DOI:
10.1039/D3RA02711B
(Paper)
RSC Adv., 2023,
13, 28743-28752
Carvacrol inhibits bacterial polysaccharide intracellular adhesin synthesis and biofilm formation of mucoid Staphylococcus aureus: an in vitro and in vivo study
Received
24th April 2023
, Accepted 25th September 2023
First published on 5th October 2023
Abstract
Staphylococcus aureus (S. aureus) is one of the important human pathogens and causes both superficial and systemic infections. More importantly, the formation of S. aureus biofilms, a main cause of its pathogenicity and drug resistance, has been a critical challenge in clinical treatment. Carvacrol, a plant-based natural product, has gained great interest for therapeutic purposes due to its effective biological activity with low cytotoxicity. The present study aimed to investigate the effect of carvacrol on anti-biofilm activity. Growth curve analysis showed that applying a sub-inhibitory concentration of carvacrol (4 μg mL−1) was not lethal to S. aureus SYN; however, the inhibition rate of biofilm formation was as high as 63.6%, and the clearance rate of mature biofilms was as high as 30.7%. In addition, carvacrol effectively reduced the production of biofilm-associated extracellular polysaccharides and showed no effect on eDNA release. Furthermore, qPCR analysis revealed that carvacrol significantly down-regulated the expression of icaA, icaB, icaC, agrA, and sarA (P < 0.05). The in vivo efficacy of carvacrol against biofilm infection was further verified with a biological model of G. mellonella larvae. The results showed that carvacrol was non-toxic to the larvae and can effectively increase the survival rate of the larvae infected with S. aureus strain SYN.
Introduction
Staphylococcus aureus (S. aureus) is an important zoonotic pathogen and has become a significant global health problem due to its multi-drug resistance. In addition to causing minor superficial skin infections, S. aureus may also cause life-threatening systemic infections such as pneumonia, sepsis, endocarditis, and toxic shock syndrome.1 A bacterial biofilm is a microbial community formed by the adhesion of bacterial colonies and extracellular matrix (ECM).2 Polysaccharide intracellular adhesin (PIA) is the main ECM in S. aureus biofilms.3 PIA mediates intercellular adhesion and forms tightly connected biofilms, which are produced by the intracellular adhesion (ica) operon.4 In addition to PIA, extracellular DNA (eDNA) and some surface proteins also contribute to biofilm stability.5 Moreover, eDNA may act as an electrostatic polymer in the early adhesion and maturation phases of biofilms,6 and stabilizes the structure of biofilms by linking PIA to biofilm-associated proteins and other components of biofilms.7
S. aureus is considered a major species of biofilm-associated infections.8 Most systemic S. aureus infections persist due to the in vivo formation of biofilms. Biofilm-protected bacterial cells are more resistant to most antibiotics and host defence system than their planktonic cells.9 It was reported that biofilms could cause a 100- to 1000-fold increase in antimicrobial tolerance.10 Biofilms may exert anti-microbial effects through multiple mechanisms including poor antibiotic permeability, genetic adaptation, slow growth rate, altered metabolism, persisters, matrix enzyme degradation, and horizontal gene transfer.9–12 Bacteria protected by biofilms require more antibiotics than planktonic bacteria, it is thus difficult to achieve the minimum antibiotic concentration in vivo to eradicate the bacteria underneath biofilms. Moreover, once a biofilm is established, it is difficult to eradicate.13 Therefore, to search for bioactive compounds that effectively inhibit and/or disrupt biofilm formation is critically important for medical applications.
Some essential oil extracted from plants are known to have pharmacological properties, such as antibacterial, antifungal, and antiviral.14 In addition, the natural products may play roles in the control of bacterial biofilm production and quorum sensing.15,16 Compared to antibiotics, some naturally occurring compounds may have improved biocompatibility and fewer side effects in humans and are considered as potential substitutes for synthetic antibiotics.17 Carvacrol (2-methyl-5-isopropylphenol) is a natural terpene compound and can be extracted from oregano and thyme.18 Previous studies reported that it could have antibacterial activity against Gram-positive and most Gram-negative bacteria, as well as some antifungal activity.19 It also shows potential in removing biofilms from different strains.20 However, the mechanism of carvacrol in anti-biofilm formation is unclear and has not been studied systematically. The aim of this study is thus to investigate its mechanism of action against S. aureus biofilms. Moreover, the in vivo anti-bacterial activity of carvacrol using a G. mellonella larvae model was investigated. The finding of the study may provide better understanding on the mode of action of carvacrol and also provides an experimental basis for further development of biofilm inhibitors based on carvacrol or other natural compounds.
Results
Antibacterial effect of carvacrol against S. aureus SYN
The antibacterial activity of carvacrol against S. aureus SYN was assessed by measuring MIC and MBC using broth microdilution method.21 The results showed that carvacrol inhibited the growth of S. aureus. Carvacrol at 32 μg mL−1 was found completely inhibited the growth of S. aureus SYN, and thus the MIC was 32 μg mL−1. LB solid agar plates also showed an MBC of 32 μg mL−1 (Fig. 1).
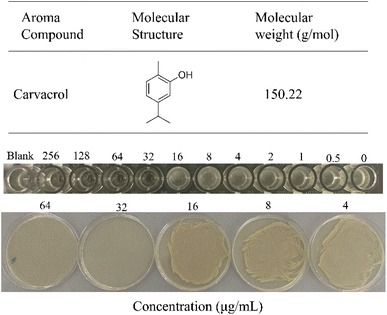 |
| Fig. 1 Chemical structure of carvacrol and its minimum inhibitory concentration (MIC) and minimum bactericidal concentration (MBC) against S. aureus SYN. | |
Time-killing growth curve
The antibacterial activity of carvacrol was investigated. The effect of carvacrol at 1/2 MIC (16 μg mL−1), 1/4 MIC (8 μg mL−1), 1/8 MIC (4 μg mL−1) concentrations on the growth and viability of S. aureus SYN was evaluated. The results show that the growth of the strain under the treatment at 4 μg mL−1 carvacrol was relatively consistent with that of the control group (untreated) (Fig. 2). The strain treated with 8 μg mL−1 carvacrol exhibited a certain degree of delay in the logarithmic phase of growth. The number of bacteria grown is obviously less than that of the control group. Under the treatment condition at 16 μg mL−1 carvacrol, there was no observable bacterial growth.
