DOI:
10.1039/D4RA03939D
(Paper)
RSC Adv., 2024,
14, 28332-28339
Metal-free approach for imidazole synthesis via one-pot N-α-C(sp3)–H bond functionalization of benzylamines†
Received
28th May 2024
, Accepted 11th August 2024
First published on 5th September 2024
Abstract
A metal-free one-pot method is established for the synthesis of tetrasubstituted imidazoles from the reaction of arylmethylamines and 1,2-dicarbonyls/benzoin. The N-α-C(sp3)–H bond functionalization of arylmethylamines using a catalytic amount of AcOH afforded polysubstituted imidazoles under aerobic conditions in significant yields of up to 95%.
Introduction
The ever-growing influence of nitrogen heterocycles in pharmaceuticals has positioned these heterocycles in drug development and medicinal chemistry as key therapeutic agents.1–4 In particular, azole heterocycles have significant representation in different biological applications.5 Especially, five-membered imidazoles have a wide diversity of pharmaceutical importance.6–11 In addition, imidazole-based drug molecules such as apoptozole, eprosartan, losartan and olmesartan have attracted the attention of chemists for designing bioactive molecules (Fig. 1).12–15 Furthermore, imidazole heterocycles have demonstrated various biological activities such as antifungal,16 antitumor,17 analgesic,18 antibacterial,19 anthelmintic,20 anti-tuberculosis,21 and anti-inflammatory.22 Zeolite imidazole frameworks act as prominent materials and are exposed to potential applications such as host–guest chemistry, catalysis, luminescence, gas separation, gas sorption and magnetism.23,24 Moreover, they have anion and/or cation selectively functioning in different applications in asymmetric catalysis.25,26 In addition, these imidazoles have significant photophysical properties.27,28
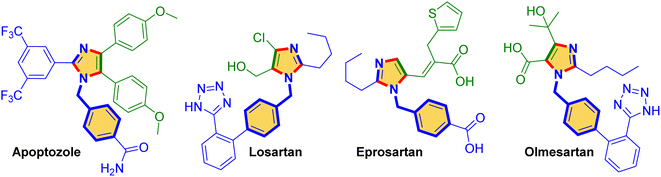 |
| Fig. 1 Imidazole containing drug molecules. | |
The traditional method for the synthesis of imidazoles is carried out through condensation of carbonyl molecules with ammonium salts. Different substrates such as aldehydes, nitriles, imines, amides, isocyanides, amidines, amino acids and benzylamines are used for the synthesis of trisubstituted imidazoles.29–34 Unfortunately, there are limited reports on tetrasubstituted imidazole synthesis. In particular, aldehyde, benzil, amine and NH4OAc with acid catalysts were used for the synthesis of tetrasubstituted imidazole.35,36 Later, the condensation of amidine and α-halo ketones was developed for tetrasubstituted imidazole synthesis using IBX as a strong oxidant.37 Subsequently, Kevin Nguyen et al. demonstrated the synthesis of tetrasubstituted imidazoles from CF3CO2NH4 and N-(2-oxo)-amide substrates at higher temperatures of up to 150 °C for the reaction.38 Next, the sulphur-catalyzed cyclization reaction between ketones and aliphatic amines with a reaction time of up to 36 h was reported for tetrasubstituted imidazole synthesis.39 Moreover, there are some methods for the synthesis of polysubstituted imidazoles constructed from benzils/benzoins and arylmethylamines using catalysts such as Mo-ZnIn2S4 photocatalysts,40 NiCl2·6H2O/Ni(OAc)2·4H2O41 and Ag2CO3.42 Earlier, 2,4,5-trisubstituted and tetrasubstituted imidazole synthesis was achieved using CuI and FeCl3 catalysts.34,43,44 Biswadip Banerji et al. synthesized a series of tetrasubstituted imidazoles using excess K2CO3 (3.0 equiv.) as an additive and a I2 catalyst.45 As a result of a few shortcomings, such as the use of excess catalyst as well as the tedious process for catalyst design and product separation, the progress of a competent protocol is greatly providential for the synthesis of polysubstituted imidazoles.
In recent years, the utility of benzylic α-(sp3)C–N bond functionalization has led to one of the most attractive strategies for the construction of complex molecules for preclinical research and discovery.46 Moreover, amines play a crucial role in N-heterocycle design as a key building block.43,44,47–52 Herein, the AcOH-catalysed synthesis of tetrasubstituted imidazoles from benzylamines and 1,2-dicarbonyl/benzoin substrates using molecular oxygen as an oxidant was developed.
