DOI:
10.1039/D3FO03598K
(Paper)
Food Funct., 2024,
15, 96-109
Targeting DSS-induced ulcerative colitis: evaluating the therapeutic potential of WPI–stachyose conjugates†
Received
28th August 2023
, Accepted 10th November 2023
First published on 17th November 2023
Abstract
The pursuit of food-based alternatives to conventional therapies for ulcerative colitis (UC) demands immediate attention. In prior investigations, we synthesized WPI–stachyose conjugates through the Maillard reaction, identifying them as functional prebiotics. However, their impact on in vivo regulation of gut microbiota remains inadequately explored. To bridge this gap, we delved into the therapeutic effects and mechanisms of WPI–stachyose conjugates as prebiotic-functional components in C57BL/6J mice afflicted with dextran sodium sulfate (DSS)-induced UC. The treatment involving WPI–stachyose conjugates led to significant therapeutic advancements, evident in the reduction of pro-inflammatory cytokine levels and restoration of gut microbiota composition. Noticeable enhancements were observed in UC-associated symptoms, including weight loss, colon length reduction, and tissue damage, notably improving in the treated mice. Remarkably, both the conjugates and the physical combination effectively lowered pro-inflammatory cytokines and oxidative stress, with the conjugates demonstrating enhanced effectiveness. Furthermore, the simultaneous administration of WPI–stachyose conjugates further amplified the presence of beneficial bacteria and elevated short-chain fatty acids, acknowledged for their favorable impact across various conditions. These findings underscore the potential therapeutic application of WPI–stachyose conjugates in addressing DSS-induced UC, offering insights into innovative therapeutic strategies.
1. Introduction
Inflammatory bowel disease (IBD) comprises Crohn's disease (CD) and ulcerative colitis (UC), complex and chronic gastrointestinal disorders characterized by mucosal inflammation, cellular damage, and inflammatory processes. UC predominantly affects the distal colon and rectum, leading to symptoms like rectal bleeding, diarrhea, and abdominal pain, while CD can affect any part of the gastrointestinal tract, resulting in diverse symptoms, including abdominal pain, diarrhea, and occult blood in the stool.1 These pathological features contribute to the manifestations of IBD. UC, influenced by environmental, genetic, microbial, and immunological factors,2 primarily exhibits widespread mucosal inflammation in the distal colon and rectum, giving rise to symptoms such as rectal bleeding, diarrhea, abdominal pain, and weight loss. Additionally, UC patients, particularly those with genetic susceptibility, face an increased risk of cancer.3 In recent years, there has been a notable rise in UC incidence. Conventional treatments, such as sulfasalazine, aminosalicylic acid, corticosteroids, and other anti-inflammatory medications, have limited efficacy and are associated with significant side effects, restricting their utility.4 Thus, a compelling imperative emerges for innovative therapies, offering enhanced effectiveness and reduced adverse effects.
Emerging research underscores the therapeutic potential of bioactive compounds derived from food, such as proteins, polyphenols, and polysaccharides, in alleviating UC symptoms.5,6 For instance, a 17-amino acid shrimp peptide has demonstrated efficacy in mitigating acute colitis induced by dextran sodium sulfate (DSS). This effect is attributed to its suppression of pro-inflammatory cytokine production and modulation of the gut microbiome composition.7 The gut, housing a substantial bacterial population, plays a crucial role in maintaining gastrointestinal health.8 Disruptions in microbiota balance, observed in both UC patients and animal models, involve shifts in bacterial composition, including Firmicutes and Proteobacteria, which might contribute to the condition.9,10 These alterations can impact the levels of microbiota-derived metabolites, such as short chain fatty acids (SCFAs), bile acids (BAs), tryptophan (TRP), sphingolipids, and vitamins, all of which play dual roles in the development and protection against IBD.11 Notably, metabolites like acetate and butyrate play a role in regulating intestinal barrier integrity, enhancing tight junction protein expression, and stimulating antimicrobial peptide and mucin secretion. Evidently, reshaping gut microbiota composition has the potential to modulate inflammation signaling pathways, including NF-KB and JAK-STAT, thus reducing pro-inflammatory factor production and ameliorating gut inflammation.12 This emphasizes the emerging concept of dietary manipulation of gut microbiota as a potential intervention for colitis-associated gut inflammation. Understanding the influence of specific food-derived substances on gut microbiota provides valuable insights into targeted dietary strategies in UC management. However, the precise targeting of inflamed IBD sites presents challenges due to the limited solubility and bioavailability of food-derived compounds. Challenges such as stability, oxidation, hydrolysis, and isomerization hinder the aggregation of functional ingredients, which in turn affects therapeutic outcomes. Therefore, optimizing the stability, targeting, and delivery efficiency of these active ingredients stands as a critical pursuit in this context.
Glycation, involving the Maillard reaction between proteins and reducing saccharides, emerges as a promising approach to enhance protein functionalities and confer novel properties.13 This process extends the residence time of proteins in the intestines, facilitating their utilization by the gut microbiota in the colon.14 Controlled Maillard reactions lead to glycoconjugates, which exhibit distinct properties compared to free carbohydrates or native proteins.15 These glycoconjugates have shown the capability to hinder Escherichia coli adhesion to mucin.16 The combination of improved digestibility and prebiotic activity holds significant potential in influencing gut pathogenesis. In our previous study, whey protein isolate (WPI)–stachyose conjugates were successfully synthesized through the Maillard reaction, demonstrating sustained release properties and potential as novel prebiotics, which are retained during gastrointestinal digestion.17 However, a more comprehensive evaluation is required to ascertain the viability of WPI–stachyose conjugates as functional ingredients for preventing or ameliorating symptoms of colitis-induced gut inflammation.
The DSS-induced mouse model is widely recognized as a robust and optimal framework for investigating UC, as it faithfully reproduces key hallmarks, including disruption of the intestinal barrier, an inflammatory response, and dysbiosis within the colonic gut microbiota.18 In this study, our primary aim was to explore the potential of glycation to enhance the prebiotic efficacy of whey protein and stachyose, with the goal of ameliorating colitis and modulating the gut microbiome by improving prebiotic bioavailability. To achieve this, we induced colitis in mice using DSS administration and subsequently assessed the therapeutic impacts of conjugates between whey protein isolate and stachyose, focusing particularly on gauging shifts in the gut microbiota and anti-inflammatory effects. The outcomes of this investigation yield valuable insights into the development of glycated products as complementary therapies for managing intestinal health, thereby contributing to advancements in the realm of UC treatment.
2. Materials and methods
2.1. Materials
Commercial whey protein isolate (WPI) containing 90% protein on a dry basis was procured from Davisco Foods International (Minnesota, USA). Stachyose, a biochemical reagent with an 85% purity level, was purchased from Coolaber Technology Co., Ltd (Beijing, China). Digestive enzymes, including α-amylase (4000 U g−1) and dextran sodium sulfate (DSS) with a molecular weight range of 36–50 kDa, were sourced from Sigma-Aldrich Chemical Co. (Shanghai, China). Pepsin (≥500 U mg−1) from porcine gastric mucosa, bile extract porcine (B8631), and pancreatin (250 U mg−1) from porcine pancreas were also purchased from Sigma-Aldrich Chemical Co. (Shanghai, China). Enzyme-linked immunosorbent assay (ELISA)-based cytokine kits were supplied by Shanghai Enzyme-linked Biotechnology Co., Ltd (Shanghai, China). Acetic acid, propionic acid, n-butyric acid, i-butyric acid, n-valeric acid, and i-valeric acid were acquired from Macklin Biochemical Technology Co., Ltd (Shanghai, China). Diethyl ether and 2-ethylbutyric acid were obtained from Aladdin Biochemical Technology Co., Ltd (Shanghai, China). All reagents and solvents used were of analytical grade. The BCA protein assay kit was purchased from Beyotime Biotechnology Co., Ltd (Shanghai, China).