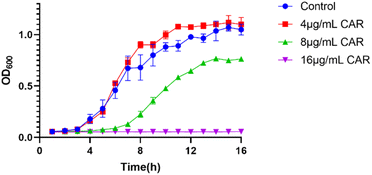 |
| Fig. 2 Growth curves of S. aureus SYN under different concentrations of carvacrol (CAR: carvacrol). | |
Determination of the composition of S. aureus SYN biofilm
To investigate the mechanism of action of carvacrol exerted on the ECM of biofilms, we first determined the main components of the ECM of S. aureus SYN. We therefore conducted the enzymatic digestion assay to find out the main components of the ECM of the S. aureus SYN biofilm. The results were shown in Fig. 3. It was found that the amount of biofilm formation after PBS treatment was not significantly different from the control group without any treatment. Nonetheless, we found that 23.7% of biofilms were disintegrated after Proteinase K treatment, 39.2% of biofilms were disintegrated after DNase I treatment, and 71.6% of biofilms were disintegrated after sodium periodate treatment. Based on these results, it could be concluded that the ECM of S. aureus SYN biofilm is mainly composed of PIA, and followed by extracellular DNA, while the least component is extracellular protein.
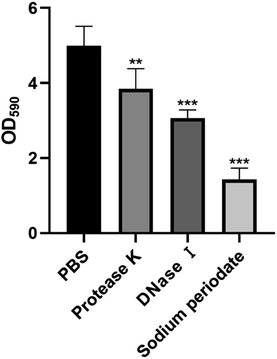 |
| Fig. 3 Enzymatic hydrolysis assays of S. aureus SYN and component analysis of the ECM. | |
Inhibitory effects of carvacrol on S. aureus SYN biofilm
The inhibitory effect of carvacrol at 4 and 8 μg mL−1 on S. aureus SYN biofilm formation at different time interval was measured by microdilution and crystal violet staining assays. The results were shown in Fig. 4. It was found that the amount of biofilm formation under each concentration was significantly lower than that of the control (untreated blank) at all experiment time points. In addition, the anti-biofilm activity of carvacrol on S. aureus SYN was concentration-dependent. A higher concentration of carvacrol treatment was able to better inhibit biofilm formation.
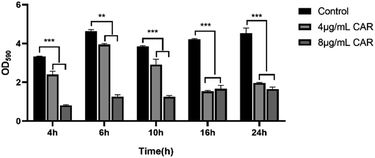 |
| Fig. 4 Inhibition of biofilm formation following treatment with different concentrations of carvacrol at different time points (CAR: carvacrol). | |
Scavenging effects of carvacrol on S. aureus SYN mature biofilm
The ability of carvacrol in scavenging mature S. aureus SYN biofilm was investigated. The results were shown in Fig. 5. Compared to the control (untreated blank), the clearance (%) of the mature biofilms under the treatment condition with 4 μg mL−1, 8 μg mL−1, 16 μg mL−1, and 32 μg mL−1 carvacrol was found able to reach 30.7%, 68.6%, 76.2%, and 93.7%, respectively. The results indicate the scavenging effect is concentration-dependent and may also support that carvacrol exhibits scavenging effect against the mature biofilms.
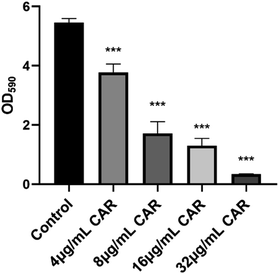 |
| Fig. 5 Scavenging effects of carvacrol on mature biofilms (CAR: carvacrol). | |
Effects of sub-inhibitory concentrations of carvacrol on PIA
Based on the experimental results thus far, we found that carvacrol at the sub-inhibitory concentration (4 μg mL−1) did not inhibit bacterial growth (Fig. 2) but it did significantly inhibit the biofilm production (Fig. 4) and disrupt the mature biofilm (Fig. 5). We thus used the phenol-concentrated sulfuric acid method and FITC-ConA to determine the effect of carvacrol (4 μg mL−1) on S. aureus SYN PIA production. The glucose standard curve, shown in Fig. 6A, was established as: Y = 0.0145X − 0.02848. The correlation coefficient was found to be R2 = 0.9927, indicating a good linear relationship between absorbance and glucose concentration. Then, the effect of carvacrol in inhibiting PIA synthesis at sub-inhibitory concentration was investigated. Compared with the control group, a significantly reduced fluorescence signal was observed (Fig. 6B), indicating that carvacrol could inhibit PIA production. In addition, S. aureus SYN after treatment with 4 μg mL−1 carvacrol, the change of biofilm structure from dense to loose was observed under fluorescence microscope at all time points (Fig. 6C). The results may support that carvacrol could possibly inhibit PIA formation at sub-inhibitory concentration.
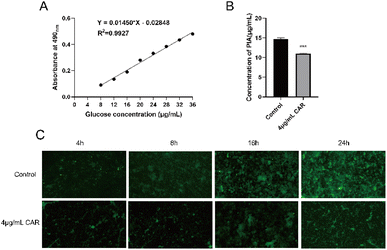 |
| Fig. 6 (A) Glucose standard curve; (B) effect of sub-inhibitory carvacrol on production of PIA; (C) fluorescence microscope detection of the effect of sub-inhibitory carvacrol on PIA at different time points (CAR: carvacrol). | |
Effects of sub-inhibitory concentration of carvacrol on eDNA
During the biofilm formation, eDNA inside the membrane is released from dead bacteria. Importantly, eDNA plays a key role in the adhesion of bacteria to the carrier surface and between bacteria. Compared to the control group, carvacrol at sub-inhibitory concentration showed no effect on eDNA release and also no difference was observed in autolysis curves between the two strains (Fig. 7). Therefore, the results indicate that carvacrol may have no effect on eDNA release.
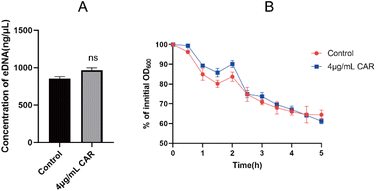 |
| Fig. 7 (A) The effect of sub-inhibitory concentration of carvacrol on the release of eDNA; (B) autolysis curve before and after sub-inhibitory concentration carvacrol treatment (CAR: carvacrol). | |
Scanning electron microscopy (SEM) assay
SEM can show more intuitively the effect of carvacrol on the structure of the biofilm, and the results are shown in Fig. 8. The bacteria in the control group were connected into a bunch of grapes, wrapped together to form a dense biofilm, and a large number of ECM adhered to the surface of the bacteria. After the treatment of sub-inhibitory concentration of carvacrol, the amount of bacteria was not significantly reduced, and some of the biofilm still remained, but the degree of mutual adhesion between the bacteria decreased. The ECM became dispersed (Fig. 8, red arrow) after treatment with carvacrol when compared to the control group (Fig. 8). It can be seen that the sub-inhibitory concentration of carvacrol has a good inhibitory effect on the formation of biofilm, and can destroy the structure of ECM to inhibit the formation of biofilm.
