DOI:
10.1039/D3RA05651A
(Paper)
RSC Adv., 2023,
13, 31891-31896
Highly regioselective 6-exo-dig iodo/bromo cyclizations of functionalized 5-amino propargyl pyrimidinones: an efficient synthesis of functionalized pteridines†
Received
19th August 2023
, Accepted 20th October 2023
First published on 31st October 2023
Abstract
The manuscript describes the highly regioselective 6-exo-dig iodo/bromo cyclization of functionalized N-propagyl-amino-pyrimidinones under ambient conditions. The cyclization afforded functionalized pteridines in excellent yields. The optimized procedures are mild, operationally simple and working successfully with different substrates. The synthesis of functionalized pteridines is of great significance because of their potential pharmacological profile.
Introduction
Bicyclic pyrimidinones, condensed with other heterocyclic systems at different positions, have been extensively explored and evaluated for a wide range of biological properties.1 Pteridines are shown to be highly biologically active in every element of the growth and development of living things, including the treatment of cancer, heart disease, neurotransmitter generation, and amino acid metabolism.2–4 Moreover, a number of prevalent diseases including inflammatory disorders, autoimmune processes, neurological diseases, and birth defects have been attributed to the problems in the synthesis, nutritional availability, and/or metabolism of these compounds.3–14 Functionalized pteridines have also been explored for the treatment of fibroproliferative disorders, hepatitis C,15,16 and vascular disorders, etc.12,17–21
A group of heterocyclic compounds known as pteridine, pyrazino[2,3-d] pyrimidines are composed up of condensed pyrimidine/pyrimidinone and pyrazine rings.22 Most naturally produced pteridines referred to as pterins (II) or generally named as 2-amino-4(3H)pteridone belong to a family of nitrogen heterocyclic compounds. The term “pteridine” refers to pyrazino[2,3-d] pyrimidine nucleus structurally, with the numbering of the ring system shown below in (I).23,24 The process of condensation of 4,5-diamino pyrimidine-2,6-dione with various dicarbonyl compounds has been exploited to synthesize pteridines of class III known as lumazines (Fig. 1).23,25–29
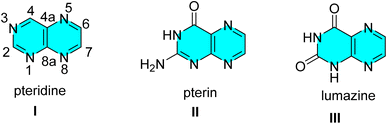 |
| Fig. 1 Structures of pteridines. | |
The synthesis of such functionalized pteridines with a variety of substitutions at different locations becomes crucial due to their potential pharmacological profile.30 As part of our ongoing interest in heterocyclic chemistry, we have previously looked into the synthesis of tricyclic pyrimidinones condensed benzodiazepines,31,32 pyrimidino[thiazenes],33 condensed lactams and thiazole condensed benzodiazepines34–36 among other compounds. The present manuscript describes the synthesis of functionalized 1,2,4,5-tetrasubstituted pyrimidinones and their 6-exo dig halocyclization to yield a variety of functionalized pteridines. The current approach has a number of benefits, including high yield, simplicity, and the provision of functionalized pteridines that can be converted into various heterocyclic systems (Fig. 2).
