DOI:
10.1039/D3RA05736D
(Paper)
RSC Adv., 2023,
13, 29607-29612
One-pot reductive amination of carbonyl compounds and nitro compounds via Ir-catalyzed transfer hydrogenation†
Received
22nd August 2023
, Accepted 25th September 2023
First published on 9th October 2023
Abstract
The formation of C–N bond is a vital synthetic tool for establishing molecular diversity, which is highly sought after in a wide range of biologically active natural products and drugs. Herein, we present a new strategy for the synthesis of secondary amines via iridium-catalyzed one-pot reductive amination of carbonyl compounds with nitro compounds. This method is demonstrated for a variety of carbonyl compounds, including miscellaneous aldehydes and ketones, which are compatible with this catalytic system, and deliver the desired products in good yields under mild conditions. In this protocol, the reduction of nitro compounds occurs in situ first, followed by reductive amination to form amine products, providing a new one-pot procedure for amine synthesis.
Amines, particularly secondary amines, are not only prevalent in dyestuff, and chemosynthesis plants as important industrial materials, but also exist in a wide range of biologically active natural products and drugs.1 Thus, the establishment of strategies to facilely form C–N bond is of longstanding significance in organic synthesis. A simple strategy to construct amines is the alkylation of amines with alkyl halides2 or alcohols.3 The successful preparation of C–N bond would also be realized by Buchwald-Hartwig4 or Ullman-type cross-coupling reactions.5 Notably, strategies of reductive amination6 and addition reactions to imines7 have been described as in the C–N bond synthesis. Additionally, the reduction of amides8 also enables the formation of amine products. Given the simple and easy accessibility of raw materials, reductive amination undoubtedly enables the practical and direct formation of the C–N bond,9 which is shown as a step and atomic economy.
Nitro compounds, as readily available feedstocks, have been extensively employed for reductive amination with carbonyl compounds, in which the nitro compounds are converted into primary amines in advance, thereby avoiding the additional purification steps.10 In this context, a pioneering report on the reductive amination of carbonyl compounds with nitro compounds was described by Major's group in early 1931 with H2 as the hydrogenation reagent.11 Since this seminal work, extensive outstanding progress has been achieved over the past decades. In this regard, a heterogeneous catalytic system utilizing noble metals (such as platinum,12 palladium,13 rhodium,14 iridium,15 ruthenium,16 silver,17 and gold18) and non-noble metals (such as nickel,19 cobalt,20 copper,21 and iron,22) as catalysts have been established (Scheme 1a). Similarly, strategies based on the catalytic system with the form of metals, oxides, and other compounds as catalysts have been developed as well for the reductive amination of carbonyl derivatives with nitro compounds (Scheme 1a). However, synthetic methods for this one-pot reductive amination of nitro compounds were limited to H2 as the hydrogen donor, which is inevitably constrained in the use of high-pressure devices and possesses potential safety issues.
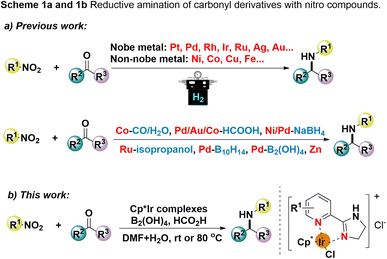 |
| Scheme 1 Reductive amination of carbonyl derivatives with nitro compounds. | |
As a consequence, approaches for replacing hydrogen gas with other hydrogen donors, such as CO/H2O,23 HCO2H,24 NaBH4,25 B10H14,26 B2(OH)4,27 and Zn,28 for the reductive amination with nitro compounds have been established (Scheme 1a). However, great efforts to circumvent the harsh reaction conditions, narrow the substrate scope, long reaction times, complicated catalyst preparation processes, high catalyst loading, and well as side-reactions have been made in the past years.
As such, transfer hydrogenation, which uses polyatomic molecules, including formic acid, alcohol, hydrazine hydrate, and silane as hydrogen donors, to migrate hydrogen to the unsaturated functional group directly with the assistance of catalysts. In this context, formic acid obtained from biomass, bearing the merits of high energy density, low cost, non-poisonousness, and stability, has been widely applied as a hydrogen source in transfer hydrogenation reactions. Recently, we have been working on the transfer hydrogenation of C
O, C
C, C
N bonds using Cp*Ir complexes as catalysts and formic acid as the hydrogen donor.29 In these methods, we have demonstrated that these Cp*Ir complexes could enable indirect and direct reductive amination for the construction of amine compounds.29a,d However, reductive amination for the construction of C–N bond via transfer hydrogenation with nitro compounds as the source of amine remains relatively limited. Herein, we present an iridium-catalyzed one-pot reductive amination of carbonyl compounds with nitro compounds (Scheme 1b). In this protocol, the reduction of nitro compounds occurs in situ first, followed by reductive amination to form the amine products, providing a new one-pot procedure for amine synthesis.