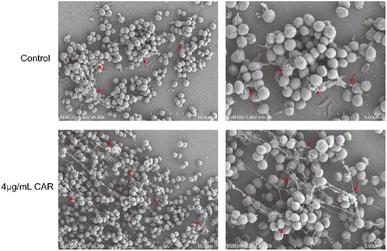 |
| Fig. 8 SEM observation of the effect of sub-inhibitory concentration of carvacrol on biofilm structure (CAR: carvacrol). The red arrow indicated the difference of ECM between treatment with or without carvacrol. | |
Real-time PCR (RT-PCR) analysis
We further determined the differential expression of biofilm-associated genes before and after the treatment with 4 μg mL−1 carvacrol (Fig. 9). The expression of icaA, icaB, and icaC in the ica locus encoding PIA was down-regulated by 3.43-, 1.82-, and 2.02-fold, respectively. There was no observed effect on icaD. In addition, the expression of agrA in the quorum sensing system was down-regulated by 3.46-fold. Moreover, expression of the staphylococcal co-regulator SarA was down-regulated by 2.7-fold. These results suggest that carvacrol may reduce biofilm formation by down-regulating the biofilm-forming genes including icaA, icaB, and icaC. As a result, it inhibits biofilm formation and reduces the expression of the icaADBC operon by down-regulating the expression of biofilm-forming regulators including agrA and sarA, which in turn exerts an anti-biofilm effect. On the other hand, there is an interesting result of the upregulation of icaR. IcaR encodes a transcriptional repressor that downregulates the expression of the icaADBC operon, which is responsible for PIA synthesis.22 Therefore, the inhibition of PIA formation by carvacrol may due to upregulation of icaR.
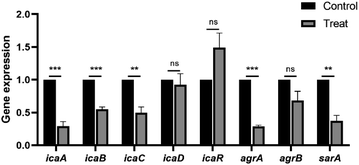 |
| Fig. 9 Changes in gene expression related to biofilm formation and regulation before and after the treatment with carvacrol at sub-inhibitory concentration. | |
G. mellonella larvae infection assay
G. mellonella larvae have been widely used as an alternative model for non-mammalian models to assess the in vivo toxicity and efficacy of anti-infective drugs.23,24 Our results showed that 96.7% survival was observed in larvae injected with 4 μg mL−1 carvacrol after 120 h, which was similar to the control group (blank) (Fig. 10). This may indicate that carvacrol is not toxic to the larvae and does not cause observable damage to the larvae. The survival rate of S. aureus SYN-infected larvae was only 13.3% after 120 h, while the survival rate of carvacrol-treated larvae was significantly increased to 83.3% after 120 h. The difference was statistically significant and also indicated that carvacrol could effectively rescue larvae from S. aureus SYN infection and it was not toxic to larvae.
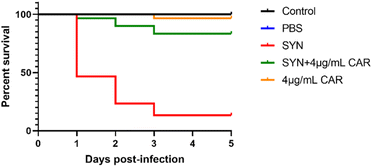 |
| Fig. 10 Evaluation of the toxicity and efficacy of sub-inhibitory carvacrol in vivo using the G. mellonella larvae models (CAR: carvacrol). | |
Discussion
Biofilm is one of the preferred growth states of bacteria which exists in more than 90% of bacteria, and acts as a survival strategy that allows the organism to adapt to almost all survival environments.25 Currently, the main strategies against biofilms include inhibition or prevention of initial biofilm formation and dispersion or removal of mature biofilms.26 The early stage of biofilm formation, when self-protection mechanisms are still incomplete, is the best time for most antibiotics to exert anti-biofilm activity.27 Therefore, rapid and accurate detection of biofilm formation at an early stage can effectively control biofilm-infected diseases.
To verify the role of a plant-based small molecule, carvacrol, in inhibiting biofilm formation, absorbance and microscopic observations were performed. The results of crystalline violet staining showed that carvacrol possessed a strong inhibitory effect on the biofilm in the early stage and the inhibitory effect was found dose-dependent. The mature biofilm often makes it difficult to counteract antibiotics because of the protective effect of its physical barrier and anti-immune clearance.28 Our results on scavenging effects of carvacrol showed that carvacrol at 1× MIC concentration cleared up to 93.75% of the mature biofilm, indicating that carvacrol could penetrate and destroy mature biofilms. This property most likely makes carvacrol exerting good antibacterial effects.
PIA, the main component of the ECM, is mainly responsible for intercellular aggregation, promotion of bacterial adherence to the carrier surface, and immune escape, making it the decisive factor in the adhesive aggregation phase of the biofilm. We showed that carvacrol at sub-inhibitory concentration inhibited PIA synthesis. Furthermore, using FITC-ConA to visualize the content of the PIA in biofilms by fluorescence microscopy, we found that there was a large amount of fluorescent signal and dense structure in the mature biofilm at 24 h in the control group, indicating that there was a large amount of PIA formation. We observed a significant reduction in the fluorescence signal after the treatment with a sub-inhibitory concentration of carvacrol. In addition, the observed loose structure of biofilm may indicate the decrease in PIA formation. These results suggest that carvacrol may have effects on reducing PIA synthesis. Importantly, eDNA not only affects bacterial aggregation, but also interacts with PIA to maintain the stability of biofilm structures, to enhance the physical strength of the biofilm, and to improve the resistance of the bacterium against biological stress.6 Nonetheless, eDNA extraction by phenol-chloroform extraction revealed that the treatment with carvacrol at sub-inhibitory concentration had no effect on eDNA release.