Results and discussion
At the outset, the reaction optimization commenced with benzylamine 1 (2.1 mmol) and benzil 2a (1.0 mmol) substrates in the presence of AcOH (30 mol%) under air oxygen (O2) (Table 1). Initially, different polar solvents such as BuOH, EtOH and MeOH were examined considering green chemistry principles in which the tetrasubstituted imidazole product 3a was obtained in 78%, 65% and 55% yields (Table 1, entries 1–3). From these results, further study was carried out with polar aprotic solvents such as CH3CN, 1,4-dioxane, ethyl acetate, DMF and DMSO where the anticipated product 3a was accomplished in yields of 60%, 62%, 15%, 75% and 72%, respectively (Table 1, entries 4–8). The reaction was further performed using non-polar solvents (such as toluene, o-xylene, chlorobenzene and 1,2-dichlorobenzene), affording lower yields of the desired product (Table 1, entries 9–12). After screening of polar and non-polar solvents, the reaction was examined at 140 °C under solvent-free conditions where the formation of product 3a was observed to be significant up to 91% of yield (Table 1, entry 13). Then, the same product 3a was furnished in lower yields when the temperature study was carried out at 120 °C and 100 °C (Table 1, entries 14–15). Furthermore, the reaction investigation was performed with catalyst loadings such as 20 mol% and 15 mol% of AcOH under solvent-free conditions, in which product 3a was obtained in slightly lower yields of 88% and 85%, respectively (Table 1, entries 16–17). At last, the product furnished up to 69% yield under anaerobic conditions, whereas the trace amount of the product obtained under N2 conditions recognized the role of air oxygen in the reaction for the product formation (Table 1, entries 18–19). Finally, the best-optimized conditions for the formation of product 3a under solvent-free conditions are benzylamine 1 (2.1 mmol) and benzil 2a (1.0 mmol), AcOH (30 mol%) under air O2 at 140 °C.
Table 1 Optimization of reaction conditionsa
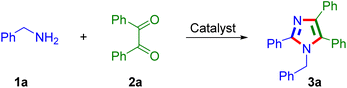
|
Entry |
Solvent |
Temp (°C) |
Time (h) |
Yieldb (%) |
Reaction conditions: 1a (2.1 mmol), 2a (1.0 mmol), AcOH (30 mol%), and solvent (1 mL) in air O2. Isolated yield. Reaction was carried out at 20 mol%. Reaction was carried out at 15 mol%. Reaction was carried out without air. Under an N2 atmosphere. |
1 |
BuOH |
100 |
8 |
78 |
2 |
EtOH |
80 |
8 |
65 |
3 |
MeOH |
60 |
8 |
55 |
4 |
CH3CN |
80 |
8 |
60 |
5 |
1,4-Dioxane |
100 |
8 |
62 |
6 |
Ethyl acetate |
78 |
8 |
15 |
7 |
DMF |
140 |
8 |
75 |
8 |
DMSO |
140 |
8 |
72 |
9 |
Toluene |
110 |
8 |
30 |
10 |
o-Xylene |
130 |
8 |
56 |
11 |
Chlorobenzene |
130 |
8 |
52 |
12 |
1,2-Dichlorobenzene |
140 |
8 |
35 |
13 |
Neat |
140 |
3 |
91 |
14 |
Neat |
120 |
4 |
80 |
15 |
Neat |
100 |
4 |
69 |
16c |
Neat |
140 |
4 |
88 |
17d |
Neat |
140 |
4 |
85 |
18e |
Neat |
140 |
4 |
69 |
19f |
Neat |
140 |
4 |
Trace |
By using the optimized conditions, the finding of the reaction scope was broadly explored with substituted benzylamines and 1,2-dicarbonyls for the synthesis of polysubstituted imidazoles (Table 2). Initially, the reaction of benzil 2a was studied with para-substituted benzylamies of electron-donating and electron-withdrawing functional groups, where the products 3a, 3b, 3c, 3d and 3e were obtained in the yields of 91%, 95%, 94%, 84% and 89%, respectively. Then, ortho-substituted benzyalmines were examined with benzil 2a and afforded products 3f (87%), 3g (86%) and 3h (82%) in excellent yields. After that, the disubstituted arylmethylamines were used to prepare tetrasubstituted products that were achieved in yields of 3i (85%) and 3j (88%). Also, the optimized conditions with heteroaryl benzylamines were found feasible for the synthesis of imidazoles as 3k (83%), 3l (81%) and 3m (86%). Additionally, the substrate scope was further explored with substituted arylmethylamines using 9,10-phenanthrenequinone 2b where the analogous products formed (3n: 83%, 3o: 85% and 3p: 86%) were in excellent yields. After that, heteroaryl methylamines were screened with 9,10-phenanthrenequinone 2b and products were obtained (3q: 80% and 3r: 87%) in significant yields (Table 2). There are a number of methods available for the synthesis of imidazoles using 1,2-diketones (benzil) but not from α-hydroxy ketone. Due to this, the optimized reaction conditions were tested against α-hydroxy ketones (benzoin) with different substituted arylmethylamines for the synthesis of imidazole, achieved in good to excellent yields as illustrated in Table 3. Here, the improved method has the utility to synthesize these polyfunctionalized imidazoles from both α-hydroxy ketone (benzoin) and 1,2-diketones (benzil) substrates by reacting with different arylmethylamines.
