosensor created during the first day is appropriate, but on the second and third days it shows a significant decrease. The decreasing trend of stability in three consecutive days is quite evident in the all used techniques. First day the current intensity of CV, SWV, and DPV was 20 μA, 21 μA and 54 μA, respectively. Also, in the second day, Ip quantity related to each technique decreased to 4 μA. These quantities at third day decreased again to 2.9, 3.2 and 8.2 μA, related to CV, SWV and DPV, respectively. Perhaps the most important limitation of this immunosensor is its relatively low stability. So, we honestly would like to point out that this failure of engineered immunosensor and hope after publication of this report, researchers suggest new immunosensors for this target (2-AG).
3.8.2. Intra-day stability. After determination of the inter-day stability, the intra-day stability was evaluated (Fig. S3 (see ESI†)). For this purpose, the stabilities of biosensors during different hours after preparation including 1, 2, 3 and 4 were evaluated. According to CV results, the generated system showed improper stability after 4 hours; and so, this electrode should be used in early time after preparation.
3.9. Reproducibility of immunosensor
Repeatability discusses to the agreement between successive measurements of the same sample while reproducibility is used to describe the closeness of agreement between results (signals) obtained with the same method under different conditions.45 For evaluation of reproducibility CV technique was applied effectively. The reproducibility of the immunosensor was measured by individually manufacturing five modified electrodes under the same investigational situation, and using them for the detection of 1 ng L−1 of 2-AG. As can be seen in the Fig. S4 (see ESI†), the current intensity of each 5 electrodes is approximately on the same statistic (Ip = 21, 19, 18.6, 18.4, and 18.2 μA). These results confirm the satisfactory reproducibility and accuracy of the immunosensor.
3.10. Specificity of immunosensor
The extremely specific sensing of 2-AG by means of the created immunosensor was studied. In this research, for the assessment of specificity, three different antigens, prostate-specific antigen (PSA), carcinoembryonic antigen (CEA) and alpha-synuclein antigen (SNCA) were applied in combination with 2-AG, and the SWV and DPV were recorded (Fig. S5 (see ESI†)). Pursuant to the achieved results, the created immunosensor presented good specificity for the detection of 2-AG in the existence of interfering biomarkers (PSA, CEA, SNCA).
4. Conclusion
This work aimed to establish a platform capable of detection of 2-AG as one of the most important biomarkers in neuroscience. In this study, in addition to electrochemical methods, AFM, and FE-SEM were used to analysis the nanostructures and conjugates to design the immunosensor efficiently. The created sensor has suitable analytical features including acceptable analytical results. Based on the results, the reproducibility (RSD = 2.11%) and repeatability (RSD = 2.45%) of the developed system is significant. The system designed in this study has a simple and low-cost structure and its development can be considered as a suitable alternative to routine and expensive methods such as HPLC. Also based on the performance of immuno-platform rat plasma samples, it's demonstrated that sleep deprivation significantly increases 2-AG in biofluids. As a result, this immunosensor is utilizable in diagnoses of depressive and sleep disorders. The engineered immunosensor showed high sensitivity and specificity in the presence of interfering antigens, proving its utility in neurological disorders detection. This immunosensor is the first sandwich type immunoassay for the detection of 2-AG in real samples. So, the first innovation of designing of the novel sandwich type immunosensor for this analyte. Also, excellent analytical results are other advantages of this biosensor for the detection of 2-AG in human plasma samples and serum samples of rat under sleep deprivation. So, this is the first report in the immunosensor of 2-AG by sandwich type immunosensor.
Conflicts of interest
The authors declare that there are not competing financial interests to influence the work reported in this paper.
Acknowledgements
Research reported in this publication was supported by Elite Researcher Grant Committee under award number [4000106] from the National Institutes for Medical Research Development (NIMAD), Tehran, Iran. [IR.NIMAD.REC.1400.059].
References
- H.-C. Lu and K. Mackie, An introduction to the endogenous cannabinoid system, Biol. Psychiatry, 2016, 79(7), 516–525 CrossRef CAS PubMed.
- B. E. Alger, Getting high on the endocannabinoid system, in, Cerebrum: the Dana Forum on Brain Science, Dana Foundation, 2013 Search PubMed.
- R. Mechoulam, M. Spatz and E. Shohami, Endocannabinoids and neuroprotection, Sci. Signaling, 2002, 2002(129), re5 CrossRef CAS PubMed.