2.2. Preparation of WPI–stachyose conjugates
The preparation of WPI–stachyose conjugates was conducted following the procedures outlined in our previous publication.17
2.3. Simulated in vitro gastrointestinal digestion
To simulate in vitro gastrointestinal digestion, WPI was subjected to the InfoGest three-phase static method and stachyose was digested using the rat small intestine extract (RSIE) method, following the protocols established in our earlier report.17
2.4.
In vitro bioaccessibility assessment
Following completion of the in vitro digestion process using both the InfoGest and RSIE models, the bioaccessibility of WPI and stachyose was evaluated, adopting the methodology proposed by Tamargo et al.19 After digestion, the digestates from both the InfoGest and RSIE models were transferred to separate dialysis bags (pore size: 12 kDa and 300 Da, Shanghai Yuanye Bio-Technology Co., Ltd, China), which were then immersed in pre-warmed Milli-Q water at 37 °C for 150 min. Dialysis was conducted to separate specific components from the digestion mixture. A 12 kDa membrane was employed to isolate protein digestion products, while a 300 Da membrane was selected to separate the digestion products of stachyose. This step allowed for a focus on the particular components of interest, ensuring the precision of the analysis. The concentrations of WPI and stachyose in the dialysate were determined using the BCA protein assay kit and anthrone colorimetry, respectively. Bioaccessibility was calculated using the equation: |  | (1) |
where C1 represents the concentration of WPI or stachyose in the dialysis bag after dialysis, C2 is the concentration of WPI or stachyose in the dialysis bag of the blank group, V1 is the volume of fluid in the dialysis membrane after dialysis, M stands for the mass of WPI or stachyose in the simulated digestive system, V2 indicates the total volume of the intestinal digestion stage during the simulated digestion process, and V3 is the volume of fluid in the dialysis membrane before the start of dialysis.
2.5. Animal study
In this study, a cohort of 36 male C57BL/6J mice, each aged 8 weeks and weighing approximately 20 ± 2 g, was procured from Liaoning Changsheng Biotechnology Co., Ltd. These mice were methodically accommodated in groups of nine within rectangular cages, maintaining a controlled environment with a consistent temperature of 25 ± 2 °C and humidity levels of 50% ± 5%. They were consistently provided with a standard diet (AIN-93) and exposed to a 12-hour light/dark cycle to ensure uniform conditions throughout the experiment.
Prior to commencing the main experiment, the mice underwent a two-week period during which they were fed a normal diet to acclimate to their environment. Simultaneously, they were regularly rotated among different cages to promote the homogenization of fecal microbiomes. This strategic rotation of mice among cages aimed to minimize potential variations in microbiota composition associated with specific cage-related microbial influences. By equalizing the microbial exposure of all mice during the acclimation phase, the study established a more uniform baseline for subsequent experiments.
Following this thorough acclimation process, the mice were evenly distributed into four distinct experimental groups, with each group comprising an equal number of mice. These groups consisted of a normal control group, a DSS-induced control group, a group receiving PM treatment, and another group treated with WPI–stachyose conjugates. The meticulous implementation of this well-balanced distribution among the various experimental conditions was undertaken to ensure the reliability and statistical significance of the findings. This approach guarantees that the results accurately reflect the impact of the interventions on DSS-induced colitis, adhering to established standards in scientific research within this field.
Ethical considerations encompassed the humane treatment of animals, provision of appropriate housing conditions, and strict adherence to guidelines aimed at minimizing distress and suffering. A 7-day acclimatization period preceded the experiments.
2.6. DSS-induced IBD mouse model
Thirty-six mice were randomly divided into four distinct groups: the normal control group (referred to as NC), the DSS-induced colitis group (referred to as DSS), the group treated with the WPI and stachyose physical mixture after DSS induction (referred to as PM–DSS), and the group treated with WPI–stachyose conjugates after DSS induction (referred to as W–S–DSS). Only the NC group had access to distilled drinking water over an 8-day period, while the other groups were exposed to 4% DSS in their drinking water to induce colitis.
To establish the optimal oral dosages for the WPI–stachyose conjugates, a series of initial experiments were conducted, as outlined in the ESI (refer to Fig. S1†). These experiments encompassed administering varying dosages of the conjugates to DSS-induced mice, with a focus on assessing physiological responses and potential impacts. Three dosages were selected: 250 mg kg−1, 500 mg kg−1, and 1000 mg kg−1, covering a range to observe potential dose-dependent effects and understand compound effects comprehensively. Analysis of the preliminary results included evaluating mice's tolerance and conjugate efficacy. The 500 mg kg−1 dosage emerged as the most balanced and effective. It demonstrated a favorable combination of physiological responses and outcomes, suggesting potential without adverse reactions. Due to its strong correlation with observed effects, it became the optimal oral dosage for the main experimental phase, offering a balance between potency and minimizing side effect risks. In summary, determining the suitable oral dosage for the experimental mice involved meticulous preliminary experiments, thorough observation, and data analysis. The 500 mg kg−1 dosage, chosen for its positive physiological responses and outcomes, will be used in the primary study to delve into WPI–stachyose conjugate effects and mechanisms.
Conversely, the NC and DSS groups were administered distilled water daily through gavage for 8 days. Upon reaching Day 9, all mice were humanely euthanized, and tissue samples were collected for subsequent analysis.
2.7. Disease activity index assessment
Throughout the experiment, daily observations included body weight, diarrhea, and bloody feces, contributing to the colitis Disease Activity Index (DAI), which ranged from 0 to 4 (details in the ESI, Table S1†).
2.8. Analysis of the immune organ index
The thymus, spleen, liver, and kidneys were surgically excised and promptly weighed. Subsequently, the immune organ index was calculated using the following formula: immune organ index = weight of immune organs (mg)/body weight (g).
2.9. Histological analysis
Colon tissue was fixed, paraffin-embedded, and sectioned. Hematoxylin and eosin (H&E) staining was performed to evaluate inflammation-associated scores and bowel wall thickness.20 The inflammation-associated scores were determined by assessing tissue damage and the presence of inflammatory cells in the lamina propria (see the ESI, Table S2†). The bowel wall thickness was measured using ImageJ software (Media Cybernetics, MD, USA). The goblet cell count and mucus layer thickness were assessed using Alcian blue periodic acid Schiff (AB/PAS) staining with a kit (ZSGB-BIO, Beijing, China), following the manufacturer's instructions.21 Light microscopy was used for histopathological examination, conducted by Shanghai Zhuye Biotechnology Co., Ltd (Shanghai, China). The mucus layer thickness and goblet cell count in the colonic epithelial cells were determined using AB/PAS staining at a magnification of 200× and analyzed using ImageJ software.
2.10. Enzyme-linked immunosorbent assay (ELISA) for measurements
The colonic tissue supernatant was obtained through homogenization in phosphate buffer solution (PBS). Enzyme-linked immunosorbent assay (ELISA) kits were employed to quantify IL-1β, IL-6, TNF-α, and IL-10 concentrations in the supernatant, following standard protocols.
2.11. Analysis of gut microbiota in mouse fecal samples
The investigation of gut microbiota in mouse fecal samples was executed using the 16S rDNA amplicon sequencing methodology, as described by Cai et al.22 This comprehensive analysis comprised a series of sequential steps: DNA extraction, PCR amplification, purification of the amplified products, library construction, and computational sequencing using the NovaSeq platform. The utilization of Novogene Biotech Co., Ltd's (Beijing, China) cloud-based network platform facilitated the evaluation of various aspects of gut microbiota composition, including relative abundance, complexity, diversity, and inter-group variations.
