DOI:
10.1039/D3SC04123A
(Edge Article)
Chem. Sci., 2023,
14, 13842-13850
Triplet dynamic nuclear polarization of pyruvate via supramolecular chemistry†
Received
8th August 2023
, Accepted 23rd October 2023
First published on 21st November 2023
Abstract
Dynamic nuclear polarization (DNP) significantly improves the sensitivity of magnetic resonance imaging, and its most important medical application is cancer diagnosis via hyperpolarized 13C-labeled pyruvate. Unlike cryogenic DNP, triplet-DNP uses photoexcited triplet electrons under mild conditions. However, triplet-DNP of pyruvate has not been observed because of incompatibility of the hydrophobic polarizing agent with hydrophilic pyruvate. This work demonstrates that supramolecular complexation with β-cyclodextrin can disperse 4,4′-(pentacene-6,13-diyl)dibenzoate (NaPDBA), a pentacene derivative with hydrophilic substituents, even in the presence of high sodium pyruvate concentrations. The polarization of photoexcited triplet electron spins in NaPDBA was transferred to the 13C spins of sodium pyruvate via triplet-DNP of 1H spins in water and 1H-to-13C cross-polarization. This provides an important step toward the widespread use of ultra-sensitive MRI for cancer diagnosis.
Introduction
Nuclear magnetic resonance (NMR) is an important non-destructive technique for analyzing chemical structures, and magnetic resonance imaging (MRI) is an essential medical procedure. However, both methods are inherently insensitive because the sensitivity is proportional to spin polarization, which is 0.004% for 1H spins and 0.001% for 13C spins under a 6 T magnetic field at room temperature. Hence, MRI is essentially limited to abundant water molecules.
Dynamic nuclear polarization (DNP) improves the sensitivity of NMR1–10 because it creates a hyperpolarized nuclear spin state by transferring the high polarization of unpaired electron spins. Dissolution-DNP11–13 rapidly dissolves hyperpolarized solid samples and has been used for various NMR analyses, such as highly sensitive protein NMR14,15 and in vivo metabolite imaging.16–20 In particular, [1-13C] pyruvate is the most important probe for high-sensitivity MRI because it is at the center of metabolism and its metabolic kinetics are used in the diagnosis of various diseases, including cancer.16,17,21–24 Dissolution-DNP has achieved 13C-NMR signal enhancement of more than 10
000-fold;25 however, it requires severe conditions, such as a high magnetic field (∼7 T) and cryogenic temperatures near 1 K, to use the near-unity electron spin polarization. Alternatively, Overhauser-DNP is a powerful technique that polarizes solutions even at room temperature,26–29 but the maximum enhancement factor depends on the difference in the gyromagnetic ratio between nuclear spins and electron spins (γe/γH ∼ 660 and γe/γC ∼ 2600).
In contrast, DNP via photoexcited triplet electron spins (triplet-DNP) can produce hyperpolarization under milder conditions.30–34 Photoexcited triplets have large non-equilibrium spin polarizations (∼70% for pentacene35) that are independent of temperature, which enables DNP at higher temperatures and lower magnetic fields. Fig. 1A depicts triplet-DNP. After photoexcitation of a polarizing agent, a temporary spin-polarized state is generated by spin-selective intersystem crossing. After the polarization is transferred from the electron spin to 1H spin, it is propagated throughout the solid via1H spin diffusion. Various host molecules and materials have been examined to hyperpolarize drugs,36–38 water,39–42 and biologically relevant molecules.43,44 Dissolution triplet-DNP has been demonstrated.45,46 However, spin-polarization transfer to lower gyromagnetic ratio spins such as in 19F and 13C via cross polarization (CP) has been limited to aromatic molecules,33,36,37,47 and triplet-DNP of [1-13C] pyruvate has never been demonstrated because of poor miscibility between the hydrophobic polarizing agent and hydrophilic pyruvate.
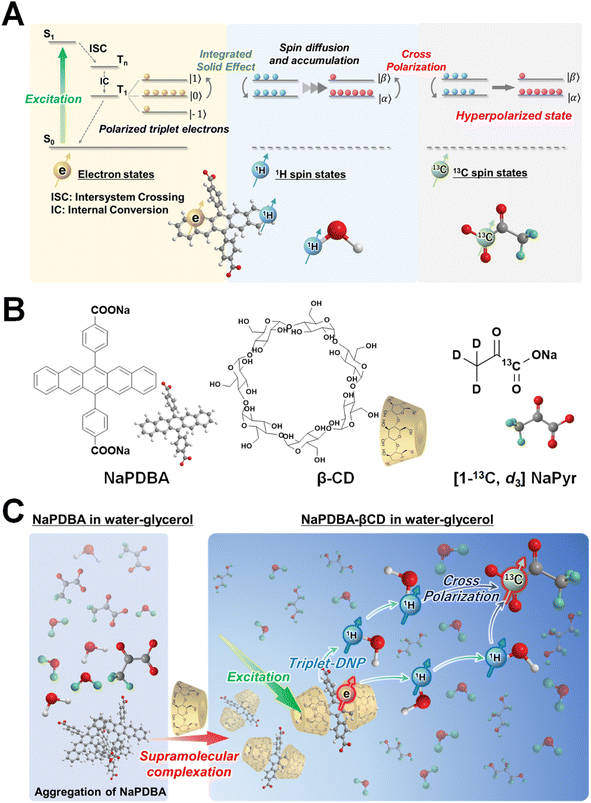 |
| Fig. 1 Hyperpolarization of [1-13C, d3] sodium pyruvate (NaPyr) by triplet dynamic nuclear polarization (DNP) and cross polarization (CP). (A) Scheme of triplet-DNP and CP. (B) Molecular structures of NaPDBA, βCD, and [1-13C, d3] NaPyr. (C) NaPDBA aggregate in DNP juice in the absence of β-cyclodextrin (βCD), but the dispersibility is significantly increased by supramolecular complexation with βCD. Polarization transfer from photoexcited triplet electron spins to 1H spins of water and then to 13C spins of [1-13C, d3] NaPyr. | |
Here, we report triplet-DNP of [1-13C, d3] sodium pyruvate (NaPyr) at ∼100 K and 0.64 T. Higher concentrations of NaPyr are desired for dissolution-DNP application because the polarized spins are diluted after dissolution.20 Water-soluble polarizing agents were developed and used for triplet-DNP in aqueous matrices,41,43,48 but hydrophilic polarizing agents easily aggregate with high concentrations of polar pyruvate. To solve this problem, we used supramolecular chemistry to increase the dispersion of the polarizing agent (Fig. 1B). Cyclodextrin can encapsulate hydrophobic dyes in water,49,50 and the triplet-DNP of water was observed by using 4,4′-(pentacene-6,13-diyl)dibenzoate (NaPDBA) as a guest in cyclodextrin.48 NaPDBA aggregation was prevented by supramolecular complexation with β-cyclodextrin (βCD) with a saturated concentration of 1.5 M NaPyr in DNP juice (H2O/D2O/glycerol-d8 = 1/3/6, v/v/v), a general glass forming solvent.20 The well-dispersed NaPDBA–βCD complex generated polarization of triplet electron spins, which was transferred to the 1H spins of water in DNP juice by triplet-DNP and then to the 13C spins of [1-13C, d3] NaPyr by CP (Fig. 1C).
