DOI:
10.1039/D4NH00307A
(Communication)
Nanoscale Horiz., 2024,
9, 2007-2015
Engineering in situ growth of Au nanoclusters on hydrophilic paper fibres for fluorescence calligraphy-based chemical logic gates and information encryption†
Received
25th June 2024
, Accepted 19th August 2024
First published on 20th August 2024
Abstract
Gold nanoclusters (AuNCs) are a type of rising-star fluorescence nanomaterials, but their properties and applications are hindered by the multi-step synthesis and purification routes, as well as the lack of desired supporting substrates. To enhance optical performance and working efficiency, the synthesis and applications of AuNCs are suggested to be merged with emerging substrates. Herein, glutathione-modified hydrophilic rice papers are incubated in chloroauric acid aqueous solutions, and the oxidation–reduction reaction between glutathione and Au ions enables the in situ formation of fluorescent AuNCs on the solid fibres of rice papers. The in situ growth of fluorescent AuNCs on rice papers resulted in eye-catching fluorescence tracks, similar to traditional Chinese conventional calligraphy; thus, this fluoresence calligraphy is defined in this work. The entire process, including synthesis and signal responses, is extremely simple, rapid, and repeatable. Moreover, the diversity of additive chemical reagents in the studied rice papers resulted in responsive fluorescence calligraphy, and the as-synthesized AuNC materials exhibited high reliability and optical stability. Significantly, with the integration of synchronous formation and application of Au nanoclusters on hydrophilic paper substrates, high-performance logical gates and information encryption systems were constructed, remarkably facilitating the progress of molecular sensing and important information transmission.
New concepts
With a one-step and rapid staining treatment of HAuCl4 solution, Au nanoclusters are grown in situ on the glutathione-modified fibres of hydrophilic paper substrates. In comparison to previous pre-synthetic Au nanocluster protocols in homogenous solution, the growth of Au nanoclusters at the solution-fibre interfaces promotes the uniform distribution of Au nanoclusters on the solid substrates, and enhanced fluorescence stabilities are also achieved. More importantly, inspired by Chinese conventional calligraphy skills, we introduced HAuCl4 solutions as the ink, and the in situ reactions on hydrophilic rice papers result in Au nanocluster-induced fluorescence marks on the rice papers, which facilitate an emerging topic of fluorescence calligraphy. Due to its merits of operation simplicity, relatively less cost, high stabilities and uniformity, Au nanocluster staining-based fluorescence calligraphy is preliminarily used for chemical logic gates and information encryption in our developed protocol, exhibiting its high potential in the innovated skills for message transmission and nanotechnologies.
|
Introduction
Au nanoparticles and nanocluster-based nanotechnologies have been widely used in numerous research fields, such as sensing,1,2 biotherapies,3,4 imaging,5,6 catalysis,7,8 and optical devices,9,10 due to their attractive plasmonic and fluorescence characteristics, respectively. So far, a majority of these studies have been conducted on the basis of the liquid solution-assisted synthesis of Au nanomaterials, and these pre-synthetic nanomaterials were further investigated after material separation and purification.11–14 Nevertheless, the optical properties of Au nanomaterials are extremely responsive to various parameters, such as surface modification, material sizes and shapes, and inter-material distances.15–18 As a consequence, non-specific surface adsorption and inter-particle aggregation of Au nanomaterials in complex samples largely weaken their optical stability and reliability.19–21 Significantly, the low stability and environment-sensitive properties of pre-synthetic Au nanomaterials in homogenous solutions have largely restricted their in-depth studies and practical applications.
There are two routes to address the above issues, thereby elevating the practicality of Au nanomaterials.22 First, Au nanomaterials could be prepared with the protection of outstanding supporters. Therefore, numerous materials, such as nucleic acids,23,24 proteins,25 inorganic materials,26 polymers,27,28 and small molecules, have been utilized for surface modification.29 Due to the electrostatic repulsion of modifiers, Au nanomaterials exhibited comparable stability in their working substrates, such as hydrogels.22,30 Second, it was noted that the reaction precursors of Au nanomaterials (e.g., chloroauric acid) are easily and stably stored. If Au nanomaterials could be freshly prepared in the presence of their reaction precursors, the synchronous synthesis and application of in situ formed Au nanomaterials would provide enhanced stability and working efficiency.26 Therefore, as an alternative to pre-synthetic materials, the in situ formation of Au nanomaterials in these attractive substrates could be facilitated for the high-performance characteristics and applications.31
In our previous works, the in situ synthesis of plasmonic Au nanoparticles in polymeric networks of hydrogels, rather than aqueous solutions, were identified, which could remarkably enhance the sensing performance of plasmonic Au nanomaterials in operation simplicity, selectivity, anti-interference capacity, and analysis accuracies. As a consequence, hydrogels were colourfully stained by the plasmonic Au nanoparticles and the Au staining-based strategies exhibited intriguing characteristics in portable and high-throughput measurements of heavy metal ions and electrophoretic protein recognition.32,33
Even the synthesis of fluorescent Au nanoclusters in liquid phase was easily achieved.34,35 The in situ growth of Au nanoclusters (AuNCs) on solid fibre-based paper substrates was rarely studied. Herein, we screened different paper substrates according to their hydrophilic and hydrophobic diversity, and a series of Chinese traditional calligraphy papers (e.g., half-raw and whole-raw rice papers) were utilized for the in situ synthesis of fluorescent AuNCs with the integration of the Au staining protocol. Based on the fluorescence marks of these in situ formed AuNCs on rice papers, an emerging topic of fluorescence calligraphy was proposed. The desired simplicity and optical stabilities of fluorescence calligraphy enabled the development of chemical logic gates and further contributed to the construction of an emerging information encryption system.