- A. H. Taylor, A. A. Amoako, K. Bambang, T. Karasu, A. Gebeh, P. M. Lam, T. H. Marzcylo and J. C. Konje, Endocannabinoids and pregnancy, Clin. Chim. Acta, 2010, 411(13–14), 921–930 CrossRef CAS PubMed.
- M. N. Hill and B. B. Gorzalka, The endocannabinoid system and the treatment of mood and anxiety disorders, CNS Neurol. Disord.: Drug Targets, 2009, 8(6), 451–458 CrossRef CAS PubMed.
- D. K. Nomura, B. E. Morrison, J. L. Blankman, J. Z. Long, S. G. Kinsey, M. C. G. Marcondes, A. M. Ward, Y. K. Hahn, A. H. Lichtman and B. Conti, Endocannabinoid hydrolysis generates brain prostaglandins that promote neuroinflammation, Science, 2011, 334(6057), 809–813 CrossRef CAS PubMed.
- I. Matias and V. Di Marzo, Endocannabinoids and the control of energy balance, Trends Endocrinol. Metab., 2007, 18(1), 27–37 CrossRef CAS PubMed.
- A. Ahmadalipour, L. M. Fanid, N. Zeinalzadeh, M. Alizadeh, H. Vaezi, Z. H. Aydinlou and S. G. Noorazar, The first evidence of an association between a polymorphism in the endocannabinoid-degrading enzyme FAAH (FAAH rs2295633) with attention deficit hyperactivity disorder, Genomics, 2020, 112(2), 1330–1334 CrossRef CAS PubMed.
- M. Ghorbani, P. Shahabi, P. Karimi, H. Soltani-Zangbar, M. Morshedi, S. Bani, M. Jafarzadehgharehziaaddin, B. Sadeghzadeh-Oskouei and A. Ahmadalipour, Impacts of epidural electrical stimulation on Wnt signaling, FAAH, and BDNF following thoracic spinal cord injury in rat, J. Cell. Physiol., 2020, 235(12), 9795–9805 CrossRef CAS PubMed.
- V. Di Marzo, The endocannabinoidome as a substrate for noneuphoric phytocannabinoid action and gut microbiome dysfunction in neuropsychiatric disorders, Dialogues Clin. Neurosci., 2020, 22(3), 259 CrossRef PubMed.
- A. C. Howlett and S. Mukhopadhyay, Cellular signal transduction by anandamide and 2-arachidonoylglycerol, Chem. Phys. Lipids, 2000, 108(1–2), 53–70 CrossRef CAS PubMed.
- S. Zou and U. Kumar, Cannabinoid receptors and the endocannabinoid system: signaling and function in the central nervous system, Int. J. Mol. Sci., 2018, 19(3), 833 CrossRef PubMed.
- L. Ye, Z. Cao, W. Wang and N. Zhou, New insights in cannabinoid receptor structure and signaling, Curr. Mol. Pharmacol., 2019, 12(3), 239 CrossRef CAS PubMed.
- I. Katona and T. F. Freund, Multiple functions of endocannabinoid signaling in the brain, Annu. Rev. Neurosci., 2012, 35, 529–558 CrossRef CAS PubMed.
- E. C. Hanlon, E. Tasali, R. Leproult, K. L. Stuhr, E. Doncheck, H. De Wit, C. J. Hillard and E. Van Cauter, Sleep restriction enhances the daily rhythm of circulating levels of endocannabinoid 2-arachidonoylglycerol, Sleep, 2016, 39(3), 653–664 CrossRef PubMed.
- Y. Wang, Y. Liu, Y. Ito, T. Hashiguchi, I. Kitajima, M. Yamakuchi, H. Shimizu, S. Matsuo, H. Imaizumi and I. Maruyama, Simultaneous measurement of anandamide and 2-arachidonoylglycerol by polymyxin B-selective adsorption and subsequent high-performance liquid chromatography analysis: increase in endogenous cannabinoids in the sera of patients with endotoxic shock, Anal. Biochem., 2001, 294(1), 73–82 CrossRef CAS PubMed.
- A. Schmidt, K. Brune and B. Hinz, Determination of the endocannabinoid anandamide in human plasma by high-performance liquid chromatography, Biomed. Chromatogr., 2006, 20(4), 336–342 CrossRef CAS PubMed.
