DOI:
10.1039/D3QO01416A
(Research Article)
Org. Chem. Front., 2024,
11, 94-99
Nickel-catalyzed dynamic kinetic cross-electrophile coupling of benzylic alcohols and alkenyl triflates†
Received
1st September 2023
, Accepted 11th November 2023
First published on 14th November 2023
Abstract
The direct deoxy-functionalization of alcohols through cross-coupling is synthetically appealing but remains a challenging task. In this manuscript, we report a dynamic kinetic cross-electrophile coupling reaction between benzylic alcohols and alkenyl triflates. This mild reaction enables the replacement of the alcohol group with an alkenyl functionality, yielding versatile benzyl-substituted cyclic alkenes. The production of cyclic alkenes makes the method orthogonal to the existing reductive coupling methodologies, which are mainly effective in producing acyclic derivatives. Functional groups such as ester, phenol, indole, heterocycle, and boronic ester are tolerated.
Introduction
Alcohols are readily available functional groups, making them highly desirable for transition-metal-catalyzed C–C coupling reactions. However, due to the high bond dissociation energy of the C–OH bond, these processes usually involve multistep operations, requiring their pre-functionalization to activated electrophilic or organometallic species (Scheme 1, path a).1 These multistep sequences are costly and time-consuming, and they always restrict the tolerance of functional groups. Consequently, new methods that allow for the direct functionalization of alcohols are in high demand, yet they are currently underdeveloped (Scheme 1, path b).2,3
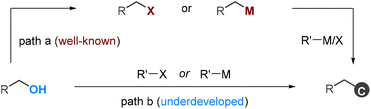 |
| Scheme 1 General approaches for deoxygenative C–C coupling of alcohols by transition metal catalysis. | |
There are only a limited number of studies that demonstrate the deoxygenative C–C coupling of benzylic alcohols. For instance, the Shi group reported transition-metal-catalyzed arylation reactions using Grignard reagents and aryl boroxines as coupling partners.4 Ukaji et al. reported titanium-assisted and nickel-catalyzed cross-electrophile coupling of benzyl alcohols with aryl and alkenyl halides.5 Very recently, our group reported a dynamic kinetic cross-coupling strategy for direct functionalization of alcohols.6 This protocol utilizes the slow equilibrium reaction between alcohols and dimethyl oxalate (DMO); the in situ-formed oxalates are available for the coupling reactions. While the feasibility of this strategy was demonstrated through a deoxyarylation reaction, its potential in organic synthesis remains largely unexplored.
Allylbenzenes are important structural motifs widely found in naturally-occurring molecules. Catalytic cross-electrophile coupling between benzyl and alkenyl electrophiles offers a convenient access to these compounds.7–9 Initially, these studies focus mainly on the reactions involving benzyl halides (Scheme 2a).9 Very recently, the scope of this chemistry has been extended to benzyl alcohols (Scheme 2b).5b Despite formidable advances, these processes are effective mainly in producing acyclic alkenes using alkenyl bromides as coupling partners. In this manuscript, we report a nickel-catalyzed dynamic kinetic cross-electrophile coupling between benzyl alcohols and alkenyl triflates (Scheme 2c). This method demonstrates the direct conversion of alcohols and easily accessible alkenyl triflates, derived from ketones, into alkenes.8 This protocol enables the production of cyclic alkenes, offering an orthogonal approach to existing methodologies.5b,9
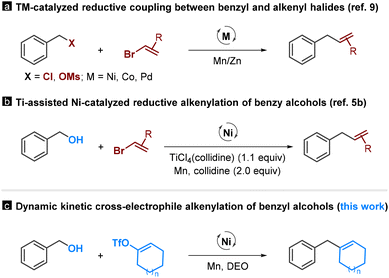 |
| Scheme 2 Cross-electrophile coupling between benzyl and alkenyl electrophiles. | |
Results and discussion
We started our investigations by examining the reaction between alcohol 1a and alkenyl triflate 2a (Table 1). Under the conditions of Ni(dppf)Cl2 (10 mol%), dppf (10 mol%), and phen (2 mol%) in DMF that we had previously established for reductive arylation reactions,6 the reaction yielded compound 3a in a modest 44% yield (Scheme S1 in the ESI†). Our extensive studies revealed that the best result was achieved by using a combination of Ni(dppf)Cl2 (10 mol%), dtbpy (20 mol%), diethyl oxalate (DEO, 1.5 equiv.), Mn (3.0 equiv.), and DMSO/MeCN (1