To explore the possibility of the reaction, the reductive amination was started by using 1-isopropoxy-4-nitrobenzene (2a) and benzaldehyde (1a) as templates, and TC as the catalyst. As shown in Table 1, 98% conversion of the desired product 3aa was observed using TC-1 as a catalyst, 15.0 equiv. HCO2H as the hydrogen donor, as well as DMF and H2O as the mixed solvent (v/v = 1
:
1) after 4 h (Table 1, entry 1). Catalyst screening demonstrated the corresponding product 3aa could be formed as well, though a lower conversion of the product was obtained (Table 1, entries 2–6). For instance, only moderate yields of the desired product were afforded when catalysts bearing methyl or chlorine substituents were employed (Table 1, entries 2 and 3). In contrast, good to excellent yields of the corresponding product were observed using fluorine or methoxyl-substituted catalysts (Table 1, entries 4–6). Of note, the increase in the reaction time had no influence on the yield of the product (Table 1, entry 7). However, a sharp decrease in the yield was observed when the catalyst loading of TC-1 was decreased to 0.5 mol% (Table 1, entry 8). A similar negative influence on the yield of the product was also observed when different reaction media were loaded (Table 1, entries 9–12).
Table 1 Optimization of the conditions for Ir-catalysed reductive amination of aldehydes with nitro compoundsa
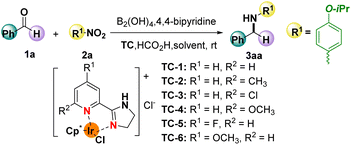
|
Entry |
Catalyst |
Solvent |
HCO2H (eq.) |
Time (h) |
Yieldb (%) |
Reaction conditions: 1a (0.5 mmol), 2a (1.0 mmol), solvent (2.0 mL), catalyst (0.005 mmol), HCO2H (15.0 equiv.), B2(OH)4 (6.0 equiv.), 4,4-bipyridine (0.05 mmol) at room temperature under air for 12 h. Determined using GC-MS. With 0.0025 mmol of the catalyst. |
1 |
TC-1 |
DMF + H2O |
15 |
4 |
98 |
2 |
TC-2 |
DMF + H2O |
15 |
4 |
66 |
3 |
TC-3 |
DMF + H2O |
15 |
4 |
48 |
4 |
TC-4 |
DMF + H2O |
15 |
4 |
92 |
5 |
TC-5 |
DMF + H2O |
15 |
4 |
89 |
6 |
TC-6 |
DMF + H2O |
15 |
4 |
76 |
7 |
TC-1 |
DMF + H2O |
15 |
12 |
99 |
8c |
TC-1 |
DMF + H2O |
15 |
12 |
54 |
9 |
TC-1 |
DMSO + H2O |
15 |
12 |
10 |
10 |
TC-1 |
H2O |
15 |
12 |
<5 |
11 |
TC-1 |
DMSO |
15 |
12 |
<5 |
12 |
TC-1 |
DMF |
15 |
12 |
<5 |
With the optimized conditions in hand, the substrate scope was investigated to explore its versatility (Scheme 2). Firstly, the 1-isopropoxy-4-nitrobenzene (2a) was loaded as an amination agent to react with various aldehydes. Gratifyingly, the desired reductive amination products of 3ba–3ga, 3ia, 3ja, as well as 3sa were afforded good yields with mono-substituted benzaldehydes as substrates. Of note, only a moderate yield of the corresponding product was achieved when 4-nitrobenzaldehyde was loaded as the substrate (3ha). Interestingly, the employment of multi-substituted benzaldehydes, including electron-donating or electron-withdrawing substituents on the phenyl group, could also enable the delivery of the corresponding products (3ka–3pa) in similar excellent yields. Obviously, the substrate 2w bearing the potential oxidized hydroxyl group reacted smoothly as well under this system. Satisfactorily, employing aliphatic aldehydes, naphthaldehyde, as well as unsaturated aldehyde as substrates also furnished the corresponding products 3ra, 3ta–3xa in moderate to good yields. It is worth noting that the amino acid product of 3ab was produced when 2-nitrobenzoic acid was loaded as the substrate.