2.12. Detection of short-chain fatty acids (SCFAs) in cecal contents
Short-chain fatty acids (SCFAs), including acetic acid, propionic acid, n-butyric acid, i-butyric acid, n-pentanoic acid and i-pentanoic acid, in cecal contents were quantified using an Agilent 7890B gas chromatography instrument. The process, as described by Sang et al.23 and Xu et al.,24 involved the collection of approximately 100 mg of cecal contents in 2 mL centrifuge tubes. Sample preparation included the addition of glass slag, 20 μL of 50% (v/v) dilute sulfuric acid, 995 μL of diethyl ether, and 5 μL of 2-ethylbutyric acid diluent (v/v = 1/100). After vortexing for 6 minutes and 30 minutes of sonication in an ice water bath to ensure complete SCFA release, centrifugation at 12
000g and 4 °C for 15 minutes produced supernatants that were transferred to 1.5 mL centrifuge tubes. Further processing involved the addition of 250 mg of anhydrous sodium sulfate to remove excess water, followed by storage at −80 °C for subsequent analysis post-centrifugation and filtration through a 0.22 μm filter membrane. Gas chromatography operating conditions comprised an initial temperature of 80 °C, programmed increments to 120 °C at 40 °C min−1, further increase to 200 °C at 10 °C min−1, and a 2-minute maintenance at 230 °C post-operation.
2.13. Statistical analysis
The data were presented as mean ± standard deviation (SD) from triplicate experiments. Statistical analysis was performed using the Statistical Package for Social Science program (SPSS 24, Chicago, USA). One-way ANOVA assessed statistical significance, and inter-group differences were determined through Tukey's test, with a significance threshold of p < 0.05. Moreover, Spearman's correlation analysis was employed to explore relationships between the relative abundance of microbiota and illness markers.
3. Results and discussion
3.1. Enhanced bioaccessibility of WPI and stachyose through glycation treatment
Bioaccessibility refers to the portion of an ingested nutrient that can be absorbed in the gut following digestion. It signifies the amount of a compound that is released from the food matrix, becoming available for interaction with the gut lining and potential absorption. In our study, it determines how much of the WPI–stachyose conjugates is accessible for interaction with the gut microbiota and the intestinal lining, influencing their effects on intestinal health.25 The bioaccessibility of WPI and stachyose was evaluated using glucose diffusion across dialysis membranes (Fig. 1). Previous research had indicated that glycation effectively reduces the loss of WPI and stachyose during gastrointestinal digestion.17 Over time, concentrations of WPI and stachyose in the dialysate consistently increased in all experimental groups. Importantly, glycation significantly improved the bioaccessibility of WPI–stachyose conjugates compared to the PM group. This enhancement suggests the potential of these conjugates as functional ingredients that can enhance intestinal health by promoting the growth of beneficial probiotics during colonic fermentation.26 These results emphasize the promise of glycation treatment in enhancing the bioavailability of these essential compounds.
 |
| Fig. 1 Bioaccessibility of native whey protein isolate (WPI), stachyose, WPI–stachyose physical mixture (PM), and WPI–stachyose conjugates. Different letters indicate statistically significant differences in content based on ANOVA (p < 0.05). | |
3.2. Disease symptoms of DSS-induced colitis in mice
In an effort to evaluate the in vivo effectiveness of WPI–stachyose conjugates against DSS-induced inflammatory bowel disease (IBD), the study divided mice into four groups: a normal control group, a DSS-induced control group, a group treated with PM, and a group treated with WPI–stachyose conjugates, as depicted in Fig. 2A. DAI scores, considering parameters such as bodyweight loss, fecal characteristics, and fecal bleeding, were significantly higher in the DSS-induced group compared to the normal control group (p < 0.05), indicating the severity of colitis induced by DSS. Remarkably, mice treated with WPI–stachyose conjugates exhibited lower DAI scores compared to both the DSS-induced and PM-treated groups (Fig. 2B). Colitis severity, as indicated by bodyweight loss, began to decrease in DSS-induced mice from day five, while mice treated with PM and WPI–stachyose conjugates displayed relatively lower weight loss (Fig. 2C). After a span of eighteen days, the DSS-induced group exhibited a weight of 18.15 ± 0.75 g. In contrast, the groups treated with PM and WPI–stachyose conjugates recorded higher weights of 19.30 ± 0.67 g and 20.36 ± 0.72 g, respectively, indicating a significant improvement in bodyweight associated with the WPI–stachyose conjugate treatment. Furthermore, the evaluation of colon length unveiled a significant mitigation of colonic shortening in the group treated with WPI–stachyose conjugates (p < 0.05), while no noteworthy distinction in colon length was observed between the DSS-induced group and the PM-treated groups (Fig. 2D and E). This observation highlights the potential of WPI–stachyose conjugates to alleviate the structural changes induced by DSS in the colon.
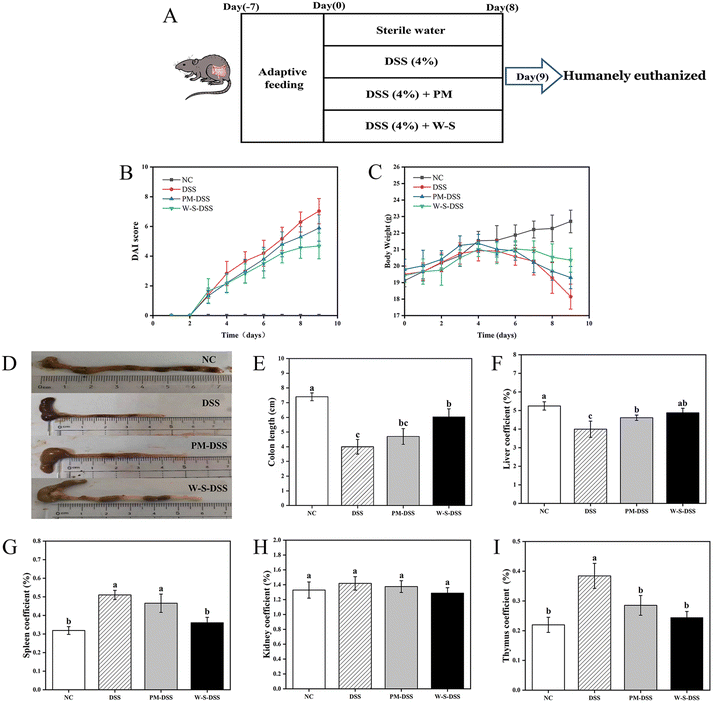 |
| Fig. 2 (A) Experimental design schematic. (B) Disease activity index (DAI) score. (C) Body weight changes. (D) Representative gut tissue images. (E) Colon length (cm) in different groups of mice. (F) Liver, (G) spleen, (H) kidney, and (I) thymus index in colitis mice. Values are mean ± SD (n = 9). Different letters within each column denote significant differences between groups (p < 0.05). | |
Distinctive hepatosplenomegaly, a hallmark of DSS-induced colitis in mice, was also evident.27 Spleen and thymus indices in DSS-induced mice markedly exceeded those in normal controls (Fig. 2G and I). Nevertheless, following treatment with PM or WPI–stachyose conjugates, these indices demonstrated a significant reduction (p < 0.05). Notably, the therapeutic impact of WPI–stachyose treatment surpassed that of PM (p < 0.05), nearly approaching control levels. Kidney indices displayed no notable differences (p > 0.05) among the various groups.
These findings collectively indicate that WPI–stachyose conjugates offer a promising approach to mitigate the severity of DSS-induced colitis. Notable improvements in disease symptoms, including DAI scores, bodyweight loss, colon length, and hepatosplenomegaly, underscore the potential therapeutic efficacy of WPI–stachyose conjugates in the context of inflammatory bowel disease.
