DOI:
10.1039/D2MD00340F
(Research Article)
RSC Med. Chem., 2023,
14, 113-121
Synthesis and bioactive evaluation of N-((1-methyl-1H-indol-3-yl)methyl)-N-(3,4,5-trimethoxyphenyl)acetamide derivatives as agents for inhibiting tubulin polymerization†
Received
20th September 2022
, Accepted 26th October 2022
First published on 28th October 2022
Abstract
Based on the inhibitory effect of CA-4 analogues and indoles on tubulin polymerization, we designed and synthesized a series of N-((1-methyl-1H-indol-3-yl)methyl)-2-(1H-pyrazol-1-yl or triazolyl)-N-(3,4,5-trimethoxyphenyl)acetamides. All the synthesized compounds were evaluated for their in vitro antiproliferative activities against HeLa, MCF-7 and HT-29 cancer cell lines, and some of the target compounds demonstrated effective activities towards the three tumour cell lines. Among them, compound 7d exhibited the most potent activities against HeLa (IC50 = 0.52 μM), MCF-7 (IC50 = 0.34 μM) and HT-29 (IC50 = 0.86 μM). Mechanistic studies revealed that compound 7d induced cell apoptosis in a dose-dependent manner, arrested the cells in the G2/M phase and inhibited polymerization of tubulin via a consistent way with colchicine. Therefore, 7d is a potential agent for the further development of tubulin polymerization inhibitors.
1. Introduction
Microtubules are dynamic filaments composed of α- and β-tubulin heterodimers. The dynamic instability of microtubules (the process of continuous polymerization and depolymerization) plays a crucial role in many cellular biological processes, including cell proliferation, division, motility, spindle formation, shape maintenance and intracellular transport.1–5 Many reports proposed that interference with microtubule dynamics is an important strategy for the development of novel microtubule-targeting agents (MTAs).6–8 MTAs disrupt the polymerization or depolymerization of microtubules by binding to different sites, such as the binding sites of paclitaxel,9,10 vincristine11,12 and colchicine.13,14 Among them, colchicine binding site inhibitors are generally not substrates of the multidrug-resistant efflux pump and can overcome the development of resistance mediated by P-glycoprotein.15,16 Some inhibitors targeting the colchicine binding site also exhibit a vasodestructive effect and act as vascular disrupting agents to exert antitumor effects.17,18 As a plant-derived inhibitor of microtubule polymerization, combretastatin A-4 (1, Fig. 1) has a simple structure, is extremely cytotoxic to a wide range of cells and binds to the colchicine binding site, which has generated interest in the design and invention of new CA-4 analogues.19–22 In the past few years, structural modifications on the cis-olefin between the two phenyl rings including replacement of the cis-olefin with a tertiary amide fragment, have been frequently utilized to design novel colchicine binding site inhibitors. The β-lactam derivative of CA-4 (2, Fig. 1) effectively inhibited tubulin polymerization (IC50 = 4.89 μM) and showed IC50 values of 0.8 and 176 nM against cancer cells MCF-7 and MDA-MB-231, respectively.22 The tertiary amide derivatives (3, 4 and 5, Fig. 1)23–25 inhibited excellently tubulin polymerization (IC50 values of 1.9, 3.35 and 2.16 μM respectively), and showed anti-proliferative activity against MGC-803 with IC50 values of 0.035, 0.45 and 0.13 μM respectively. Indoles have a wide range of biological activities.26 Indole-like tubulin inhibitors that target the colchicine binding site have been developed. VERU-111 (6, Fig. 1) is a potent tubulin inhibitor targeting the colchicine site with potent antiproliferative activities with an IC50 value of 5.2 nM against melanoma and prostate cancer cell lines.27,28 ZIO 301 (7, Fig. 1) is an orally administered tubulin polymerisation inhibitor with minimal neurotoxicity that reduces inter-kinetochoric tension, produces abnormal spindle bodies, activates the mitotic checkpoint proteins Mad2 and BubR1, and induces mitotic arrest and apoptosis.29 The CA-4 analogue (8, Fig. 1) showed excellently antiproliferative activity against HL-60, A549, HeLa and HT-29 cells with IC50 values in the range of 0.3–2.7 nM.30 Meanwhile, pyrazole derivatives (10 and 11, Fig. 1) exhibit anticancer activities.31,32 3-Methoxy-6-[4-(3,4,5-trimethoxyphenyl)-1H-pyrazol-3-yl]benzene-1,2-diol (9, Fig. 1), a compound with a pyrazolyl fragment, strongly inhibited tubulin polymerization with low nanomolar potency and showed effective anti-proliferative activity against SH-SY5Y cells (IC50 = 5.4 ± 1.1 nM).33 Up to now, although a large number of colchicine binding site inhibitors have been developed, none are currently available for clinical use.34,35 Based on the structures of the tubulin polymerisation inhibitors described above (Fig. 1), we designed a series of new compounds, 3,4,5-trimethoxy-N-((1-methyl-1H-indol-3-yl)methyl) anilines, in which the C–N fragment between the 3,4,5-trimethoxyphenyl and indole rings is similar to compounds 3, 4 and 5 (Fig. 1). After converting the anilines to 2-chloro-N-((1-methyl-1H-indol-3-yl)methyl)-N-(3,4,5-trimethoxyphenyl)acetamides, chlorines were substituted by pyrazole derivatives to give target compounds (Fig. 2). Herein, we report the synthesis and evaluation of their anticancer activities in vitro.
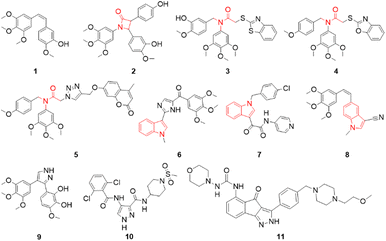 |
| Fig. 1 Examples of colchicine binding site inhibitors and pyrazole anticancer compounds. | |
 |
| Fig. 2 Design strategy of N-((1-methyl-1H-indol-3-yl)methyl)-N-(3,4,5-trimethoxyphenyl)acetamide derivatives. | |
2. Results and discussion
2.1 Chemistry
The target compounds were designed and synthesized (Scheme 1). The indole derivatives 1a–1k were treated with phosphorus oxychloride in DMF to generate compounds 2a–2k. Indole-3-formaldehyde derivatives 2a–2k reacted with sodium hydride and iodomethane in DMF to obtain compounds 3a–3k. Then N-methylindole-3-carbaldehyde derivatives 3a–3k reacted with 3,4,5-trimethoxyaniline in the presence of acetic acid to give imines 4a–4k. Imines 4a–4k were reduced with NaBH4 in methanol to give the secondary amines 5a–5k, and 5a–5k reacted with chloroacetyl chloride in the presence of triethylamine to give amides 6a–6k. Finally, 6a–6k reacted with pyrazoles or triazoles catalysed by potassium carbonate to give the target products, N-((1-methyl-1H-indol-3-yl)methyl)-2-(1H-pyrazol-1-yl or triazolyl)-N-(3,4,5-trimethoxyphenyl)acetamides 7a–7r. Structures of synthesized compounds 7a–7r were determined by 1H NMR, 13C NMR and ESI-MS.