Results and discussion
Evaluation of dispersibility of polarizing agents
The dispersed state of NaPDBA in DNP juice was evaluated via absorption spectra (Fig. 2) since random aggregation of polarizing agents could induce rapid relaxation of photoexcited triplet electron-spin polarization, significantly decreasing triplet-DNP efficiency. The π–π* absorption peak of 1 mM dispersed NaPDBA in methanol was observed at 593 nm (Fig. S5†). A red-shift to 604 nm was observed in DNP juice, indicating NaPDBA aggregation (Fig. S6†).48 When 1.5 M of NaPyr was dissolved in DNP juice, the NaPDBA peak exhibited a large red shift to 614.5 nm, turning the solution blue (Fig. 2). This indicated that the NaPyr salt disrupted NaPDBA hydration and increased its aggregation.51 The absorption peak was blue-shifted to 603 nm by adding 5 mM of βCD in DNP juice, in the presence of NaPyr (Fig. 2 and S7†). Previously, the 1
:
2 inclusion complex of NaPDBA–βCD was formed in a water–glycerol mixture [glycerol/H2O (5/5, v/v)],48 and the absorption peak was also blue-shifted by adding 5 mM of βCD in DNP juice [glycerol/H2O (6/4, v/v)] in the absence of NaPyr (Fig. S8†). No significant change in the absorption spectra of NaPDBA–βCD in DNP juice was observed with or without NaPyr (Fig. 2). Here, the NaPDBA–βCD inclusion complex was intact at high NaPyr concentrations because the addition of salt increased water structuring and shifted the equilibrium toward the bound state.52,53
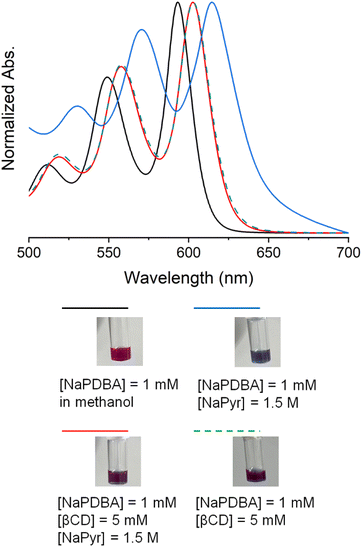 |
| Fig. 2 Dispersion of NaPDBA in the presence of NaPyr. Absorption spectra of NaPDBA in methanol (black line), NaPDBA in DNP juice containing NaPyr (blue line), the NaPDBA–βCD complex in DNP juice (dashed green line) and the NaPDBA–βCD complex in DNP juice containing NaPyr (red line) at room temperature. The concentrations of NaPDBA, NaPyr, and βCD were 1 mM, 1.5 M and 5 mM, respectively. Photographs of each solution are shown. | |
Evaluation of supramolecular structures
The structure of the NaPDBA–βCD inclusion complex was investigated with NMR and molecular dynamics (MD) simulations. The room-temperature 1H NMR spectra of NaPDBA shifted upfield when adding βCD in glycerol-d8/D2O (6/4, v/v) that contained NaPyr, which indicated formation of the inclusion complex (Fig. S9†).54,55 Nuclear-Overhauser-effect room-temperature NMR of NaPDBA and βCD in D2O with NaPyr revealed cross peaks between the NaPDBA pentacene skeleton and the βCD inner region (Fig. S10†). This indicated that NaPDBA was incorporated into the βCD hydrophobic cavity. NMR spectra of NaPDBA and βCD with different concentrations in D2O which contained NaPyr indicated a 1
:
2 molar ratio of NaPDBA to βCD in the inclusion complex (Fig. S11†). In the MD simulations, the 1
:
2 inclusion complex was stable in glycerol/H2O (6/4, v/v) (Fig. 3A and B), and remained stable in the presence of 1.5 M NaPyr (Fig. 3C and D). These results were consistent with the dispersibility of the NaPDBA–βCD complex with and without NaPyr.