3.3. Histological changes in colon tissues of DSS-induced colitic mice
Upon histopathological examination of colonic tissues, distinct alterations were observed in colitis-induced mice.28 The control group displayed healthy colon tissues characterized by intact colonic mucosa, well-formed and aligned crypts, and abundant goblet cells forming the mucosal barrier (Fig. 3A). Inflammatory cell infiltration was minimal in the submucosa, with a few inflammatory cells observed in the mucosal muscularis. Conversely, DSS-induced colitis mice exhibited thinning of the mucosa, severe crypt damage, goblet cell depletion, and submucosal infiltration of inflammatory cells, as indicated by H&E staining (Fig. 3). Remarkably, both PM and WPI–stachyose conjugate treatments effectively attenuated DSS-induced morphological changes, with WPI–stachyose conjugates showing superior improvements (Fig. 3). The assessment of colon wall thickness, conducted using established criteria (Table S2†) on H&E-stained sections by a blinded observer,29 revealed that DSS administration led to increased colon wall thickness, neutrophil infiltration, and tissue damage, consistent with previous studies (Fig. 3B and C).30 The DSS group exhibited significantly greater colon wall thickness and tissue damage compared to the normal control group, likely due to tissue edema (p < 0.05). In contrast, the groups treated with WPI–stachyose conjugates demonstrated remarkably lower damage and colon wall thickness than both the PM group and the DSS group (p < 0.05), underscoring the protective effect of WPI–stachyose conjugates.
 |
| Fig. 3 (A) H&E staining images (40× magnification). Scale bars: 500 μm. (B) Histopathology scores and (C) colon muscular layer thickness. Values are mean ± SD (n = 9). Different letters within each column signify significant differences between groups (p < 0.05). | |
To quantify goblet cells and assess mucus thickness, AB/PAS staining was conducted on histological sections following the protocol established by Gao et al.31 Illustrative AB/PAS-stained cross-sections of the colon (Fig. 4A) vividly exhibited heightened goblet cell counts in the groups treated with PM and WPI–stachyose conjugates in comparison with those in the DSS group (p < 0.05, Fig. 4B). Furthermore, the evaluation of mucus thickness in AB/PAS-stained colon sections (Fig. 4C) was aimed to explore the impacts of WPI–stachyose conjugates on the intestinal mucosal layer. It is noteworthy that no substantial disparities were noted between the normal mice and PM groups. However, the administration of WPI–stachyose conjugates significantly ameliorated mucus thickness in colitis mice (p < 0.05, Fig. 4C).
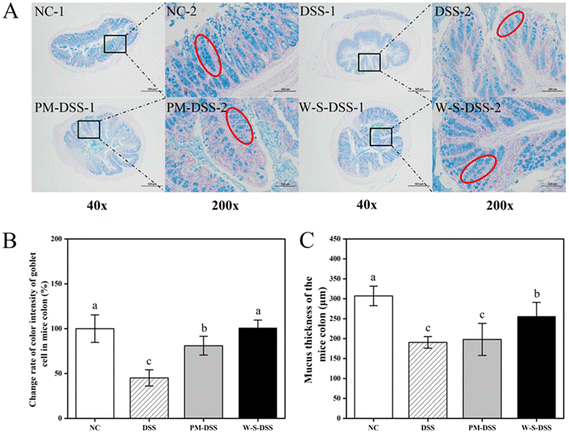 |
| Fig. 4 (A) AB/PAS staining images (40× and 200× magnification). Scale bars: 500 μm and 100 μm. (B) Goblet cell color intensity change rate in mice colon. (C) Mucus thickness of mice colon. Values are mean ± SD (n = 6). Different letters within each column represent significant differences between groups (p < 0.05). | |
The results, revealed through AB and PAS staining, suggest that treatment with WPI–stachyose conjugates enhances the maturation of mucin-producing cells. This observation is consistent with previous findings by Guo et al.32 and Xia et al.,2 further corroborating this inference. Moreover, the preservation of colonic architecture, characterized by increased mucosa thickness, well-defined crypt structures, higher goblet cell numbers, and cleaner submucosa, further underscores the favorable effects of both PM and, notably, WPI–stachyose conjugates in alleviating colitis symptoms.
Together, these histological changes, including increased mucosa thickness, well-defined crypt structures, higher goblet cell numbers, and cleaner submucosa, highlight the substantial benefits of PM and, particularly, WPI–stachyose conjugates in alleviating colitis symptoms. These findings support the role of these conjugates in preserving colon architecture and mucosal integrity.
3.4. Cytokine levels in the colonic tissue of DSS-induced colitic mice
The impact of WPI–stachyose conjugates on IBD was evaluated by assessing cytokine levels in the colonic tissue of mice with DSS-induced colitis. This analysis included both inflammatory cytokines (TNF-α, IL-β, and IL-6) and an anti-inflammatory cytokine (IL-10) as representative markers. Upon inducing colitis with DSS, the group exposed to the colitis model exhibited notably elevated levels of TNF-α, IL-1β, and IL-6 compared to the normal control group. In contrast, the treatment involving the administration of WPI–stachyose conjugates led to a significant reduction in the levels of TNF-α, IL-1β, and IL-6 compared to that involving the DSS group (p < 0.05, Fig. 5). It is worth noting that there were no significant variations in IL-6 levels between the group receiving the PM treatment and the mice with DSS-induced colitis. However, the IL-6 levels observed in the PM group were significantly higher than those in the WPI–stachyose conjugate group (p < 0.05).
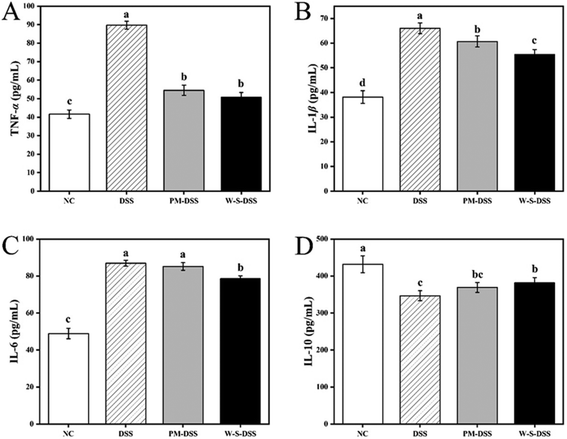 |
| Fig. 5 Inflammatory cytokine levels: (A) tumor necrosis factor (TNF)-α, (B) interleukin (IL)-1β, (C) IL-6, and (D) IL-10 in different treatment groups. Different letters within each column represent significant differences between groups (p < 0.05). | |
Shifting to the realm of anti-inflammatory cytokines, there was a noticeable increase in the secretion of IL-10 in the colonic tissue. Specifically, the administration of WPI–stachyose conjugates had a significantly enhancing effect on IL-10 secretion, while the supplementation with PM showed no significant impact. These results indicate that WPI–stachyose conjugates have a substantial impact on the cytokine profile in colonic tissue, with a notable reduction in pro-inflammatory cytokines and an increase in the anti-inflammatory cytokine IL-10. These findings emphasize the potential of these conjugates as a nutritional intervention for managing inflammatory bowel disease in a mouse model.