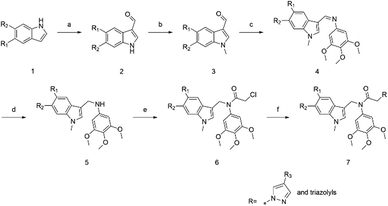 |
| Scheme 1 Reagents and conditions: (a) corresponding indoles, POCl3, DMF, 0 °C, 30 min and then rt, 3 h; (b) MeI, NaH, THF, 0 °C, 30 min and then rt, 3 h; (c) 3,4,5-trimethoxyaniline, CH3COOH, EtOH, 80 °C, 6 h; (d) NaBH4, MeOH, 70 °C, 5 h; (e) CH2ClCOCl, TEA, DCM, 0 °C, 30 min and then rt, 3 h; (f) pyrazole derivatives or triazoles, K2CO3, ACN, 85 °C, 8 h. | |
2.2 Biological evaluations
2.2.1 Antiproliferative activities in vitro.
The synthesized compounds 7a–7r were evaluated for their anti-proliferative activities against HeLa (human cervical carcinoma), MCF-7 (human breast adenocarcinoma) and HT-29 (human colon carcinoma) cell lines by MTT assay. Colchicine was selected as the positive control. The three cell lines were treated with five different concentrations of drugs (0.1, 0.5, 2.5, 12.5 and 30 μM) for 24 hours and then assayed by MTT. IC50 values are expressed as the mean ± SD of three independent experiments. The results (Table 1) showed that compounds 7c, 7d, 7f and 7i exhibited effective anti-proliferative activities with IC50 values ranging from 0.34 to 6.68 μM against the three cancer cell lines. Among them, compound 7d showed significant antiproliferative activities against HeLa, MCF-7 and HT-29 with IC50 values of 0.52, 0.34 and 0.86 μM, respectively. Compounds 7a–7g, which have no substituents in their 1-methylindole ring (R1 = R2 = H) and various substituents as R3 in their pyrazole ring, exhibited different inhibitory effects on the proliferation of HeLa, MCF-7 and HT-29 cell lines. Compounds 7c, 7d, 7e and 7f with OCH3, Cl, Br and CO2C2H5 as R3 respectively inhibited the cancer cell lines more effectively than the non-substituted 7a and other R3-substituted compounds such as 7b (CH3) and 7g (NO2). This result implied that some substituents, such as Cl, CO2C2H5 and OCH3 in the pyrazole ring, are reasonable. When CH3, OCH3, Cl and Br were used as the R1 or R2 substituents of 7h–7o, most of these compounds were more effective in the inhibition of the three cancer cell lines compared to 7a without substituents on the indole ring. The enhanced inhibitory effect of compounds 7h–7o on cell proliferation compared to compound 7a is thought to be mainly due to their substituents R2 and R3. The addition of electron-donating groups or halogens enhances the electron cloud density of the compounds, creating a greater opportunity for indole binding to microtubule proteins. Compounds 7a (containing pyrazol-1-yl) and 7p–7r (containing triazolyl), with an unsubstituted 1-methylindole ring, were structurally similar and showed weaker inhibition (IC50 > 20 μM) against the three cancer cell lines. This result suggested that the unsubstituted pyrazole group and these triazole groups exert similar bioactive effects.
Table 1 Antiproliferative activity (IC50 in μM) of compounds 7a–7r against HeLa, MCF-7 and HT-29 cancer cell lines in vitro
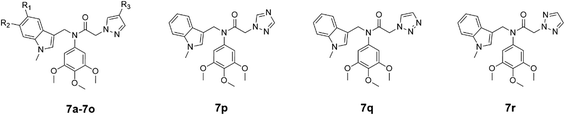
|
Compound |
R1 |
R2 |
R3 |
HeLa |
MCF-7 |
HT-29 |
7a
|
H |
H |
H |
>30 |
>30 |
26.91 ± 2.21 |
7b
|
H |
H |
CH3 |
>30 |
25.13 ± 2.31 |
23.42 ± 1.35 |
7c
|
H |
H |
OCH3 |
5.96 ± 1.09 |
4.14 ± 0.15 |
6.68 ± 0.32 |
7d
|
H |
H |
Cl |
0.52 ± 0.041 |
0.34 ± 0.023 |
0.86 ± 0.032 |
7e
|
H |
H |
Br |
13.10 ± 0.53 |
16.71 ± 0.37 |
9.52 ± 0.41 |
7f
|
H |
H |
CO2C2H5 |
2.36 ± 0.37 |
2.21 ± 0.23 |
1.27 ± 0.17 |
7g
|
H |
H |
NO2 |
>30 |
>30 |
>30 |
7h
|
CH3 |
H |
H |
14.21 ± 1.21 |
18.63 ± 2.04 |
20.92 ± 1.52 |
7i
|
OCH3 |
H |
H |
5.25 ± 0.34 |
3.86 ± 0.065 |
4.31 ± 0.25 |
7j
|
Cl |
H |
H |
9.18 ± 0.27 |
6.56 ± 0.24 |
7.23 ± 0.42 |
7k
|
Br |
H |
H |
10.13 ± 0.31 |
8.63 ± 0.60 |
12.56 ± 0.24 |
7l
|
H |
CH3 |
H |
>30 |
>30 |
25.94 ± 2.08 |
7m
|
H |
OCH3 |
H |
13.21 ± 1.05 |
12.95 ± 1.23 |
15.49 ± 0.86 |
7n
|
H |
Cl |
H |
7.28 ± 1.58 |
10.33 ± 0.95 |
9.92 ± 1.21 |
7o
|
H |
Br |
H |
>30 |
18.21 ± 1.05 |
15.38 ± 1.33 |
7p
|
|
|
|
>30 |
>30 |
>30 |
7q
|
|
|
|
>30 |
24.55 ± 1.52 |
21.09 ± 2.31 |
7r
|
|
|
|
>30 |
>30 |
25.46 ± 1.62 |
Colchicine |
|
|
|
0.28 ± 0.004 |
0.41 ± 0.031 |
0.22 ± 0.062 |
2.2.2 Tubulin polymerization inhibition in vitro.
To assess whether the inhibition of proliferation was attributed to the interaction of the compounds with tubulin, compound 7d was selected to assess its inhibitory effect on tubulin polymerization, colchicine was used as the positive control. As shown in Fig. 3, the optical density (OD) value decreased with increasing concentrations of 7d, which effectively inhibited microtubule polymerisation at 12 μM compared to the positive control colchicine (6 μM). The IC50 for the inhibitory activity of compound 7d on microtubules was 7.70 μM. Although less active than colchicine, this result suggested that compound 7d interacted directly to tubulin and is an alternative potential inhibitor of tubulin polymerization.
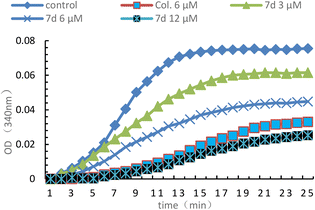 |
| Fig. 3 Effect of 7d (3, 6 and 12 μM) on tubulin polymerization in vitro and colchicine (6 μM) was used as the positive control and 0.1% DMSO was used as the blank control. 7d (3, 6 or 12 μM), colchicine (5 μM) and 0.1% DMSO were added into a 96-well plate and then polymerizations were detected over 25 min at 37 °C. | |
2.2.3 Immunofluorescence analysis.
To directly confirm the inhibitory effect of compound 7d on the dynamics of intracellular tubule aggregation, immunofluorescence assay in MCF-7 cells was performed. As shown in Fig. 4, the control group had a well-assembled microtubule network that exhibited normal arrangement and organization. After treatments with colchicine (0.35 μM) and 7d (0.35 and 1 μM) for 24 hours, respectively, the morphology of MCF-7 cells has been changed, the microtubule networks were disrupted and aggregated around the cell nuclei. This result confirmed that compound 7d effectively could interfere with the polymerization of microtubules.
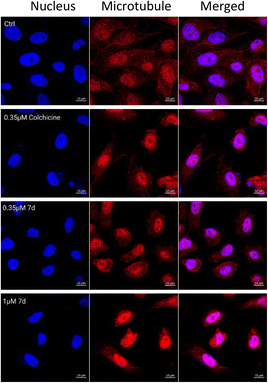 |
| Fig. 4 Images of immunofluorescence staining of microtubules in MCF-7 cells treated with colchicine (0.35 μM) and 7d (0.35 and 1 μM) for 24 h. The right and middle panels represent the tubulin assembly stained with anti-β-tubulin-Cy3 antibody and DAPI, and the left panel represents a merge of the corresponding right and middle panels. | |
2.2.4 Induce cell apoptosis.
To identify whether the compound 7d could induce apoptosis in MCF-7 cells, an apoptosis assay was carried out using an Annexin V-FITC/PI assay. As shown in Fig. 5, the percentage of apoptotic cells was 19.62% and 41.5% respectively after treating MCF-7 cells with two concentrations (0.35 and 1 μM) of compound 7d for 24 hours (meanwhile 36.7% for colchicine with 0.35 μM). The results indicated that compound 7d exerted apoptosis on MCF-7 cells in a dose-dependent manner.