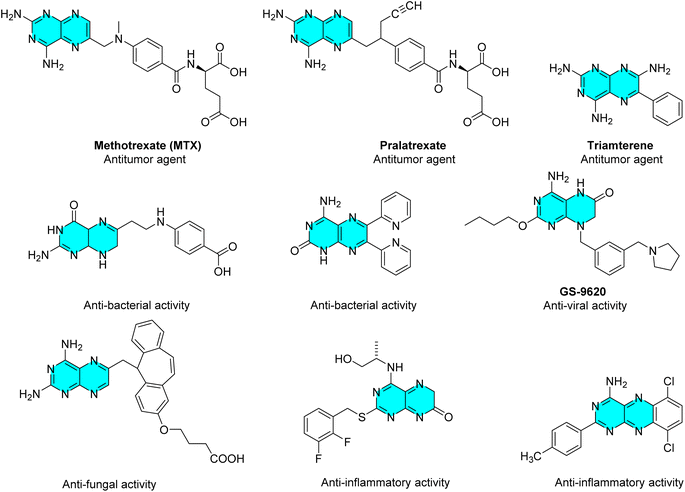 |
| Fig. 2 Biological applications of some pteridines. | |
Results & discussion
The functionalized 5-amino pyrimidinones, 1a–h were prepared by the reaction of phthloylglycine, B with functionalized 1,3-diazabuta-1,3-dienes, A and their subsequent amino deprotection reactions of C using hydrazine hydrate and ethanol (Scheme 1).37
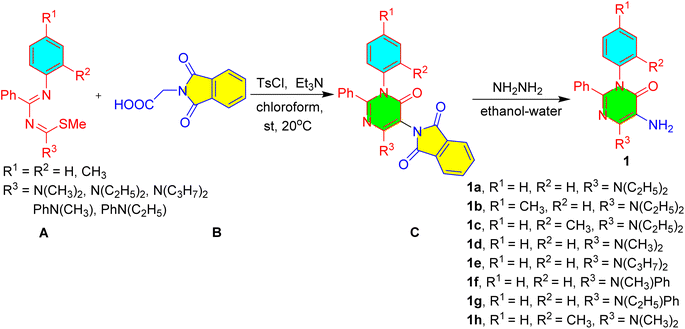 |
| Scheme 1 Synthesis of starting materials, 5-amino-pyrimidinones. | |
These functionalized 5-amino pyrimidinones, 1a–h were explored in 6-exo dig halocyclization reactions to yield 4-oxo-2,3-diaryl-pteridin-8-ium halide, 4a–k in excellent yields. The synthetic methodology involved the initial mono-tosylation of functionalized 5-amino pyrimidinones, 1a–h using tosyl chloride and mild base as triethylamine to yield N-(4-diaryl/alkylamino-6-oxo-1,2-diaryl-1,6-dihydro-pyrimidin-5-yl)-4-methyl-benzenesulfonamides, 2a–h. These mono-aryl-sulphonated 5-amino pyrimidinones, 2a–h were explored in mono-propargylation to provide a series of N-propargyl-N-(4-dialkyl/aryl-amino-6-oxo-1,2-diaryl-1,6-dihydro-pyrimidin-5-yl)-aryl sulfonamides, 3a–h in excellent yields (77–92% yield, Scheme 2).
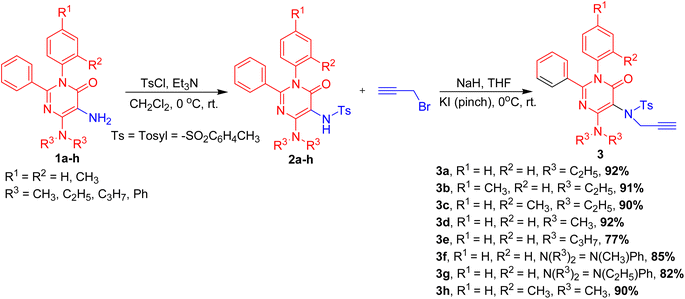 |
| Scheme 2 Synthesis of N-propagyl-N-(4-dialkyl/aryl-amino-6-oxo-1,2-diaryl-1,6-dihydro-pyrimidin-5-yl)-arylsulfonamides, 3a–h. | |
These functionalized pyrimidinones, 3a–h were explored in 6-exo-dig halocyclization reactions. The reaction resulted in the formation of 4-oxo-2,3-diaryl-pteridin-8-ium halide, 4a–k in good to excellent yields. Different solvents such as DCM, toluene, acetonitrile, etc., and different halogenated agents such as NCS, NBS, Br2, I2, etc were attempted for better yield and selectivity in the synthesis of functionalized 4-oxo-2,3-diaryl-pteridin-8-ium halide, 4a–k. The results are summarized in Table 1. It has been found that the iodocyclization occurs efficiently using I2 (3 eq.) in DCM (20 mL) and the reaction gave poor yield in other tested solvents such as acetonitrile and toluene. The 6-exo-dig halocyclizations of functionalized pyrimidinones using alternate iodocyclization agents such as NIS afforded undesired products (Table 1, entry 1). The iodocyclization reactions also occurred efficiently in the absence of base (Table 1; entries 8–14). Next, we optimized the reaction conditions for 6-exo-dig bromo cyclizations using different brominating agents such as NBS, Br2, etc. The 6-exo-dig bromocyclization afforded 4-oxo-2, 3-diaryl-pteridin-8-ium bromide in good yields using Br2 (3 eq.) in DCM (20 mL) (Table 1, entries 6, and 12–15). The 6-exo-dig bromocyclization led to poor yields of product when a higher amount of bromine (4 to 6 eq.) was used during haloaminations. The 6-exo-dig bromocyclizations were inefficient and undesired products were found when NBS was used as a halogen source under different reaction conditions (Table 1, entries 4 and 5). Moreover, the chloro–amination reactions were unsuccessful using N-bromosuccinamide (NCS) was used as a halogen source in attempted 6-exo-dig chloroamination reactions (Table 1, entry 3).