3.5. Impact of WPI–stachyose conjugates on gut microbiota
The composition of the gut microbiota plays a pivotal role in the initiation and progression of IBD.28 To gauge the impact of WPI–stachyose conjugates in a murine model of DSS-induced colitis, fecal microbiota analysis was conducted using 16S rDNA amplicon sequencing. The sequencing demonstrated adequate sample sizes, species richness, and consistency across treatment groups, as evident from the rarefaction curve (Fig. 6A). The species accumulation boxplot, evaluating the sample number and richness, consistently demonstrated increased species count with larger samples (Fig. 6B). As the sample size reached saturation, the curve stabilized, indicating precise representation of the gut microbiota.
 |
| Fig. 6 (A) Dilution curve and (B) species accumulation box. (C) Phylogenetic tree of microbiota genus-level sequences. PM: whey protein isolate-stachyose physical mixture; W-S: whey protein isolates glycated with stachyose. Different letters above the columns signify significant differences (p < 0.05). | |
α-Diversity analysis, assessed through Chao1, Shannon, and Simpson indices, revealed reduced microbial diversity in the DSS-induced group compared to that in the normal control group. While PM intervention showed slight improvement, it lacked statistical significance. In contrast, supplementation with WPI–stachyose conjugates significantly countered the DSS-induced reduction in the richness (Chao1 index) and diversity (Simpson index) of gut microbiota (Fig. 5A and B). These findings underscore the favorable effects of WPI–stachyose conjugates in mitigating DSS-induced gut microbiota disturbances.
Analyzing gut microbiota composition and abundance unveiled Firmicutes and Bacteroidetes as the major phyla in colitis mice feces (Fig. 7A).33 The Firmicutes-to-Bacteroidetes ratio significantly dropped in the DSS-induced group compared to that in normal control mice. Yet, WPI–stachyose conjugates effectively restored this ratio (Fig. 7B), aligning with previous studies on whey protein and stachyose extract in DSS-induced colitis mice.34,35 Notably, WPI–stachyose conjugates reduced the abundance of detrimental bacteria (Proteobacteria and Actinobacteria), while increasing beneficial bacteria (Lachnospiraceae_NK4A136_group, Muribaculum, Muribaculaceae, Lactobacillus, and Prevotellaceae_UCG_001) in the gut microbiota (Fig. 7E and F). Importantly, Lachnospiraceae_NK4A136_group and Prevotellaceae_UCG_001 are known to produce short-chain fatty acids (SCFAs), contributing to a healthier gut environment.
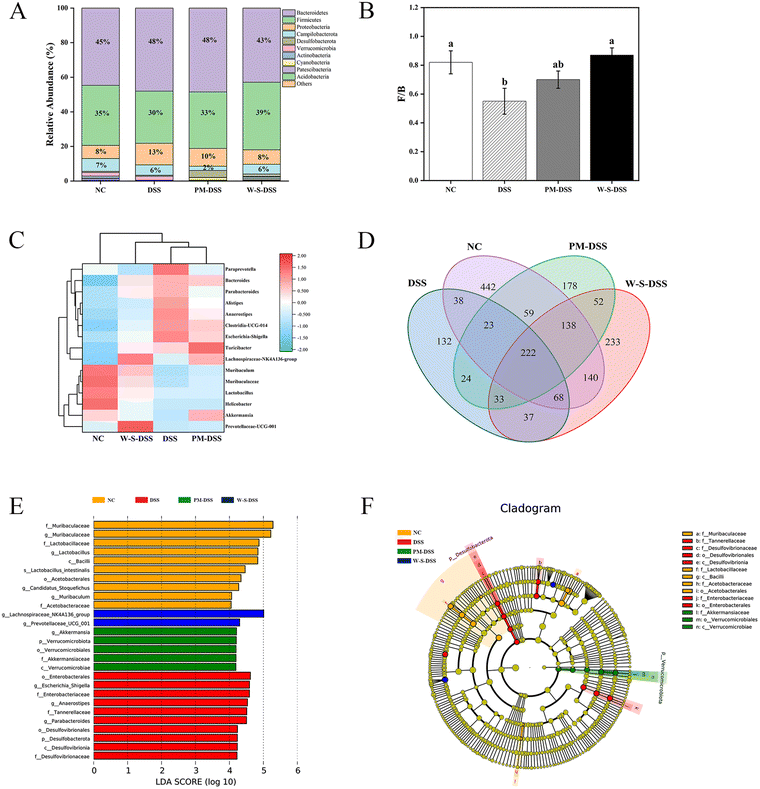 |
| Fig. 7 (A–D) Venn diagrams illustrating shared and unique intestinal flora species in mice across different groups based on the OTU taxonomic level. (E and F) Differential abundance of dominant microorganisms among three groups shown as a cladogram and distribution histogram based on LDA. Values are mean ± SD (n = 3). Different letters within each column denote significant differences between groups (p < 0.05). | |
Analyzing species abundance and composition at phylum and genus levels revealed that the WPI–stachyose conjugate-treated group and normal controls formed distinct clusters, separate from that of DSS-induced mice. This suggests a closer resemblance between WPI–stachyose-treated group and normal controls in gut microbiota composition.
The Venn diagram depicted an overlap of 222 operational taxonomic units (OTUs) within the treatment groups, as shown in Fig. 7D. Importantly, distinct species showed considerable variation, suggesting that the presence or absence of specific bacterial types has a more pronounced impact on gut microbiota homeostasis than mere numbers alone.
In the analysis of significant microbial biomarkers across groups, linear discriminant analysis effect size (LEfSe) was a valuable tool.36 The normal group exhibited higher levels of Muribaculaceae, Lactobacillaceae, Lactobacillus, Bacilli, Acetobacterales, Muribaculum, and Acetobacteraceae (Fig. 7E). Conversely, the DSS-induced group showed elevated levels of Enterobacterales, Escherichia-Shigella, and Desulfobacterota, which are known contributors to gut dysbiosis. Interestingly, the WPI–stachyose-treated group demonstrated higher levels of Lachnospiraceae_NK4A136_group and Prevotellaceae_UCG_001, which serve as significant representative biomarkers (Fig. 7E). Previous research has underscored the significance of short-chain fatty acids (SCFAs) as essential metabolites produced by the gut microbiota, with a central role in colitis.37 Notably, during active UC, a decrease in fecal SCFA levels is frequently observed.16 These identified biomarkers suggest the potential contribution of beneficial bacteria in regulating DSS-induced inflammation through the enhanced production of SCFAs.38
Our analysis of gut microbiota composition and abundance reveals the favorable modulation achieved by WPI–stachyose conjugates. They promote the growth of beneficial bacteria while reducing detrimental ones, thus creating a healthier gut environment. These results indicate a promising role of WPI–stachyose conjugates in shaping the gut microbiota composition.
3.6. Quantitative analysis of short-chain fatty acids (SCFAs)
SCFAs are crucial byproducts of intestinal microbial metabolism that play a pivotal role in gastrointestinal health and are essential in the context of IBD.39 In this study, gas chromatography and mass spectroscopy were employed to quantify prominent SCFAs, including acetic acid, propionic acid, n-butyric acid, i-butyric acid, n-pentanoic acid, and i-pentanoic acid. Results revealed a marked decline in total SCFA levels in the DSS-induced control group compared to those in the normal controls (Fig. 8G). However, interventions involving PM and WPI–stachyose conjugates effectively elevated the total SCFA content.