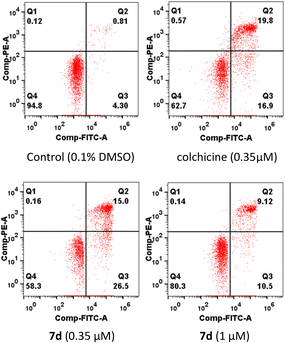 |
| Fig. 5 Effects of compound 7d to induce apoptosis of MCF-7 cells. MCF-7 cells were treated with 0.1% DMSO, colchicine (0.35 μM) and 7d (0.35 and 1 μM) and for 24 h, stained with PITC AV and PI, and determined by flow cytometric analysis. | |
2.2.5 Induce cell cycle arrest.
Tubulin polymerization inhibitors can arrest the cell cycle in the G2/M phase.36 The cell cycle blocking effect of compound 7d on MCF-7 cells was verified by flow cytometric analysis (Fig. 6). After treatments with 7d in concentrations of 0.35 and 1 μM for 24 hours, MCF-7 cells in the G2/M phase of the cell cycle were 22.44% and 42.69% respectively (27.11% for colchicine in 0.35 μM and 4.17% for the control). These results showed that the effect of compound 7d on cell cycle arrest was found to be concentration-dependent.
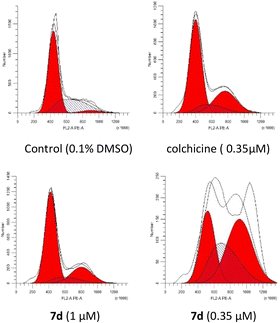 |
| Fig. 6 Compound 7d arrested cell cycle progression in a dose-dependent manner in MCF-7 cells. MCF-7 cells were treated with 1% DMSO, colchicine (0.35 μM) and 7d (0.35 and 1 μM) for 24 h, and then analysed by flow cytometry. | |
2.2.6 Cytotoxicity evaluation.
Compound 7d was evaluated in vitro against human normal LO2 cells and 293T to estimate the potential cytotoxicity on non-tumour cell lines. As shown in Table 2, compound 7d has lower toxicity toward LO2 cells and 293T cells than that of colchicine.
Table 2 Antiproliferative activity of 7d against human normal LO2 cells and 293T
Compound |
IC50 (μM) |
LO2 |
293T |
7d
|
48.35 ± 3.55 |
61.23 ± 4.37 |
Colchicine |
5.74 ± 0.43 |
3.86 ± 0.65 |
2.2.7 Molecular docking studies.
To confirm the interaction of compounds and tubulin, the protein residue of tubulin (PDB ID: 6O5N) was selected for the molecular docking study. The results showed (Fig. 7A and B) that compounds along with CA-4 were superimposed at the colchicine binding site of the protein. The direct binding of 7d to tubulin is probably due to 3,4,5-trimethoxybenzene forming a π–π interaction with HIS-192, and the phenyl ring in the pyrazole fragment forms a π–H and π–cation interaction with GLU-196 and ARG-264 (Fig. 7C and D). This means that compound 7d distorts the conformation of tubulin, similar to the behaviour of colchicine and CA-4, and is attributed to its biological activity. Compounds 7a–7g, which have no substituents in the indole ring, had docking scores of −5.90, −6.08, −6.74, −7.16, −6.49, −7.36 and −6.22 kcal mol−1 respectively, showing that these scores were inversely proportional to their biological activity. The lower scoring 7a, 7b and 7g were not effective in inhibiting cancer cells. Other compounds with lower scores also had weak inhibitory effects on cancer cells (more detailed data are available in the ESI†).
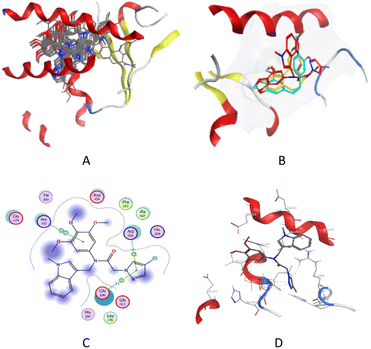 |
| Fig. 7 (A) All compounds inserted into the microtubule (PDB ID: 6O5N). (B) Possible binding mode of compound 7d (red), CA-4 (blue), and colchicine (yellow) with the protein residue of tubulin. (C) 2D view of the interaction of 7d at the binding site. (D) 3D view of the interaction of 7d at the binding site. | |
3. Conclusion
In brief, the synthesis and biological evaluation of a new series of N-((1-methyl-1H-indol-3-yl)methyl)-2-(1H-pyrazol-1-yl or triazolyl)-N-(3,4,5-trimethoxyphenyl)acetamide derivatives 7a–7r were described. Some of the compounds showed effective antiproliferative activity against three types of cancer cells. Among them, the promising compound 7d displayed potent antiproliferative activities towards HeLa, MCF-7, and HT-29 with IC50 values of 0.52, 0.34, and 0.86 μM, respectively. The results of mechanistic studies revealed that 7d induced cell apoptosis in a dose-dependent manner, arrested the cells in the G2/M phase and inhibited significantly tubulin polymerization. The results of molecular docking studies and bioactive behaviours were consistent with that of colchicine, 7d was a tubulin polymerization inhibitor.
4. Experimental section
4.1 Chemistry
4.1.1 Materials and methods.
Melting points were determined using an electrothermal melting point apparatus WRX-4 (Shanghai, China), NMR data of compounds were measured on a Bruker DRX 500 MHz (1H/13C, 500 MHz/125 MHz) spectrometer (Bruker, Bremerhaven, Germany), and chemical shifts were quoted in ppm with tetramethyl silane (TMS) as an internal reference. Coupling constants, J, is expressed in hertz (Hz). All reactions were monitored using thin layer chromatography (TLC) on silica gel plates. All synthesized compounds have purity >95%.
4.1.2 General procedure for the preparation of 1H-indole-3-carboxaldehyde (2a–2k).
The relevant indoles (1a–1k, 5 mmol) in DMF (6 mL) was added to a stirred and completely mixed solution of POCl3 (3 mL) in DMF (6 mL) at 0 °C. After 30 minutes, the ice bath was removed and stirring was continued at room temperature for 3 hours. At the end of the reaction, the mixture was poured into 100 mL ice water, and then 1 M NaOH was added to adjust to pH = 10. A precipitant was obtained as 2a (or 2b–2k), and was used in the next step without further purification.
4.1.3 General procedure for the preparation of 1-methyl-1H-indole-3-carboxaldehyde (3a–3k).
The indole-3-carboxaldehyde (2a, or 2b–2k, 5 mmol) was dissolved in DMF (25 mL), and NaH (60%, 6 mmol) was added to the solution at 0 °C and CH3I (13.2 mmol) was added after 30 min stirring. Then the reaction mixture was poured into 100 mL ice water. 3a (or 3b–3k) was obtained by filtering and was used in the next step without further purification.
4.1.4 General procedure for the preparation of 1-(1-methyl-1H-indol-3-yl)-N-(3,4,5-trimethoxyphenyl)methenamine (4a–4k).
1-Methyl-1H-indole-3-carboxaldehyde (3a, or 3b–3k, 2 mmol) was dissolved in ethanol (20 mL), and 3,4,5-trimethoxyaniline (2 mmol) and acetic acid (1 ml) were added under stirring and refluxed at 80 °C for 6 hours. The reaction mixture was cooled to room temperature and filtered to give a crude product. This crude product was crystallized in cold ethanol to give 1-(1-methyl-1H-indol-3-yl)-N-(3,4,5-trimethoxyphenyl)methenamine (4a) (or 4b–4k).