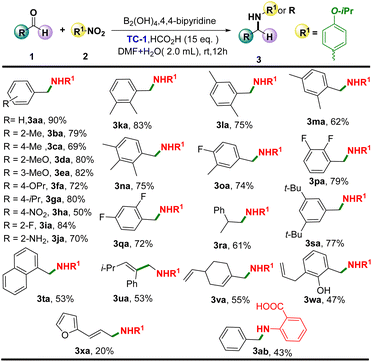 |
| Scheme 2 Substrate scope of the aldehydes and nitro compounds. Reaction conditions: 1 (0.5 mmol), 2 (1.0 mmol), solvent (2.0 mL), catalyst (0.005 mmol), HCO2H (10.0 equiv.), B2(OH)4 (6.0 equiv.), 4,4-bipyridine (0.05 mmol) at room temperature in air for 12 h. Yield of the isolated product. | |
Encouraged by the results of the Ir-catalysed reductive amination of aldehydes with nitro compounds, the feasibility of using ketones as substrates was also investigated (Table 2). Firstly, 4-phenyl-2-butanone (5a) and 1-isopropoxy-4-nitrobenzene (2a) were chosen for the model reaction to optimize the reaction parameters. Catalyst screening (Table 2, entries 1–6) demonstrated that, unlike aldehydes, TC-4 was found to be the optimal catalyst for the reductive amination of ketones, delivering 90% conversion of the desired product 4aa at room temperature (Table 2, entry 4). Gratifyingly, the yield of the product was slightly increased when the reaction temperature was increased to 80 °C (Table 2, entry 7). Similar to aldehydes, solvents have a significant influence on the reaction, producing completely different yields of the product in different reaction media (Table 2, entries 8–11). Of note, a similar high yield of the product was obtained even when the loading of the catalyst was decreased to 0.1 mol%, or the reaction time was shortened to 2 h (Table 2, entries 12–15). Control experiments evidenced that, indeed, a 30% yield of the corresponding reductive amination product of 4aa could be afforded in the absence of B2OH4 under standard conditions (Table 2, entry 16). However, the iridium catalyst was essential for the formation of the desired product (Table 2, entry 17) (see ESI† for further details, F).
Table 2 Optimization of conditions for the Ir-catalysed reductive amination of ketone with nitro compoundsa
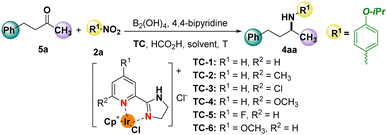
|
Entry |
Cat. |
T (°C) |
Solvent |
HCO2H (eq.) |
Time (h) |
Yieldb (%) |
Reaction conditions: 5a (0.5 mmol), 2a (0.75 mmol), solvent (2.0 mL), catalyst (1.0% mol), HCO2H (15.0 equiv.), B2(OH)4(2.25 mmol), 4,4-bipyridine (0.0375 mmol) at 80 °C in air for 12 h. Determined using GC-MS. The reaction was carried out using 0.0005 mmol of the catalyst. Without B2(OH)4. |
1 |
TC-1 |
rt |
DMF + H2O |
15 |
12 |
59 |
2 |
TC-2 |
rt |
DMF + H2O |
15 |
12 |
66 |
3 |
TC-3 |
rt |
DMF + H2O |
15 |
12 |
38 |
4 |
TC-4 |
rt |
DMF + H2O |
15 |
12 |
90 |
5 |
TC-5 |
rt |
DMF + H2O |
15 |
12 |
34 |
6 |
TC-6 |
rt |
DMF + H2O |
15 |
12 |
34 |
7 |
TC-4 |
80 |
DMF + H2O |
15 |
12 |
97 |
8 |
TC-4 |
80 |
DMF |
15 |
12 |
34 |
9 |
TC-4 |
80 |
DMSO |
15 |
12 |
<5 |
10 |
TC-4 |
80 |
H2O |
15 |
12 |
n.d. |
11 |
TC-4 |
80 |
DMSO + H2O |
15 |
12 |
n.d. |
12 |
TC-4 |
80 |
DMF + H2O |
10 |
12 |
95 |
13 |
TC-4 |
80 |
DMF + H2O |
12 |
12 |
97 |
14 |
TC-4 |
80 |
DMF + H2O |
15 |
2 |
97 |
15c |
TC-4 |
80 |
DMF + H2O |
15 |
12 |
94 |
16d |
TC-4 |
80 |
DMF + H2O |
15 |
12 |
30 |
17 |
— |
80 |
DMF + H2O |
15 |
12 |
n.d. |
Based on the above optimizations, the substrate scope of ketones and nitro compounds was investigated (Scheme 3).