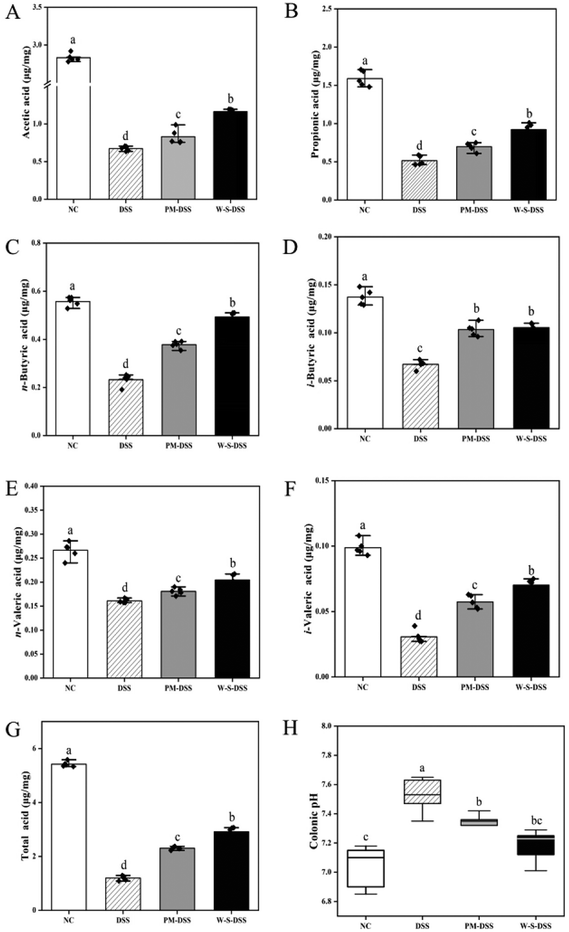 |
| Fig. 8 Concentrations of (A) acetic acid, (B) propionic acid, (C) n-butyric acid, (D) i-butyric acid, (E) n-valeric acid, (F) i-valeric acid, and (G) total acid, and (H) pH of colonic contents. Values are mean ± SD (n = 5). Different letters within each column represent significant differences between groups (p < 0.05). | |
Following DSS induction, a substantial reduction in acetic acid, propionic acid, n-butyric acid, i-butyric acid, n-pentanoic acid, and i-pentanoic acid levels was observed (Fig. 8A). Notably, significant differences emerged in propionic acid, n-butyric acid, i-butyric acid, n-pentanoic acid, and i-pentanoic acid between the PM and WPI–stachyose conjugate treatment groups (Fig. 8A–F). WPI–stachyose conjugates markedly boosted acetic acid, propionic acid, n-butyric acid, n-pentanoic acid, and i-pentanoic acid levels compared to the PM group, demonstrating superior regulation of SCFA levels.
Previous research highlights dynamic interactions among food components during gastrointestinal digestion, influencing compound bioaccessibility and affecting the impact of WPI–stachyose conjugates on colonic microbiota composition and functional ingredient metabolism.19 The study integrated glycation treatment, significantly enhancing WPI and stachyose bioaccessibility, as depicted in Fig. 1. This treatment substantially augmented SCFA production during colonic fermentation. Notably, butyrate, known for its favorable effects on gastrointestinal health, serves as the primary energy source for colonocytes,40 reducing oxidative stress,41 suppressing pro-inflammatory cytokine release,42 and reinforcing gut barrier function.43 Elevated SCFA levels resulting from the WPI–stachyose conjugate intervention effectively mitigated inflammation in colitis mice.
In addition, the DSS group exhibited a noteworthy increase in pH levels compared to the control condition (p < 0.05, Fig. 8G). Conversely, both the PM and WPI–stachyose conjugate groups displayed significant reductions in pH compared to the DSS group (p < 0.05). This observation further supports the notion that the decreased pH environment may foster the growth of beneficial intestinal bacteria.44 WPI–stachyose conjugates led to a more pronounced reduction in pH values compared to the PM group, resulting in intestinal pH levels closely resembling those of the normal control group. Consequently, the effectiveness of regulatory control was significantly superior in the WPI–stachyose conjugate group as opposed to the PM group.
Quantitative analysis of SCFAs reveals that WPI–stachyose conjugates effectively enhance the levels of these essential metabolites, particularly butyrate. These findings highlight the potential of WPI–stachyose conjugates to positively impact gastrointestinal health by promoting SCFA production and regulating intestinal pH, contributing to the mitigation of inflammation in colitis mice.
4. Conclusions
This study underscores the promising therapeutic potential of WPI–stachyose conjugates in the treatment of UC. The uniqueness of these findings lies in their multifaceted approach, setting this study apart from previous research and offering novel insights into potential clinical applications for individuals with UC. Foremost, the study demonstrates the remarkable efficacy of WPI–stachyose conjugates in alleviating UC symptoms and mitigating colonic organ damage. What distinguishes this work is the emphasis on restoring the integrity of the colonic mucous layer, a critical factor in UC pathogenesis. Unlike previous research, which often focused solely on a single aspect of UC management, such as inflammation or microbiota modulation, this study comprehensively addresses multiple aspects of the disease. This holistic approach provides a more complete picture of how WPI–stachyose conjugates can impact UC. These findings highlight the pivotal role of WPI–stachyose conjugates in rebalancing the intestinal microflora, reducing inflammatory cytokines, particularly IL-1β, IL-6, and TNF-α. While previous studies have touched upon the influence of dietary components on inflammation, the unique aspect of this work lies in the ability to attribute these therapeutic outcomes to enhanced bioaccessibility of WPI and stachyose through glycation. This innovative approach allows for greater efficacy and specificity in UC management.
Furthermore, this study elucidates how WPI–stachyose conjugates can effectively modulate the composition and relative abundance of the gut microbiota. By increasing the levels of short-chain fatty acids (SCFAs), particularly butyrate, it provides a comprehensive understanding of how these conjugates contribute to the alleviation of colitis symptoms. This shift in focus from isolated observations to a more integrative perspective makes these findings more applicable to clinical scenarios.
This research opens new avenues for UC treatment by harnessing the potential of WPI–stachyose conjugates. These innovative dietary supplements offer a holistic approach to UC management, addressing the complex and multifaceted nature of the disease. This work provides a promising foundation for future clinical applications, potentially offering individuals with UC a synergistic therapeutic intervention. By encompassing various aspects of the disease, it aims to pave the way for a more effective and well-rounded approach for managing UC.
Live subject statement
The experimental procedures involving animal samples in this study were conducted in strict compliance with the principles outlined in the Guide for the Care and Use of Laboratory Animals (Eighth Edition) and adhered to the relevant ethical regulations of Dalian Polytechnic University. The welfare and ethical treatment of animals were of utmost priority throughout all experiments.
All experimental protocols were subjected to a rigorous review and approval process by the Animal Ethics Committee of Dalian Polytechnic University and received approval under the reference number SYXK2017-0005. This committee is responsible for ensuring that the experiments align with established institutional guidelines and uphold the highest standards of ethical conduct in animal research.
The researchers involved in this study followed all relevant laws, guidelines, and institutional protocols to guarantee the humane and ethical treatment of the animal subjects. In the case of human subjects, no such involvement occurred in this study, and therefore, informed consent was not applicable.
We affirm our commitment to maintaining the highest standards of ethical conduct in research and ensuring the welfare of all living subjects involved in our experiments.
Author contributions
Zi-han Wang: writing – original draft; Guang-yao Zhang: investigation; Cong Sun: methodology; Shu-xin Ning: methodology; Da-yong Zhou: supervision; Liang Song: conceptualization, funding acquisition, project administration, supervision, and writing – review and editing.
Conflicts of interest
There are no conflicts of interest to declare.
Acknowledgements
This work was supported by the National Natural Science Foundation of China (Grant No. 31871795).
References
- Q. Y. Cui, X. Y. Tian, X. Liang, Z. Zhang, R. Wang, Y. Zhou, H. X. Yi, P. M. Gong, K. Lin, T. J. Liu and L. W. Zhang, Bifidobacterium bifidum relieved DSS-induced colitis in mice potentially by activating the aryl hydrocarbon receptor, Food Funct., 2022, 13, 5115–5123, 10.1039/d1fo04219j.
- P. Xia, T. Hou, M. Ma, S. Li, H. Jin, X. Luo, J. Li, F. Geng and B. Li, Konjac oligosaccharides attenuate DSS-induced ulcerative colitis in mice: mechanistic insights, Food Funct., 2022, 13, 5626–5639, 10.1039/d1fo04004a.
- B. Katsandegwaza, W. Horsnell and K. Smith, Inflammatory Bowel Disease: A Review of Pre-Clinical Murine Models of Human Disease, Int. J. Mol. Sci., 2022, 23, 9344, DOI:10.3390/ijms23169344.