Results and discussion
Aqueous droplets of pre-synthetic AuNCs split on diverse papers
Glutathione-stabilized AuNCs (GSH–AuNCs) were simply prepared via the addition of HAuCl4 solution in the GSH solution. It was supposed that GSH could reduce Au ions, forming low-valence Au in the aqueous phase. Furthermore, the Au+ and Au atoms aggregated and a great number of NCs were formed.36,37 To implement the solid substrate-based applications, the behaviours of AuNCs on different paper substrates were studied. First, the contact angle experiments were carried out in order to investigate the surface tension of AuNCs aqueous solutions on different paper-based materials. The surface tension values were characterized by comparing the contact angle of droplets on diverse widely used paper bases (Fig. 1(a)). The low contact angle results indicated that the NC membrane, half-raw rice paper, and whole-raw rice paper exhibited high affinity toward the AuNCs aqueous solution. Additionally, the whole-raw rice paper displayed the highest affinity among these hydrophilic materials. Moreover, the fluorescence of pre-synthetic GSH–AuNCs on different paper substrates was investigated by dropping the AuNCs solution on the top surfaces of the studied papers (Fig. 1(b)). Due to the hydrophobic characteristics of PVDF and other studied papers, droplets of AuNCs could be clearly observed on those substrates in the beginning, and the fluorescence properties with the irradiation of UV light (254 nm) were varied (Fig. 1(b)). The hydrophobic paper bases displayed small fluorescent rings while the strongly hydrophilic papers displayed larger rings. It was suggested that due to the coffee ring effect, AuNCs aggregated when the liquid dried, and consequently, and the fluorescence properties, which relied on the dispersion and aggregation states of AuNCs, were randomly altered (Fig. 1(c)). Nevertheless, in terms these those hydrophilic paper substrates, the liquid of AuNCs solution was rapid adsorbed by the papers on the basis of the capillary effect, but the diffusion of AuNCs nanomaterials caused by the liquid flow caused the nonuniform distribution of nanomaterials on paper substrates (Fig. 1(d)).38 To facilitate the paper-based applications of fluorescent AuNCs, improved strategies should be proposed to address the low fluorescent stabilities and nonuniform material distribution of the as-synthesized AuNCs on paper substrates.
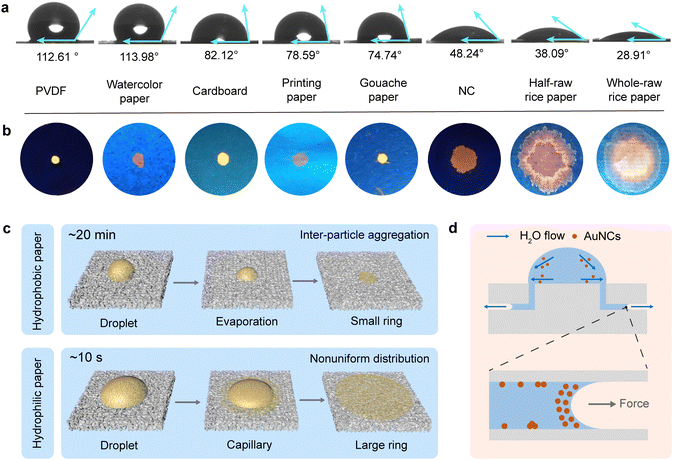 |
| Fig. 1 Studies of AuNCs aqueous droplets on diverse hydrophilic and hydrophobic paper substrates. (a) Image results of contact angle tests of AuNCs aqueous droplets on different paper substrates. (b) Fluorescence images of the dried droplets of liquid-phase prepared GSH–AuNCs solution under the irradiation of a 254 nm UV lamp. (c) Scheme showing the differences in liquid droplets split on hydrophobic and hydrophilic paper substrates. (d) Illustration showing the water flow of a typical liquid droplet on the hydrophilic papers due to the capillary effect. | |
As an alternative of the homogenous-phase liquid solution method, emerging strategies about the in situ growth of AuNCs on hydrophilic paper substrates were proposed. Rice papers, as the ideal substrates of Chinese conventional calligraphy, were considered as the examples. First, the studied rice papers were incubated in GSH solutions and GSH molecules were immobilized on paper fibres due to the molecular penetration. Subsequently, after drying, the modified papers were used for the drop-staining of HAuCl4 solutions (Fig. 2(a)). In addition, the morphologies of the rice paper bases were viewed by SEM images (Fig. 2(b) and (c)). Additionally, the wire-like structures of both half-raw and whole-raw rice papers remained constant even after the modification of GSH. After drop-staining with different concentrations of HAuCl4, the rice papers exhibited dependent fluorescence performances under the irradiation of UV light. When the concentrations were lower than 10.0 mM, uniform fluorescence spots were observed from the rice papers and the fluorescence intensities were increased with the increasing concentrations of HAuCl4 (Fig. 2(d)). In contrast, when the concentrations were higher than 15.0 mM, only fluorescence circles were identified at the boundaries of the droplets. It was indicated that the fluorescence of these reactant materials might be quenched when the high-concentration HAuCl4 was employed.