Table 2 Substrate scope of arylmethylamines with 1,2-dicarbonylsa
Reaction conditions: 1 (2.1 mmol, 2.1 equiv.), 2 (1.0 mmol, 1.0 equiv.), AcOH (30 mol%), open air at 140 °C under neat condition for 3–6 h. |
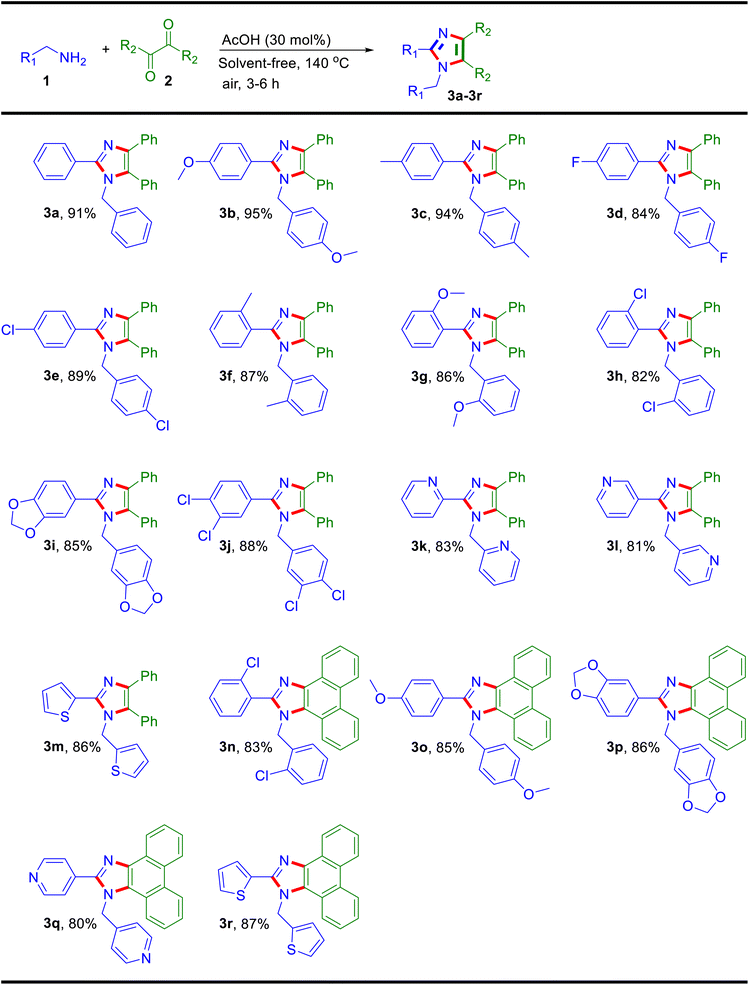 |
Table 3 Substrate scope of arylmethylamines with benzoina
Reaction conditions: 1 (2.1 mmol, 2.1 equiv.), 2c (1.0 mmol, 1.0 equiv.), AcOH (30 mol%), open air at 140 °C under neat condition for 3–6 h. |
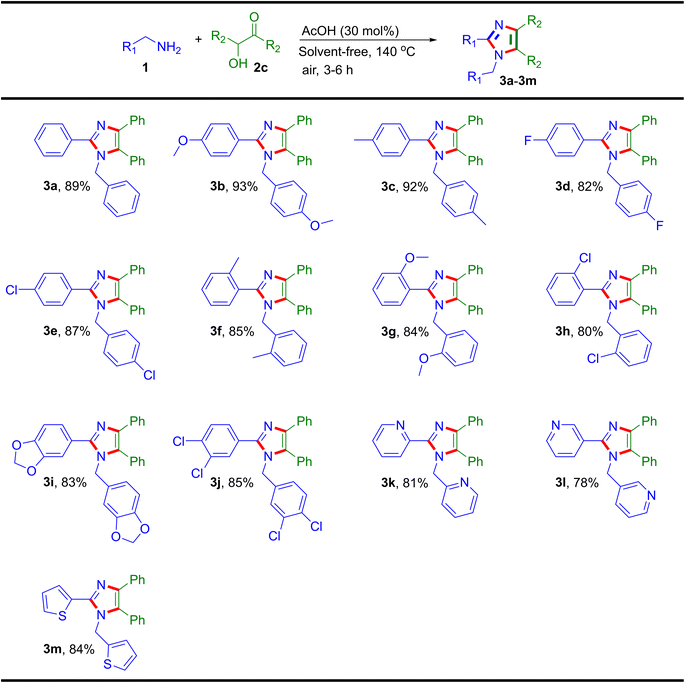 |
The mechanism of the reaction is proposed on the basis of literature and experimental results (Scheme 1).43,44,47 At first, the condensation of 1,2-diketone 2 with arylmethylamine 1 takes place, which gives intermediate I in the presence of a catalytic amount of AcOH. On the other hand, α-hydroxy ketone (benzoin) 2c and arylmethylamine 1 condensation leads to the formation of intermediate IV, which is further converted into intermediate V to produce a similar intermediate I in the presence of an AcOH catalyst under aerobic conditions. Afterward, the 1,5-H shift of intermediate I produces intermediate II, which further cyclizes into III in the presence of AcOH. Lastly, the aerobic oxidation of intermediate III affords the anticipated product 3.51,53
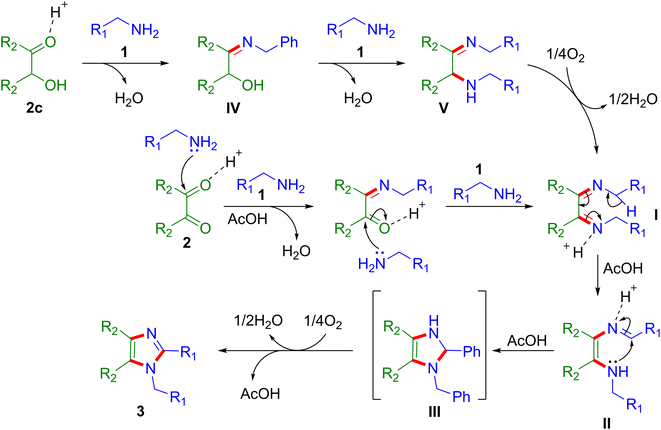 |
| Scheme 1 The possible reaction mechanism. | |
Conclusions