4.1.5 General procedure for the preparation of 3,4,5-trimethoxy-N-((1-methyl-1H-indol-3-yl)methyl)aniline (5a–5k).
1-(1-Methyl-1H-indol-3-yl)-N-(3,4,5-trimethoxyphenyl)methenamine (4a, or 4b–4k, 1.5 mmol) was dissolved in methanol (20 ml), and sodium borohydride (6 mmol) was added under stirring and refluxed at 70 °C for 5 hours. Then the mixture was poured into 100 mL water and mixed completely. 5a (or 5b–5k) was obtained after filtering, and was used in the next step without further purification.
4.1.6 General procedure for the preparation of 2-chloro-N-((1-methyl-1H-indol-3-yl)methyl)-N-(3,4,5-trimethoxyphenyl)acetamide (6a–6k).
3,4,5-Trimethoxy-N-((1-methyl-1H-indol-3-yl)methyl)aniline (5a, or 5b–5k, 1 mmol) was dissolved in dichloromethane (10 ml), and triethylamine (1 mmol) and chloroacetyl chloride (1.2 mmol) were successively added to the dichloromethane solution at 0 °C, and then the reaction mixture was stirred at room temperature for 3 hours. After the completion of the reaction, the solvent was removed from the reaction mixture to give a residue. To the residue, water was added and then extracted with ethyl acetate. The ethyl acetate solution was washed with water and brine, and then dried over anhydrous sodium sulfate. 6a (or 6b–6k) was obtained from the ethyl acetate solution after evaporation under vacuum, and was used in the next step without further purification.
4.1.7 General procedure for the preparation of N-((1-methyl-1H-indol-3-yl)methyl)-2-(1H-pyrazol-1-yl)-N-(3,4,5-trimethoxyphenyl)acetamide (7a–7r).
2-Chloro-N-((1-methyl-1H-indol-3-yl)methyl)-N-(3,4,5-trimethoxyphenyl)acetamide 6a (or 6b–6k) (1 mmol), pyrazole derivatives (1.2 mmol), and K2CO3 (1.5 mmol) were dissolved in acetonitrile (8 ml), and the reaction mixture was refluxed at 85 °C for 8 hours. After the completion of the reaction, the solvent was removed from the reaction mixture to provide a residue, water was added to the residue, and then was extracted with ethyl acetate (3 × 50 ml). The crude product was obtained from the ethyl acetate solution, and was purified by flash silica gel H column chromatography to give 7a (or 7b–7r) using ethyl acetate and petroleum ether (EA
:
PE = 1
:
2) as solvents.
4.1.8
N-((1-Methyl-1H-indol-3-yl)methyl)-2-(1H-pyrazol-1-yl)-N-(3,4,5-trimethoxyphenyl)acetamide (7a).
White solid. Yield 65%. mp 156.9–157.6 °C. 1H NMR (500 MHz, Chloroform-d) δ 7.53 (d, J = 1.9 Hz, 1H), 7.50 (d, J = 2.3 Hz, 1H), 7.34 (dt, J = 7.8, 1.0 Hz, 1H), 7.29 (d, J = 8.2 Hz, 1H), 7.20 (ddd, J = 8.2, 6.9, 1.1 Hz, 1H), 7.05 (ddd, J = 7.9, 6.9, 1.0 Hz, 1H), 7.03 (s, 1H), 6.31 (t, J = 2.1 Hz, 1H), 6.23 (s, 2H), 5.02 (s, 2H), 4.73 (s, 2H), 3.87 (s, 3H), 3.73 (s, 3H), 3.62 (s, 6H). 13C NMR (126 MHz, Chloroform-d) δ 166.07, 153.67, 139.64, 138.11, 136.73, 136.31, 130.92, 129.95, 127.48, 121.74, 119.56, 119.32, 110.15, 109.17, 106.07, 105.98, 61.01, 56.10, 53.59, 44.66, 32.72. MS (ESI) m/z: 433.1875 [M − H]− (calcd for 433.1954, C24H26N4O4).
4.1.9
N-((1-Methyl-1H-indol-3-yl)methyl)-2-(4-methyl-1H-pyrazol-1-yl)-N-(3,4,5-trimethoxyphenyl)acetamide (7b).
White solid. Yield 54%. mp 179.4–180.2 °C. 1H NMR (500 MHz, Chloroform-d) δ 7.34 (dd, J = 8.0, 0.9 Hz, 1H), 7.33 (s, 1H), 7.29 (d, J = 8.1 Hz, 1H), 7.25 (s, 1H), 7.21 (ddd, J = 8.2, 7.0, 1.2 Hz, 1H), 7.07–7.04 (m, 1H), 7.03 (s, 1H), 6.22 (s, 2H), 5.01 (s, 2H), 4.65 (s, 2H), 3.87 (s, 3H), 3.74 (s, 3H), 3.62 (s, 6H), 2.10 (s, 3H). 13C NMR (126 MHz, Chloroform-d) δ 166.32, 153.65, 140.20, 138.08, 136.71, 136.37, 129.97, 129.64, 127.49, 121.72, 119.58, 119.31, 116.52, 110.20, 109.15, 105.97, 61.01, 56.08, 53.58, 44.67, 32.71, 8.98. MS (ESI) m/z: 449.2179 [M + H]+ (calcd for 449.2111, C25H28N4O4).
4.1.10 2-(4-Methoxy-1H-pyrazol-1-yl)-N-((1-methyl-1H-indol-3-yl)methyl)-N-(3,4,5-trimethoxyphenyl)acetamide (7c).
White solid. Yield 58%. mp 160.1–161.5 °C. 1H NMR (500 MHz, Chloroform-d) δ 7.35 (dt, J = 8.0, 1.0 Hz, 1H), 7.29 (dd, J = 8.2, 1.0 Hz, 1H), 7.24 (d, J = 0.9 Hz, 1H), 7.20 (ddd, J = 8.1, 6.8, 1.1 Hz, 1H), 7.17 (d, J = 0.9 Hz, 1H), 7.05 (ddd, J = 8.0, 6.8, 1.0 Hz, 1H), 7.02 (s, 1H), 6.20 (s, 2H), 5.01 (s, 2H), 4.61 (s, 2H), 3.86 (s, 3H), 3.75 (s, 3H), 3.73 (s, 3H), 3.60 (s, 6H). 13C NMR (126 MHz, Chloroform-d) δ 166.15, 153.65, 147.10, 138.10, 136.73, 136.23, 129.93, 127.41 (d, J = 15.4 Hz), 121.76, 119.55, 119.33, 115.40, 110.14, 109.18, 105.94, 61.00, 58.75, 56.07, 54.35, 44.61, 32.71. MS (ESI) m/z: 465.2129 [M + H]+ (calcd for 465.2060, C25H28N4O5).
4.1.11 2-(4-Chloro-1H-pyrazol-1-yl)-N-((1-methyl-1H-indol-3-yl)methyl)-N-(3,4,5-trimethoxyphenyl)acetamide (7d).
White solid. Yield 63%. mp 207.2–208.1 °C. 1H NMR (500 MHz, Chloroform-d) δ 7.50 (s, 1H), 7.43 (s, 1H), 7.33 (dt, J = 7.9, 1.0 Hz, 1H), 7.30 (d, J = 8.0 Hz, 1H), 7.21 (ddd, J = 8.1, 6.9, 1.1 Hz, 1H), 7.06 (ddd, J = 7.9, 6.9, 1.0 Hz, 1H), 7.02 (s, 1H), 6.21 (s, 2H), 5.01 (s, 2H), 4.67 (s, 2H), 3.87 (s, 3H), 3.74 (s, 3H), 3.62 (s, 6H). 13C NMR (126 MHz, Chloroform-d) δ 165.46, 153.74, 138.15 (d, J = 17.8 Hz), 136.74, 136.05, 129.94, 128.98, 127.43, 121.81, 119.45 (d, J = 14.8 Hz), 110.43, 109.99, 109.22, 105.90, 61.03, 56.11, 54.23, 44.76, 32.74. MS (ESI) m/z: 467.1487 [M − H]− (calcd for 467.1564, C24H25N4O4Cl).