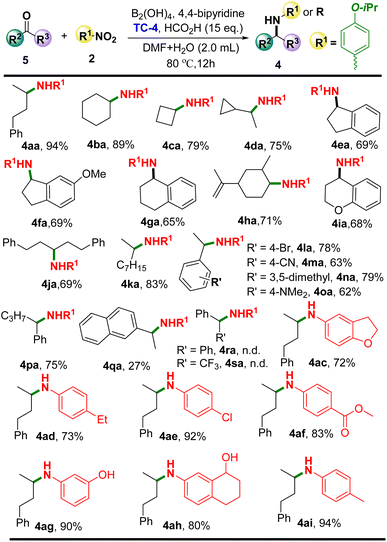 |
| Scheme 3 Substrate scope of ketones and nitro compounds for reductive amination. Reaction conditions: 5 (0.5 mmol), 2 (0.75 mmol), solvent (2.0 mL), catalyst (1.0 mol%), HCO2H (15.0 equiv.), B2(OH)4 (2.25 mmol), 4,4-bipyridine (0.0375 mmol) at room temperature under air for 12 h. Yield of the isolated product. | |
Firstly, the use of 4-phenylbutan-2-one (5a) as the substrate to react with 4-isopropylnitrobenzene or heterocyclic nitrobenzene was explored to examine the versatility of this reductive amination. Gratifyingly, various cyclic aliphatic ketones loaded as substrates enabled the delivery of the target products (4ba–4ga) in moderate to good yields. Similar results were also observed (4ha and 4ia) when unsaturated ketones or heterocyclic ketones were employed in this catalytic system. Obviously, the use of aliphatic ketones with long carbon chains 5j and 5k as the substrates did not have an influence on the yields of the desired products (4ja and 4ka). It should be noted that aromatic ketones were also well tolerated in this system to produce the corresponding products (4la–4pa). Nevertheless, only 27% of the target product (4qa) was provided using a more steric hindrance of 1-(naphthalen-2-yl)ethan-1-one (5q) as the substrate. Disappointingly, benzophenone (5r) or 2,2,2-trifluoro-1-phenylethan-1-one (5s) substrates could not be tolerated in this catalytic system. On the other hand, different substituted nitro compounds were also investigated in this system. Satisfyingly, heterocyclic substrates such as 5-nitro-2,3-dihydrobenzofuran (2c) were well tolerated to afford the desired product 4ac in good yield. Interestingly, di-functional conversion products of 4ad and 4ah were observed when 1-ethynyl-4-nitrobenzene (2d) and 7-nitro-3,4-dihydronaphthalen-1(2H)-one (2h) were loaded, in which, both the alkyne and carbonyl groups were reduced simultaneously. It is notable that the chlorine (2e), methyl (2i), ester (2f), and even the readily oxidized phenol hydroxyl (2g) substituted nitro compounds were compatible with this system, delivering the corresponding products in excellent yields.
The model reaction was scaled up to investigate the practicability of this protocol (Scheme 4). Gratifyingly, 2.32 g target product of 4aa was produced in 82% yield after 12 h by loading 10.0 mmol of 5a as substrate under standard conditions, indicating that this protocol could be followed for scalable performance. Of note, this model reaction was also investigated with chiral TC-7 as the catalyst, and only the racemic product 4aa was afforded (see ESI† E).
 |
| Scheme 4 Scheme for the gram-scale experiment. | |
According to the experiment and previous work,29d a possible mechanism was proposed, as shown in Scheme 5. This catalytic cycle involved two processes, in which amine 6 was produced in situ with B2(OH)4 as the catalyst, followed by condensation with carbonyl compounds to form the imine intermediate A. With the intermediate in hand, the second process occurred successively, in which the Int-I was formed firstly, then followed by transfer hydrogenation to afford the desired product 4 and the catalytic cycle.
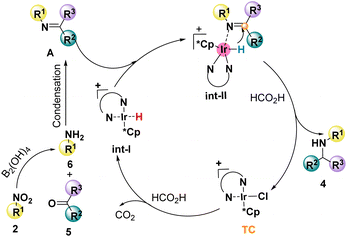 |
| Scheme 5 Proposed mechanism. | |
Conclusions
In summary, we established a new strategy for the synthesis of secondary amines via the iridium-catalysed reductive amination of carbonyl compounds with nitro compounds. A wide range of carbonyl compounds, including aliphatic or aromatic aldehydes and ketones, were well tolerated in this catalytic system, delivering the desired products in moderated to excellent yields under mild conditions. In this transformation, first, the reduction of nitro compounds was realized in situ with B2(OH)4 as the reductant, followed by reductive amination of carbonyl compounds to afford amine products, providing a new one-pot procedure for amine synthesis.