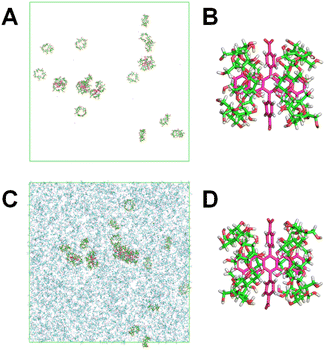 |
| Fig. 3 Molecular dynamics (MD) simulations of NaPDBA–βCD supramolecular complexes. (A and B) MD simulation snapshots of the NaPDBA–βCD complex ([NaPDBA] = 1 mM and [βCD] = 5 mM) in glycerol/H2O (6/4, v/v) at 300 K. Glycerol and H2O were omitted for clarity. (C and D) MD simulation snapshots of the NaPDBA–βCD complex with NaPyr ([NaPDBA] = 1 mM [βCD] = 5 mM and [NaPyr] = 1.5 M) in glycerol/H2O (6/4, v/v) at 300 K. Glycerol and H2O are omitted for clarity. | |
Time-resolved ESR measurements
The polarization of photoexcited triplet electron spins in NaPDBA in DNP juice was evaluated by time-resolved X-band electron spin resonance (ESR) at 140 K (Fig. 4) after 527 nm pulsed-laser excitation. Almost no ESR signal was observed for NaPDBA in DNP juice containing NaPyr in the absence of βCD (Fig. 4A). This was because of random NaPDBA aggregation, which induced rapid relaxation of the triplet electron polarization. When a chromophore aggregate is in a random orientation, the photoexcited triplet electron spins hop between chromophores with different orientations, causing the electron spins subject to magnetic field fluctuations and inducing relaxation of electron spin polarization.56,57 In contrast, the NaPDBA–βCD complex exhibited a clear ESR signal in DNP juice, even with NaPyr, reflecting well-dispersed NaPDBA via supramolecular complexation. The ESR spectra were fitted with the EasySpin toolbox in MATLAB.58 The zero-field splitting parameters and relative populations of the NaPDBA–βCD complex were almost the same with and without NaPyr, and were almost the same as those for dispersed pentacene and its derivatives (Table S1†).59,60 This confirmed that the supramolecular structure was intact in the presence of NaPyr, consistent with the absorption measurements. Fig. 4B shows the signal decay of the ESR absorption peak. The NaPDBA–βCD complex had 27 μs and 29 μs polarization lifetimes in the presence and absence of NaPyr, respectively. These lifetimes were long enough to use the triplet-DNP sequence.
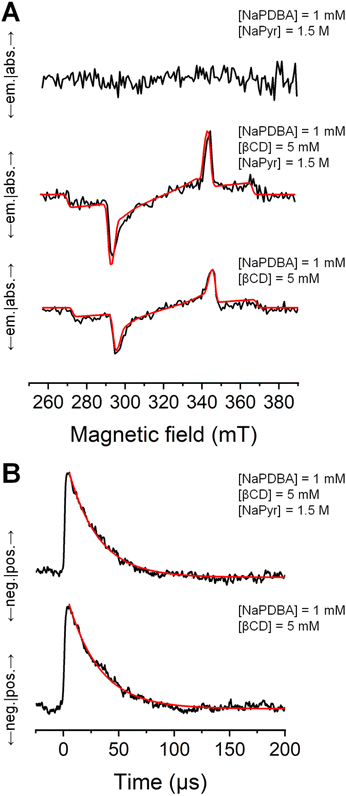 |
| Fig. 4 Time-resolved electron spin resonance (ESR) spectra of NaPDBA and peak signal decays in DNP juice (glycerol/H2O (6/4, v/v)). (A) ESR spectra of NaPDBA containing NaPyr (top), the NaPDBA–βCD complex containing NaPyr (middle), and the NaPDBA–βCD complex (bottom) after 527 nm photoexcitation at 140 K. The concentrations of NaPDBA, NaPyr and βCD were 1 mM, 1.5 M and 5 mM, respectively. Spectra were fitted with the EasySpin toolbox in MATLAB (red lines). (B) Decays of peak ESR signals (black lines). Single-exponential fits are also shown (red lines). | |
Evaluation of the spin–lattice relaxation time
Because the accumulation of spin polarization via triplet-DNP competes with 1H spin relaxation, a solid sample must have a sufficiently long spin–lattice relaxation time (T1) for efficient triplet-DNP. The 1H T1 of glassy DNP juice with NaPDBA and βCD was 46 s at 100 K and 0.64 T, while the addition of NaPyr significantly decreased it to 2 s (Fig. S12†). This was because of increased 1H spin relaxation via methyl group rotation in NaPyr.61 The use of deuterated sodium pyruvate (d3-NaPyr) recovered the 1H T1 (26 s), and the difference from 1H T1 without NaPyr (46 s) may have been because of remaining 1H spins in the methyl groups of [1-13C, d3] NaPyr.
DNP measurements
Polarization of the photoexcited NaPDBA triplet electron spins was transferred to the 1H spins of water in DNP juice [glycerol-d8/D2O/H2O, 60/30/10, (v/v/v)] by the ISE sequence at 100 K and 0.64 T (see the ESI for details, Fig. S13†).31,34 NaPDBA was photoexcited with a 527 nm pulsed laser to produce the polarized electron spins. Then, 17.3 GHz microwaves were irradiated to transfer the polarization from electron spins to 1H spins by matching the frequency of the electron spin in the effective magnetic field in the rotating frame with the Larmor frequency of the 1H spins in the laboratory frame. The magnetic field was swept during microwave irradiation to use more electron spin packets, since the resonant field of the triplet electron spins is broadened by the random orientation of the polarizing agents and hyperfine interactions. By repeating the ISE sequence at 500 Hz, the 1H spins were polarized throughout the glassy DNP juice until the accumulation of 1H spin polarization reaches equilibrium with the spin–lattice relaxation. After the triplet-DNP process in a microwave resonator, the sample was shuttled within 1 s to a coil via a motor to acquire 1H NMR. Fig. 5A shows 1H NMR of DNP juice containing 1 mM NaPDBA, 5 mM βCD, and 1.5 M [1-13C, d3] NaPyr obtained at the thermal state after 2 min of triplet-DNP, in which the accumulation of spin polarization and spin relaxation reached equilibrium. Enhancement factors of 58 and 174 were obtained relative to thermal equilibria at 100 K and room temperature, respectively (Fig. 5B).