:
1). These alternative conditions afforded compound 3a in an impressive 94% isolated yield (entry 1). The combination of phosphine and nitrogen ligands was found to be crucial for the activation of both alcohols and alkenyl triflates. The use of a single nitrogen (entries 2 and 3) or a phosphine (entry 4) ligand resulted in the recovery of most of these two substrates. The use of Ni(dppp)Cl2 provided 3a in 68% yield (entry 5). The alkenylation reaction in DMF afforded 3a in 79% yield (entry 6). While performing the reaction in either DMSO or MeCN led to a low yield of 3a, utilizing DMSO/MeCN (1
:
1) significantly enhanced the reaction efficiency (entries 7 and 8). The reaction with Zn instead of Mn delivered 3a in 65% yield (entry 9). The use of DMO (dimethyl oxalate) instead of DEO provided 3a in 82% yield (entry 10). This reagent may affect the efficiency of transesterification of alcohols to oxalates, as well as the activation of oxalates, leading to a slight decrease in the yield. No reaction was observed in the absence of DEO, a nickel catalyst, or a reducing agent (entry 11). While the phen ligand L1 was found to be essential in our prior arylation reaction, it led to the target product in 52% yield. Reactions using bipyridine ligands (L2–3) exhibited greater effectiveness, with the best outcome achieved using dtbpy (L3). Pybox ligand L4 and tridentate nitrogen ligands L5 displayed lower efficacy.
Table 1 Optimization of reaction conditionsa

|
Entry |
Change from standard conditions |
3a (%) |
Reaction conditions: 1a (0.1 mmol) and 2a (0.15 mmol) were used; the yields were determined by NMR analysis using CH2Br2 as an internal standard; DEO: diethyl oxalate.
Isolated yield in parentheses is obtained from 1a (0.2 mmol) and 2a (0.3 mmol).
|
1 |
None |
98 (94)b |
2 |
NiCl2 instead of Ni(dppf)Cl2 |
Trace |
3 |
Ni(COD)2 instead of Ni(dppf)Cl2 |
Trace |
4 |
No dtbpy |
Trace |
5 |
Ni(dppp)Cl2 instead of Ni(dppf)Cl2 |
68 |
6 |
DMF |
79 |
7 |
DMSO |
43 |
8 |
CH3CN |
7 |
9 |
Zn instead of Mn |
65 |
10 |
DMO instead of DEO |
82 |
11 |
No DEO, Ni or Mn |
0 |
|
The substrate scope of alcohol was investigated using alkenyl triflate 2a as a coupling partner (Table 2). Electron-neutral (1b), -rich (1c–j), and -poor (1k–n) benzyl alcohols all coupled well with triflate 2a. The methoxy groups placed at para- (1c), meta- (1d), and ortho-positions (1e) are tolerated, yielding the desired products in 78–85% yields. The reactions involving electron-poor benzyl alcohols showed moderate yields (1k–n). Organosilanes and boronic esters, widely utilized in cross-coupling reactions, were compatible with our conditions and could be incorporated into the coupling products (1p–s). The reactions involving heterobenzylic alcohols, including furan (1t), thiophene (1u and 1v), pyridine (1w), benzothiophene (1x), and indole (1y), generally afforded the target products in good yields. Additional functionalities such as phenol (1z), alkene (1aa and 1ab), amide (1ac), and ester (1ad) were tolerated.
Table 2 The substrate scope of primary benzyl alcoholsa
1a (0.2 mmol) and 2a (0.3 mmol) were used; isolated yields are given.
|
|
We then examined the scope of this reaction with respect to alkenyl triflates (Table 3). In addition to six-membered cyclic alkenyl triflates, five- and eight-membered ring derivatives gave the coupling products in 60% and 43% yields, respectively (2b and 2c). The 4- and 5-substituted alkenyl triflates exhibited good coupling efficiency, affording the alkenylation products in yields up to 93% (2d–j). A bridged cyclic alkenyl triflate 2k resulted in a moderate yield. Heterocyclic alkenyl triflates, such as 3,6-dihydro-2H-pyran (2l) and 3-piperidine (2m–o), were coupled efficiently, delivering the coupling products in 80–95% yields. The reaction could be scaled up to the gram scale without significant loss in efficiency (2n). Acyclic alkenyl triflates are presently less effective; the reactions of 2p and 2q gave moderate yields of the desired products.