Conflicts of interest
There are no conflicts to declare.
Acknowledgements
This work was supported by the National Natural Science Foundation of China (21961002, 22161004), the Fundamental Research Funds for Gannan Medical University (QD202019, QD202106, and TD2021YX05) and Shaoguan University (408/9900064703) for financial support.
Notes and references
-
(a) C. A. Buehler and D. E. Pearson, Survey of Organic Synthesis, Wiley Interscience, New York, 1970, vol. 1, p. 413 Search PubMed;
(b) O. Mitsunobu, B. M. Trost and I. Fleming, Comprehensive Organic Synthesis, Oxford, 1991, vol. 6, p. 65 Search PubMed;
(c) R. S. Vardanyan and V. J. Hruby, Synthesis of Best-Seller Drugs, Academic Press, Amsterdam, 2016 Search PubMed;
(d) S. A. Lawrence, Amines. Synthesis, Properties and Applications, Cambridge University Press, Cambridge, UK, NY, 2004 Search PubMed.
-
(a) R. N. Salvatore, C. H. Yoon and K. W. Jung, Synthesis of secondary amines, Tetrahedron, 2001, 57, 7785 CrossRef CAS;
(b) J.-C. Castillo, J. Orrego-Hernández and J. Portilla, Cs2CO3-Promoted Direct N-Alkylation: Highly Chemoselective Synthesis of N-Alkylated Benzylamines and Anilines, Eur. J. Org Chem., 2016, 2016, 3824 CrossRef CAS.
-
(a) S. Elangovan, J. Neumann, J. B. Sortais, K. Junge, C. Darcel and M. Beller, Efficient and selective N-alkylation of amines with alcohols catalysed by manganese pincer complexes, Nat. Commun., 2016, 7, 12641 CrossRef PubMed;
(b) S. Rösler, M. Ertl, T. Irrgang and R. Kempe, Cobalt-Catalyzed Alkylation of Aromatic Amines by Alcohols, Angew. Chem., Int. Ed., 2015, 54, 15046 CrossRef PubMed;
(c) M. Mastalir, B. Stöger, E. Pittenauer, M. Puchberger, G. Allmaier and K. Kirchner, Air Stable Iron (II) PNP Pincer Complexes as Efficient Catalysts for the Selective Alkylation of Amines with Alcohols, Adv. Synth. Catal., 2016, 358, 3824 CrossRef CAS.
-
(a) D. S. Surry and S. L. Buchwald, Biaryl phosphane ligands in palladium-catalyzed amination, Angew. Chem., Int. Ed., 2008, 47, 6338 CrossRef CAS PubMed;
(b) J. F. Hartwig, Evolution of a fourth-generation catalyst for the amination and thioetherification of aryl halides, Acc. Chem. Res., 2008, 41, 1534 CrossRef CAS PubMed.
-
(a) S. V. Ley and A. W. Thomas, Modern synthetic methods for copper-mediated C(aryl)[bond]O, C(aryl)[bond]N, and C(aryl)[bond]S bond formation, Angew. Chem., Int. Ed., 2003, 42, 5400 CrossRef CAS PubMed;
(b) S. D. Surry and S. L. Buchwald, Diamine Ligands in Copper-Catalyzed Reactions, Chem. Sci., 2010, 1, 13 RSC.
- E. W. Baxter and A. B. Reitz, Reductive Aminations of Carbonyl Compounds with Borohydride and Borane Reducing Agents. Organic Reactions, John Wiley & Sons, Inc, 2002 Search PubMed.
- R. E. Malz and H. Greenfield, Tertiary Amine Preparation by Reductive Alkylation of Aliphatic Secondary Amines with Ketones, Stud. Surf. Sci. Catal., 1991, 59, 351 CrossRef CAS.
-
(a) S. Das, S. Zhou, D. Addis, S. Enthaler, K. Junge and M. Beller, Selective Catalytic Reductions of Amides and Nitriles to Amines, Top. Catal., 2010, 53, 979 CrossRef CAS;
(b) M. Stein and B. Breit, Catalytic hydrogenation of amides to amines under mild conditions, Angew. Chem., Int. Ed., 2013, 52, 2231 CrossRef CAS PubMed.