Table 1 Optimization of the reaction conditions for 6-exo-dig halocyclizations
S. no |
Pyrimidinone |
Reaction conditions |
Reaction timeb |
Yieldsa (%) |
Reagent |
Eq. |
Base (5 eq.) |
Solvent (20 mL) |
Isolated yields after purification. Reaction time. Dry DCM used as a solvent. |
1 |
3a |
NIS |
4 |
K2CO3 |
DCM |
— |
— |
2 |
3ac |
I2 |
3 |
K2CO3 |
DCM |
20 min |
86 |
3 |
3a |
NCS |
4 |
K2CO3 |
DCM |
— |
— |
4 |
3a |
NBS |
4 |
K2CO3 |
DCM |
— |
— |
5 |
3a |
NBS |
4 |
NaH |
DCM |
— |
— |
6 |
3a |
Br2 |
2.5 |
K2CO3 |
DCM |
20 min |
79 |
7 |
3a |
I2 |
4.5 |
t-BuOK |
THF |
— |
— |
8 |
3ac |
I2 |
3 |
— |
DCM |
20 min |
89 |
9 |
3a |
I2 |
3.5 |
— |
Toluene |
3 h |
55 |
10 |
3a |
I2 |
3.5 |
— |
THF |
1 h |
50 |
11 |
3a |
I2 |
3.5 |
— |
Acetonitrile |
1.5 h |
40 |
12 |
3a |
Br2 |
3 |
— |
DCM |
20 min |
84 |
13 |
3a |
Br2 |
3 |
— |
Toluene |
3 h |
53 |
14 |
3a |
Br2 |
3 |
— |
THF |
3 h |
49 |
15 |
3a |
Br2 |
3 |
— |
Acetonitrile |
3 h |
35 |
We next investigated these 6-exo-dig halocyclization reactions using a variety of functionalized pyrimidinones. Different pyrimidinones, 3a–h with a variety of substituents such as dimethyl, diethyl, dipropyl, etc. at the C-4 position were studied in these halocyclization reactions. The reactions resulted in the formation of 4-oxo-2,3-diaryl-pteridin-8-ium halide 4a–k in good to excellent yields (Table 2, entries 1–11). The various substituents at the C-1 or C-2 position did not change the yield of the product of these halocyclization reactions (Table 2; entries 1–11). The 6-exo-dig halocyclization reactions tolerate a variety of steric bulk at the C-4 position (Table 2; entries 3–7). Functionalized pyrimidinones with a dimethyl or diethyl amino group at the C-4 position resulted in efficient 6-exo-dig cyclizations (Table 2; entries 1–4 and 8–11). With dipropyl amine at its C-4 position, the halo amination of 3e took a relatively longer reaction time and yielded 4e with a slightly lower yield (Table 2; entry 5). With a hindered secondary amine (N-aryl methyl/ethyl amine) at the C-4 position, the 2,3-dialkyl-5-propynylsulfanyl-3H-pyrimidin-4-ones, 3f & 3g effectively accomplished 6-endo-dig cyclization reactions to provide 4f, g in good yields (Table 2; entries 6 and 7). These experimental findings demonstrate that the various sterically hindered amines at the C-4 position are successfully tolerated by the 6-exo-dig haloamination reactions of pyrimidinones, 3a–h. (Table 2; entries 5–7). The yield decreases with an increase in steric bulk at the C-4 position. The bromocyclization afforded comparatively lower yields of 4-oxo-2,3-diaryl-pteridin-8-ium halide owing to the more reactive nature of the bromine (Table 2, entries 8–11). All these reactions resulted in the formation of 4-oxo-2,3-diaryl-pteridin-8-ium halide, 4a–k, and competitive 7-endo dig cyclized products were not formed. The Impure compounds, 4a–k were purified by using a solvent mixture of dichloromethane and diethyl ether (1
:
9) without performing any column chromatography.