Table 3 The substrate scope of alkenyl triflatesa
1a (0.2 mmol) and 2a (0.3 mmol) were used; isolated yields are given.
Reaction at 80 °C.
1a (5.0 mmol) and 2n (7.5 mmol) were used.
Ni(PCy3)2Cl2 (0.02 mmol), 4,4′-dimethyl-2,2′-bipyridyl (0.04 mmol), dppf (0.02 mmol), and ZrCl4 (0.04 mmol) were used, and reaction at 30 °C.
|
|
Standard conditions failed to yield the desired products from secondary alcohols. Reducing dtbpy (10 mol%) and adding MgCl2 (1.2 equiv.) resulted in the formation of coupling products 1ae–ah, albeit with relatively low yields (Table 4). Tertiary alcohols remain unreactive, likely due to their low efficiency in transesterification.
Table 4 The substrate scope of secondary benzyl alcoholsa
1 (0.2 mmol) and 2s (0.3 mmol) were used; isolated yields are given.
Alkenyl triflate 2a was used.
|
|
Late-stage modification of biologically active molecules presents a promising approach for obtaining compounds with modified pharmacological profiles. In this context, benzyl alcohols derived from flufenamic acid and adapalene were successfully coupled with alkenyl triflates, leading to the formation of alkenes 4 and 5 (Scheme 3). Additionally, a nootkatone-derived triflate underwent a benzylation reaction with a yield of 74% (6).
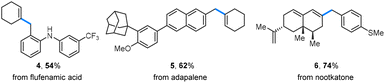 |
| Scheme 3 Late-stage modification of biologically active molecules. Benzyl alcohols (0.2 mmol) were used, and isolated yields are given. | |
In the absence of DEO, the reaction of 1c and 2a under the standard conditions did not give any desired product, indicating the crucial role of DEO in the success of this process (Scheme 4a, left). However, when oxalate 7 was employed, the reaction proceeded efficiently, leading to the formation of 3c in 75% yield (Scheme 4a, right). These results suggest that an in situ-generated oxalate might serve as a key intermediate in this reaction.
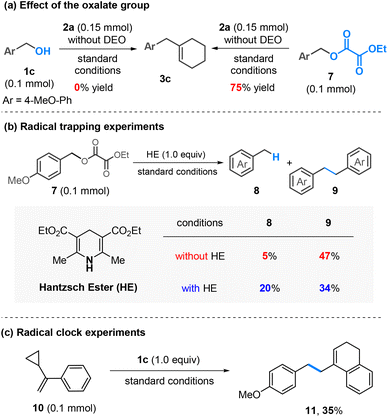 |
| Scheme 4 Mechanistic studies. Standard conditions are as shown in Table 1, entry 1; see the ESI† for the reaction conditions; isolated yields are given for product 3c; the yields were determined by NMR analysis using CH2Br2 as an internal standard for compounds 8, 9, and 11. | |
Our previous studies revealed that nickel activates the oxalate through a single-electron process,6a while cobalt undergoes a two-electron oxidative addition.6b To determine the process involved under the current conditions, several control experiments were conducted. (1) Hantzsch ester (HE) has been established as a good hydrogen atom donor capable of trapping carbon radicals.10 In the presence of HE (1.0 equiv.), the formation of benzyl-H was significantly enhanced, whereas the homo-coupling of benzyl alcohol to dimer 9 was suppressed (Scheme 4b). (2) The reaction of 1c with α-cyclopropylstyrene (10), a well-known radical clock substrate probe,11 yielded a ring-opening and cyclization product 11 in 35% yield (Scheme 4c). These results suggest that the in situ-generated benzyl oxalates may be activated by nickel through a radical process.
Based on the above results, we proposed a catalytic cycle that is analogous to our previous proposal for the deoxy-arylation reaction (Scheme 5).6a The transesterification between alcohol and diethyl oxalate produces a highly reactive oxalate. The reaction of benzyl oxalate with Ni(0), possibly via a radical process, generates benzyl-Ni(II)X.12 Reduction of benzyl-Ni(II)X, followed by oxidative addition with alkenyl triflates, leads to the formation of benzyl-Ni(III)(Ar)X.13 Subsequent reductive elimination resulted in the formation of the coupling product.