-
(a) T. Irrgang and R. Kempe, Transition-Metal-Catalyzed Reductive Amination Employing Hydrogen, Chem. Rev., 2020, 120, 9583 CrossRef CAS PubMed;
(b) R. I. Storer, D. E. Carrera, Y. Ni and D. W. MacMillan, Enantioselective organocatalytic reductive amination, J. Am. Chem. Soc., 2006, 128, 84 CrossRef CAS PubMed;
(c) S. Liang, P. Monsen, G. B. Hammond and B. Xu, Au/TiO2 catalyzed reductive amination of aldehydes and ketones using formic acid as reductant, Org. Chem. Front., 2016, 3, 505 RSC.
-
(a) N. Ono, The nitro group in organic synthesis, John Wiley & Sons, 2003 Search PubMed;
(b) D. Green and T. Johnson, Nitroalkane chemistry, Innovations Pharm. Technol., 2000, 1, 79 Search PubMed.
- R. T. Major, Catalytic reduction of mixtures of para-nitro- and nitrosophenols with aldehydes and ketones, J. Am. Chem. Soc., 1931, 53, 1901 CrossRef CAS.
-
(a) L. Hu, X. Cao, D. Ge, H. Hong, Z. Guo, L. Chen, X. Sun, J. Tang, J. Zheng, J. Lu and H. Gu, Ultrathin platinum nanowire catalysts for direct C-N coupling of carbonyls with aromatic nitro compounds under 1 bar of hydrogen, Chemistry, 2011, 17, 14283 CrossRef CAS PubMed;
(b) F. G. Cirujano, A. Leyva-Pérez, A. Corma, F. Llabrés and F. X. Xamena, MOFs as Multifunctional Catalysts: Synthesis of Secondary Arylamines, Quinolines, Pyrroles, and Arylpyrrolidines over Bifunctional MIL-101, ChemCatChem, 2013, 5, 538 CrossRef CAS.
-
(a) M. O. Sydnes, M. Kuse and M. Isobe, Reductive monoalkylation of nitro aryls in one-pot, Tetrahedron, 2008, 64, 6406 CrossRef CAS;
(b) B. Sreedhar, P. S. Reddy and D. K. Devi, Direct one-pot reductive amination of aldehydes with nitroarenes in a domino fashion: catalysis by gum-acacia-stabilized palladium nanoparticles, J. Org. Chem., 2009, 74, 8806 CrossRef CAS PubMed;
(c) M. M. Dell'anna, P. Mastrorilli, A. Rizzuti and C. Leonelli, Onepot synthesis of aniline derivatives from nitroarenes under mild conditions promoted by a recyclable polymer-supported palladium catalyst, Appl. Catal., A, 2011, 401, 134 CrossRef;
(d) S. Wei, Z. Dong, Z. Ma, J. Sun and J. Ma, Palladium supported on magnetic nanoparticles as recoverable catalyst for one-pot reductive amination of aldehydes with nitroarenes under ambient conditions, Catal. Commun., 2013, 30, 40 CrossRef CAS;
(e) X. Zhou, X. Li, L. Jiao, H. Huo and R. Li, Programmed Synthesis Palladium supported on Fe3O4@C: an efficient and heterogeneous recyclable catalyst for one-pot reductive amination of aldehydes with nitroarenes in aqueous reaction medium, Catal. Lett., 2015, 145, 1591 CrossRef CAS;
(f) H. Sharma, M. Bhardwaj, M. Kour and S. Paul, Highly efficient magnetic Pd (0) nanoparticles stabilized by amine functionalized starch for organic transformations under mild conditions, Mol. Catal., 2017, 435, 58 CrossRef CAS.
- L. Huang, Z. Wang, L. Geng, R. Chen, W. Xing, Y. Wang and J. Huang, Selective and recyclable rhodium nanocatalysts for the reductive N-alkylation of nitrobenzenes and amines with aldehydes, RSC Adv., 2015, 5, 56936 RSC.
-
(a) M. Pintado-Sierra, A. M. Rasero-Almansa, A. Corma, M. Iglesias and F. Sánchez, Bifunctional iridium-(2-aminoterephthalate)-Zr-MOF chemoselective catalyst for the synthesis of secondary amines by one-pot three step cascade reaction, J. Catal., 2013, 299, 137 CrossRef CAS;
(b) D. Sui, F. Mao, H. Fan, Z. Qi and J. Huang, General reductive amination of aldehydes and ketones with amines and nitroaromatics under H2 by recyclable iridium catalysts, Chin. J. Chem., 2017, 35, 1371 CrossRef CAS.