The biofilm is a complex microecological structure. Each step of biofilm formation is controlled by multiple strict regulatory systems or regulatory factors. We also studied the possible role of carvacrol on the regulators of biofilm formation. The key regulatory genes in biofilm formation include sarA, agrA, and agrB. Importantly, sarA is a global regulator of biofilm formation and binds to the ica promoter sequence to upregulate ica expression and to promote biofilm formation.29 The disruption of the quorum sensing system has also been a hot topic in anti-biofilm research. AgrA and AgrB, the main regulatory molecules of the quorum sensing system. Inhibiting their signaling mainly affects the maturation stage of the biofilm, which reduces MRSA resistance to antimicrobial drugs and decreases α-toxin production and abscess formation.30 In this study, we found that carvacrol at sub-inhibitory concentration exhibited inhibitory effects on the expression of sarA and agrA. Consequently, carvacrol may reduce the production of PIA by inhibiting the expression of icaA, icaB, icaC, sarA and agrA genes, prevent the mutual adhesion of the bacteria and affects the morphology of the bacteria, and thus destabilizes the biofilm.
The in vivo study is also key to translating in vitro results into clinical therapeutic applications. G. mellonella larvae have been widely used as surrogate host models for a variety of human bacterial and fungal pathogens,31 and also is useful for assay microbial virulence or to test the in vivo efficacy and toxicity of antimicrobial compounds.32 Since the nodules produced by larvae during S. aureus infection are structurally and functionally similar to abscesses commonly found in S. aureus skin and soft tissue infections,33 we thus used a larval infection model to test the feasibility of using sub-inhibitory carvacrol as an antimicrobial agent. The results showed that the survival rate of the infected group injected with S. aureus SYN was only 13.3% after 5 days. The blackened nodules could occur at the inoculation site after infection, probably due to uncontrollable phenol oxidase activation caused by the uncontrollable bacterial burden.34 The survival rate of the carvacrol-treated group was increased to 83.3% after 5 days, which was significantly higher compared to that of the infected group. The blackened nodules also did not appear at the inoculation site. The survival rate of the carvacrol injection group alone was 96.7%, indicating that carvacrol had no toxic effect on the larvae and effectively increased their survival rate. However, further studies are needed to assess the safety and efficacy of oral administration of carvacrol in humans.
S. aureus is a pathogenic bacterium that is not resistant to carvacrol. So far, there are a few studies about antibiofilm activity of carvacrol against S. aureus. Knowles et al. showed that continuous exposure of S. aureus NCTC 10788 to non-biocidal concentrations of carvacrol disrupted normal biofilm development, prevented the accumulation of protein clusters, arrested at the microcolony stage.35 Selvaraj et al. found that carvacrol can inhibit the growth and biofilm formation of MRSA (ATCC-33591) at the concentration of 150 μg mL−1 and 75 μg mL−1 respectively. The mechanism study revealed that carvacrol mitigate biofilm formation via targeting the SarA protein.21 We showed here that carvacrol disrupts pre-formed S. aureus SYN biofilm and interferes with biofilm formation during its growth at the concentration of 4 μg mL−1. And the antibacterial susceptibility assay showed that 16 μg mL−1 of carvacrol can inhibit the growth of S. aureus SYN. The cause of this may be due to the synergistic or individual action of many factors, including inhibition of certain enzymes and resistance mechanisms, such as efflux pumps, elimination of the biofilm and damage to the cell wall.36 Due to its hydrophobicity, carvacrol interacts with the lipid bilayer of the cell membrane, causing the loss of cellular material such as ions, ATP and nucleic acids.37,38 Also carvacrol can diffuse through the polysaccharide matrix of biofilm and destabilize them.39 Modification of bacterial membranes may prevent secretion of toxins due to the effect of carvacrol on plasma membrane transmembrane transport processes, thus limiting the release of toxins into the external environment.40,41
However, the efficacy of carvacrol may vary considerably depending on the tested strain, but although the inhibitory effect varied between strains, the level of biofilm formation was generally attenuated at sub-inhibitory concentrations of carvacrol.39 Finally, carvacrol can be used in combination with conventional antibiotics to find synergies and improve bacterial susceptibility.
Experiment
Bacteria strains and growth conditions
The clinical mucoid S. aureus SYN (high biofilm-producing strain) used in this study was isolated from the renal pus of patients with kidney stones and identified using an automated bacterial identification and drug sensitivity analysis system (BioMerieux, VITEK-2 Compact, France). The genome of S. aureus SYN was sequenced and the whole genome sequence was submitted to GenBank (accession number SAMN24425091). Bacteria were incubated overnight in Tryptone Soy broth (TSB; Rishui Biotech, China) at 37 °C with shaking at 200 rpm min−1. The strain subjected to antimicrobial susceptibility tests was cultured in Mueller Hinton broth (MH; Rishui Biotech, China) at 37 °C with shaking at 200 rpm min−1.
Phytocompound
Carvacrol (50 mg mL−1, 98%) was purchased from Sigma-Aldrich (St. Louis, MO, USA) and prepared as 2560 μg mL−1 stock solution in DMSO and stored at 4 °C.
MIC and MBC
The minimum inhibitory concentration (MIC) and minimum bactericidal concentration (MBC) of carvacrol against S. aureus SYN were determined by microbroth dilution assays. Added the bacterial suspension to double dilute the carvacrol. Wells with untreated cells were used as negative control. The wells containing drug but no bacterial cells were used as blank control. The assay plate was incubated at 37 °C for 24 h. MIC was defined as the minimum concentration required to inhibit bacterial growth. Thereafter, 10 μL per well were spread on LB plates and incubated at 37 °C for 24 h. MBC was defined as the minimum concentration required in the absence of bacterial growth.
Time-killing growth curve assay
Bacterial suspension was added to TSB containing different concentrations of carvacrol (final concentration, 4, 8, and 16 μg mL−1) in a 6-well plate and placed in an incubator at 37 °C with shaking at 200 rpm. Next, three replicate wells were set up and the wells without carvacrol were used as a negative control. After that, the absorbance at an optical density of 600 nm (OD600) was measured every hour to generate the time-killing growth curve.42
Enzymatic hydrolysis assay
An overnight culture of S. aureus was diluted 200-fold with TSB, of which 200 μL were added to the wells of a 96-well polystyrene microtiter plate and incubated for 24 h at 37 °C. After maturation of the culture, biofilms were washed gently twice with sterile phosphate-buffered saline (PBS, 0.1 M, pH 7.4). Next, 200 μL of Protease K (Solarbio, China), DNase I (Coolaber, China), sodium periodate (MERCK, China), and PBS were added and then cultured at 37 °C for 2 h; the control group was not subjected to any treatment.43 After 2 h, the solution was discarded and methanol was added to fix the biofilms for 15 min. Subsequently, the cell culture before being washed off with PBS, 0.5% of crystal violet was added to stain for 20 min. Then, 33% of glacial acetic acid was added to dissolve the crystal violet. The absorbance was then read at 590 nm.