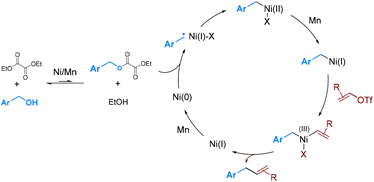 |
| Scheme 5 Proposed mechanism. | |
Conclusions
In conclusion, we have developed a nickel-catalyzed dynamic kinetic cross-electrophile coupling reaction between benzyl alcohols and alkenyl triflates. This method enables the direct transformation of alcohols into structurally diverse benzyl-substituted cyclic alkenes. The reaction proceeds under mild conditions and exhibits good functionality tolerance with ester, phenol, indole, heterocycle, boronic ester etc. These findings expand the synthetic toolbox for deoxy-functionalization of alcohols. Further expansion of the scope of the dynamic kinetic cross-electrophile reaction is ongoing in our laboratory.
Conflicts of interest
There are no conflicts to declare.
Acknowledgements
We thank the National Natural Science Foundation of China (22071084, 22271127) and the Fundamental Research Funds for the Central Universities (lzujbky-2022-ey01) for their financial support.
References
-
(a) A. Rudolph and M. Lautens, Secondary Alkyl Halides in Transition-Metal-Catalyzed Cross-Coupling Reactions, Angew. Chem., Int. Ed., 2009, 48, 2656–2670 CrossRef CAS PubMed;
(b) R. Kuwano, Catalytic Transformations of Benzylic Carboxylates and Carbonates, Synthesis, 2009, 1049 CrossRef CAS;
(c) R. Jana, T. P. Pathak and M. S. Sigman, Advances in Transition Metal (Pd,Ni,Fe)-Catalyzed Cross-Coupling Reactions Using Alkyl-organometallics as Reaction Partners, Chem. Rev., 2011, 111, 1417–1492 CrossRef CAS PubMed;
(d) N. Kambe, T. Iwasaki and J. Terao, Pd-catalyzed cross-coupling reactions of alkyl halides, Chem. Soc. Rev., 2011, 40, 4937–4947 RSC;
(e)
A. de Meijere, S. Bräse and M. Oestreich, Metal Catalyzed Cross-Coupling Reactions and More, Wiley-VCH, 2014, pp. 533–663 CrossRef;
(f) A. H. Cherney, N. T. Kadunce and S. E. Reisman, Enantioselective and Enantiospecific Transition-Metal-Catalyzed Cross-Coupling Reactions of Organometallic Reagents to Construct C–C Bonds, Chem. Rev., 2015, 115, 9587–9652 CrossRef CAS;
(g) E. J. Tollefson, L. E. Hanna and E. R. Jarvo, Stereospecific Nickel-Catalyzed Cross-Coupling Reactions of Benzylic Ethers and Esters, Acc. Chem. Res., 2015, 48, 2344 CrossRef CAS;
(h) G. C. Fu, Transition- Metal Catalysis of Nucleophilic Substitution Reactions: A Radical Alternative to SN1 and SN2 Processes, ACS Cent. Sci., 2017, 3, 692–700 CrossRef CAS PubMed.
-
(a) R. Kumar and E. V. Van der Eycken, Recent approaches for C–C bond formation via direct dehydrative coupling strategies, Chem. Soc. Rev., 2013, 42, 1121–1146 RSC;
(b) X. Pang and X.-Z. Shu, Reductive Deoxygenative Functionalization of Alcohols by First-Row Transition Metal Catalysis, Chin. J. Chem., 2023, 41, 1637 CrossRef CAS.
- Selected examples of cross-electrophile C–C coupling of alcohols:
(a) X.-G. Jia, P. Guo, J. Duan and X.-Z. Shu, Dual nickel and Lewis acid catalysis for cross-electrophile coupling: the allylation of aryl halides with allylic alcohols, Chem. Sci., 2018, 9, 640–645 RSC;
(b) H. Xie, J. Guo, Y.-Q. Wang, K. Wang, P. Guo, P.-F. Su, X. Wang and X.-Z. Shu, Radical Dehydroxylative Alkylation of Tertiary Alcohols by Ti Catalysis, J. Am. Chem. Soc., 2020, 142, 16787–16794 CrossRef CAS PubMed;
(c) Z. Dong and D. W. C. MacMillan, Metallaphotoredox-enabled deoxygenative arylation of alcohols, Nature, 2021, 598, 451–456 CrossRef CAS PubMed;
(d) Q. Lin, G. Ma and H. Gong, Ni-Catalyzed Formal Cross-Electrophile Coupling of Alcohols with Aryl Halides, ACS Catal., 2021, 11, 14102–14109 CrossRef CAS;
(e) B. K. Chi, J. K. Widness, M. M. Gilbert, D. C. Salgueiro, K. J. Garcia and D. J. Weix, In-Situ Bromination Enables Formal Cross-Electrophile Coupling of Alcohols with Aryl and Alkenyl Halides, ACS Catal., 2022, 12, 580–586 CrossRef CAS PubMed;
(f) T. Suga, Y. Takahashi, C. Miki and Y. Ukaji, Direct and Unified Access to Carbon Radicals from Aliphatic Alcohols by Cost-Efficient Titanium-Mediated Homolytic C−OH Bond Cleavage, Angew. Chem., Int. Ed., 2022, 61, e202112533 CrossRef CAS PubMed;
(g) H. A. Sakai and D. W. C. MacMillan, Nontraditional Fragment Couplings of Alcohols and Carboxylic Acids: C(sp3)–C(sp3) Cross-Coupling via Radical Sorting, J. Am. Chem. Soc., 2022, 144, 6185–6192 CrossRef CAS PubMed.