In conclusion, the facile and convenient metal-free approach demonstrated the synthesis of polyfunctionalized imidazoles from simple starting materials. The formation of all products was achieved with good functional group tolerance, leading to further investigation towards designing different organic scaffolds.
Experimental section
General information
The distilled solvents were used for performing reactions. The physical constants were recorded using the Buchi melting point apparatus and are uncorrected. By using silica gel plates (0.25 mm), thin-layer chromatography (TLC) was performed to study the reaction progress under a UV lamp. The synthesised compounds were analysed by NMR (1H NMR at 400 MHz and 13C NMR at 100 MHz) on a JEOL NMR spectrometer and mass on 6530 Accurate-Mass Q-TOF LC/MS of Agilent Technologies.
General procedure for synthesis of tetrasubstituted imidazoles (3)
Arylmethylamine 1 (2.1 mmol), 1,2-diketone/benzoin 2 (1.0 mmol), and AcOH (30 mol%) were stirred at 140 °C temperature under solvent-free aerobic conditions in a 25 mL round bottom flask. The progress of the reaction was monitored by thin-layer chromatography (TLC). After completion of the reaction, the reaction mixture was cooled and poured into crushed ice. After that, the mixture was stirred and filtered to obtain the crude imidazole product 3. The purified imidazole product 3 was achieved by column chromatography using hexane/ethyl acetate as eluent.