Table 2 6-exo-dig haloamination reactions of functionalized pyrimidinones, 3a–h
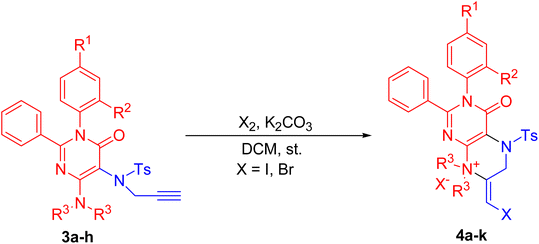
|
S. no |
R1 |
R2 |
R3 |
X |
Substrateb |
Productc |
Yielda (%) |
Isolated yields after purification. Reaction time 20 min. Starting substrates (3a–g) taken = 500 mg, 0.870–1.000 mmoles. |
1 |
H |
H |
C2H5 |
I |
3a |
4a |
89 |
2 |
CH3 |
H |
C2H5 |
I |
3b |
4b |
87 |
3 |
H |
CH3 |
C2H5 |
I |
3c |
4c |
88 |
4 |
H |
H |
CH3 |
I |
3d |
4d |
90 |
5 |
H |
H |
C3 H7 |
I |
3e |
4e |
71 |
6 |
H |
H |
CH3 & Ph |
I |
3f |
4f |
84 |
7 |
H |
H |
C2H5 & Ph |
I |
3g |
4g |
82 |
8 |
H |
CH3 |
CH3 |
Br |
3h |
4h |
86 |
9 |
H |
H |
CH3 |
Br |
3d |
4i |
85 |
10 |
H |
H |
C2H5 |
Br |
3a |
4j |
84 |
11 |
CH3 |
H |
C2H5 |
Br |
3b |
4k |
82 |
The plausible mechanism involved the iodonium ion's coordination with the triple bond of the N-propargyl of the pyrimidinone ring during its initial formation. The subsequent exo-dig nucleophilic attack of the C-4 substituted secondary amino group results in the production of the 4-oxo-2,3-diaryl-3,4,5,6,7,8-hexahydro-pteridin-8-ium halide in good yields. Approach-a for haloamination is preferred while competitive approach-b is disfavored due to the development of a more stabilised six-membered fused pyrazine ring than the competitive seven-membered fused diazepine ring (Scheme 3).
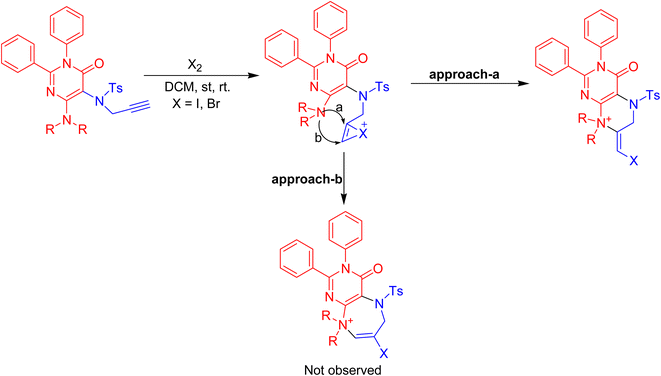 |
| Scheme 3 Mechanism for the formation of hexahydropteridin-8-ium derivatives, 4a–k. | |
Conclusion
In summary, an efficient regioselective protocol for the formation of functionalized pteridines has been reported. The operational simplicity, shorter reaction time, good substrate scope, column chromatography-free approach, and regioselectivity are the attractive features of the present method. Further exploration of the full scope of these reactions and their extension to other arenes and heteroarenes will be reported in due course.
Experimental section
General procedure for the formation of N-(4-dialkylamino-6-oxo-1,2-diaryl-1,6-dihydro-pyrimidinin-5-yl)-4-methyl-benzenesulfonamide (2a–h)
To a solution of 5-amino pyrimidinones 1a–h (2 g, 1.950–2.550 mmoles) and triethylamine (3 eq.) in dry CHCl3 (50 mL) at 0 °C, was added dropwise a solution of p-TsCl (2.0 eq.) mixed in dry chloroform. The advancement of the reaction was checked by tlc. At the end of the reaction (overnight stirring), a usual workup was carried out using water and chloroform. The organic layers were combined, dried over sodium sulfate, and concentrated to get the crude product. The impure crude product was loaded into the column and purified by using ethyl acetate and hexane (2
:
8) as an eluent. The crude compounds were further purified using a mixture of 10% dichloromethane in diethyl ether to obtain N-(4-dialkylamino-6-oxo-1,2-diaryl-1,6-dihydro-pyrimidinin-5-yl)-4-methyl-benzenesulfonamide (2a–h) as pure compounds in good yields.