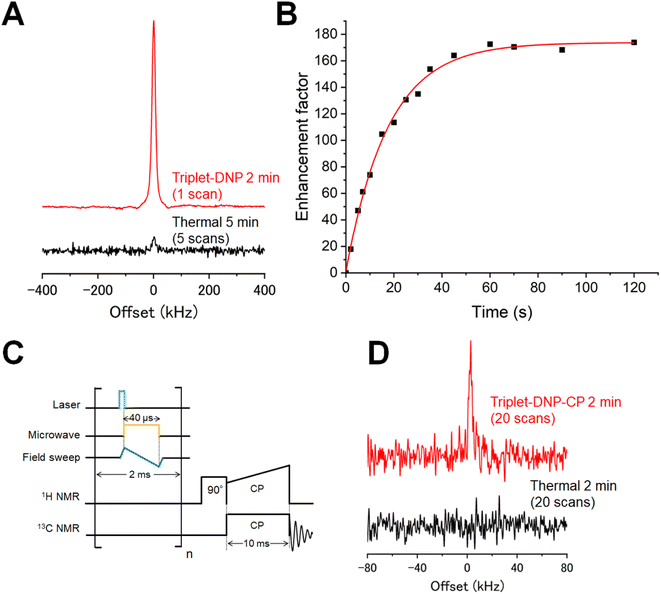 |
| Fig. 5 (A) 1H NMR signals of water in DNP juice (glycerol-d8/D2O/H2O, 60/30/10, v/v/v) containing NaPDBA, βCD and [1-13C, d3] NaPyr under thermal conditions (five scans every 5 min) and after triplet-DNP (integrated solid effect sequence for 2 min and 1 scan) at 100 K. (B) 1H polarization buildup curve of DNP juice containing NaPDBA, βCD and [1-13C, d3] NaPyr at 100 K. The enhancement factors were calculated by comparing the peak areas after triplet-DNP with that of thermal equilibrium. The enhancement factor relative to thermal equilibrium at room temperature is shown. (C) Sequence of triplet-DNP and ramped-amplitude cross-polarization (RAMP-CP). (D) 6.864 MHz 13C NMR spectra of [1-13C, d3] NaPyr. The red line shows the spectra after triplet-DNP and RAMP-CP (integrated solid effect sequence for 2 min, followed by RAMP-CP, 20 scans) and the black line shows the spectra after RAMP-CP with thermal 1H spins (20 scans). | |
The 1H spin polarization in DNP juice was transferred intermolecularly to 13C spins in [1-13C, d3] NaPyr with a ramped-amplitude cross-polarization (RAMP-CP) sequence (Fig. 5C).62,63 After triplet-DNP at 100 K and 0.64 T, the sample was shuttled into a double-resonance coil with 27.30 MHz and 6.864 MHz resonance frequencies for 1H and 13C spins, respectively. Polarization transfer from 1H spins to 13C spins was conducted using a 10 ms contact. The irradiated field for the 13C spins was fixed at 20 kHz, while that for the 1H spins was swept over the range of 15–25 kHz. After triplet-DNP and RAMP-CP, enhanced 13C NMR of [1-13C, d3] NaPyr was observed (Fig. 5D). The enhancement was clear from the fact that no 13C NMR peak was observed via RAMP-CP when using 1H spins at thermal equilibrium. 13C-methanol at room temperature was used as a reference, and an enhancement factor of 122 was estimated (Fig. S14†). 13C spins in [1-13C, d3] NaPyr accounted for ∼85% of the total 13C spins in the entire solid sample; thus, the polarization enhancement factor of the 13C spins in [1-13C, d3] NaPyr should have been close to 122. Because the theoretical 13C NMR enhancement was ∼4 times (γH/γC) that of 1H NMR with ideal CP, the CP efficiency was 18%. The low efficiency could be attributed to the absence of 1H spins in [1-13C, d3] NaPyr, because 40–60% efficiencies were observed when 1H spins were present in the target molecule.64 Furthermore, ideal CP requires that the radiofrequency pulse amplitude be stronger than the 1H NMR linewidth (∼50 kHz).65 A higher CP efficiency could be obtained by using a stronger radiofrequency pulse.
Conclusions
In conclusion, triplet-DNP of [1-13C, d3] NaPyr, the most important biomolecular probe in MRI applications, was observed by increasing the polarizing agent dispersion via supramolecular complexation. It is important to perform DNP with highly concentrated NaPyr, but the method to disperse the polarizing agent in such a highly polar medium had not been clear. Here, the polarizing agent could be modified with ionic carboxylate moieties and then complexed in supramolecular cyclodextrin, which enabled adequate dispersion. This enabled hyperpolarization of the [1-13C, d3] NaPyr 13C spins via triplet-DNP-CP under a low magnetic field (∼0.64 T) and above liquid nitrogen temperatures (∼100 K). Since the final polarization obtained by triplet-DNP is determined by the build up time constant, Tb, and T1, higher 1H and 13C spin polarizations require a shorter Tb and longer T1. Recently, novel pentacene derivatives showing sharper ESR lines exhibits a shorter Tb than pentacene and the 1H spin polarization is four times higher than that of pentacene.66 In addition, the polarization increased 10-fold as T1 increased from 1 to 3 min, and a spin polarization of 8% was achieved in a model amorphous matrix.66 Higher 1H and 13C spin polarization can be obtained by using such a new polarizing agent instead of NaPDBA to obtain shorter Tb and by diluting the 1H spins or using other matrices with longer T1. In addition, the implementation of more advanced polarization transfer methods with field/frequency modulation would improve the final spin polarization.67,68 Hyperpolarized MRI with triplet-DNP will be possible by combining the optimized molecular design of polarizing agents with the key finding of the present study that supramolecular complexation is useful to hyperpolarize NaPyr at high concentrations.