- C. Del Pozo, A. Corma, M. Iglesias and F. Sánchez, Multisite solid (NHC)NN-Ru-catalysts for cascade reactions: synthesis of secondary amines from nitro compounds, J. Catal., 2012, 291, 110 CrossRef CAS.
- E. A. Artiukha, A. L. Nuzhdin, G. A. Bukhtiyarova and V. I. Bukhtiyarov, Flow synthesis of secondary amines over Ag/Al2O3 catalyst by one-pot reductive amination of aldehydes with nitroarenes, RSC Adv., 2017, 7, 45856 RSC.
-
(a) Y. Yamane, X. Liu, A. Hamasaki, T. Ishida, M. Haruta, T. Yokoyama and M. Tokunaga, One-pot synthesis of indoles and aniline derivatives from nitroarenes under hydrogenation condition with supported gold nanoparticles, Org. Lett., 2009, 11, 5162 CrossRef CAS PubMed;
(b) E. A. Artiukha, A. L. Nuzhdin, G. A. Bukhtiyarova, S. Y. Zaytsev, P. E. Plyusnin and Y. V. Shubin, One-pot reductive amination of aldehydes with nitroarenes over an Au/Al2O3 catalyst in a continuous flow reactor, Catal. Sci. Technol., 2015, 5, 4741 RSC.
-
(a) A. M. Fiore, G. Romanazzi, M. M. Dell’anna, M. Latronico, C. Leonelli and M. Mali, Mild and efficient synthesis of secondary aromatic amines by one-pot stepwise reductive amination of arylaldehydes with nitroarenes promoted by reusable nickel nanoparticles, Mol. Catal., 2019, 476, 110507 CrossRef;
(b) J. Li, B. Wang, Y. Qin, Q. Tao and L. Chen, MOF-derived Ni@NC catalyst: synthesis, characterization, and application in one-pot hydrogenation and reductive amination, Catal. Sci. Technol., 2019, 9, 3726 RSC.
-
(a) T. Stemmler, F. A. Westerhaus, A.-E. Surkus, M.-M. Pohl, K. Junge and M. Beller, General and selective reductive amination of carbonyl compounds using a core-shell structured Co3O4/NGr@C catalyst, Green Chem., 2014, 16, 4535 RSC;
(b) R. V. Jagadeesh, K. Murugesan, A. S. Alshammari, H. Neumann, M.-M. Pohl and J. Radnik, J. MOF-derived cobalt nanoparticles catalyze a general synthesis of amines, Science, 2017, 358, 326 CrossRef CAS PubMed;
(c) T. Senthamarai, K. Murugesan, K. Natte, N. V. Kalevaru, H. Neumann and P. C. J. Kamer, Expedient synthesis of N-methyl- and N-alkylamines by reductive amination using reusable cobalt oxide nanoparticles, ChemCatChem, 2018, 10, 1235 CrossRef CAS.
- A. L. Nuzhdin, E. A. Artiukha, G. A. Bukhtiyarova, E. A. Derevyannikova and V. I. Bukhtiyarov, Synthesis of secondary amines by reductive amination of aldehydes with nitroarenes over supported copper catalysts in a flow reactor, Catal. Commun., 2017, 102, 108 CrossRef CAS.
- K. Natte, H. Neumann, R. V. Jagadeesh and M. Beller, Convenient iron catalyzed reductive aminations without hydrogen for selective synthesis of N-methylamines, Nat. Commun., 2017, 8, 1344 CrossRef PubMed.
-
(a) P. Zhou, C. Yu, L. Jiang, K. Lv and Z. Zhang, One-pot reductive amination of carbonyl compounds with nitro compounds with CO/H2O as the hydrogen donor over non-noble cobalt catalyst, J. Catal., 2017, 352, 264 CrossRef CAS;
(b) J. W. Park and Y. K. Chung, Hydrogen-free cobalt-rhodium heterobimetallic nanoparticle-catalyzed reductive amination of aldehydes and ketones with amines and nitroarenes in the presence of carbon monoxide and water, ACS Catal., 2015, 5, 4846 CrossRef CAS.