Biofilm formation inhibition assay
Here, 100 μL of bacteria suspension and 100 μL of TSB containing different concentrations of carvacrol (final concentration at 4 and 8 μg mL−1, respectively) were added to a 96-well plate. The negative control group was not treated with carvacrol. Biofilms formed following static incubation at 37 °C for 24 h. After that, crystal violet staining was performed.44
Mature biofilm scavenging assay
Bacterial suspensions were added to a 96 well plate and incubated at 37 °C for 24 h until biofilms matured. After 24 h, the culture solution was discarded and 200 μL of TSB containing carvacrol (final concentration: 4, 8, 16 and 32 μg mL−1) was added. The negative control group was not treated with carvacrol. After incubation for 24 h, crystal violet staining was performed.
Effects of sub-inhibitory concentration of carvacrol on PIA
First, 20 mg of standard glucose was accurately weighed before adding ddH2O to make up to 500 mL. Next, 0.4, 0.6, 0.8, 1.0, 1.2, 1.4, and 1.8 mL of glucose solutions were aspirated. Each sample was supplemented with ddH2O to 2.0 mL. Then, 1 mL of 6% (v/v) phenol and 5 mL of H2SO4 were added and incubated for 30 min in dark before measuring the absorbance at 490 nm. The standard curve was established based on the glucose concentration and optical density values.
The bacterial suspensions and TSB containing different concentrations of carvacrol (final concentration was 4 μg mL−1) were then added to a 6-well plate. The untreated wells were used as the negative control and were incubated at 37 °C for 24 h. After that, cells were gently wash with sterile PBS twice. Before heating samples at 70 °C in a water bath for 1 h, 3 mL of TE buffer was used to resuspend the biofilm. Samples were then centrifuged at 12
000g for 10 min. After centrifugation, the bacteria were resuspended in 3 mL of 3% (v/v) EDTA and centrifuged at 12
000g for 12 min. The supernatant was then filtered with a microporous membrane. Then, 1 mL of filtrate was mixed with 1 mL of ddH2O, 1 mL of 6% (v/v) phenol and 5 mL of H2SO4. After that, it was incubated for 30 min in dark. The absorbance of the sample supernatant was then measured at 490 nm.45
Fluorescence microscopy assay
The cell climbing tablets were placed into a 6-well plate containing a sub-inhibitory concentration of carvacrol and incubated for 24 h at 37 °C, using the untreated well as a positive control. Sterile PBS was used to wash off floating bacteria on the surface. Next, 2.5% (v/v) of glutaraldehyde was added to fix at 4 °C for 20 min, followed by FITC-ConA (MERCK, China) incubation at 4 °C in dark for 40 min. Samples were then observed under a fluorescence microscope (Zeiss, Axioscope5, Germany).46,47
Effect of sub-inhibitory concentration of carvacrol on eDNA
Bacterial suspensions and TSB containing different concentrations of carvacrol (final concentration was 4 μg mL−1) were added to a 6-well plate. Untreated wells were used as the positive control. These samples were incubated at 37 °C for 24 h. After 24 h, the culture solution was discarded and gently washed twice with sterile PBS. After that, 1 mL TEN buffer was added to each well. Biofilms that adhered to the bottom of the plate were resuspended and transferred to a tube. After centrifugation at 4 °C and 12
000g for 5 min, 500 μL of supernatant was taken and added 300 μL TE buffer. Subsequently, eDNA was extracted once with equal amounts of phenol/chloroform/isoamyl alcohol (25
:
24
:
1) and then extracted again with chloroform/isoamyl alcohol (24
:
1). Samples were then mixed with three volumes of precooled anhydrous ethanol and 1/10 volume of precooled sodium acetate and stored at −20 °C. The next day, eDNA was precipitated with ethanol and collected by centrifugation at 4 °C, 15
000g for 20 min. Samples were washed and precipitated with 70% precooled ethanol, air dried, and dissolved in 20 μL TE buffer.48 A Nanodrop 2000 spectrophotometer (Thermo Fisher Scientific, USA) was used to detect and measure eDNA concentration.
Scanning electron microscopy (SEM) assay
The silica was incubated overnight in a six-well plate containing the samples. After incubation, rinsed the samples three times with pre-cooled sterile PBS for 15 min each time. Added 1 mL of 2.5% (vol/vol) glutaraldehyde and fixed at 4 °C for 8 h. After fixation, the samples were rinsed three times with sterile PBS for 10 min each time. Next, ethanol gradient dehydration was performed using 30%, 50%, 70%, 80%, 90%, and 100% (v/v) ethanol, and finally treated with isoamyl acetate for 15 min. The samples were dried in a critical point dryer. Then, the samples are attached to metallic stubs using carbon stickers and sputter-coated with gold for 30 s. Observe and take images with scanning electron microscope (Zesis, Merlin, Germany).
Quantitative real-time PCR (qRT-PCR) analysis
Total RNA was extracted from carvacrol-treated (final concentration was 4 μg mL−1) and untreated cultured bacteria using RNAiso Plus (TaKaRA, China). RNA was reverse transcribed into cDNA using the PrimeScript II 1st Strand cDNA Synthesis Kit (TaKaRa Biotech, China), and then was analyzed by qRT-PCR using the TB Green PCR reagents (TB Green Premix Ex Taq; TaKaRa Biotech, China). Using gyrB (DNA gyrase subunit B gene) as the housekeeping gene, the cyclic threshold (Ct) values of all tested genes were normalized. The transcription level expression of biofilm related genes (icaA, icaB, icaC, icaD, icaR, sarA, agrA, and agrB) were detected. The 2(−ΔΔCt) quantitative expression method was used for analyses.49 The primers used in this study were shown in Table 1. Assays were performed in biological triplicates with three technical replicates.