-
(a) D.-G. Yu, X. Wang, R.-Y. Zhu, S. Luo, X.-B. Zhang, B.-Q. Wang, L. Wang and Z.-J. Shi, Direct Arylation/Alkylation/Magnesiation of Benzyl Alcohols in the Presence of Grignard Reagents via Ni-, Fe-, or Co-Catalyzed sp3 C–O Bond Activation, J. Am. Chem. Soc., 2012, 134, 14638 CrossRef CAS PubMed;
(b) Z.-C. Cao, D.-G. Yu, R.-Y. Zhu, J.-B. Wei and Z.-J. Shi, Direct cross-coupling of benzyl alcohols to construct diarylmethanes via palladium catalysis, Chem. Commun., 2015, 51, 2683 RSC;
(c) S. Akkarasamiyo, J. Margalef and J. S. M. Samec, Nickel-Catalyzed
Suzuki-Miyaura Cross-Coupling Reaction of Naphthyl and Quinolyl Alcohols with Boronic Acids, Org. Lett., 2019, 21, 4782 CrossRef CAS PubMed.
-
(a) T. Suga and Y. Ukaji, Nickel-Catalyzed Cross-Electrophile Coupling between Benzyl Alcohols and Aryl Halides Assisted by Titanium Co-reductant, Org. Lett., 2018, 20, 7846 CrossRef CAS PubMed;
(b) T. Suga, Y. Takahashi and Y. Ukaji, One-Shot Radical Cross Coupling Between Benzyl Alcohols and Alkenyl Halides Using Ni/Ti System, Adv. Synth. Catal., 2020, 362, 5622 CrossRef CAS.
-
(a) P. Guo, K. Wang, W.-J. Jin, H. Xie, L. Qi, X.-Y. Liu and X.-Z. Shu, Dynamic Kinetic Cross-Electrophile Arylation of Benzyl Alcohols by Nickel Catalysis, J. Am. Chem. Soc., 2021, 143, 513 CrossRef CAS PubMed;
(b) W.-Y. Ma, G.-Y. Han, S. Kang, X. Pang, X.-Y. Liu and X.-Z. Shu, Cobalt-Catalyzed Enantiospecific Dynamic Kinetic Cross-Electrophile Vinylation of Allylic Alcohols with Vinyl Triflates, J. Am. Chem. Soc., 2021, 143, 15930–15935 CrossRef CAS PubMed;
(c) X.-B. Yan, C.-L. Li, W.-J. Jin, P. Guo and X.-Z. Shu, Reductive coupling of benzyl oxalates with highly functionalized alkyl bromides by nickel catalysis, Chem. Sci., 2018, 9, 4529 RSC.
- Selected reviews on cross-electrophile coupling:
(a) D. J. Weix, Methods and Mechanisms for Cross-Electrophile Coupling of Csp2 Halides with Alkyl Electrophiles, Acc. Chem. Res., 2015, 48, 1767 CrossRef CAS PubMed;
(b) J. Liu, Y. Ye, J. L. Sessler and H. Gong, Cross-Electrophile Couplings of Activated and Sterically Hindered Halides and Alcohol Derivatives, Acc. Chem. Res., 2020, 53, 1833 CrossRef CAS PubMed;
(c) K. E. Poremba, S. E. Dibrell and S. E. Reisman, Nickel-Catalyzed Enantioselective Reductive Cross-Coupling Reactions, ACS Catal., 2020, 10, 8237 CrossRef CAS PubMed;
(d) X. Pang, P.-F. Su and X.-Z. Shu, Reductive Cross-Coupling of Unreactive Electrophiles, Acc. Chem. Res., 2022, 55, 2491 CrossRef CAS PubMed.