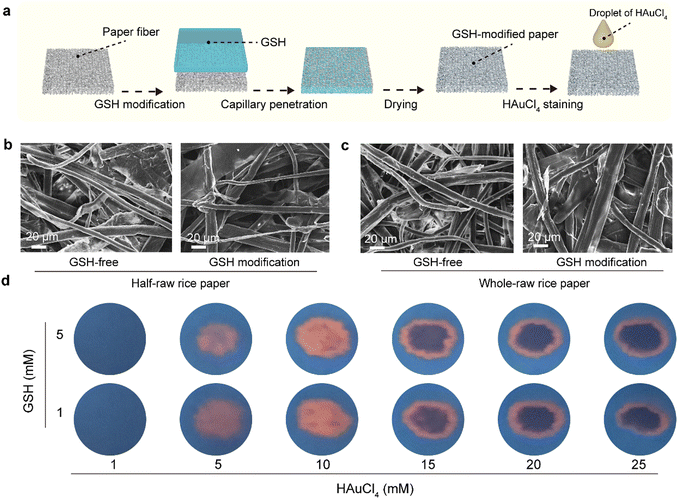 |
| Fig. 2 GSH modification of paper substrates and the drop-staining of HAuCl4 solutions on the paper substrates. (a) Scheme showing the GSH modification and the HAuCl4 staining of different paper substrates. (b) and (c) SEM images showing the microstructures of the half-raw (b) and whole-raw (c) rice paper substrates before and after the GSH modification, respectively. (d) Fluorescence images of the GSH-modified paper substrates after the staining of HAuCl4 solutions and irradiation by a 254 nm ultraviolet lamp. | |
Chloroauric acid-induced fluorescence calligraphy on hydrophilic rice papers
Herein, inspired by Chinese conventional calligraphy, which marks the characters and information with the black carbon ink on rice papers, we used chloroauric acid as an alternative of black carbon ink and painted the characters of SWU on the hydrophilic papers using a Chinese brush (Fig. 3(a)). After incubation for different times, the rice papers were irradiated by UV light and a red fluorescence image representing the label of SWU was correspondingly recorded (Fig. 3(b)). After the incubation for only 30 s, the fluorescence intensity remained constant, indicating the great speed of the reaction on rice papers (Fig. S1, ESI†). Significantly, the fluorescence mark was rapidly presented and supposed to be used for calligraphy. In our work, we called the staining of HAuCl4 on modified rice papers as the fluorescence calligraphy. Furthermore, to implement the quantitative analysis of fluorescence intensities, the fluorescence calligraphy pictures were transferred into a gel imager instrument, which are widely used for gel electrophoretic imaging, and fluorescence imaging tests were correspondingly conducted (Fig. 3(c)). The fluorescence imaging results were in accordance with the fluorescence pictures, exhibiting the quantitative performances of the fluorescence calligraphy protocol for further applications. As a comparison, the HAuCl4 staining of rice papers was conducted under room temperature and 90 °C, respectively. To record their reaction processes and further quantify the fluorescence intensity, the reactions were tracked with a gel fluorescence imager (Fig. S2, ESI†). From fluorescence analysis, it was indicated that the temperature had weak influences on AuNCs formation. Even a high temperature, such as 90 °C, could contribute to the uniform fluorescence staining of rice papers using the in situ-prepared AuNCs. Moreover, the three-dimensional analysis of fluorescence calligraphy on rice papers could be easily and clearly presented via a gel imager (Fig. 3(d)), which exhibited high potential in fluorescence sensing and optical characterization skills.
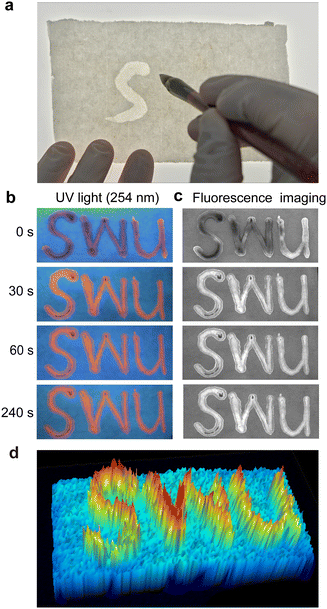 |
| Fig. 3 Fluorescence staining of rice papers induced by the in situ growth of Au nanoclusters on the hydrophilic solid-phase paper fibres. (a) Photo displaying the calligraphy on a rice paper with the ink of HAuCl4 solution. (b) Time-dependent images of the HAuCl4 stained rice paper under the irradiation of 254 nm UV lamp. (c) Corresponding time-dependent fluorescence intensity images of the HAuCl4 stained rice paper from the gel imager. (d) Three-dimensional fluorescence responses of the stained rice paper. | |
To emphasize the benefits of the in situ reaction, the stabilities of different AuNCs were explored. Both in situ prepared AuNCs and pre-synthesized AuNCs were compared (Fig. S3 and S4, ESI†). Nevertheless, in comparison to pre-synthesized AuNCs, the in situ prepared AuNCs promoted the uses of non-aqueous substrates, such as rice papers, for expanded optical applications. In contrast, due to the capillary effects, pre-synthesized AuNCs aggregated rapidly. Consequently, the droplets of pre-synthesized AuNCs were detrimental to uniform patterning. In addition, the reaction rate in liquid phase was much slower than that in the paper phase considering the highly effective interfacial process during the growth of AuNCs. The optical performance of the in situ prepared samples was highly stable even after 8 days.