Data availability
All experimental data are available in the article and ESI.†
Author contributions
T. H., Y. K., H. K. and N. Y. designed the research. T. H. and S. K. prepared and characterized the materials. K. T. and T. U. built time-resolved ESR and DNP setups. T. H. and S. K. conducted time-resolved ESR measurements. T. H. and K. N. conducted DNP measurements. S. S. and G. W. carried out MD simulation. T. H., K. N. and N. Y. wrote the manuscript with contributions from all authors.
Conflicts of interest
There are no conflicts to declare.
Acknowledgements
This work was partially supported by the JST-PRESTO program on “Creation of Life Science Basis by Using Quantum Technology” (JPMJPR18GB), JST-FOREST Program (JPMJFR201Y), JSPS KAKENHI (JP20H02713, JP22K19051, JP21J13049, and JP22J21293), the Shinnihon Foundation of Advanced Medical Treatment Research, RIKEN-Kyushu Univ. Science and Technology Hub Collaborative Research Program, and the RIKEN Cluster for Science, Technology and Innovation Hub (RCSTI).
Notes and references
- T. R. Carver and C. P. Slichter, Polarization of Nuclear Spins in Metals, Phys. Rev., 1953, 92, 212–213 CrossRef CAS
.
- A. W. Overhauser, Polarization of Nuclei in Metals, Phys. Rev., 1953, 92, 411–415 CrossRef CAS
.
- D. A. Hall, D. C. Maus, G. J. Gerfen, S. J. Inati, L. R. Becerra, F. W. Dahlquist and R. G. Griffin, Polarization-enhanced NMR spectroscopy of biomolecules in frozen solution, Science, 1997, 276, 930–932 CrossRef CAS
.
- V. S. Bajaj, M. L. Mak-Jurkauskas, M. Belenky, J. Herzfeld and R. G. Griffin, Functional and shunt states of bacteriorhodopsin resolved by 250 GHz dynamic nuclear polarization–enhanced solid-state NMR, Proc. Natl. Acad. Sci. U. S. A., 2009, 106, 9244–9249 CrossRef CAS
.
- A. Lesage, M. Lelli, D. Gajan, M. A. Caporini, V. Vitzthum, P. Miéville, J. Alauzun, A. Roussey, C. Thieuleux, A. Mehdi, G. Bodenhausen, C. Coperet and L. Emsley, Surface Enhanced NMR Spectroscopy by Dynamic Nuclear Polarization, J. Am. Chem. Soc., 2010, 132, 15459–15461 CrossRef CAS
.
- J. M. Franck, A. Pavlova, J. A. Scott and S. Han, Quantitative cw Overhauser effect dynamic nuclear polarization for the analysis of local water dynamics, Prog. Nucl. Magn. Reson. Spectrosc., 2013, 74, 33–56 CrossRef CAS
.
- A. Ajoy, K. Liu, R. Nazaryan, X. Lv, P. R. Zangara, B. Safvati, G. Wang, D. Arnold, G. Li, A. Lin, P. Raghavan, E. Druga, S. Dhomkar, D. Pagliero, J. A. Reimer, D. Suter, C. A. Meriles and A. Pines, Orientation-independent room temperature optical 13C hyperpolarization in powdered diamond, Sci. Adv., 2018, 4, eaar5492 CrossRef
.
- D. Dai, X. Wang, Y. Liu, X. L. Yang, C. Glaubitz, V. Denysenkov, X. He, T. Prisner and J. Mao, Room-temperature dynamic nuclear polarization enhanced NMR spectroscopy of small biological molecules in water, Nat. Commun., 2021, 12, 6880 CrossRef CAS
.
- A. Bertarello, P. Berruyer, M. Artelsmair, C. S. Elmore, S. Heydarkhan-Hagvall, M. Schade, E. Chiarparin, S. Schantz and L. Emsley, In-Cell Quantification of Drugs by Magic-Angle Spinning Dynamic Nuclear Polarization NMR, J. Am. Chem. Soc., 2022, 144, 6734–6741 CrossRef CAS
.
- T. El Daraï, S. F. Cousin, Q. Stern, M. Ceillier, J. Kempf, D. Eshchenko, R. Melzi, M. Schnell, L. Gremillard, A. Bornet, J. Milani, B. Vuichoud, O. Cala, D. Montarnal and S. Jannin, Porous functionalized polymers enable generating and transporting hyperpolarized mixtures of metabolites, Nat. Commun., 2021, 12, 4695 CrossRef
.
- J. H. Ardenkjaer-Larsen, B. Fridlund, A. Gram, G. Hansson, L. Hansson, M. H. Lerche, R. Servin, M. Thaning and K. Golman, Increase in signal-to-noise ratio of > 10,000 times in liquid-state NMR, Proc. Natl. Acad. Sci. U. S. A., 2003, 100, 10158–10163 CrossRef CAS
.
- J. H. Ardenkjær-Larsen, On the present and future of dissolution-DNP, J. Magn. Reson., 2016, 264, 3–12 CrossRef
.
- S. Jannin, J. N. Dumez, P. Giraudeau and D. Kurzbach, Application and methodology of dissolution dynamic nuclear polarization in physical, chemical and biological contexts, J. Magn. Reson., 2019, 305, 41–50 CrossRef CAS
.