- For a review on cross-electrophile alkenylation reactions for the production of alkenes, see: X. Pang, X. Peng and X.-Z. Shu, Reductive Cross-Coupling of Vinyl Electrophiles, Synthesis, 2020, 3751–3763 CAS.
- Selected examples of reductive coupling between benzyl (pseudo)halides and alkenyl halides:
(a) V. Krasovskaya, A. Krasovskiy, A. Bhattacharjya and B. H. Lipshutz, “On water” sp3–sp2 cross-couplings between benzylic and alkenyl halides, Chem. Commun., 2011, 47, 5717–5719 RSC;
(b) A. H. Cherney and S. E. Reisman, Nickel-Catalyzed Asymmetric Reductive Cross-Coupling Between Vinyl and Benzyl Electrophiles, J. Am. Chem. Soc., 2014, 136, 14365–14368 CrossRef CAS PubMed;
(c) L. K. G. Ackerman, L. L. Anka-Lufford, M. Naodovic and D. J. Weix, Cobalt co-catalysis for cross-electrophile coupling: diarylmethanes from benzyl mesylates and aryl halides, Chem. Sci., 2015, 6, 1115–1119 RSC;
(d) Y. Cai, A. D. Benischke, P. Knochel and C. Gosmini, Cobalt-Catalyzed Reductive Cross-Coupling Between Styryl and Benzyl Halides, Chem. – Eur. J., 2017, 23, 250–253 CrossRef CAS PubMed;
(e) J. L. Hofstra, A. H. Cherney, C. M. Ordner and S. E. Reisman, Synthesis of Enantioenriched Allylic Silanes via Nickel-Catalyzed Reductive Cross-Coupling, J. Am. Chem. Soc., 2018, 140, 139–142 CrossRef CAS PubMed.
- P.-Z. Wang, J.-R. Chen and W.-J. Xiao, Hantzsch esters: an emerging versatile class of reagents in photoredox catalyzed organic synthesis, Org. Biomol. Chem., 2019, 17, 6936 RSC.
- C. Chatalova-Sazepin, Q. Wang, G. M. Sammis and J. Zhu, Copper-Catalyzed Intermolecular Carboetherification of Unactivated Alkenes by Alkyl Nitriles and Alcohols, Angew. Chem., Int. Ed., 2015, 54, 5443 CrossRef CAS PubMed.
- Selected reports on radical oxidative addition:
(a) T. T. Tsou and J. K. Kochi, Mechanism of Oxidative Addition. Reaction of Nickel(0) Complexes with Aromatic Halides, J. Am. Chem. Soc., 1979, 101, 6319 CrossRef CAS. For related elegant work, see:
(b) R. Kehoe, M. Mahadevan, A. Manzoor, G. McMurray, P. Wienefeld and M. C. Baird, Reactions of the Ni(0) Compound Ni(PPh3)4 with Unactivated Alkyl Halides: Oxidative Addition Reactions Involving Radical Processes and Nickel(I) Intermediates, Organometallics, 2018, 37, 2450 CrossRef CAS.
-
(a) O. Gutierrez, J. C. Tellis, D. N. Primer, G. A. Molander and M. C. Kozlowski, Nickel-Catalyzed Cross-Coupling of Photoredox-Generated Radicals: Uncovering a General Manifold for Stereoconvergence in Nickel-Catalyzed Cross-Couplings, J. Am. Chem. Soc., 2015, 137, 4896 CrossRef CAS PubMed;
(b) Z.-X. Tian, J.-B. Qiao, G.-L. Xu, X. Pang, L. Qi, W.-Y. Ma, Z. Zhao, J. Duan, Y.-F. Du, P. Su, X.-Y. Liu and X.-Z. Shu, Highly Enantioselective Cross-Electrophile Aryl-Alkenylation of Unactivated Alkenes, J. Am. Chem. Soc., 2019, 141, 7637 CrossRef CAS PubMed.
Footnote |
† Electronic supplementary information (ESI) available: Detailed optimization of reaction conditions, part of the mechanistic studies, detailed experimental procedures, characterization data, and copies of 1H and 13C NMR spectra of new compounds. See DOI: https://doi.org/10.1039/d3qo01416a |
|
This journal is © the Partner Organisations 2024 |
Click here to see how this site uses Cookies. View our privacy policy here.