Table 1 Primers used for qRT-PCR analysis
Primer |
Sequence (5′ → 3′) |
RT-gyrB-F |
GGTGGCGACTTTGATCTAGC |
RT-gyrB-R |
TTATACAACGGTGGCTGTGC |
RT-icaA-F |
TTTCGGGTGTCTTCACTCTAT |
RT-icaA-R |
CGTAGTAATACTTCGTGTCCC |
RT-icaB-F |
ATACCGGCAACTGGGTTTAT |
RT-icaB-R |
TGCAAATCGTGGGTATGTGT |
RT-icaC-F |
CTTGGGTATTTGCACGCATT |
RT-icaC-R |
GCAATATCATGCCGACACCT |
RT-icaD-F |
ACCCAACGCTAAAATCATCG |
RT-icaD-R |
GCGAAAATGCCCATAGTTTC |
RT-icaR-F |
CGCCTGAGGAATTTTCTG |
RT-icaR-R |
GGATGCTTTCAAATACCAAC |
RT-agrA-F |
GTGAAATTCGTAAGCATGACCCAGTTG |
RT-agrA-R |
TGTAAGCGTGTATGTGCAGTTTCTAAAC |
RT-agrB-F |
GCCCATTCCTGTGCGACTTA |
RT-agrB-R |
TGGGCAAATGGCTCTTTGATG |
RT-sarA-F |
TTGTTTTCGCTGATGTAT |
RT-sarA-R |
CAATGGTCACTTATGCTG |
Survival assay
G. mellonella larvae weighing between 0.2 and 0.4 g were randomly selected and 20 μL of corresponding solution was injected per larva with one group for every 10 larvae using 50 μL microinjector (HAMILTON, Swiss). Three parallel experimental groups were included. The following analysis groups were tested: control group (without any treatment), normal saline group (injected with normal saline), infected group (injected with bacterial suspension (final concentration was 0.5 × 107 CFU mL−1)), compound group (injected with carvacrol (final concentration was 4 μg mL−1)) treatment group (injected with bacterial suspension containing carvacrol (final concentration was 4 μg mL−1)). After injection, samples were cultured at a constant temperature of 37 °C in a dark environment. The number of surviving cells in each group was recorded every 24 h for a total of 120 h. The percentage of survival was calculated and plotted as a graph.50
Statistical analysis
The experimental results were statistically analyzed and plotted using GraphPad Prism 8.0 software (GraphPad Software, San Diego, California USA). The experimental data were expressed as mean ± standard deviation (x ± s). An independent sample t-test was used to compare data between the two groups, and one-way analysis of variance (ANOVA) was used to compare data between multiple groups. Kaplan Meier was used for survival curve analysis, and Log rank test was used for statistical significance evaluation; P < 0.05 indicates a statistically significant difference, *P < 0.05, **P < 0.01, ***P < 0.001.
Conclusions
In conclusion, carvacrol was found to show good antibacterial effects against S. aureus, significantly inhibiting the biofilm formation of S. aureus. The in vivo analysis using the G. mellonella larvae model system further validated the anti-infective potential of carvacrol against S. aureus. This study demonstrated the potential of using carvacrol as an effective and low-toxic agent to treat S. aureus biofilm-associated infections.
Author contributions
Conceptualization, project administration, and supervision: F. C. and W. C. Y.; methodology, software: Q. P., X. H. T. and W. Y. D.; formal analysis, data curation, validation: Q. P., Z. L. Z., T. Z., S. N. L., X. P. Q. and J. Y. Y.; writing—original draft: Q. P., X. H. T. and W. Y. D.; writing—review and editing: Q. P., X. H. T., W. Y. D., Z. L. Z., T. Z., S. N. L., J. Y. Y., X. P. Q., F. C. and W. C. Y. submission: Q. P., F. C. and W. C. Y. All authors have read and agreed to the published version of the manuscript.
Conflicts of interest
There are no conflicts to declare.
Acknowledgements
This work was supported by the Natural Science Foundation of Guangdong Province (Grant No. 2021A1515011360), Guangzhou Science and Technology Project (Grant No. 202102080469), Guangzhou Health Science and Technology Project (Grant No. 2020A010015), Medical Scientific Research Foundation of Guangdong Province (Grant No. C2021077).
References
- S. Y. C. Tong, J. S. Davis, E. Eichenberger, T. L. Holland and V. G. Fowler Jr, Staphylococcus aureus Infections: Epidemiology, Pathophysiology, Clinical Manifestations, and Management, Clin. Microbiol. Rev., 2015, 28, 603–661, DOI:10.1128/cmr.00134-14.
- L. Karygianni, Z. Ren, H. Koo and T. Thurnheer, Biofilm Matrixome: Extracellular Components in Structured Microbial Communities, Trends Microbiol., 2020, 28, 668–681, DOI:10.1016/j.tim.2020.03.016.
- H. S. Joo and M. Otto, Molecular basis of in vivo biofilm formation by bacterial pathogens, Chem. Biol., 2012, 19, 1503–1513, DOI:10.1016/j.chembiol.2012.10.022.
- D. Mack, W. Fischer, A. Krokotsch, K. Leopold, R. Hartmann, H. Egge and R. Laufs, The intercellular adhesin involved in biofilm accumulation of Staphylococcus epidermidis is a linear beta-1,6-linked glucosaminoglycan: purification and structural analysis, J. Bacteriol., 1996, 178, 175–183, DOI:10.1128/jb.178.1.175-183.1996.
- S. Y. Tong, J. S. Davis, E. Eichenberger, T. L. Holland and V. G. Fowler Jr, Staphylococcus aureus infections: epidemiology, pathophysiology, clinical manifestations, and management, Clin. Microbiol. Rev., 2015, 28, 603–661, DOI:10.1128/cmr.00134-14.
- D. Campoccia, L. Montanaro and C. R. Arciola, Extracellular DNA (eDNA). A Major Ubiquitous Element of the Bacterial Biofilm Architecture, Int. J. Mol. Sci., 2021, 22(16), 9100, DOI:10.3390/ijms22169100.
- J. T. Blakeman, A. L. Morales-García, J. Mukherjee, K. Gori, A. S. Hayward, N. J. Lant and M. Geoghegan, Extracellular DNA Provides Structural Integrity to a Micrococcus luteus Biofilm, Langmuir, 2019, 35, 6468–6475, DOI:10.1021/acs.langmuir.9b00297.
- U. Romling and C. Balsalobre, Biofilm infections, their resilience to therapy and innovative treatment strategies, J. Intern. Med., 2012, 272, 541–561, DOI:10.1111/joim.12004.
- T.-F. Mah, Biofilm-specific antibiotic resistance, Food Res. Int., 2012, 7, 1061–1072, DOI:10.2217/fmb.12.76.
- I. Olsen, Biofilm-specific antibiotic tolerance and resistance, Eur. J. Clin. Microbiol. Infect. Dis., 2015, 34, 877–886, DOI:10.1007/s10096-015-2323-z.
- T. F. Mah and G. A. O'Toole, Mechanisms of biofilm resistance to antimicrobial agents, Trends Microbiol., 2001, 9, 34–39, DOI:10.1016/s0966-842x(00)01913-2.