- P. Kadeřávek, F. Ferrage, G. Bodenhausen and D. Kurzbach, High-Resolution NMR of Folded Proteins in Hyperpolarized Physiological Solvents, Chem.–Eur. J., 2018, 24, 13418–13423 CrossRef
.
- J. Kim, R. Mandal and C. Hilty, Observation of Fast Two-Dimensional NMR Spectra during Protein Folding Using Polarization Transfer from Hyperpolarized Water, J. Phys. Chem. Lett., 2019, 10, 5463–5467 CrossRef
.
- S. J. Nelson, J. Kurhanewicz, D. B. Vigneron, P. E. Larson, A. L. Harzstark, M. Ferrone, M. van Criekinge, J. W. Chang, R. Bok, I. Park, G. Reed, L. Carvajal, E. J. Small, P. Munster, V. K. Weinberg, J. H. Ardenkjær-Larsen, A. P. Chen, R. E. Hurd, L. I. Odegardstuen, F. J. Robb, J. Tropp and J. A. Murray, Metabolic imaging of patients with prostate cancer using hyperpolarized [1-13C]pyruvate, Sci. Transl. Med., 2013, 5, 198ra108 Search PubMed
.
- K. Golman, R. in 't Zandt and M. Thaning, Real-time metabolic imaging, Proc. Natl. Acad. Sci. U. S. A., 2006, 103, 11270–11275 CrossRef CAS
.
- F. A. Gallagher, M. I. Kettunen, S. E. Day, D. E. Hu, J. H. Ardenkjær-Larsen, R. Zandt, P. R. Jensen, M. Karlsson, K. Golman, M. H. Lerche and K. M. Brindle, Magnetic resonance imaging of pH in vivo using hyperpolarized 13C-labelled bicarbonate, Nature, 2008, 453, 940–943 CrossRef CAS
.
- K. R. Keshari, D. M. Wilson, A. P. Chen, R. Bok, P. E. Larson, S. Hu, M. Van Criekinge, J. M. Macdonald, D. B. Vigneron and J. Kurhanewicz, Hyperpolarized [2-13C]-fructose: a hemiketal DNP substrate for in vivo metabolic imaging, J. Am. Chem. Soc., 2009, 131, 17591–17596 CrossRef CAS
.
- K. R. Keshari and D. M. Wilson, Chemistry and biochemistry of 13C hyperpolarized magnetic resonance using dynamic nuclear polarization, Chem. Soc. Rev., 2014, 43, 1627–1659 RSC
.
- S. E. Day, M. I. Kettunen, F. A. Gallagher, D.-E. Hu, M. Lerche, J. Wolber, K. Golman, J. H. Ardenkjaer-Larsen and K. M. Brindle, Detecting tumor response to treatment using hyperpolarized 13C magnetic resonance imaging and spectroscopy, Nat. Med., 2007, 13, 1382–1387 CrossRef CAS
.
- A. Z. Lau, A. P. Chen, N. R. Ghugre, V. Ramanan, W. W. Lam, K. A. Connelly, G. A. Wright and C. H. Cunningham, Rapid multislice imaging of hyperpolarized 13C pyruvate and bicarbonate in the heart, Magn. Reson. Med., 2010, 64, 1323–1331 CrossRef CAS
.
- A. W. Barb, S. K. Hekmatyar, J. N. Glushka and J. H. Prestegard, Probing alanine transaminase catalysis with hyperpolarized 13CD3-pyruvate, J. Magn. Reson., 2013, 228, 59–65 CrossRef CAS
.
- M. Liu and C. Hilty, Metabolic Measurements of Nonpermeating Compounds in Live Cells Using Hyperpolarized NMR, Anal. Chem., 2018, 90, 1217–1222 CrossRef CAS
.
- S. Jannin, J.-N. Dumez, P. Giraudeau and D. Kurzbach, Application and methodology of dissolution dynamic nuclear polarization in physical, chemical and biological contexts, J. Magn. Reson., 2019, 305, 41–50 CrossRef CAS
.
- B. D. Armstrong and S. Han, Overhauser Dynamic Nuclear Polarization To Study Local Water Dynamics, J. Am. Chem. Soc., 2009, 131, 4641–4647 CrossRef CAS
.
- C. Griesinger, M. Bennati, H. M. Vieth, C. Luchinat, G. Parigi, P. Höfer, F. Engelke, S. J. Glaser, V. Denysenkov and T. F. Prisner, Dynamic nuclear polarization at high magnetic fields in liquids, Prog. Nucl. Magn. Reson. Spectrosc., 2012, 64, 4–28 CrossRef CAS
.
- J. G. Krummenacker, V. P. Denysenkov, M. Terekhov, L. M. Schreiber and T. F. Prisner, DNP in MRI: an in-bore approach at 1.5T, J. Magn. Reson., 2012, 215, 94–99 CrossRef CAS
.
- D. Dai, X. Wang, Y. Liu, X.-L. Yang, C. Glaubitz, V. Denysenkov, X. He, T. Prisner and J. Mao, Room-temperature dynamic nuclear polarization enhanced NMR spectroscopy of small biological molecules in water, Nat. Commun., 2021, 12, 6880 CrossRef CAS
.
- A. Henstra, P. Dirksen and W. T. Wenckebach, Enhanced dynamic nuclear-polarization by the integrated solid effect, Phys. Lett. A, 1988, 134, 134–136 CrossRef CAS
.
- A. Henstra, T.-S. Lin, J. Schmidt and W. T. Wenckebach, High dynamic nuclear polarization at room temperature, Chem. Phys. Lett., 1990, 165, 6–10 CrossRef CAS
.
- M. Iinuma, Y. Takahashi, I. Shake, M. Oda, A. Masaike, T. Yabuzaki and H. M. Shimizu, Proton polarization with p-terphenyl crystal by integrated solid effect on photoexcited triplet state, J. Magn. Reson., 2005, 175, 235–241 CrossRef CAS
.