- H. Yang, S. Jeong, M. S. Ryu, G. Ha, D. Jeong, Y. M. Park, H. Y. Lee and J. S. Bae, Protective effect of traditional Korean fermented soybean foods (doenjang) on a dextran sulfate sodium-induced colitis mouse model, Food Funct., 2022, 13, 8616, 10.1039/d2fo01347a.
- H. Li, L. M. Christman, R. Li and L. Gu, Synergic, Interactions between Polyphenols and Gut Microbiota in Mitigating Inflammatory Bowel Diseases, Food Funct., 2022, 11, 4878–4891, 10.1039/D0FO00713G.
- A. Machado, M. V. Geraldi, R. P. do Nascimento, A. Moya, T. Vezza, P. Diez-Echave, J. J. Galvez, C. B. B. Cazarin and M. R. Marostica Junior, Polyphenols from food by-products: An alternative or complementary therapy to IBD conventional treatments, Food Res. Int., 2021, 140, 110018, DOI:10.1016/j.foodres.2020.110018.
- X. Xiang, Q. Jiang, W. Shao, J. Li, Y. Zhou, L. Chen, S. Deng, B. Zheng and Y. Chen, Protective Effects of Shrimp Peptide on Dextran Sulfate Sodium-Induced Colitis in Mice, Front. Nutr., 2021, 8, 773064, DOI:10.3389/fnut.2021.773064.
- Z. J. Zhao, S. M. Xu, W. Y. Zhang, D. J. Wu and G. S. Yang, Probiotic Escherichia coli NISSLE 1917 for inflammatory bowel disease applications, Food Funct., 2022, 13, 5914–5924, 10.1039/d2fo00226d.
- Z. G. Li, S. Zhang, L. Xu, X. X. Fang, Y. Z. Wan, D. H. Yu and Y. Guo, A tetrapeptide from maize combined with probiotics exerted strong anti-inflammatory effects and modulated gut microbiota in DSS-induced colitis mice, Food Funct., 2022, 12, 12606–12608, 10.1039/d2fo02678c.
- X. Yang, S. Li, C. Wang, Y. Lou, X. Xia and H. Xu, Whole and polysaccharide powdered Sporisorium reilianum improves DSS-induced colitis in BALB/c mice by modulating gut microbiota, J. Funct. Foods, 2021, 79, 104409, DOI:10.1016/j.jff.2021.104409.
- L. Dong, J. Xie, Y. Wang, H. Jiang, K. Chen, D. Li, J. Wang, Y. Liu, J. He, J. Zhou, L. Zhang, X. Lu, X. Zou, X. Y. Wang, Q. Wang, Z. Chen and D. Zuo, Mannose ameliorates experimental colitis by protecting intestinal barrier integrity, Nat. Commun., 2022, 13, 4804, DOI:10.1038/s41467-022-32505-8.
- B. Zhao, B. Xia, X. Li, L. Zhang, X. Liu, R. Shi, R. Kou, Z. Liu and X. Liu, Sesamol Supplementation Attenuates DSS-Induced Colitis via Mediating Gut Barrier Integrity, Inflammatory Responses, and Reshaping Gut Microbiome, J. Agric. Food Chem., 2020, 68, 10697–10708, DOI:10.1021/acs.jafc.0c04370.
- M. Corzo-Martínez, F. J. Moreno, M. Villamiel and F. M. Harte, Characterization and improvement of rheological properties of sodium caseinate glycated with galactose, lactose and dextran, Food Hydrocolloids, 2010, 24, 88–97, DOI:10.1016/j.foodhyd.2009.08.008.
- C. Delgado-Andrade, S. Pastoriza de la Cueva, M. J. Peinado, J. A. Rufian-Henares, M. P. Navarro and L. A. Rubio, Modifications in bacterial groups and short chain fatty acid production in the gut of healthy adult rats after long-term consumption of dietary Maillard reaction products, Food Res. Int., 2017, 100, 134–142, DOI:10.1016/j.foodres.2017.06.067.
- M. Corzo-Martínez, O. Hernandez-Hernandez, M. Villamiel, R. A. Rastall and F. J. Moreno,
In vitro bifidogenic effect of Maillard-type milk protein–galactose conjugates on the human intestinal microbiota, Int. Dairy J., 2013, 31, 127–131, DOI:10.1016/j.idairyj.2013.01.004.
- J. Moisés Laparra, M. Corzo-Martinez, M. Villamiel, F. Javier Moreno and Y. Sanz, Maillard-type glycoconjugates from dairy proteins inhibit adhesion of Escherichia coli to mucin, Food Chem., 2011, 129, 1435–1443, DOI:10.1016/j.foodchem.2011.05.102.
- G. Y. Zhang, C. Sun, J. R. Song, W. Y. Jin, Y. Tang, D. Y. Zhou and L. Song, Glycation of whey protein isolate and stachyose modulates their in vitro digestibility: Promising prebiotics as functional ingredients, Food Biosci., 2023, 52, 102379, DOI:10.1016/j.fbio.2023.102379.
- X. Li, H. Lv, F. Shi, J. Song and Z. Zhang, The potential therapeutic effects of hydroxypropyl cellulose on acute murine colitis induced by DSS, Carbohydr. Polym., 2022, 289, 119430, DOI:10.1016/j.carbpol.2022.119430.
- A. Tamargo, C. Cueva, M. Silva, N. Molinero, B. Miralles, B. Bartolome and M. V. Moreno-Arribas, Gastrointestinal co-digestion of wine polyphenols with glucose/whey proteins affects their bioaccessibility and impact on colonic microbiota, Food Res. Int., 2022, 155, 111010, DOI:10.1016/j.foodres.2022.111010.
- J. Cai, J. Liu, P. Fan, X. Dong, K. Zhu, X. Liu, N. Zhang and Y. Cao, Dioscin prevents DSS-induced colitis in mice with enhancing intestinal barrier function and reducing colon inflammation, Int. Immunopharmacol., 2021, 99, 108015, DOI:10.1016/j.intimp.2021.108015.
- W. Guo, M. Zeng, S. Zhu, S. Li, Y. Qian and H. Wu, Phycocyanin ameliorates mouse colitis via phycocyanobilin-dependent antioxidant and anti-inflammatory protection of the intestinal epithelial barrier, Food Funct., 2022, 13, 3294–3307, 10.1039/d1fo02970c.
- G. F. Cai, A. Wusiman, P. F. Gu, M. N. Mao, S. W. Xu, T. Y. Zhu, J. He, Z. G. Liu and D. Y. Wang, Supplementation of Alhagi honey polysaccharides contributes to the improvement of the intestinal immunity regulating the structure of intestinal flora in mice, Food Funct., 2021, 12, 9693–9707, 10.1039/d1fo01860d.
- T. Sang, C. Guo, D. Guo, J. Wu, Y. Wang, J. Chen, C. Chen, K. Wu, K. Na, K. Li, L. Fang, C. Guo and X. Wang, Suppression of obesity and inflammation by polysaccharide from sporoderm-broken spore of Ganoderma lucidum via gut microbiota regulation, Carbohydr. Polym., 2021, 256, 117594, DOI:10.1016/j.carbpol.2020.117594.
- Y. Xu, B. W. Zhu, X. Li, Y. F. Li, X. M. Ye and J. N. Hu, Glycogen-based pH and redox sensitive nanoparticles with ginsenoside Rh(2) for effective treatment of ulcerative colitis, Biomaterials, 2021, 280, 121077, DOI:10.1016/j.biomaterials.2021.121077.
- J. Bié, B. Sepodes, P. C. B. Fernandes and M. H. L. Ribeir, Polyphenols in Health and Disease: Gut Microbiota, Bioaccessibility, and Bioavailability, Compounds, 2023, 3, 40–72, DOI:10.1016/j.jff.2019.103689.