General procedure for the formation of N-(4-dialkylamino-6-oxo-1,2-diaryl-1,6-dihydro-pyrimidinin-5-yl)-N-prop-2-ynyl-benzenesulfonamide (3a–h)
To a well-stirred solution of N-(4-dialkylamino-6-oxo-1,2-diaryl-1,6-dihydro-pyrimidinin-5-yl)-4-methyl-benzenesulfonamide (2a–h) (1 g, 1.870–2.170 mmoles) in dry CHCl3 (30 mL) at 0 °C, was added, a solid sodium hydride (1.2 eq.) in small increments. The reaction was initially stirred for fifteen minutes and then the propargyl bromide (1.2 eq.) was added dropwise. The advancement of the reaction was checked by tlc. At the end of the reaction (5 hours stirring), a usual workup was carried out using ethyl acetate and water. The organic layers were combined, dried over sodium sulfate, and concentrated to obtain the crude product. The impure crude product was loaded into the column and purified by using a solution of ethyl acetate and hexane (1
:
9) as an eluent. The crude product was further purified using 10% dichloromethane in diethyl ether to obtain pure N-(4-dialkylamino-6-oxo-1,2-diaryl-1,6-dihydro-pyrimidinin-5-yl)-N-prop-2-ynyl-benzenesulfonamide (3a–h) in good yields.
N-(4-(diethylamino)-6-oxo-1,2-diphenyl-1,6-dihydropyrimidin-5-yl)-4-methyl-N-(prop-2-yn-1-yl)benzenesulfonamide (3a). (1 g, 2.05 mmoles of 2a); yield-92%; white solid; 1H NMR (400 MHz, CDCl3): δ 7.71 (d, J = 8.3 Hz, 2H), 7.18–7.26 (m, 10H), 6.84 (dd, J = 7.3, 2.2 Hz, 2H), 4.69 (dd, J = 17.2, 2.6 Hz, 1H), 4.58 (dd, J = 17.2, 2.6 Hz, 1H), 4.13 (m, J = 14.1, 7.1 Hz, 2H), 3.82 (m, J = 14.1, 7.1 Hz, 2H), 2.42 (s, 3H), 2.31 (t, J = 2.5 Hz, 1H), 1.39 (t, J = 7.0 Hz, 6H). 13C NMR (101 MHz, CDCl3): δ 161.05, 158.61, 154.84, 143.39, 137.19, 135.72, 134.95, 129.65, 129.23, 129.08, 128.83, 128.54, 128.33, 127.98, 127.80, 96.62, 79.51, 73.25, 43.69, 39.74, 21.62, 13.80. HRMS (ESI + TOF) calcd for C30H31N4O3S+ (MH+): 527.2111, found: 527.2115.
General procedure for the synthesis of hexahydro-pteridines (4a–k). To a solution of pyrimidinones, 3a–h (500 mg, 0.870–1.000 mmoles) in dry dichloromethane (20 mL) was added bromine or iodine (3 eq.) in small amounts at room temperature. The advancement of the reaction was checked by tlc. At the end of the reaction, (20 minutes stirring) the mixture was first quenched with an aqueous solution of sodium thiosulphate, and then workup was carried out using dichloromethane and brine solution. The filtrate was dried over sodium sulfate and concentrated to get the crude product. The crude product was purified using a solution of 10% dichloromethane in diethyl ether to get a pure compound, 4a–k in good yields.