4.1.12 2-(4-Bromo-1H-pyrazol-1-yl)-N-((1-methyl-1H-indol-3-yl)methyl)-N-(3,4,5-trimethoxyphenyl)acetamide (7e).
White solid. Yield 63%. mp 198.9–200.5 °C. 1H NMR (500 MHz, Chloroform-d) δ 7.53 (s, 1H), 7.47 (s, 1H), 7.33 (dt, J = 8.0, 1.0 Hz, 1H), 7.29–7.31 (m, 1H), 7.22 (ddd, J = 8.2, 6.9, 1.1 Hz, 1H), 7.06 (ddd, J = 8.0, 6.9, 1.0 Hz, 1H), 7.02 (s, 1H), 6.20 (s, 2H), 5.01 (s, 2H), 4.69 (s, 2H), 3.87 (s, 3H), 3.74 (s, 3H), 3.62 (s, 6H).13C NMR (126 MHz, Chloroform-d) δ 165.44, 153.73, 140.19, 138.22, 136.74, 136.05, 131.12, 129.94, 127.43, 121.81, 119.50, 119.39, 109.98, 109.21, 105.89, 93.61, 61.04, 56.11, 54.15, 44.77, 32.74. MS (ESI) m/z: 511.0980 [M − H]− (calcd for 511.1059, C24H25N4O4Br).
4.1.13 Ethyl 1-(2-(((1-methyl-1H-indol-3-yl)methyl)(3,4,5-trimethoxyphenyl)amino)-2-oxoethyl)-1H-pyrazole-4-carboxylate (7f).
White solid. Yield 71%. mp 181.4–182.8 °C. 1H NMR (500 MHz, Chloroform-d) δ 7.97 (s, 1H), 7.89 (s, 1H), 7.30 (dt, J = 7.9, 1.0 Hz, 1H), 7.26 (dt, J = 8.2, 0.9 Hz, 1H), 7.18 (ddd, J = 8.2, 6.9, 1.1 Hz, 1H), 7.02 (ddd, J = 8.0, 7.0, 1.0 Hz, 1H), 6.98 (s, 1H), 6.19 (s, 2H), 4.98 (s, 2H), 4.69 (s, 2H), 4.27 (q, J = 7.1 Hz, 2H), 3.84 (s, 3H), 3.70 (s, 3H), 3.58 (s, 6H), 1.32 (t, J = 7.1 Hz, 3H). 13C NMR (126 MHz, Chloroform-d) δ 165.16, 162.89, 153.75, 141.21, 138.25, 136.74, 135.98, 134.58, 129.93, 127.42, 121.81, 119.43 (d, J = 10.7 Hz), 115.73, 109.93, 109.22, 105.90, 61.01, 60.13, 56.10, 53.89, 44.77, 32.72, 14.41. MS (ESI) m/z: 507.2233 [M + H]+ (calcd for 507.2165, C27H30N4O6).
4.1.14
N-((1-Methyl-1H-indol-3-yl)methyl)-2-(4-nitro-1H-pyrazol-1-yl)-N-(3,4,5-trimethoxyphenyl)acetamide (7g).
White solid. Yield 43%. mp 183.9–185.2 °C. 1H NMR (500 MHz, Chloroform-d) δ 8.29 (s, 1H), 8.05 (s, 1H), 7.33 (dt, J = 7.9, 0.9 Hz, 1H), 7.29 (d, J = 7.7 Hz, 1H), 7.21 (ddd, J = 8.1, 6.9, 1.1 Hz, 1H), 7.05 (ddd, J = 8.0, 6.9, 1.0 Hz, 1H), 6.99 (s, 1H), 6.24 (s, 2H), 5.02 (s, 2H), 4.74 (s, 2H), 3.87 (s, 3H), 3.73 (s, 3H), 3.63 (s, 6H).13C NMR (126 MHz, Chloroform-d) δ 164.30, 153.87, 138.41, 136.78, 136.20, 135.67 (d, J = 18.8 Hz), 130.76, 129.94, 127.36, 121.90, 119.42 (d, J = 7.4 Hz), 109.69, 109.32, 105.88, 77.35, 76.99, 61.03, 56.16, 54.47, 44.89, 32.74. MS (ESI) m/z: 478.1726 [M − H]− (calcd for 478.1805, C24H25N5O6).
4.1.15
N-((1,5-Dimethyl-1H-indol-3-yl)methyl)-2-(1H-pyrazol-1-yl)-N-(3,4,5-trimethoxyphenyl)acetamide (7h).
White solid. Yield 72%. mp 121.0–122.3 °C. 1H NMR (500 MHz, Chloroform-d) δ 7.52 (d, J = 1.8 Hz, 1H), 7.49 (d, J = 2.3 Hz, 1H), 7.17 (d, J = 8.3 Hz, 1H), 7.07 (t, J = 0.9 Hz, 1H), 7.02 (dd, J = 8.4, 1.7 Hz, 1H), 6.98 (s, 1H), 6.31 (t, J = 2.2 Hz, 1H), 6.25 (s, 2H), 4.98 (s, 2H), 4.74 (s, 2H), 3.87 (s, 3H), 3.69 (s, 3H), 3.62 (s, 6H), 2.38 (s, 3H).13C NMR (126 MHz, Chloroform-d) δ 166.02, 153.63, 139.62, 138.09, 136.42, 135.19, 130.92, 130.07, 128.44, 127.68, 123.32, 119.19, 109.55, 108.92, 106.07 (d, J = 5.0 Hz), 77.40, 77.14, 76.89, 61.00, 56.06, 53.59, 44.72, 32.72, 21.42. MS (ESI) m/z: 447.2032 [M + H]+ (calcd for 447.2111, C25H28N4O4).
4.1.16
N-((5-Methoxy-1-methyl-1H-indol-3-yl)methyl)-2-(1H-pyrazol-1-yl)-N-(3,4,5-trimethoxyphenyl)acetamide (7i).
White solid. Yield 69%. mp 119.6–121.1 °C. 1H NMR (500 MHz, Chloroform-d) δ 7.51 (d, J = 1.8 Hz, 1H), 7.48 (d, J = 2.3 Hz, 1H), 7.17 (d, J = 8.8 Hz, 1H), 6.97 (s, 1H), 6.85 (dd, J = 8.8, 2.4 Hz, 1H), 6.78 (d, J = 2.4 Hz, 1H), 6.30 (t, J = 2.1 Hz, 1H), 6.24 (s, 2H), 4.98 (s, 2H), 4.73 (s, 2H), 3.85 (s, 3H), 3.72 (s, 3H), 3.69 (s, 3H), 3.63 (s, 6H).13C NMR (126 MHz, Chloroform-d) δ 166.04, 154.06, 153.65, 139.63, 138.08, 136.31, 132.07, 130.89, 130.38, 127.78, 112.28, 110.02, 109.80, 106.05, 101.00, 77.31, 60.94, 56.10, 55.70, 53.56, 44.74, 32.86. MS (ESI) m/z: 463.1982 [M − H]− (calcd for 463.2060, C25H28N4O5).
4.1.17
N-((5-Chloro-1-methyl-1H-indol-3-yl)methyl)-2-(1H-pyrazol-1-yl)-N-(3,4,5-trimethoxyphenyl)acetamide (7j).