In situ growth of AuNCs on solid-phase fibres of hydrophilic papers
To investigate the products of fluorescence calligraphy, the characterization of the stained rice papers was conducted. The fluorescence spectra of the products after the liquid phase reaction and in situ reaction were recorded (Fig. S5, ESI†). The in situ reacted rice paper showed stable fluorescence intensity under 530 nm light excitation. After the reactions, the SEM images of the stained rice paper showed the micro-structures of the long paper fibres (Fig. 4(a)). In addition, the energy dispersive X-ray spectrum (EDX) mapping result exhibited that Au elements overlapped the C and O elements, suggesting the formation of small-scale Au nanostructures on paper fibres. Furthermore, the materials on rice papers were collected after the sonication and centrifugation treatments. After that, the separated reactant products were redispersed into the aqueous solution and TEM tests were carried out (Fig. 4(b)). In the low-magnification TEM image, the presence of long paper fibres and numerous dots was observed, indicating the supporting role of paper fibres to nanomaterials. Moreover, the high-resolution TEM image of these dots showed crystal lattices with spaces of 0.236 nm, which corresponded to the Au {111} faces of the typical face-centred cubic (fcc) Au crystals (Fig. 4(c)). The fast Fourier transform (FFT) crystal analysis result also supported the presence of crystal lattice results (Fig. 4(d)). In addition, the size distribution was displayed in Fig. S6 (ESI†), and the average size of the as-synthesized Au nanoclusters was calculated to be 3.16 nm in diameter. Therefore, the fluorescence calligraphy was attributed to the in situ formed AuNCs on the paper fibres. The Fourier transform infrared (FT-IR) spectrum results provided insights into the reaction mechanisms. The series peaks at 3400-800 cm−1 were the characteristic peaks of cellulose and hemicellulose in rice papers. The peak at 897 cm−1 was generated by the deformation and expansion vibration of the heterohead carbon in hemicellulose (Fig. 4(e) and (f)). The peaks of 1052 cm−1 and 1029 cm−1 corresponded to the stretching vibration of cellulose alcohol hydroxyl C-OH, and the 1160 cm−1 peak was attributed to the asymmetric vibration of the cellulose ring. As shown in Fig. 4(g), it could be observed that the spectrum of in situ formed AuNCs had an obvious peak at 609 cm−1, which might be derived from the characteristic peak of the Au–S bond. In summary, the characteristic absorbance peaks of GSH could be identified on the modified rice paper, indicating the initial modification of GSH molecules on the fibres of rice papers. After the staining reaction, the presence of an Au-S characteristic peak and the dropped C
O peak of rice paper indicated that chlorauric acid reacted with the carbonyl groups in GSH, which facilitated the in situ synthesis of GSH–AuNCs.
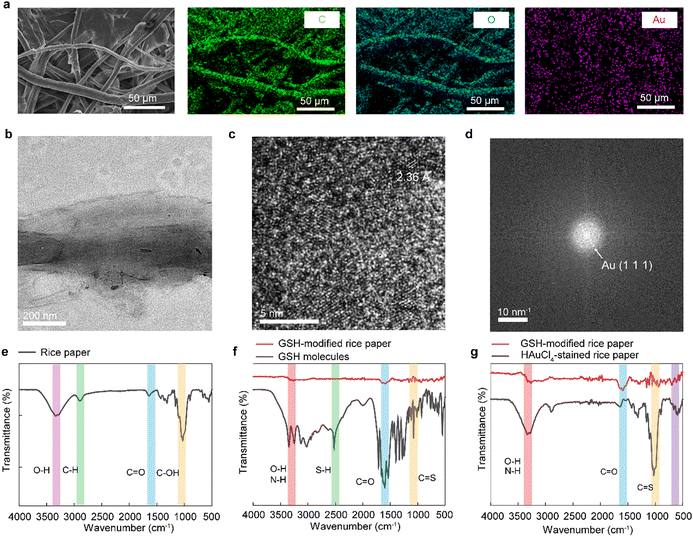 |
| Fig. 4 Characterization of materials grown on paper fibres and proposed identification of the reaction mechanism. (a) SEM and EDX of the stained rice paper. (b)–(d) TEM (b), HRTEM (c) and FFT crystal analysis (d) of the extracted products collected from the stained rice papers. (e) and (f) FTIR results of the rice papers before (e) and after (f) GSH modification. (g) FTIR tests of GSH-modified rice papers before and after the HAuCl4 staining-based fluorescence calligraphy. | |
Fluorescence calligraphy for chemical logic gates
It was proved that fluorescence calligraphy was motivated by AuNCs, which were in situ formed on paper fibres (Fig. 5(a)). Moreover, the in situ reaction showed the appearance of unique staining patterns and provided the input values for encryption systems. With the stabilization effect of paper fibres, AuNCs were uniformly dispersed on paper substrates and the enhanced fluorescence properties were identified. To identify the effect of fluorescence calligraphy caused by different concentrations of GSH, the fluorescence values (R) of diverse molecule-modified rice papers under the irradiation of UV lamp were recorded (Fig. 5(b)). Moreover, the successful achievement of AuNC-stained rice papers with GSH modification inspired the application potential of molecular recognition. Herein, as alternatives of GSH, other 13 types of molecule reagents were studied. In the beginning, the rice papers were modified with arrays of small molecules before the staining of HAuCl4. As the emission lights of the samples were orange, the R values of the paper bases were selected as the output values. According to the R values from the 14 studied substances, which are GSH, cysteine (Cys), L-methionine (Met), L-proline (Pro), L-serine (Ser), L-aspartic acid (Asp), L-lysine (Lys), L-glutamic acid (Glu), L-leucine (Leu), L-threonine (Thr), L-arginine (Arg), L-valine (Val), L-phenylalanine (Phe) and ascorbic acid (AA). Their molecular responses were divided into two categories. After the reaction, the R values, obtained from 5 mM GSH, 50 mM Cys, and 50 mM Met, were