- R. B. Mounsey, S. Mustafa, L. Robinson, R. A. Ross, G. Riedel, R. G. Pertwee and P. Teismann, Increasing levels of the endocannabinoid 2-AG is neuroprotective in the 1-methyl-4-phenyl-1, 2, 3, 6-tetrahydropyridine mouse model of Parkinson's disease, Exp. Neurol., 2015, 273, 36–44 CrossRef CAS PubMed.
- I. D. Souza, L. W. Hantao and M. E. C. Queiroz, Polymeric ionic liquid open tubular capillary column for on-line in-tube SPME coupled with UHPLC-MS/MS to determine endocannabinoids in plasma samples, Anal. Chim. Acta, 2019, 1045, 108–116 CrossRef CAS PubMed.
- P. M. Lam, T. H. Marczylo, M. El-Talatini, M. Finney, V. Nallendran, A. H. Taylor and J. C. Konje, Ultra performance liquid chromatography tandem mass spectrometry method for the measurement of anandamide in human plasma, Anal. Biochem., 2008, 380(2), 195–201 CrossRef CAS PubMed.
- M. Bobrich, R. Schwarz, R. Ramer, P. Borchert and B. Hinz, A simple LC-MS/MS method for the simultaneous quantification of endocannabinoids in biological samples, J. Chromatogr. B: Anal. Technol. Biomed. Life Sci., 2020, 1161, 122371 CrossRef CAS PubMed.
- J. Musee and L. J. Marnett, Prostaglandin H synthase-2-catalyzed oxygenation of 2-arachidonoylglycerol is more sensitive to peroxide tone than oxygenation of arachidonic acid, J. Biol. Chem., 2012, 287(44), 37383–37394 CrossRef CAS PubMed.
- K. Nithipatikom, M. P. Endsley, A. W. Pfeiffer, J. R. Falck and W. B. Campbell, A novel activity of microsomal epoxide hydrolase: metabolism of the endocannabinoid 2-arachidonoylglycerol, J. Lipid Res., 2014, 55(10), 2093–2102 CrossRef CAS PubMed.
- A. Mobed, F. Kohansal, A. Ahmadalipour, M. Hasanzadeh and F. Zargari, Bioconjugation of 2-arachidonoyl glycerol (2-AG) biotinylated antibody with gold nano-flowers toward immunosensing of 2-AG in human plasma samples: A novel immuno-platform for the screening of immunomodulation and neuroprotection using biosensing, Anal. Methods, 2021, 13(3), 311–321 RSC.
- H. Chen, D. Tang, B. Zhang, B. Liu, Y. Cui and G. Chen, Electrochemical immunosensor for carcinoembryonic antigen based on nanosilver-coated magnetic beads and gold-graphene nanolabels, Talanta, 2012, 91, 95–102 CrossRef CAS PubMed.
- D. Tang, Y. Cui and G. Chen, Nanoparticle-based immunoassays in the biomedical field, Analyst, 2013, 138(4), 981–990 RSC.
- H. Ashrafi, A. Mobed, M. Hasanzadeh, P. Babaie, K. Ansarin and A. Jouyban, Monitoring of five benzodiazepines using a novel polymeric interface prepared by layer by layer strategy, Microchem. J., 2019, 146, 121–125 CrossRef CAS.
- R. Ozdurak, T. Şeker, P. Korkusuz and F. Korkusuz, Quantification of Anandamide and 2-Arachidonylglycerol in Plasma Samples: A Short, Non-toxic HPLC Method and Sample Storage, 2010 Search PubMed.
- C. Marchioni, I. D. de Souza, C. F. Grecco, J. A. Crippa, V. Tumas and M. E. C. Queiroz, A column switching ultrahigh-performance liquid chromatography-tandem mass spectrometry method to determine anandamide and 2-arachidonoylglycerol in plasma samples, Anal. Bioanal. Chem., 2017, 409(14), 3587–3596 CrossRef CAS PubMed.
- M. Y. Zhang, Y. Gao, J. Btesh, N. Kagan, E. Kerns, T. A. Samad and P. K. Chanda, Simultaneous determination of 2-arachidonoylglycerol, 1-arachidonoylglycerol and arachidonic acid in mouse brain tissue using liquid chromatography/tandem mass spectrometry, J. Mass Spectrom., 2010, 45(2), 167–177 CrossRef CAS PubMed.