White solid. Yield 65%. mp 124.5–125.9 °C. 1H NMR (500 MHz, Chloroform-d) δ 7.51 (d, J = 1.9 Hz, 1H), 7.46 (d, J = 2.4 Hz, 1H), 7.20 (d, J = 2.0 Hz, 1H), 7.18 (d, J = 8.5 Hz, 1H), 7.13 (dd, J = 8.7, 2.0 Hz, 1H), 7.10 (s, 1H), 6.29 (t, J = 2.1 Hz, 1H), 6.26 (s, 2H), 4.93 (s, 2H), 4.73 (s, 2H), 3.88 (s, 3H), 3.71 (s, 3H), 3.66 (s, 6H). 13C NMR (126 MHz, Chloroform-d) δ 166.16, 153.82, 139.69, 138.35, 136.38, 135.08, 131.45, 130.92, 128.46, 125.18, 121.96, 118.93, 110.36, 109.96, 106.02 (d, J = 14.7 Hz), 77.33, 61.08, 56.16, 53.54, 44.67, 32.92. MS (ESI) m/z: 467.1485 [M − H]− (calcd for 467.1564, C24H25N4O4Cl).
4.1.18
N-((5-Bromo-1-methyl-1H-indol-3-yl)methyl)-2-(1H-pyrazol-1-yl)-N-(3,4,5-trimethoxyphenyl)acetamide (7k).
White solid. Yield 58%. mp 120.2–121.4 °C. 1H NMR (500 MHz, Chloroform-d) δ 7.51 (d, J = 1.9 Hz, 1H), 7.46 (d, J = 2.4 Hz, 1H), 7.34 (d, J = 1.9 Hz, 1H), 7.25 (dd, J = 8.6, 1.9 Hz, 1H), 7.14 (d, J = 8.7 Hz, 1H), 7.09 (s, 1H), 6.29 (t, J = 2.1 Hz, 1H), 6.26 (s, 2H), 4.92 (s, 2H), 4.72 (s, 2H), 3.89 (s, 3H), 3.70 (s, 3H), 3.67 (s, 6H). 13C NMR (126 MHz, Chloroform-d) δ 166.17, 153.82, 139.70, 138.35, 136.41, 135.32, 131.33, 130.92, 129.12, 124.50, 122.04, 112.70, 110.81, 109.90, 106.01 (d, J = 17.2 Hz), 61.12, 56.18, 53.54, 44.70, 32.91. MS (ESI) m/z: 511.0979 [M − H]− (calcd for 511.1059, C24H25N4O4Br).
4.1.19
N-((1,6-Dimethyl-1H-indol-3-yl)methyl)-2-(1H-pyrazol-1-yl)-N-(3,4,5-trimethoxyphenyl)acetamide (7l).
White solid. Yield 58%. mp 119.3–121.5 °C. 1H NMR (500 MHz, Chloroform-d) δ 7.52 (d, J = 1.9 Hz, 1H), 7.50 (d, J = 2.3 Hz, 1H), 7.25 (d, J = 8.1 Hz, 1H), 7.08 (s, 1H), 6.92 (s, 1H), 6.89 (dd, J = 8.1, 1.5 Hz, 1H), 6.31 (t, J = 2.1 Hz, 1H), 6.23 (s, 2H), 4.99 (s, 2H), 4.73 (s, 2H), 3.87 (s, 3H), 3.69 (s, 3H), 3.63 (s, 6H), 2.48 (s, 3H). 13C NMR (126 MHz, Chloroform-d) δ 166.02, 153.64, 139.62, 138.08, 137.18, 136.32, 131.56, 130.91, 129.31, 125.35, 121.10, 119.32, 110.00, 109.12, 106.03 (d, J = 7.2 Hz), 61.00, 56.11, 53.60, 44.72, 32.61, 21.83. MS (ESI) m/z: 447.2033 [M − H]− (calcd for 447.2111, C25H28N4O4).
4.1.20
N-((6-Methoxy-1-methyl-1H-indol-3-yl)methyl)-2-(1H-pyrazol-1-yl)-N-(3,4,5-trimethoxyphenyl)acetamide (7m).
White solid. Yield 67%. mp 131.1–132.4 °C. 6.42, 153.62, 139.57, 138.05, 137.51, 136.24, 130.93, 128.74, 121.81, 120.32, 110.16, 109.17, 105.98 (d, J = 6.4 Hz), 92.72, 60.96, 56.11, 55.70, 53.57, 44.66, 32.67. MS (ESI) m/z: 463.1981 [M − H]− (calcd for 463.2060, C25H28N4O5).
4.1.21
N-((6-Chloro-1-methyl-1H-indol-3-yl)methyl)-2-(1H-pyrazol-1-yl)-N-(3,4,5-trimethoxyphenyl)acetamide (7n).
White solid. Yield 67%. mp 123.8–124.6 °C. 1H NMR (500 MHz, Chloroform-d) δ 7.52 (d, J = 1.9 Hz, 1H), 7.47 (d, J = 2.3 Hz, 1H), 7.27 (d, J = 1.8 Hz, 1H), 7.26 (d, J = 8.5 Hz, 1H), 7.02–6.99 (m, 1H), 6.99 (s, 1H), 6.30 (t, J = 2.1 Hz, 1H), 6.21 (s, 2H), 4.96 (s, 2H), 4.72 (s, 2H), 3.86 (s, 3H), 3.68 (s, 3H), 3.64 (s, 6H). 13C NMR (126 MHz, Chloroform-d) δ 166.15, 153.75, 139.70, 138.19, 137.13, 136.17, 130.89, 130.58, 127.85, 126.00, 120.57, 119.99, 110.53, 109.26, 106.10, 105.85, 61.01, 56.15, 53.55, 44.49, 32.81. MS (ESI) m/z: 467.1483 [M − H]− (calcd for 467.1564, C24H25N4O4Cl).
4.1.22
N-((6-Bromo-1-methyl-1H-indol-3-yl)methyl)-2-(1H-pyrazol-1-yl)-N-(3,4,5-trimethoxyphenyl)acetamide (7o).
White solid. Yield 65%. mp 109.4–110.8 °C. 1H NMR (500 MHz, Chloroform-d) δ 7.51 (d, J = 1.8 Hz, 1H), 7.46 (d, J = 2.3 Hz, 1H), 7.43 (d, J = 1.7 Hz, 1H), 7.21 (d, J = 8.4 Hz, 1H), 7.12 (dd, J = 8.4, 1.7 Hz, 1H), 6.97 (s, 1H), 6.29 (t, J = 2.1 Hz, 1H), 6.20 (s, 2H), 4.95 (s, 2H), 4.71 (s, 2H), 3.86 (s, 3H), 3.67 (s, 3H), 3.63 (s, 6H).13C NMR (126 MHz, Chloroform-d) δ 166.15, 153.74, 139.69, 138.18, 137.53, 136.14, 130.91, 130.51, 126.31, 122.54, 120.93, 115.44, 112.28, 110.55, 106.09, 105.85, 61.01, 56.15, 53.53, 44.46, 32.81. MS (ESI) m/z: 511.0977 [M − H]− (calcd for 511.1059, C24H25N4O4Br).
4.1.23
N-((1-Methyl-1H-indol-3-yl)methyl)-2-(1H-1,2,4-triazol-1-yl)-N-(3,4,5-trimethoxyphenyl)acetamide (7p).
White solid. Yield 74%. mp 189.2–190.4 °C. 1H NMR (500 MHz, Chloroform-d) δ 8.21 (s, 1H), 7.93 (s, 1H), 7.32 (d, J = 7.9 Hz, 1H), 7.28 (d, J = 8.2 Hz, 1H), 7.20 (ddd, J = 8.2, 6.9, 1.1 Hz, 1H), 7.04 (ddd, J = 7.9, 6.9, 1.0 Hz, 1H), 6.99 (s, 1H), 6.22 (s, 2H), 5.01 (s, 2H), 4.76 (s, 2H), 3.86 (s, 3H), 3.72 (s, 3H), 3.61 (s, 6H).13C NMR (126 MHz, Chloroform-d) δ 164.61, 153.79, 151.65, 144.77, 138.31, 136.75, 135.85, 129.90, 127.39, 121.85, 119.42, 109.82, 109.26, 105.90, 61.01, 56.13, 51.04, 44.79, 32.74. MS (ESI) m/z: 434.1830 [M − H]− (calcd for 434.1907, C23H25N5O4).