- N. K. Archer, M. J. Mazaitis, J. W. Costerton, J. G. Leid, M. E. Powers and M. E. Shirtliff, Staphylococcus aureus biofilms Properties, regulation and roles in human disease, Virulence, 2011, 2, 445–459, DOI:10.4161/viru.2.5.17724.
- H. Wu, C. Moser, H.-Z. Wang, N. Hoiby and Z.-J. Song, Strategies for combating bacterial biofilm infections, Int. J. Oral Sci., 2015, 7, 1–7, DOI:10.1038/ijos.2014.65.
- S. Gao, G. Liu, J. Li, J. Chen, L. Li, Z. Li, X. Zhang, S. Zhang, R. F. Thorne and S. Zhang, Antimicrobial Activity of Lemongrass Essential Oil (Cymbopogon flexuosus) and Its Active Component Citral Against Dual-Species Biofilms of Staphylococcus aureus and Candida Species, Front. Cell. Infect. Microbiol., 2020, 10 DOI:10.3389/fcimb.2020.603858.
- A. Sharifi, A. Mohammadzadeh, T. Z. Salehi and P. Mahmoodi, Antibacterial, antibiofilm and antiquorum sensing effects of Thymus daenensis and Satureja hortensis essential oils against Staphylococcus aureus isolates, J. Appl. Microbiol., 2018, 124, 379–388, DOI:10.1111/jam.13639.
- R. Hakimi Alni, K. Ghorban and M. Dadmanesh, Combined effects of Allium sativum and Cuminum cyminum essential oils on planktonic and biofilm forms of Salmonella typhimurium isolates, 3 Biotech, 2020, 10, 315, DOI:10.1007/s13205-020-02286-2.
- K. Winska, W. Maczka, J. Lyczko, M. Grabarczyk, A. Czubaszek and A. Szumny, Essential Oils as Antimicrobial Agents - Myth or Real Alternative?, Molecules, 2019, 24(11), 2130, DOI:10.3390/molecules24112130.
- S. A. Burt, V. T. Ojo-Fakunle, J. Woertman and E. J. Veldhuizen, The natural antimicrobial carvacrol inhibits quorum sensing in Chromobacterium violaceum and reduces bacterial biofilm formation at sub-lethal concentrations, PLoS One, 2014, 9, e93414, DOI:10.1371/journal.pone.0093414.
- S. Roller and P. Seedhar, Carvacrol and cinnamic acid inhibit microbial growth in fresh-cut melon and kiwifruit at 4 degrees and 8 degrees C, Lett. Appl. Microbiol., 2002, 35, 390–394, DOI:10.1046/j.1472-765x.2002.01209.x.
- M. Walczak, M. Michalska-Sionkowska, D. Olkiewicz, P. Tarnawska and O. Warżyńska, Potential of Carvacrol and Thymol in Reducing Biofilm Formation on Technical Surfaces, Molecules, 2021, 26(9), 2723, DOI:10.3390/molecules26092723.
- A. Selvaraj, A. Valliammai, P. Muthuramalingam, A. Priya, M. Suba, M. Ramesh and S. K. Pandian, Carvacrol Targets SarA and CrtM of Methicillin-Resistant Staphylococcus aureus to Mitigate Biofilm Formation and Staphyloxanthin Synthesis: An In Vitro and In Vivo Approach, ACS Omega, 2020, 5, 31100–31114, DOI:10.1021/acsomega.0c04252.
- Q. Peng, X. Tang, W. Dong, N. Sun and W. Yuan, A Review of Biofilm Formation of Staphylococcus aureus and Its Regulation Mechanism, Antibiotics (Basel), 2022, 12(1), 12, DOI:10.3390/antibiotics12010012.
- C. J.-Y. Tsai, J. M. S. Loh and T. Proft, Galleria mellonella infection models for the study of bacterial diseases and for antimicrobial drug testing, Virulence, 2016, 7, 214–229, DOI:10.1080/21505594.2015.1135289.
- E. Allegra, R. W. Titball, J. Carter and O. L. Champion, Galleria mellonella larvae allow the discrimination of toxic and non-toxic chemicals, Chemosphere, 2018, 198, 469–472, DOI:10.1016/j.chemosphere.2018.01.175.
- L. Lu, W. Hu, Z. Tian, D. Yuan, G. Yi, Y. Zhou, Q. Cheng, J. Zhu and M. Li, Developing natural products as potential anti-biofilm agents, Chin. Med., 2019, 14(11) DOI:10.1186/s13020-019-0232-2.
- M. Bhattacharya, D. J. Wozniak, P. Stoodley and L. Hall-Stoodley, Prevention and treatment of Staphylococcus aureus biofilms, Expert Rev. Anti-Infect. Ther., 2015, 13, 1499–1516, DOI:10.1586/14787210.2015.1100533.
- D. Cue, J. M. Junecko, M. G. Lei, J. S. Blevins, M. S. Smeltzer and C. Y. Lee, SaeRS-Dependent Inhibition of Biofilm Formation in Staphylococcus aureus Newman, Plos One, 2015, 10(4), e0123027, DOI:10.1371/journal.pone.0123027.
- Q. Ji, P. J. Chen, G. Qin, X. Deng, Z. Hao, Z. Wawrzak, W. S. Yeo, J. W. Quang, H. Cho and G. Z. Luo, et al., Structure and mechanism of the essential two-component signal-transduction system WalKR in Staphylococcus aureus, Nat. Commun., 2016, 7, 11000, DOI:10.1038/ncomms11000.
- M. A. Tormo, M. Martí, J. Valle, A. C. Manna, A. L. Cheung, I. Lasa and J. R. Penadés, SarA is an essential positive regulator of Staphylococcus epidermidis biofilm development, J. Bacteriol., 2005, 187, 2348–2356, DOI:10.1128/jb.187.7.2348-2356.2005.
- Y. Q. Xiong, J. Willard, M. R. Yeaman, A. L. Cheung and A. S. Bayer, Regulation of Staphylococcus aureus alpha-toxin gene (hla) expression by agr, sarA, and sae in vitro and in experimental infective endocarditis, J. Infect. Dis., 2006, 194, 1267–1275, DOI:10.1086/508210.
- N. Ramarao, C. Nielsen-Leroux and D. Lereclus, The insect Galleria mellonella as a powerful infection model to investigate bacterial pathogenesis, J. Visualized Exp., 2012, e4392, DOI:10.3791/4392.