(E)-8,8-Diethyl-7-(iodomethylene)-4-oxo-2,3-diphenyl-5-tosyl-3,4,5,6,7,8-hexahydropteridin-8-ium, iodide (4a). (500 mg, 0.95 mmol of 3a); (680 mg recovered, yield-89%); white solid; 1H NMR (400 MHz, CDCl3): δ 7.63 (d, J = 8.5 Hz, 2H), 7.39–7.32 (m, 6H), 7.25–7.20 (m, 6H), 5.43 (d, J = 1.8 Hz, 1H), 4.99 (d, J = 17.3 Hz, 1H), 4.79 (dd, J = 17.1, 2.1 Hz, 1H), 4.51 (m, J = 14.7, 7.4 Hz, 1H), 4.16 (m, J = 13.8, 7.2 Hz, 1H), 3.94 (m, J = 14.6, 7.4 Hz, 1H), 3.78 (m, J = 14.6, 7.1 Hz, 1H), 2.49 (s, 3H), 1.56 (t, J = 7.4 Hz, 3H), 1.42 (t, J = 7.4 Hz, 3H). 13C NMR (101 MHz, CDCl3): δ 157.56, 155.46, 153.81, 146.41, 145.19, 134.10, 132.06, 131.94, 131.29, 130.29, 129.89, 129.78, 128.77, 128.29, 98.53, 64.57, 46.35, 21.99, 13.09; HRMS (ESI + TOF) calcd for C30H30IN4O3S+ (M+): 653.1078, found: 653.1107.
Conflicts of interest
Authors declare no conflict of interest.
Acknowledgements
I. K. Gujral Punjab Technical University, Kapurthala 144601, Punjab is acknowledged for providing research facilities.
References
- A. Burger, Medicinal Chemistry, New York (NY), 1970, vol. 72, p. 719 Search PubMed.
- J. Delgado and W. W. Remers, Wilson and Gisvold's Textbook of Organic Medicinal and Pharmaceutical Chemistry, ed. Lippinicott Williams and Wilkins, Philadelphia, PA, USA, 1998, p. 185 Search PubMed.
- S. Milstien, G. Kapatos, R. A. Levine and B. Shane, Chemistry and Biology of Pteridines and Folates: Proceedings of the 12th International Symposium on Pteridines and Folates, National Institutes of Health, Springer Science & Business Media, Bethesda, Maryland, 2012 Search PubMed.
- T. Sasada, F. Kobayashi, N. Sakai and T. Konakahara, Org. Lett., 2009, 11, 2161–2164 CrossRef CAS PubMed.
- J. Dörrstein, R. Scholz, D. Schwarz, D. Schieder, V. Sieber, F. Walther and C. Zollfrank, Compos. Struct., 2018, 189, 349–356 CrossRef.
- U. Leurs, E. Lajkó, G. Mező, E. Orbán, P. Öhlschläger, A. Marquardt, L. Kőhidai and M. Manea, Eur. J. Med. Chem., 2012, 52, 173–183 CrossRef CAS PubMed.
- M.-H. Li, S. K. Choi, T. P. Thomas, A. Desai, K.-H. Lee, A. Kotlyar, M. M. B. Holl and J. R. Baker Jr., Eur. J. Med. Chem., 2012, 47, 560–572 CrossRef CAS PubMed.
- A. Rosowsky, R. A. Forsch, J. H. Freisheim and R. G. Moran, J. Med. Chem., 1989, 32, 517–520 CrossRef CAS PubMed.
- A. Rosowsky, J. Galivan, G. Beardsley, H. Bader and B. O'Connor, Cancer Res., 1992, 52, 2148–2155 CAS.
- Z. Zhang, J. Wu, F. Ran, Y. Guo, R. Tian, S. Zhou, X. Wang, Z. Liu, L. Zhang and J. Cui, Eur. J. Med. Chem., 2009, 44, 764–771 CrossRef CAS PubMed.
- G. Fredi and A. Dorigato, Adv. Ind. Eng. Polym. Res., 2021, 4, 159–177 CAS.
- C.-H. Lee, M. Jiang, M. Cowart, G. Gfesser, R. Perner, K. H. Kim, Y. G. Gu, M. Williams, M. F. Jarvis and E. A. Kowaluk, J. Med. Chem., 2001, 44, 2133–2138 CrossRef CAS PubMed.
- C. Enzinger, B. Wirleitner, N. Spöttl, G. Böck, D. Fuchs and G. Baier-Bitterlich, Neurochem. Int., 2002, 41, 71–78 CrossRef CAS PubMed.
- D. Voet and J. Voet, Biochemistry, John Wiley & Sons, Hoboken, NJ, 3rd edn, 2004, pp. 909–984 Search PubMed.
- Y. Ding, J.-L. Girardet, K. L. Smith, G. Larson, B. Prigaro, V. C. Lai, W. Zhong and J. Z. Wu, Bioorg. Med. Chem. Lett., 2005, 15, 675–678 CrossRef CAS PubMed.