4.1.24
N-((1-Methyl-1H-indol-3-yl)methyl)-2-(1H-1,2,3-triazol-1-yl)-N-(3,4,5-trimethoxyphenyl)acetamide (7q).
White solid. Yield 43%. mp 98.7–100.3 °C. 1H NMR (500 MHz, Chloroform-d) δ 7.81 (d, J = 1.0 Hz, 1H), 7.74 (d, J = 1.1 Hz, 1H), 7.33 (dt, J = 8.1, 1.0 Hz, 1H), 7.30 (dt, J = 8.1, 0.9 Hz, 1H), 7.21 (ddd, J = 8.2, 6.9, 1.1 Hz, 1H), 7.06 (ddd, J = 7.9, 6.9, 1.0 Hz, 1H), 6.99 (s, 1H), 6.23 (s, 2H), 5.02 (s, 2H), 4.99 (s, 2H), 3.88 (s, 3H), 3.74 (s, 3H), 3.63 (s, 6H). 13C NMR (126 MHz, Chloroform-d) δ 164.43, 153.83, 138.36, 136.76, 135.78, 133.83, 129.89, 127.37, 125.31, 121.89, 119.43 (d, J = 5.9 Hz), 109.80, 109.28, 105.90, 61.03, 56.15, 51.18, 44.76, 32.75. MS (ESI) m/z: 434.1830 [M − H]− (calcd for 434.1907, C23H25N5O4).
4.1.25
N-((1-Methyl-1H-indol-3-yl)methyl)-2-(2H-1,2,3-triazol-2-yl)-N-(3,4,5-trimethoxyphenyl)acetamide (7r).
White solid. Yield 25%. mp 158.2–159.7 °C. 11H NMR (500 MHz, Chloroform-d) δ 7.69 (s, 2H), 7.32 (dt, J = 7.9, 1.0 Hz, 1H), 7.30–7.28 (m, 1H), 7.20 (ddd, J = 8.2, 6.9, 1.2 Hz, 1H), 7.08–7.01 (m, 2H), 6.27 (s, 2H), 5.07 (s, 2H), 5.02 (s, 2H), 3.87 (s, 3H), 3.73 (s, 3H), 3.62 (s, 6H). 13C NMR (126 MHz, Chloroform-d) δ 165.04, 153.74, 138.20, 136.69, 136.05, 134.88, 130.02, 127.48, 121.74, 119.51, 119.35, 109.97, 109.16, 105.95, 61.01, 56.18, 56.10, 44.68, 32.71. MS (ESI) m/z: 434.1839 [M − H]− (calcd for 434.1907, C23H25N5O4).
4.2 Biological assays
4.2.1 Cell proliferation inhibitory assay.
HeLa (human cervical carcinoma cell line), MCF-7 (human breast adenocarcinoma cell line), HT-29 (human colon carcinoma cell line), LO2 (human normal liver cell line) and 293T (human renal epithelial cell line) (ATCC cell bank, USA) were employed to evaluate the antiproliferative activity using MTT assay. Cells were seeded at a density of 5000 cells per well in 96-well plates and incubated at 37 °C, 5% CO2 and saturated humidity for 24 hours. Then cells were treated with different concentrations of the test compounds and the positive control colchicine and incubated for 24 h. The MTT reagent (5 mg mL−1 in PBS) was added to each well and incubated at 37 °C for 4 hours. At the end of the incubation, the supernatant was carefully removed from each well and 150 μl of DMSO was added to completely dissolve the MTT formazan. Spectra were read at 570 nm using a SuPerMax 3100 multifunctional zymography (SuPer, Shanghai, China) and expressed as percentage inhibition. IC50 values were calculated using SPSS software and expressed as the mean ± SD of three independent experiments.
4.2.2 Tubulin polymerization inhibitory assay.
Inhibitions of tubulin polymerization were carried out by using a fluorescence-based tubulin polymerization assay kit (Cytoskeleton-Cat. #BK011P) according to the manufacturer's protocol. Different concentrations of compound 7d or the positive control colchicine were added to 96-well plates and incubated at 37 °C for 1 min. Then 100 μl tubulin (4 mg ml−1 porcine brain tubulin was dissolved in general tubulin buffer containing 80 mM PIPES (pH = 6.9), 2 mM MgCl2, 0.5 mM EGTA, 1 mM GTP and 5% (v/v) glycerol) was added to the corresponding wells to initiate the polymerization reaction. Tubulin polymerization was monitored (emission wavelength of 450 ± 20 nm and excitation wavelength of 360 ± 20 nm) using SuPerMax 3100 multifunctional enzyme markers (SuPer, Shanghai, China) at 37 °C every 60 s over 25 min.
4.2.3 Immunofluorescence analysis.
MCF-7 cells were seeded in 6-well plates and incubated in a cell culture incubator (37 °C, 5% CO2, saturated humidity) for 24 hours. Then different concentrations of compound 7d and the positive control colchicine were added and incubated for further 24 hours. The cells were fixed with 4% paraformaldehyde for 25 min at room temperature and washed three times with PBS. 1 mL of 0.1% TritonX-100 was added to each well and left at room temperature for 15 minutes. The cells were incubated in 3% BSA for 1 h. Then the cells were incubated overnight with the primary antibody (β-tubulin, 1
:
500 dilution in 2.5% BSA). After incubation, the cells were washed three times with PBS and incubated with the secondary antibody (Cyanine 3, 1
:
500 dilution in 2.5% BSA) for 2 hours at 25 °C in the dark. Finally, the cells were washed three times with PBS and then stained with DAPI for 25 minutes at room temperature. The cells were visualized using an Olympus FV3000 laser confocal microscope (Olympus, Japan).
4.2.4 Apoptosis analysis.
MCF-7 cells were seeded in 6-well plates and incubated in a cell culture incubator (37 °C, 5% CO2, saturated humidity) for 24 hours. Then the cells were treated with different concentrations of compound 7d and the positive control colchicine for 24 h. The cells were collected and suspended in binding buffer containing Annexin V-FITC (0.5 mg mL−1) and PI (0.5 mg mL−1) at room temperature for 20 min in the dark. The cells were detected by flow cytometry using a FACSCanto-II instrument (Becton Dickinson, USA).
4.2.5 Cell cycle analysis.
MCF-7 cells were seeded in 6-well plates and incubated in a cell culture incubator (37 °C, 5% CO2, saturated humidity) for 24 hours. Then the cells were treated with different concentrations of compound 7d and the positive control colchicine and subsequently incubated for further 24 hours. The cells were collected and fixed overnight at 4 °C in 70% ethanol. The fixed cells were washed and resuspended with PBS containing 0.1 mg mL−1 RNase A and 5 mg mL−1 PI and incubated for 20 min in the dark. The cell concentration was adjusted with PBS and the cells were analysed by flow cytometry using a FACSCanto-II instrument (Becton Dickinson, USA).
4.2.6 Molecular docking studies.
The X-ray crystal structure of tubulin α-1B chain (PDB: 6O5N)37 was downloaded from the RCSB Protein Data Bank (https://www.rcsb.org) using Molecular Operating Environment 2015 for docking studies. The protein was prepared according to the MOE 2015 protocol. After removal of small molecules and ions, the chain structures were repaired, then were protonated and added partial charges. Compound 7d with optimised conformation38,39 was docked to the protein. The docking parameters were left at their default values and the docking score was used to assess the interactions between the test compound and the protein.40
Conflicts of interest
There are no conflicts to declare.
Acknowledgements
This work was supported by the grants from the National Natural Science Foundation of China (81760635).
Notes and references
- S. Honore, E. Pasquier and D. Braguer, Cell. Mol. Life Sci., 2005, 62, 3039–3056 CrossRef CAS PubMed.
- H. Kadavath, R. V. Hofele, J. Biernat, S. Kumar, K. Tepper, H. Urlaub, E. Mandelkow and M. Zweckstetter, Proc. Natl. Acad. Sci. U. S. A., 2015, 112, 7501–7506 CrossRef CAS.