- K. Tateishi, M. Negoro, S. Nishida, A. Kagawa, Y. Morita and M. Kitagawa, Room temperature hyperpolarization of nuclear spins in bulk, Proc. Natl. Acad. Sci. U. S. A., 2014, 111, 7527–7530 CrossRef CAS
.
- K. Nishimura, H. Kouno, Y. Kawashima, K. Orihashi, S. Fujiwara, K. Tateishi, T. Uesaka, N. Kimizuka and N. Yanai, Materials chemistry of triplet dynamic nuclear polarization, Chem. Commun., 2020, 56, 7217–7232 RSC
.
- D. J. Sloop, H. L. Yu, T. S. Lin and S. Weissman, Electron spin echoes of a photoexcited triplet: pentacene in p-terphenyl crystals, J. Chem. Phys., 1981, 75, 3746–3757 CrossRef CAS
.
- K. Tateishi, M. Negoro, A. Kagawa and M. Kitagawa, Dynamic nuclear polarization with photoexcited triplet electrons in a glassy matrix, Angew Chem. Int. Ed. Engl., 2013, 52, 13307–13310 CrossRef CAS
.
- S. Fujiwara, N. Matsumoto, K. Nishimura, N. Kimizuka, K. Tateishi, T. Uesaka and N. Yanai, Triplet Dynamic Nuclear Polarization of Guest Molecules through Induced Fit in a Flexible Metal-Organic Framework, Angew Chem. Int. Ed. Engl., 2021, e202115792, DOI:10.1002/anie.202115792
.
- S. Fujiwara, M. Hosoyamada, K. Tateishi, T. Uesaka, K. Ideta, N. Kimizuka and N. Yanai, Dynamic Nuclear Polarization of Metal-Organic Frameworks Using Photoexcited Triplet Electrons, J. Am. Chem. Soc., 2018, 140, 15606–15610 CrossRef CAS
.
- K. Nishimura, H. Kouno, K. Tateishi, T. Uesaka, K. Ideta, N. Kimizuka and N. Yanai, Triplet dynamic nuclear polarization of nanocrystals dispersed in water at room temperature, Phys. Chem. Chem. Phys., 2019, 21, 16408–16412 RSC
.
- K. Tateishi, M. Negoro, H. Nonaka, A. Kagawa, S. Sando, S. Wada, M. Kitagawa and T. Uesaka, Dynamic nuclear polarization with photo-excited triplet electrons using 6,13-diphenylpentacene, Phys. Chem. Chem. Phys., 2019, 21, 19737–19741 RSC
.
- H. Kouno, K. Orihashi, K. Nishimura, Y. Kawashima, K. Tateishi, T. Uesaka, N. Kimizuka and N. Yanai, Triplet dynamic nuclear polarization of crystalline ice using water-soluble polarizing agents, Chem. Commun., 2020, 56, 3717–3720 RSC
.
- N. Matsumoto, K. Nishimura, N. Kimizuka, Y. Nishiyama, K. Tateishi, T. Uesaka and N. Yanai, Proton Hyperpolarization Relay from Nanocrystals to Liquid Water, J. Am. Chem. Soc., 2022, 144, 18023–18029 CrossRef CAS
.
- T. Hamachi, K. Nishimura, H. Kouno, Y. Kawashima, K. Tateishi, T. Uesaka, N. Kimizuka and N. Yanai, Porphyrins as Versatile, Aggregation-Tolerant, and Biocompatible Polarizing Agents for Triplet Dynamic Nuclear Polarization of Biomolecules, J. Phys. Chem. Lett., 2021, 12, 2645–2650 CrossRef CAS
.
- T. R. Eichhorn, A. J. Parker, F. Josten, C. Müller, J. Scheuer, J. M. Steiner, M. Gierse, J. Handwerker, M. Keim, S. Lucas, M. U. Qureshi, A. Marshall, A. Salhov, Y. Quan, J. Binder, K. D. Jahnke, P. Neumann, S. Knecht, J. W. Blanchard, M. B. Plenio, F. Jelezko, L. Emsley, C. C. Vassiliou, P. Hautle and I. Schwartz, Hyperpolarized Solution-State NMR Spectroscopy with Optically Polarized Crystals, J. Am. Chem. Soc., 2022, 144, 2511–2519 CrossRef CAS
.
- M. Negoro, A. Kagawa, K. Tateishi, Y. Tanaka, T. Yuasa, K. Takahashi and M. Kitagawa, Dissolution Dynamic Nuclear Polarization at Room Temperature Using Photoexcited Triplet Electrons, J. Phys. Chem. A, 2018, 122, 4294–4297 CrossRef CAS
.
- A. Kagawa, K. Miyanishi, N. Ichijo, M. Negoro, Y. Nakamura, H. Enozawa, T. Murata, Y. Morita and M. Kitagawa, High-field NMR with dissolution triplet-DNP, J. Magn. Reson., 2019, 309, 106623 CrossRef CAS
.
- A. Kagawa, M. Negoro, R. Ohba, N. Ichijo, K. Takamine, Y. Nakamura, T. Murata, Y. Morita and M. Kitagawa, Dynamic nuclear polarization using photoexcited triplet electron spins in eutectic mixtures, J. Phys. Chem. A, 2018, 122, 9670–9675 CrossRef CAS
.
- Y. Kawashima, T. Hamachi, A. Yamauchi, K. Nishimura, Y. Nakashima, S. Fujiwara, N. Kimizuka, T. Ryu, T. Tamura, M. Saigo, K. Onda, S. Sato, Y. Kobori, K. Tateishi, T. Uesaka, G. Watanabe, K. Miyata and N. Yanai, Singlet fission as a polarized spin generator for dynamic nuclear polarization, Nat. Commun., 2023, 14, 1056 CrossRef CAS
.