more than 120 and they were marked as AR. In contrast, the R values from other samples were lower than 120 and consequently labelled as UR. The fluorescence responses from different additive modifiers were recorded (Fig. S7, ESI†). The responsive differences in fluorescence intensities gave rise to the construction of the chemical logical neurons. AR, UR, chlorauric acid (10 mM) and ultraviolet lamp (UV) were taken as inputs, and the digital signal R values were considered as outputs when the R value of 120 was the threshold. The obtained R values higher than 120 were assigned to the logical value “1”, and the responses lower than 120 were assigned to the logical value “0” (Fig. 5(c)). Therefore, a chemical logic gate one based on the fluorescence calligraphy protocol was constructed (Fig. 5(d)).39 In addition, the readouts of the sample under different UV wavelengths and visible light were recorded, respectively (Fig. S8, ESI†). Similarly, other two logic gates were also established on the basis of different experimental parameters (Fig. S9 and S10, ESI†).
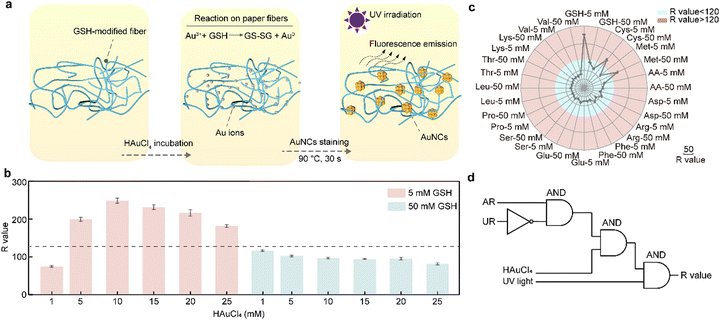 |
| Fig. 5 Construction of the chemical logic gate based on the AuNCs staining of hydrophilic papers. (a) Scheme showing the structural changes in the in situ formation of GSH–AuNCs. (b) Column plot of the R values of the stained papers with different concentrations of HAuCl4 and GSH. (c) Radar plot showing the relationship between the studied small molecules and the fluorescence R values of fluorescence calligraphy. (d) Scheme of a constructed logic system corresponding to the fluorescence R values. | |
Fluorescence calligraphy for information encryption
Calligraphy was previously used for character recording and message transmission in ancient China. Our proposed fluorescence calligraphy might exhibit high-performance in relevant fields, such as information encryption. As is known, cryptographic steganography usually hides information through the two steps of cryptography and steganography (Fig. 6(a)).40 Consequently, the encrypted messages were decoded through the cryptography, which remarkably reduced the risk of information disclosure; thus, it ensured the security of information transmission. In order to explore the applications of the AuNCs staining-based chemical logic system in information encryption using the above logic gate (Fig. 5(d)), we converted the digital signals of fluorescence calligraphy into a specific order of binary strings so as to build a 28-bit molecular logic computation. The advanced information security system could responsively encode and decode information.41 Based on the encrypted information, we built a simple cryptographic steganography model, which converted information through a cryptographic algorithm and hid the encrypted content with the disguised information (Fig. 6(b)). The encrypted content could only be decoded by the specific steganographic key. Herein, 14 substances with concentrations of 5.0 mM and 50.0 mM were employed as 28 inputs of the encryption system. According to the specified threshold (R value = 120), the value exceeding the threshold was defined as the logical value “1”. Otherwise, it was defined as zero. Thus, a 28-bit binary string can be obtained in a specific order (Fig. 6(c)), and the logical values can be employed for encoding specific characters, such as English letters, numbers, and conversely decrypting the information with a specific key through the steganographic model (Fig. 6(d)). Moreover, the Arabic numbers from 1 to 9 and parts of English letters were encoded into 7-bit binary characters (27 = 128, Fig. 6(c) and (d)), 8-bit binary strings (28 = 256), and 9-bit binary strings (29 = 512), respectively, to form a simple substitution cipher. The fluorescence signals of different reactants in the AuNCs staining-based fluorescence calligraphy can be encoded with 14 (7-bit), 14 (8-bit), and 14 (9-bit) keys by successively shifting 1 bit. Considering that all information encryption technologies have the risk of being cracked, setting uncertain multi-key and diversified text layout can significantly increase the difficulty of information cracking. Therefore, the designed binary string key randomly corresponds to the alphabet and Arabic numerals, which increases the difficulty of information encryption. As a result, the security of encrypted information is improved. Finally, the encrypted information is hidden in a molecular logic system, and since the letters and Arabic numerals correspond to the same binary string, it can be decrypted into multiple encrypted texts through an encryption key. Herein, the exact ciphertext is as follows: “The world puts off its mask of vastness to its lover.” (Fig. 6(e)). Unless the chemical logic system and its codebook are known, the correct information cannot be decrypted. These results showed that our molecular encryption system had unique information concealment and controllability, exhibiting a highly promising application prospect in security-enhanced information transmission.