- A. Akhmanova and M. Steinmetz, Nat. Rev. Mol. Cell Biol., 2015, 16, 711–726 CrossRef CAS PubMed.
- A. Muroyama and T. Lechler, Development, 2017, 144, 3012–3021 CrossRef CAS.
- C. Dumontet and M. A. Jordan, Nat. Rev. Drug Discovery, 2010, 9, 790–803 CrossRef CAS.
- M. Tagliamento, C. Genova, G. Rossi, S. Coco, E. Rijavec, M. G. Dal Bello, S. Boccardo, F. Grossi and A. Alama, Expert Opin. Invest. Drugs, 2019, 28, 513–523 CrossRef CAS.
- N. Vindya, N. Sharma, M. Yadav and K. Ethiraj, Curr. Top. Med. Chem., 2015, 15, 73–82 CrossRef CAS.
- G. Chandrasekaran, P. Tátrai and F. Gergely, Br. J. Cancer, 2015, 113, 693–698 CrossRef CAS PubMed.
- J. Parness and S. B. Horwitz, J. Cell Biol., 1981, 91, 479–487 CrossRef CAS.
- E. Nogales, S. Grayer Wolf, I. A. Khan, R. F. Ludueña and K. H. Downing, Nature, 1995, 375, 424–427 CrossRef CAS PubMed.
- B. Gigant, C. Wang, R. B. Ravelli, F. Roussi, M. O. Steinmetz, P. A. Curmi, A. Sobel and M. N. Knossow, Nature, 2005, 435, 519–522 CrossRef CAS PubMed.
- F. M. Ranaivoson, B. Gigant, S. Berritt, M. Joullié and M. Knossow, Acta Crystallogr., Sect. D: Biol. Crystallogr., 2012, 68, 927–934 CrossRef CAS.
- W. Wei, N. G. Ayad, Y. Wan, G.-J. Zhang, M. W. Kirschner and W. G. Kaelin, Nature, 2004, 428, 194–198 CrossRef CAS PubMed.
- J. Wang, D. D. Miller and W. Li, Drug Discovery Today, 2021, 27, 759–776 CrossRef.
- K. E. Arnst, Y. Wang, D.-J. Hwang, Y. Xue, T. Costello, D. Hamilton, Q. Chen, J. Yang, F. Park and J. T. Dalton, Cancer Res., 2018, 78, 265–277 CrossRef CAS PubMed.
- Z. Wang, J. Chen, J. Wang, S. Ahn, C.-M. Li, Y. Lu, V. S. Loveless, J. T. Dalton, D. D. Miller and W. Li, Pharm. Res., 2012, 29, 3040–3052 CrossRef CAS.
- Y. Izumi and S. Takagi, Res. Vet. Sci., 2019, 122, 1–6 CrossRef CAS.
- D. Bates and A. Eastman, Br. J. Clin. Pharmacol., 2017, 83, 255–268 CrossRef CAS.
- Y. Lu, J. Chen, M. Xiao, W. Li and D. D. Miller, Pharm. Res., 2012, 29, 2943–2971 CrossRef CAS.
- S. Zheng, Q. Zhong, M. Mottamal, Q. Zhang, C. Zhang, E. LeMelle, H. McFerrin and G. Wang, J. Med. Chem., 2014, 57, 3369–3381 CrossRef CAS.
- R. Zaninetti, S. V. Cortese, S. Aprile, A. Massarotti, P. L. Canonico, G. Sorba, G. Grosa, A. A. Genazzani and T. Pirali, ChemMedChem, 2013, 8, 633–643 CrossRef CAS.
- N. M. O'Boyle, M. Carr, L. M. Greene, O. Bergin, S. M. Nathwani, T. McCabe, D. G. Lloyd, D. M. Zisterer and M. J. Meegan, J. Med. Chem., 2010, 53, 8569–8584 CrossRef.
- J. Song, Q.-L. Gao, B.-W. Wu, T. Zhu, X.-X. Cui, C.-J. Jin, S.-Y. Wang, S.-H. Wang, D.-J. Fu and H.-M. Liu, Eur. J. Med. Chem., 2020, 203, 112618 CrossRef CAS.
- D.-J. Fu, J.-J. Yang, P. Li, Y.-H. Hou, S.-N. Huang, M. A. Tippin, V. Pham, L. Song, X. Zi and W.-L. Xue, Eur. J. Med. Chem., 2018, 157, 50–61 CrossRef CAS.
- D.-J. Fu, P. Li, B.-W. Wu, X.-X. Cui, C.-B. Zhao and S.-Y. Zhang, Eur. J. Med. Chem., 2019, 165, 309–322 CrossRef CAS.
- L. C. Klein-Junior, C. dos Santos Passos, A. P. Moraes, V. G. Wakui, E. L. Konrath, A. Nurisso, P.-A. Carrupt, C. M. A. de Oliveira, L. Kato and A. T. Henriques, Curr. Top. Med. Chem., 2014, 14, 1056–1075 CrossRef CAS PubMed.
- Q. Wang, K. E. Arnst, Y. Wang, G. Kumar, D. Ma, S. W. White, D. D. Miller, W. Li and W. Li, J. Med. Chem., 2019, 62, 6734–6750 CrossRef CAS.
- W. Qinghui, A. E. Kinsie, D. D. Miller and W. Li, Cancer Res., 2016, 76, 4848 CrossRef.
- S. Kapoor, S. Srivastava and D. Panda, Sci. Rep., 2018, 8, 1–12 CAS.
- R. Álvarez, C. Gajate, P. Puebla, F. Mollinedo, M. Medarde and R. Peláez, Eur. J. Med. Chem., 2018, 158, 167–183 CrossRef PubMed.
- D. W. McMillin, J. Delmore, J. Negri, L. Buon, H. M. Jacobs, J. Laubach, J. Jakubikova, M. Ooi, P. Hayden, R. Schlossman, N. C. Munshi, C. Lengauer, P. G. Richardson, K. C. Anderson and C. S. Mitsiades, Br. J. Haematol., 2011, 152, 420–432 CrossRef CAS.
- W.-G. Józefa, C. Ivo and K. Vladimír, Future Med. Chem., 2009, 1, 1561–1581 CrossRef.
- R. Zaninetti, S. V. Cortese, S. Aprile, A. Massarotti, P. L. Canonico, G. Sorba, G. Grosa, A. A. Genazzani and T. Pirali, ChemMedChem, 2013, 8, 633–643 CrossRef CAS.
- I. K. Lindamulage, H. Y. Vu, C. Karthikeyan, J. Knockleby, Y. F. Lee, P. Trivedi and H. Lee, Sci. Rep., 2017, 7, 10298 CrossRef PubMed.
- X. Wang, E. Wu, J. Wu, T. L. Wang, H. P. Hsieh and X. Liu, PLoS One, 2013, 8, e65686 CrossRef CAS.
- I. A. Gracheva, E. S. Shchegravina, H.-G. Schmalz, I. P. Beletskaya and A. Y. Fedorov, J. Med.
Chem., 2020, 63, 10618–10651 CrossRef CAS PubMed.
- Q. Wang, K. E. Arnst, Y. Wang, G. Kumar, D. Ma, S. W. White, D. D. Miller, W. Li and W. Li, J. Med. Chem., 2019, 62, 6734–6750 CrossRef CAS PubMed.
- S. Dastmalchi, M. Hamzeh-Mivehroud, T. Ghafourian and H. Hamzeiy, J. Mol. Graphics Modell., 2008, 26, 834–844 CrossRef CAS PubMed.
- S. Liu, W. Wei, Y. Li, X. Liu, X. Cao, K. Lei and M. Zhou, Eur. J. Med. Chem., 2015, 95, 473–482 CrossRef CAS PubMed.
- G. Baltayiannis and P. Karayiannis, J. Viral Hepatitis, 2014, 21, 753–761 CrossRef CAS.
|
This journal is © The Royal Society of Chemistry 2023 |
Click here to see how this site uses Cookies. View our privacy policy here.