- J. Krämer, R. Kang, L. M. Grimm, L. De Cola, P. Picchetti and F. Biedermann, Molecular Probes, Chemosensors, and Nanosensors for Optical Detection of Biorelevant Molecules and Ions in Aqueous Media and Biofluids, Chem. Rev., 2022, 122, 3459–3636 CrossRef
.
- S. Dutta Choudhury and H. Pal, Supramolecular and suprabiomolecular photochemistry: a perspective overview, Phys. Chem. Chem. Phys., 2020, 22, 23433–23463 RSC
.
- S. Bhattacharya and S. K. Samanta, Unusual salt-induced color modulation through aggregation-induced emission switching of a bis-cationic phenylenedivinylene-based π hydrogelator, Chem.–Eur. J., 2012, 18, 16632–16641 CrossRef CAS
.
- H. J. Schneider, R. Kramer, S. Simova and U. Schneider, Host-guest chemistry. 14. Solvent and salt effects on binding constants of organic substrates in macrocyclic host compounds. A general equation measuring hydrophobic binding contributions, J. Am. Chem. Soc., 1988, 110, 6442–6448 CrossRef CAS
.
- R. Holm, C. Schönbeck, P. Somprasirt, P. Westh and H. Mu, A study of salt effects on the complexation between β-cyclodextrins and bile salts based on the Hofmeister series, J. Inclusion Phenom. Macrocyclic Chem., 2014, 80, 243–251 CrossRef CAS
.
- H.-J. Schneider, F. Hacket, V. Rudiger and H. Ikeda, NMR studies of cyclodextrins and cyclodextrin complexes, Chem. Rev., 1998, 98, 1755–1785 CrossRef CAS
.
- A. Bernini, O. Spiga, A. Ciutti, M. Scarselli, G. Bottoni, P. Mascagni and N. Niccolai, NMR studies of the inclusion complex between β-cyclodextrin and paroxetine, Eur. J. Pharm. Sci., 2004, 22, 445–450 CrossRef CAS
.
- W. Bizzaro, L. Yarmus, J. Rosenthal and N. Berk, EPR linewidth of triplet excitons in molecular crystals. II. Tetracene, Phys. Rev. B, 1981, 23, 5673 CrossRef CAS
.
- P. Jaegermann, M. Plato, B. von Maltzan and K. Möbius, Time-resolved EPR study of exciton hopping in porphyrin dimers in their photoexcited triplet state, Mol. Phys., 1993, 78, 1057–1074 CrossRef CAS
.
- S. Stoll and A. Schweiger, EasySpin, a comprehensive software package for spectral simulation and analysis in EPR, J. Magn. Reson., 2006, 178, 42–55 CrossRef CAS
.
- M. Schröder, D. Rauber, C. Matt and C. W. Kay, Pentacene in 1,3,5-Tri(1-naphtyl)benzene: A Novel Standard for Transient EPR Spectroscopy at Room Temperature, Appl. Magn. Reson., 2022, 53, 1043–1052 CrossRef
.
- T.-C. Yang, D. J. Sloop, S. Weissman and T.-S. Lin, Zero-field magnetic resonance of the photo-excited triplet state of pentacene at room temperature, J. Chem. Phys., 2000, 113, 11194–11201 CrossRef CAS
.
- L. Latanowicz, NMR relaxation study of methyl groups in solids from low to high temperatures, Concepts Magn. Reson., Part A, 2005, 27A, 38–53 CrossRef CAS
.
- G. Metz, X. L. Wu and S. O. Smith, Ramped-Amplitude Cross Polarization in Magic-Angle-Spinning NMR, J. Magn. Reson., Ser. A, 1994, 110, 219–227 CrossRef CAS
.
- A. Pines, M. G. Gibby and J. S. Waugh, Proton-enhanced NMR of dilute spins in solids, J. Chem. Phys., 1973, 59, 569–590 CrossRef CAS
.
- A. Bornet, R. Melzi, S. Jannin and G. Bodenhausen, Cross Polarization for Dissolution Dynamic Nuclear Polarization Experiments at Readily Accessible Temperatures 1.2 < T < 4.2 K, Appl. Magn. Reson., 2012, 43, 107–117 CrossRef CAS
.
- A. Bornet, R. Melzi, A. J. Perez Linde, P. Hautle, B. van den Brandt, S. Jannin and G. Bodenhausen, Boosting Dissolution Dynamic Nuclear Polarization by Cross Polarization, J. Phys. Chem. Lett., 2013, 4, 111–114 CrossRef CAS
.
- K. Sakamoto, T. Hamachi, K. Miyokawa, K. Tateishi, T. Uesaka, Y. Kurashige and N. Yanai, Polarizing agents beyond pentacene for efficient triplet dynamic nuclear polarization in glass matrices, Proc. Natl. Acad. Sci. U. S. A., 2023, 120, e2307926120 CrossRef
.
- Y. Quan, J. Steiner, Y. Ouyang, K. O. Tan, W. T. Wenckebach, P. Hautle and R. G. Griffin, Integrated, Stretched, and Adiabatic Solid Effects, J. Phys. Chem. Lett., 2022, 13, 5751–5757 CrossRef CAS
.
- Y. Quan, M. V. H. Subramanya, Y. Ouyang, M. Mardini, T. Dubroca, S. Hill and R. G. Griffin, Coherent Dynamic Nuclear Polarization using Chirped Pulses, J. Phys. Chem. Lett., 2023, 14, 4748–4753 CrossRef CAS
.
|
This journal is © The Royal Society of Chemistry 2023 |
Click here to see how this site uses Cookies. View our privacy policy here.