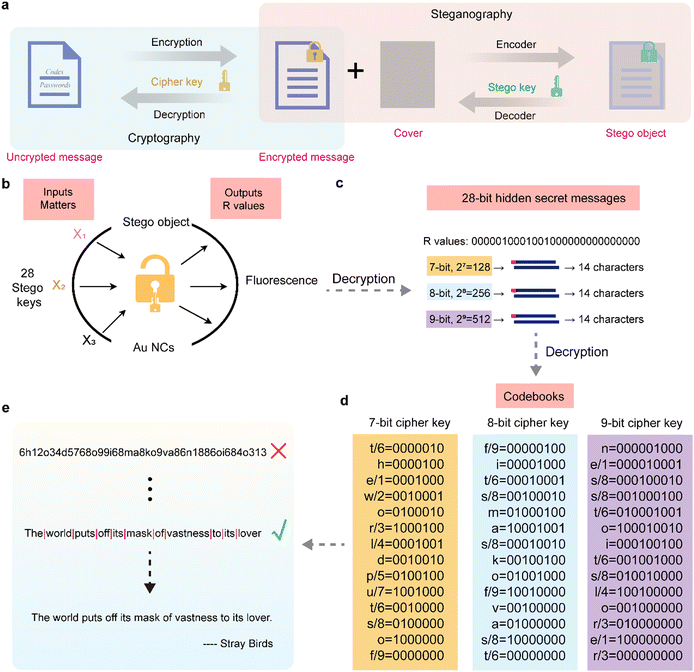 |
| Fig. 6 Improved information encryption based on AuNC-based fluorescence calligraphy. (a) Basic principles of encryption and decryption. (b) Scheme showing the password steganography on the basis of the fluorescence calligraphy-induced logic system 1. (c) Information coding of selective patterns. (d) Decryption of secret messages with their corresponding meanings. (e) Illustration indicating the decryption of molecular steganography information. | |
Conclusions
In this work, the fluorescence staining of papers was implemented via the in situ formation of ultrasmall AuNCs on the fibres of GSH-modified paper substrates. The AuNC-stained paper exhibited high optical stabilities due to the protection of paper fibres. Compared to fluorescent molecules, AuNCs could be used in diverse surroundings without material degradation. Therefore, as the carriers of information, the AuNCs exhibited high potential in multi-functional uses with effective characteristics. Facilitated by AuNCs staining, an emerging concept of fluorescence calligraphy was proposed. The fluorescence AuNCs staining effect relied on the hydrophilic and hydrophobic performances of the papers. The comparable surface tension of the reaction solutions on hydrophilic papers resulted in the uniform and stable synthesis of AuNCs; thus, the effective staining of hydrophilic substrates was achieved in this strategy. Herein, for the widely used papers in Chinese traditional calligraphy, rice papers were taken as examples of hydrophilic materials for the paper staining study. After the incubation of GSH-modified rice papers in HAuCl4 solutions, the growth of AuNCs enables the fluorescence calligraphy of rice papers. Due to the high stabilities, simplicity, and speed of fluorescence calligraphy, the AuNCs-based fluorescence staining system contributed to the construction of chemical logic gates and advanced systems of information encryptions with high potential for practical message transmission and molecular recognition.
Experimental section
Synthesis of GSH-stabilized AuNCs in aqueous solutions
The synthesis of GSH-stabilized AuNCs in aqueous solutions was conducted according to the previous reports.42,43 Firstly, the stock solutions of 60 mM GSH and 4.0 mM HAuCl4 were prepared, respectively. To initiate the reaction, the HAuCl4 solution (10 mL) and diluted GSH solution (10 mL) were added into a glass bottle. After the mixing, the samples were placed in a water bath (90 °C) for 1 h to obtain the products of GSH-stabilized AuNCs.
Fluorescence staining of rice papers by HAuCl4 solutions
Rice papers were incubated into the GSH aqueous solutions with different concentrations for 10 min, enabling the modification of GSH molecules on the paper fibres. After drying, a drop of chloroauric acid solution (3.0 μL) with different concentrations was split onto the rice papers. Subsequently, the rice papers were transferred to an oven for incubation (90 °C, 30 s). The reactant rice papers were taken from the oven and irradiated under UV light (254 nm) for fluorescence recording. The rice papers were stained via the synthesis of fluorescent AuNCs. Significantly, the AuNCs were in situ formed on the paper fibres with the desired characteristics of simplicity, high fluorescence emission, and optical stabilities.
Characterization of paper fibre-supported AuNCs
The SEM scanning results of the AuNCs-stained papers were obtained after the leakage of Pt coating. In terms of TEM characterization, the as-synthesized nanomaterials were separated from paper fibres via the sonication-assisted method. First, the papers after AuNCs staining were divided into small pieces and put into a 20 mL glass bottle with ultrasonic treatments for 1 h. The treated supernatant was collected and further treated with centrifugation (1000 rpm, 15 min). After centrifugation, the AuNCs were dispersed in the supernatant and the solution was transferred onto the TEM copper mesh for material characterization.