Analysis data of the synthesised tetrasubstituted imidazoles
1-Benzyl-2,4,5-triphenyl-1H-imidazole (3a). M.P.: 165–166 °C; (Lit.43 163–166 °C); white solid; 1H-NMR (400 MHz, DMSO-D6) δ 7.62 (d, J = 4.3 Hz, 2H), 7.41 (q, J = 6.7 Hz, 8H), 7.27–7.14 (m, 8H), 6.72 (d, J = 6.7 Hz, 2H), 5.13 (s, 2H) ppm; 13C-NMR (400 MHz, DMSO-D6) δ 147.6, 137.8, 137.4, 135.1, 131.4, 131.1, 130.7, 129.5, 129.4, 129.1, 128.6, 127.7, 126.8, 126.6, 126.2, 48.2 ppm.
1-(4-Methoxybenzyl)-2-(4-methoxyphenyl)-4,5-diphenyl-1H-imidazole (3b). M.P.: 155–157 °C; (Lit.43 153–155 °C); off-white solid; 1H-NMR (400 MHz, CDCl3): δ 1H-NMR (400 MHz, DMSO-D6) δ 7.55 (d, J = 8.6 Hz, 2H), 7.41–7.37 (m, 5H), 7.25 (d, J = 3.7 Hz, 2H), 7.16 (t, J = 7.6 Hz, 2H), 7.08 (t, J = 7.3 Hz, 1H), 6.97 (d, J = 8.6 Hz, 2H), 6.72 (d, J = 8.6 Hz, 2H), 6.62 (d, J = 8.6 Hz, 2H), 5.02 (s, 2H), 3.75 (s, 3H), 3.62 (s, 3H) ppm; 13C-NMR (400 MHz, DMSO-D6) δ 160.1, 158.8, 147.5, 137.1, 135.2, 131.4, 130.5, 130.3, 129.8, 129.4, 129.3, 128.6, 127.4, 126.6, 123.7, 114.6, 114.5, 55.8, 55.5, 47.6 ppm.
1-(4-Methylbenzyl)-4,5-diphenyl-2-p-tolyl-1H-imidazole (3c). M.P.: 131–134 °C (Lit.43 132–134 °C); white solid; 1H-NMR (400 MHz, DMSO-D6) δ 7.51 (d, J = 7.9 Hz, 2H), 7.42–7.38 (m, 5H), 7.26–7.15 (m, 6H), 7.09 (t, J = 7.0 Hz, 1H), 6.97 (d, J = 7.3 Hz, 2H), 6.61 (d, J = 7.9 Hz, 2H), 5.06 (s, 2H), 2.29 (s, 3H), 2.16 (s, 3H) ppm; 13C-NMR (100 MHz, DMSO-D6) δ 147.6, 138.8, 137.3, 136.8, 135.2, 135.0, 131.4, 131.2, 130.6, 129.7, 129.6, 129.4, 129.3, 128.9, 128.6, 128.5, 126.7, 126.6, 126.0, 47.9, 21.4, 21.1 ppm.
1-(4-Fluorobenzyl)-2-(4-fluorophenyl)-4,5-diphenyl-1H-imidazole (3d). M.P.: 161–163 °C (Lit.43 159–162 °C); white solid; 1H-NMR (400 MHz, DMSO-D6) δ 7.68–7.64 (m, 2H), 7.41 (d, J = 9.2 Hz, 5H), 7.26 (t, J = 8.6 Hz, 4H), 7.17 (t, J = 7.6 Hz, 2H), 7.10 (t, J = 7.0 Hz, 1H), 6.97 (t, J = 8.6 Hz, 2H), 6.73 (t, J = 6.4 Hz, 2H), 5.08 (s, 2H) ppm; 13C-NMR (100 MHz, DMSO-D6) δ 164.1, 162.9, 161.6, 160.5, 146.7, 137.4, 134.9, 133.8, 131.4, 131.3, 131.0, 130.7, 129.5, 129.5, 128.6, 128.4, 128.3, 127.8, 127.8, 126.8, 126.6, 116.3, 116.0, 116.0, 115.8, 47.6 ppm.