-
(a) X. Xu, J. Luo, L. Li, D. Zhang, Y. Wang and G. Li, Unprecedented catalytic performance in amine syntheses via Pd/g-C3N4 catalyst assisted transfer hydrogenation, Green Chem., 2018, 20, 2038 RSC;
(b) P. Zhou and Z. Zhang, One-pot reductive amination of carbonyl compounds with nitro compounds by transfer hydrogenation over Co-Nx as catalyst, ChemSusChem, 2017, 10, 1892 CrossRef CAS PubMed;
(c) H. Guo, B. Wang, P. Qiu, R. Gao, M. Sun and L. N. Chen, S codoped carbon shells embedded with ultrafine co NPs for reductive amination with formic acid, ACS Sustain. Chem. Eng., 2019, 7, 8876 CrossRef CAS.
-
(a) R. J. Kalbasi and O. Mazaheri, Synthesis and characterization of hierarchical ZSM-5 zeolite containing Ni nanoparticles for one-pot reductive amination of aldehydes with nitroarenes, Catal. Commun., 2015, 69, 86 CrossRef CAS;
(b) R. J. Kalbasi and O. Mazaheri, Facile one-pot tandem reductive amination of aldehydes from nitroarenes over a hierarchical ZSM-5 zeolite containing palladium nanoparticles, New J. Chem., 2016, 40, 9627 RSC.
- J. W. Bae, Y. J. Cho, S. H. Lee, C.-O. M. Yoon and C. M. Yoon, A one-pot synthesis of -alkylaminobenzenes from nitroaromatics: reduction followed by reductive amination using BH, Chem. Commun., 2000, 19, 1857 RSC.
-
(a) H. Zhou, S. Liu, D. Pi and G. Shen, Water as a hydrogen source in palladium-catalyzed reduction and reductive amination of nitroarenes mediated by diboronic acid, Tetrahedron, 2017, 73, 3898 CrossRef;
(b) M. Jang, T. Lim, B. Y. Park and M. S. Han, Metal-Free, Rapid, and Highly Chemoselective Reduction of Aromatic Nitro Compounds at Room Temperature, J. Org. Chem., 2022, 87, 910 CrossRef CAS PubMed;
(c) D. Chen, Y. Zhou, H. Zhou, S. Liu, Q. Liu, K. Zhang and Y. Uozumi, Metal-free Reduction of Nitro Aromatics to Amines with B2(OH)4/H2O, Synlett, 2018, 29, 1765 CrossRef CAS;
(d) H.-C. Du, N. Simmons, J. C. Faver, Z. Yu, M. Palaniappan, K. Riehle and M. M. Matzuk, A Mild, DNA-Compatible Nitro Reduction Using B2(OH)4, Org. Lett., 2019, 21, 2194 CrossRef CAS PubMed.
-
(a) C.-H. Lin, B.-C. Hong and G.-H. Lee, Asymmetric synthesis of functionalized pyrrolizidines by an organocatalytic and pot-economy strategy, RSC Adv., 2016, 6, 8243 RSC;
(b) A. E. Wahba and M. T. Hamann, Reductive N-alkylation of nitroarenes: a green approach for the N-alkylation of natural products, J. Org. Chem., 2012, 77, 4578 CrossRef CAS PubMed.
-
(a) L. Ouyang, R. Miao, Z. Yang and R. Luo, Iridium-catalyzed reductive amination of carboxylic acids, J. Catal., 2023, 418, 283–289 CrossRef CAS;
(b) L. Ouyang, Y. Xia, R. Miao, J. Liao and R. Luo, Iridium-catalyzed reductive etherification of α,β-unsaturated ketones and aldehydes with alcohols, Org. Biomol. Chem., 2022, 20, 2621 RSC;
(c) Y. Xia, S. Wang, R. Miao, J. Liao, L. Ouyang and R. Luo, Synthesis of N-alkoxy amines and hydroxylamines via the iridium-catalyzed transfer hydrogenation of oximes, Org. Biomol. Chem., 2022, 20, 6394 RSC;
(d) L. Ouyang, Y. Xia, J. Liao and R. Luo, One-Pot Transfer Hydrogenation Reductive Amination of Aldehydes and Ketones by Iridium Complexes “on Water”, Eur. J. Org Chem., 2020, 2020, 6387 CrossRef CAS;
(e) N. Luo, J. Liao, L. Ouyang, H. Wen, J. Liu, W. Tang and R. Luo, Highly pH-Dependent Chemoselective Transfer Hydrogenation of α,β-Unsaturated Aldehydes in Water, ACS Omega, 2019, 38, 3025 CAS.
|
This journal is © The Royal Society of Chemistry 2023 |
Click here to see how this site uses Cookies. View our privacy policy here.