- P. Raboisson, O. Lenz, T.-I. Lin, D. Surleraux, S. Chakravarty, A. Scholliers, K. Vermeiren, F. Delouvroy, T. Verbinnen and K. Simmen, Bioorg. Med. Chem. Lett., 2007, 17, 1843–1849 CrossRef CAS PubMed.
- H. Iwamura, N. Masuda, K. Koshimizu and S. Matsubara, Plant Sci. Lett., 1980, 20, 15–18 CrossRef CAS.
- E. Katilius, Z. Katiliene and N. W. Woodbury, Anal. Chem., 2006, 78, 6484–6489 CrossRef CAS PubMed.
- M. S. Palanki, E. Dneprovskaia, J. Doukas, R. M. Fine, J. Hood, X. Kang, D. Lohse, M. Martin, G. Noronha and R. M. Soll, J. Med. Chem., 2007, 50, 4279–4294 CrossRef CAS PubMed.
- R. J. Stanley, Z. Hou, A. Yang and M. E. Hawkins, J. Phys. Chem. B, 2005, 109, 3690–3695 CrossRef CAS PubMed.
- G. Wenska, B. Skalski, I. Tomska-Foralewska and S. Paszyc, Helv. Chim. Acta, 2001, 84, 3726–3734 CrossRef CAS.
- F. E. Hahn and M. C. Jahnke, Angew. Chem., Int. Ed., 2008, 47, 3122–3172 CrossRef CAS PubMed.
- D. J. Brown, Fused Pyrimidines, John Wiley & Sons, 2009, Part 3: Pteridines, vol. 24 Search PubMed.
- M. Koller, in Handbook of Microalgae-Based Processes and Products, ed. E. Jacob-Lopes, M. M. Maroneze, M. I. Queiroz and L. Q. Zepka, Academic Press, 2020, pp. 597–645, DOI:10.1016/B978-0-12-818536-0.00022-1.
- A. Albert, Q. Rev., Chem. Soc., 1952, 6, 197–237 RSC.
- E. S. H. E. Ashry, S. Youssif, M. E. Ahwany and M. E. Sanan, J. Chem. Res., 2005, 2005, 262–266 CrossRef.
- S. Goswami and A. C. Maity, Chem. Lett., 2007, 36(9), 1118–1119 CrossRef CAS.
- V. A. Mamedov, N. A. Zhukova, A. T. Gubaidullin, V. V. Syakaev, M. S. Kadyrova, T. Y. N. Beschastnova, O. B. Bazanova, I. D. K. Rizvanov and S. K. Latypov, J. Org. Chem., 2018, 83, 14942–14953 CrossRef CAS PubMed.
- A. Marchal, M. Melguizo, M. Nogueras, A. Sanchez and J. N. Low, Synlett, 2002, 2002, 0255–0258 CrossRef.
- V. Carmona-Martínez, A. J. Ruiz-Alcaraz, M. Vera, A. Guirado, M. Martínez-Esparza and P. García-Peñarrubia, Med. Res. Rev., 2019, 39, 461–516 CrossRef PubMed.
- R. A. Naikoo, R. Kumar, P. Singh and G. Bhargava, Synth. Commun., 2021, 51, 1232–1241 CAS.
- R. A. Naikoo, R. Kumar, V. Kumar and G. Bhargava, Synth. Commun., 2021, 51, 1451–1477 CAS.
- R. Sharma and C. Mohan, J. Heterocycl. Chem., 2017, 54, 1833–1839 CrossRef CAS.
- B. Kuila, Y. Kumar, D. Mahajan, K. Kumar, P. Singh and G. Bhargava, RSC Adv., 2016, 6, 57485–57489 RSC.
- Y. Kumar, B. Kuila, D. Mahajan, P. Singh, B. Mohapatra and G. Bhargava, Tetrahedron Lett., 2014, 55, 2793–2795 CrossRef CAS.
- G. M. Shelke, V. K. Rao, M. Jha, T. S. Cameron and A. Kumar, Synlett, 2015, 26, 404–407 CrossRef CAS.
- R. Sharma, D. Y. Gawande, C. Mohan and R. K. Goel, Med. Chem. Res., 2016, 25, 1420–1424 CrossRef CAS.
|
This journal is © The Royal Society of Chemistry 2023 |
Click here to see how this site uses Cookies. View our privacy policy here.