- D. R. McDougle, A. Kambalyal, D. D. Meling and A. Das, Endocannabinoids anandamide and 2-arachidonoylglycerol are substrates for human CYP2J2 epoxygenase, J. Pharmacol. Exp. Ther., 2014, 351(3), 616–627 CrossRef PubMed.
- A. Feliu, L. Mestre, F. J. Carrillo-Salinas, V. W. Yong, M. Mecha and C. Guaza, 2-arachidonoylglycerol reduces chondroitin sulphate proteoglycan production by astrocytes and enhances oligodendrocyte differentiation under inhibitory conditions, Glia, 2020, 68(6), 1255–1273 CrossRef PubMed.
- F. Bahavarnia, A. Mobed, M. Hasanzadeh, A. Saadati, S. Hassanpour and A. Mokhtarzadeh, Bio-assay of Acintobacter baumannii using DNA conjugated with gold nano-star: A new platform for microorganism analysis, Enzyme Microb. Technol., 2020, 133, 109466 CrossRef PubMed.
- T. Green, Gold etching for microfabrication, Gold Bull., 2014, 47(3), 205–216 CrossRef CAS.
- L. M. Fischer, M. Tenje, A. R. Heiskanen, N. Masuda, J. Castillo, A. Bentien, J. Émneus, M. H. Jakobsen and A. Boisen, Gold cleaning methods for electrochemical detection applications, Microelectron. Eng., 2009, 86(4–6), 1282–1285 CrossRef CAS.
- M. Hasanzadeh, N. Shadjou, Y. Lin and M. de la Guardia, Nanomaterials for use in immunosensing of carcinoembryonic antigen (CEA): Recent advances, Trends Anal, Chem., 2017, 86, 185–205 CrossRef CAS.
- A. Weber, J. Ni, K.-H. J. Ling, A. Acheampong, D. D. S. Tang-Liu, R. Burk, B. F. Cravatt and D. Woodward, Formation of prostamides from anandamide in FAAH knockout mice analyzed by HPLC with tandem mass spectrometry, J. Lipid Res., 2004, 45(4), 757–763 CrossRef CAS PubMed.
- Y. Arai, T. Fukushima, M. Shirao, X. Yang and K. Imai, Sensitive determination of anandamide in rat brain utilizing a coupled-column HPLC with fluorimetric detection, Biomed. Chromatogr., 2000, 14(2), 118–124 CrossRef CAS PubMed.
- S. Grasso, M. Santonico, G. Pennazza, A. Zompanti, A. Piccoli, T. Bisogno and M. Maccarrone, BIONOTE as an Innovative Biosensor for Measuring Endocannabinoid Levels, Sensors, 2021, 21(2), 489 CrossRef CAS PubMed.
- B. Wei, L. Wang and B. C. Blount, Analysis of cannabinoids and their metabolites in human urine, Anal. Chem., 2015, 87(20), 10183–10187 CrossRef CAS PubMed.
- B. J. Hall, M. Satterfield-Doerr, A. R. Parikh and J. S. Brodbelt, Determination of cannabinoids in water and human saliva by solid-phase microextraction and quadrupole ion trap gas chromatography/mass spectrometry, Anal. Chem., 1998, 70(9), 1788–1796 CrossRef CAS PubMed.
- H. Stevenson, A. Bacon, K. M. Joseph, W. R. W. Gwandaru, A. Bhide, D. Sankhala, V. N. Dhamu and S. Prasad, A rapid response electrochemical biosensor for detecting THC in saliva, Sci. Rep., 2019, 9(1), 1–11 CAS.
- M. Klimuntowski, M. M. Alam, G. Singh and M. M. Howlader, Electrochemical Sensing of Cannabinoids in Biofluids: A Noninvasive Tool for Drug Detection, ACS Sens., 2020, 5(3), 620–636 CrossRef CAS PubMed.
- Q. Zhang, D. Berg and S. M. Mugo, Molecularly imprinted carbon based electrodes for tetrahydrocannabinol sensing, Inorg. Chem. Commun., 2019, 107, 107459 CrossRef CAS.
- H. T. Karnes, G. Shiu and V. P. Shah, Validation of bioanalytical methods, Pharm. Res., 1991, 8(4), 421–426 CrossRef CAS PubMed.
|
This journal is © The Royal Society of Chemistry 2022 |
Click here to see how this site uses Cookies. View our privacy policy here.