- M. Piatek, G. Sheehan and K. Kavanagh, UtilisingGalleria mellonella larvae for studying in vivo activity of conventional and novel antimicrobial agents, Pathog. Dis., 2020, 78(8), ftaa059, DOI:10.1093/femspd/ftaa059.
- S. D. Kobayashi, N. Malachowa and F. R. DeLeo, Pathogenesis of Staphylococcus aureus abscesses, Am. J. Pathol., 2015, 185, 1518–1527, DOI:10.1016/j.ajpath.2014.11.030.
- G. Sheehan, A. Dixon and K. Kavanagh, Utilization of Galleria mellonella larvae to characterize the development of Staphylococcus aureus infection, Microbiology (Reading), 2019, 165, 863–875, DOI:10.1099/mic.0.000813.
- J. R. Knowles, S. Roller, D. B. Murray and A. S. Naidu, Antimicrobial action of carvacrol at different stages of dual-species biofilm development by Staphylococcus aureus and Salmonella enterica serovar Typhimurium, Appl. Environ. Microbiol., 2005, 71, 797–803, DOI:10.1128/aem.71.2.797-803.2005.
- S. Soltani, A. Shakeri, M. Iranshahi and M. Boozari, A Review of the Phytochemistry and Antimicrobial Properties of Origanum vulgare L. and Subspecies, Iran. J. Pharm. Res., 2021, 20, 268–285, DOI:10.22037/ijpr.2020.113874.14539.
- M. K. Swamy, M. S. Akhtar and U. R. Sinniah, Antimicrobial Properties of Plant Essential Oils against Human Pathogens and Their Mode of Action: An Updated Review, J. Evidence-Based Complementary Altern. Med., 2016, 2016, 3012462, DOI:10.1155/2016/3012462.
- D. Trombetta, F. Castelli, M. G. Sarpietro, V. Venuti, M. Cristani, C. Daniele, A. Saija, G. Mazzanti and G. Bisignano, Mechanisms of antibacterial action of three monoterpenes, Antimicrob. Agents Chemother., 2005, 49, 2474–2478, DOI:10.1128/aac.49.6.2474-2478.2005.
- A. Nostro, A. S. Roccaro, G. Bisignano, A. Marino, M. A. Cannatelli, F. C. Pizzimenti, P. L. Cioni, F. Procopio and A. R. Blanco, Effects of oregano, carvacrol and thymol on Staphylococcus aureus and Staphylococcus epidermidis biofilms, J. Med. Microbiol., 2007, 56, 519–523, DOI:10.1099/jmm.0.46804-0.
- A. Ultee and E. J. Smid, Influence of carvacrol on growth and toxin production by Bacillus cereus, Int. J. Food Microbiol., 2001, 64, 373–378, DOI:10.1016/s0168-1605(00)00480-3.
- E. L. de Souza, J. C. de Barros, C. E. de Oliveira and M. L. da Conceição, Influence of Origanum vulgare L. essential oil on enterotoxin production, membrane permeability and surface characteristics of Staphylococcus aureus, Int. J. Food Microbiol., 2010, 137, 308–311, DOI:10.1016/j.ijfoodmicro.2009.11.025.
- N. M. Wijesundara, S. F. Lee, Z. Cheng, R. Davidson and H. P. V. Rupasinghe, Carvacrol exhibits rapid bactericidal activity against Streptococcus pyogenes through cell membrane damage, Sci. Rep., 2021, 11, 1487, DOI:10.1038/s41598-020-79713-0.
- B. Schwartbeck, J. Birtel, J. Treffon, L. Langhanki, A. Mellmann, D. Kale, J. Kahl, N. Hirschhausen, C. Neumann and J. C. Lee, Dynamic in vivo mutations within the ica operon during persistence of Staphylococcus aureus in the airways of cystic fibrosis patients, PLoS Pathog., 2016, 12, e1006024, DOI:10.1371/journal.ppat.1006024.
- B. Wang, P.-W. Wei, S. Wan, Y. Yao, C.-R. Song, P.-P. Song, G.-B. Xu, Z.-Q. Hu, Z. Zeng and C. Wang, Ginkgo biloba exocarp extracts inhibit S. aureus and MRSA by disrupting biofilms and affecting gene expression, J. Ethnopharmacol., 2021, 271, 113895, DOI:10.1016/j.jep.2021.113895.
- A. Kannappan, S. Gowrishankar, R. Srinivasan, S. K. Pandian and A. V. Ravi, Antibiofilm activity of Vetiveria zizanioides root extract against methicillin-resistant Staphylococcus aureus, Microb. Pathog., 2017, 110, 313–324, DOI:10.1016/j.micpath.2017.07.016.
- L. J. Wu, B. Wang, Q. P. Liao and R. Zhang, Confocal laser scanning electron microscopy for assessment of vaginal Lactobacillus crispatus biofilm, Beijing Daxue Xuebao, Yixueban, 2015, 47, 933–938 Search PubMed.
- N. Høiby, T. Bjarnsholt, M. Givskov, S. Molin and O. Ciofu, Antibiotic resistance of bacterial biofilms, Int. J. Antimicrob. Agents, 2010, 35, 322–332, DOI:10.1016/j.ijantimicag.2009.12.011.
- K. C. Rice, E. E. Mann, J. L. Endres, E. C. Weiss, J. E. Cassat, M. S. Smeltzer and K. W. Bayles, The cidA murein hydrolase regulator contributes to DNA release and biofilm development in Staphylococcus aureus, Proc. Natl. Acad. Sci. U. S. A., 2007, 104, 8113–8118, DOI:10.1073/pnas.0610226104.
- K. J. Livak and T. D. Schmittgen, Analysis of relative gene expression data using real-time quantitative PCR and the 2(-Delta Delta C(T)) Method, Methods (San Diego, Calif.), 2001, 25, 402–408, DOI:10.1006/meth.2001.1262.
- C.-L. Dong, L.-X. Li, Z.-H. Cui, S.-W. Chen, Y. Q. Xiong, J.-Q. Lu, X.-P. Liao, Y. Gao, J. Sun and Y.-H. Liu, Synergistic Effect of Pleuromutilins with Other Antimicrobial Agents against Staphylococcus aureus In Vitro and in an Experimental Galleria mellonella Model, Front. Pharmacol, 2017, 8, 553, DOI:10.3389/fphar.2017.00553.
|
This journal is © The Royal Society of Chemistry 2023 |
Click here to see how this site uses Cookies. View our privacy policy here.