Construction of emerging logic gates and information encryption system
To obtain increasing number of chemical inputs, 13 extra types of small molecules were introduced, which replaced GSH for the modification of rice papers and preparation of AuNCs. Meanwhile, other incubation processes remained consistent. The developed molecular encryption and steganography system comprised a paper-based in situ Au nanoclusters-formation system as the stego object.44 Additionally, the molecular paper modifiers, including GSH, Met, Cys, Pro, Ser, Asp, Lys, Glu, Leu, Thr, Arg, Val, Phe and AA, served as the stego keys for the signal inputs. Additionally, the keys were designed as binary strings for broader usage.
Author contributions
Jun Jiang Luo: writing – original draft, methodology, investigation; Dun Ying Guo: methodology, investigation; Zi Bo Qu: methodology, investigation; Hong Qun Luo: conceptualization, writing – review and editing; Nian Bing Li: supervision, writing – review and editing, project administration; Hao Lin Zou: investigation, methodology; Bang Lin Li: investigation, methodology, supervision, writing – original draft, funding acquisition, project administration.
Data availability
This work presents all of the data in figures and tables within the text and ESI.†
Conflicts of interest
There are no conflicts to declare.
Acknowledgements
This study was financially supported by the National Natural Science Foundation of China (No. 22004103), Natural Science Foundation of Chongqing (No. cstc2021jcyj-msxmX0295), and Fundamental Research Funds for the Central Universities (SWU-KT22028). J. J. Luo acknowledges the support from the Innovation Foundation of Chongqing City for Postgraduates (No. CYS240134). Additionally, we acknowledge Miss Hao Lin Zou for the FTIR tests (in situ IRTracer-100, Shimadzu, Japan).
References
- Y. Sun, Z. Zhou, P. Peng, T. Shu, L. Su and X. Zhang, Anal. Chem., 2023, 95, 5886–5893 CrossRef.
- L. Zhu, Z. Y. Wang, J. J. Luo, Y. J. Zheng, H. L. Zou, H. Q. Luo, L. B. Zhao, N. B. Li and B. L. Li, Anal. Chem., 2023, 95, 18859–18870 CrossRef.
- D. Hao, X. Zhang, R. Su, Y. Wang and W. Qi, J. Mater. Chem. B, 2023, 11, 5051–5070 RSC.
- L. Sun, H. Liu, Y. Ye, Y. Lei, R. Islam, S. Tan, R. Tong, Y.-B. Miao and L. Cai, Signal Transduction Targeted Ther., 2023, 8, 418 CrossRef.
- S. Zhou, L. Gustavsson, G. Beaune, S. Chandra, J. Niskanen, J. Ruokolainen, J. V. I. Timonen, O. Ikkala, B. Peng and R. H. A. Ras, Angew. Chem., Int. Ed., 2023, 62, e202312679 CrossRef PubMed.
- B. Singh, R. Bahadur, P. Maske, M. Gandhi, D. Singh and R. Srivastava, Nanoscale, 2023, 15, 2932–2947 RSC.
- L. Tang, T. Duan, Y. Pei and S. Wang, ACS Nano, 2023, 17, 4279–4286 CrossRef PubMed.
- G. X. Pei, L. Zhang and X. Sun, Coord. Chem. Rev., 2024, 506, 215692 CrossRef.
- S. Chandra, A. Sciortino, S. Das, F. Ahmed, A. Jana, J. Roy, D. Li, V. Liljeström, H. Jiang, L. S. Johansson, X. Chen, Nonappa, M. Cannas, T. Pradeep, B. Peng, R. H. A. Ras, Z. Sun, O. Ikkala and F. Messina, Adv. Opt. Mater., 2023, 11, 2202649 CrossRef.
- L. Francés-Soriano, E. Zaballos-García and J. Pérez-Prieto, Adv. Opt. Mater., 2023, 11, 2300337 CrossRef.
- X. Pan, Y. Yao, M. Zhang, X. Yuan, Q. Yao and W. Hu, Nanoscale, 2024, 16, 8196–8215 RSC.
- Q. Yao, Z. Wu, Z. Liu, Y. Lin, X. Yuan and J. Xie, Chem. Sci., 2021, 12, 99–127 RSC.
- H. Zhu, N. Liu, Z. Wang, Q. Xue, Q. Wang, X. Wang, Y. Liu, Z. Yin and X. Yuan, Nanoscale, 2021, 13, 18996–19003 RSC.
- X. Pan, Z. Zuo, Z. Wang, G. Yang, H. Zhu, Y. Li and X. Yuan, Mater. Chem. Front., 2023, 7, 1146–1152 RSC.
- X. Hu, P. Wang, C. Huang, C. Fang, F. Li and D. Ling, Coord. Chem. Rev., 2024, 502, 215632 CrossRef CAS.
- C. Gao, F. Lyu and Y. Yin, Chem. Rev., 2021, 121, 834–881 CrossRef PubMed.
- G. Yang, X. Mu, X. Pan, Y. Tang, Q. Yao, Y. Wang, F. Jiang, F. Du, J. Xie, X. Zhou and X. Yuan, Chem. Sci., 2023, 14, 4308–4318 RSC.