- X. Sun, X. Cheng, J. Zhang, Y. Ju, Z. Que, X. Liao, F. Lao, Y. Fang and T. Ma, Letting wine polyphenols functional: Estimation of wine polyphenols bioaccessibility under different drinking amount and drinking patterns, Food Res. Int., 2020, 127, 108704, DOI:10.1016/j.foodres.2019.108704.
- M. Y. Zhang, Y. Zhao, N. Wu, M. S. Xu, H. Y. Du and Y. G. Tu, The anti-inflammatory activity of peptides from simulated gastrointestinal digestion of preserved egg white in DSS-induced mouse colitis, Food Funct., 2019, 9, 6445–6455, 10.1039/c8fo01939h.
- S. Luo, R. Wen, Q. Wang, Z. Zhao, F. Nong, Y. Fu, S. Huang, J. Chen, L. Zhou and X. Luo, Rhubarb Peony Decoction ameliorates ulcerative colitis in mice by regulating gut microbiota to restoring Th17/Treg balance, J. Ethnopharmacol., 2019, 231, 39–49, DOI:10.1016/j.jep.2018.08.033.
- D. Wu, S. Chen, X. Ye, S. Ahmadi, W. Hu, C. Yu, K. Zhu, H. Cheng, R. J. Linhardt and Q. He, Protective effects of six different pectic polysaccharides on DSS-induced IBD in mice, Food Hydrocolloids, 2021, 127, 107209, DOI:10.1016/j.foodhyd.2021.107209.
- Q. Li, Y. Cui, B. Xu, Y. Wang, F. Lv, Z. Li, H. Li, X. Chen, X. Peng, Y. Chen, E. Wu, D. Qu, Y. Jian and H. Si, Main active components of Jiawei Gegen Qinlian decoction protects against ulcerative colitis under different dietary environments in a gut microbiota-dependent manner, Pharmacol. Res., 2021, 170, 105694, DOI:10.1016/j.phrs.2021.105694.
- C. C. Gao, G. W. Li, T. T. Wang, L. Gao, F. F. Wang, H. W. Shang, Z. J. Yang, Y. X. Guo, B. Y. Wang and J. D. Xu, Rhubarb extract relieves constipation by stimulating mucus production in the colon and altering the intestinal flora, Biomed. Pharmacother., 2021, 138, 111479, DOI:10.1016/j.biopha.2021.111479.
- M. Guo, D. Xing, J. Wang, Y. Zhang, Z. Li and X. Jiao, Potent Intestinal Mucosal Barrier Enhancement of Nostoc commune Vaucher Polysaccharide Supplementation Ameliorates Acute Ulcerative Colitis in Mice Mediated by Gut Microbiota, Nutrients, 2023, 15, 3054, DOI:10.3390/nu15133054.
- L. Cui, X. Guan, W. Ding, Y. Luo, W. Wang, W. Bu, J. Song, X. Tan, E. Sun, Q. Ning, G. Liu, X. Jia and L. Feng, Scutellaria baicalensis Georgi polysaccharide ameliorates DSS-induced ulcerative colitis by improving intestinal barrier function and modulating gut microbiota, Int. J. Biol. Macromol., 2020, 166, 1035–1045, DOI:10.1016/j.ijbiomac.2020.10.259.
- R. C. Sprong, A. J. Schonewille and R. van der Meer, Dietary cheese whey protein protects rats against mild dextran sulfate sodium-induced colitis: role of mucin and microbiota, J. Dairy Sci., 2010, 93, 1364–1371, DOI:10.3168/jds.2009-2397.
- C. Wang, J. Bai, B. Wang, L. Yu, F. Tian, J. Zhao, H. Zhang, H. Suo, W. Chen and Q. Zhai, Stachyose modulates gut microbiota and alleviates DSS-induced ulcerative colitis in mice, Food Sci. Hum. Wellness, 2023, 12, 2211–2220, DOI:10.1016/j.fshw.2023.03.041.
- M. Xi, Q. Yao, W. Ge, Y. Chen, B. Cao, Z. Wang, X. Cui and Q. Sun, Effects of stachyose on intestinal microbiota and immunity in mice infected with enterotoxigenic Escherichia coli, J. Funct. Foods, 2020, 64, 103689, DOI:10.1016/j.jff.2019.103689.
- N. Wang, W. Chen, C. Cui, Y. Zheng, Q. Yu, H. Ren, Z. Liu, C. Xu and G. Zhang, The Peanut Skin Procyanidins Attenuate DSS-Induced Ulcerative Colitis in C57BL/6 Mice, Antioxidants, 2022, 11, 2098, DOI:10.3390/antiox11112098.
- M. E. U. Rehman, A. Chattaraj, A. Mahboob, Z. Ijaz, D. Franco, M. Farhan, K. Dharma, H. Mumtaz, S. Saeed, J. Basit, M. M. Aslam, A. Iftikhar, F. Faraz and F. Anwer, Total Body Irradiation Versus Chemotherapy Conditioning in Pediatric Acute Lymphoblastic Leukemia Patients Undergoing Hematopoietic Stem Cell Transplant: A Systematic Review and Meta-Analysis, Clin. Lymphoma, Myeloma Leuk., 2023, 23, 249–258, DOI:10.1016/j.clml.2023.01.004.
- Z. Li, S. Zhang, L. Xu, X. Fang, Y. Wan, D. Yu and Y. Guo, A tetrapeptide from maize combined with probiotics exerted strong anti-inflammatory effects and modulated gut microbiota in DSS-induced colitis mice, Food Funct., 2022, 13, 12602–12618, 10.1039/d2fo02678c.
- H. M. Hamer, D. Jonkers, K. Venema, S. Vanhoutvin, F. J. Troost and R. J. Brummer, Review article: the role of butyrate on colonic function, Aliment. Pharmacol. Ther., 2008, 27, 104–119, DOI:10.1111/j.1365-2036.2007.03562.x.
- S. Rose, S. C. Bennuri, J. E. Davis, R. Wynne, J. C. Slattery, M. Tippett, L. Delhey, S. Melnyk, S. G. Kahler, D. F. MacFabe and R. E. Frye, Butyrate enhances mitochondrial function during oxidative stress in cell lines from boys with autism, Transl. Psychiatry, 2018, 8, 42, DOI:10.1038/s41398-017-0089-z.
- E. J. Laserna-Mendieta, A. G. Clooney, J. F. Carretero-Gomez, C. Moran, D. Sheehan, J. A. Nolan, C. Hill, C. G. M. Gahan, S. A. Joyce, F. Shanahan and M. J. Claesson, Determinants of Reduced Genetic Capacity for Butyrate Synthesis by the Gut Microbiome in Crohn's Disease and Ulcerative Colitis, J. Crohns Colitis, 2018, 12, 204–216, DOI:10.1093/ecco-jcc/jjx137.
- L. Su, C. Mao, X. Wang, L. Li, H. Tong, J. Mao, D. Ji, T. Lu, M. Hao, Z. Huang, C. Fei, K. Zhang and G. Yan, The Anti-colitis Effect of Schisandra chinensis Polysaccharide Is Associated With the Regulation of the Composition and Metabolism of Gut Microbiota, Front. Cell. Infect. Microbiol., 2020, 10, 519479, DOI:10.3389/fcimb.2020.519479.
- G. Ma, B. Muinde, L. Zhao, W. Yang, F. Pei and Q. Hu, In vivo fermentation of Pleurotus eryngii polysaccharide and its effects on fecal microbiota composition and immune response, Food Funct., 2017, 8(5), 1810–1821, 10.1039/c7fo00341b.
|
This journal is © The Royal Society of Chemistry 2024 |
Click here to see how this site uses Cookies. View our privacy policy here.