- X. Dou, X. Yuan, Y. Yu, Z. Luo, Q. Yao, D. T. Leong and J. Xie, Nanoscale, 2014, 6, 157–161 RSC.
- Y. Hang, A. Wang and N. Wu, Chem. Soc. Rev., 2024, 53, 2932–2971 RSC.
- S. Stangherlin and J. Liu, J. Mater. Chem. B, 2023, 11, 6994–7003 RSC.
- E. T. Athira and J. Satija, Analyst, 2023, 148, 6188–6200 RSC.
- J. J. Luo, L. R. Zhu, Z. Guo, N. Pi, X. Li, H. L. Zou, H. Q. Luo, N. B. Li and B. L. Li, Coord. Chem. Rev., 2024, 511, 215874 CrossRef.
- Z. Ye, W. Liao, Z. Deng, L. Wang, B. Wen, D. Zhang, H. Wang, W. Xie and H. Peng, Trends Anal. Chem., 2024, 175, 117724 CrossRef CAS.
- H. Watanabe, D. Maehara, T. Nishihara and K. Tanabe, RSC Adv., 2023, 13, 20756–20760 RSC.
- D. Baimanov, L. Wang, K. Liu, M. Pan, R. Cai, H. Yuan, W. Huang, Q. Yuan, Y. Zhou, C. Chen and Y. Zhao, Nanoscale Horiz., 2023, 8, 859–869 RSC.
- H. Zhang, J. J. Luo, Z. Sun, H. L. Zou, H. Q. Luo, N. B. Li and B. L. Li, J. Mater. Chem. C, 2022, 10, 14508–14516 RSC.
- W. Zhang, Y. Bai, Z. Yang, R. Wang, L. Meng and Y. Wang, Eur. Polym. J., 2023, 198, 112386 CrossRef.
- A. Abdelaal, S. Blaize, A. Issa and S. Jradi, Langmuir, 2023, 39, 4583–4588 CrossRef PubMed.
- M. D. A. Ikbal, S. Kang, X. Chen, L. Gu and C. Wang, ACS Sens., 2023, 8, 4696–4706 CrossRef CAS.
- B. L. Li, J. J. Luo, H. L. Zou, Q. M. Zhang, L. B. Zhao, H. Qian, H. Q. Luo, D. T. Leong and N. B. Li, Nat. Commun., 2022, 13, 7289 CrossRef CAS.
- S. Mao, B. Sun, G. Zhou, T. Guo, J. Wang and Y. Zhao, Nanoscale Horiz., 2022, 7, 822–848 RSC.
- H. Zhang, J. J. Luo, R. L. Wang, X. Y. He, H. L. Zou, H. Q. Luo, N. B. Li and B. L. Li, Anal. Chem., 2024, 96, 10074–10083 CrossRef CAS PubMed.
- X. J. Du, Y. Chen, L. Y. Qin, H. Q. Luo, N. B. Li and B. L. Li, Environ. Sci. Technol., 2022, 56, 1041–1052 CrossRef CAS PubMed.
- Y. Li and R. Jin, Nanoscale Horiz., 2023, 8, 991–1013 RSC.
- C. Zhou, P. Pan, X. Wei, Z. Lin, C. Chen, X. Kang and M. Zhu, Nanoscale Horiz., 2022, 7, 1397–1403 RSC.
- Z. Luo, X. Yuan, Y. Yu, Q. Zhang, D. T. Leong, J. Y. Lee and J. P. Xie, J. Am. Chem. Soc., 2012, 134, 16662–16670 CrossRef.
- M. F. Matus and H. Häkkinen, Nat. Rev. Mater., 2023, 8, 372–389 CrossRef.
- C. Zhang, Y. Xu, P. Lu, X. Zhang, F. Xu and J. Shi, J. Am. Chem. Soc., 2017, 139, 16620–16629 CrossRef PubMed.
- Z. X. Xie, Y. Wu, J. Zhou, J. Y. Lu and W. T. Huang, Small, 2024 DOI:10.1002/smll.202401510.
- Y. J. Fan, J. X. Dong, Y. L. Li, Z. G. Wang, Y. Q. Chang, L. F. Ren, M. Su, L. Guan, S. G. Shen, Z. F. Gao, Q. Wei and F. Xia, Anal. Chem., 2023, 95, 11113–11123 CrossRef CAS PubMed.
- Q. Y. Liu, Y. Wu, Z. Q. Bu, M. X. Quan, J. Y. Lu and W. T. Huang, Small, 2023, 19, 2207436 CrossRef CAS.
- C. Z. Peng, H. Zhang, N. Pi, X. Li, Q. Yang, H. L. Zou, H. Q. Luo, N. B. Li and B. L. Li, Environ. Sci.: Nano, 2023, 10, 2880–2889 RSC.
- M. Yi, L. Ma, W. Zhao, J. Zhao, Q. Fan and J. Hao, Langmuir, 2021, 37, 3130–3138 CrossRef CAS.
- L. L. Zheng, J. Z. Li, M. Wen, D. Xi, Y. Zhu, Q. Wei, X.-B. Zhang, G. Ke, F. Xia and Z. F. Gao, Sci. Adv., 2023, 9, eadf5868 CrossRef CAS.
|
This journal is © The Royal Society of Chemistry 2024 |
Click here to see how this site uses Cookies. View our privacy policy here.