1-(4-Chlorobenzyl)-2-(4-chlorophenyl)-4,5-diphenyl-1H-imidazole (3e). M.P.:158–160 °C (Lit.43 159–162 °C); white solid; 1H-NMR (400 MHz, DMSO-D6) δ 7.64 (d, J = 7.9 Hz, 2H), 7.48 (d, J = 8.6 Hz, 2H), 7.43–7.39 (m, 5H), 7.28 (d, J = 3.7 Hz, 1H), 7.23–7.16 (m, 4H), 7.11 (t, J = 7.3 Hz, 1H), 6.75 (d, J = 8.6 Hz, 2H), 5.11 (s, 2H) ppm; 13C-NMR (100 MHz, DMSO-D6) δ 146.4, 137.7, 136.6, 134.8, 134.2, 132.3, 131.3, 131.0, 130.9, 130.7, 130.0, 129.6, 129.3, 129.1, 128.6, 128.1, 126.9, 126.6, 47.7 ppm.
1-(2-Methylbenzyl)-2-(2-methylphenyl)-4,5-diphenyl-1H-imidazole (3f). M.P.: 178–180 °C; brown solid; 1H-NMR (400 MHz, DMSO-D6) δ 7.42 (d, J = 7.3 Hz, 2H), 7.37–7.33 (m, 4H), 7.29–7.25 (m, 4H), 7.17 (t, J = 7.3 Hz, 3H), 7.09 (t, J = 7.3 Hz, 1H), 7.03–6.96 (m, 2H), 6.88 (d, J = 7.3 Hz, 1H), 6.47 (d, J = 6.7 Hz, 1H), 4.84 (s, 2H), 2.21 (s, 3H), 1.75 (s, 3H) ppm; 13C-NMR (100 MHz, DMSO-D6) δ 147.4, 138.4, 136.9, 135.9, 135.2, 134.5, 131.2, 130.9, 130.5, 130.2, 129.7, 129.4, 129.2, 128.6, 127.4, 126.7, 126.5, 126.1, 125.8, 45.5, 20.0, 18.7 ppm; HRMS: m/z [M + H]+ calcd for C30H26N2: 415.2096; found: 415.2182.
1-(2-Methoxybenzyl)-2-(2-methoxyphenyl)-4,5-diphenyl-1H-imidazole (3g). M.P.: 135–136 °C; (Lit.43 132–134 °C); yellow solid; 1H-NMR (400 MHz, DMSO-D6) δ 7.37 (d, J = 12.2 Hz, 7H), 7.21–6.94 (m, 8H), 6.69 (d, J = 6.7 Hz, 2H), 6.40 (d, J = 6.1 Hz, 1H), 4.82 (s, 2H), 3.71 (s, 3H), 3.48 (s, 3H) ppm; 13C-NMR (100 MHz, DMSO-D6) δ 157.6, 156.0, 145.8, 137.1, 135.3, 132.6, 131.4, 131.4, 131.2, 129.9, 129.4, 129.1, 128.7, 128.6, 127.0, 126.5, 125.5, 120.9, 120.6, 112.0, 110.8, 55.9, 55.7, 43.1 ppm.
1-(2-Chlorobenzyl)-2-(2-chlorophenyl)-4,5-diphenyl-1H-imidazole (3h). M.P.: 143–145 °C (Lit.43 142–144 °C); white solid; 1H-NMR (400 MHz, DMSO-D6) δ 7.55 (d, J = 7.9 Hz, 1H), 7.50 (d, J = 7.3 Hz, 1H), 7.46–7.33 (m, 6H), 7.28 (d, J = 6.7 Hz, 2H), 7.20–7.09 (m, 7H), 6.64 (d, J = 7.3 Hz, 1H), 4.95 (s, 2H) ppm; 13C-NMR (100 MHz, DMSO-D6) δ 145.2, 137.3, 134.8, 134.3, 134.0, 133.0, 131.9, 131.2, 131.2, 130.7, 130.3, 130.2, 130.0, 129.6, 129.5, 128.7, 128.2, 127.9, 127.8, 126.9, 126.5, 45.8 ppm.
2-(Benzo[d][1,3]dioxol-5-yl)-1-(benzo[d][1,3]dioxol-5-ylmethyl)-4,5-diphenyl-1H-imidazole (3i). M.P.: 178–180 °C (Lit.43 175–177 °C); white solid; 1H-NMR (400 MHz, DMSO-D6) δ 7.44–7.34 (m, 6H), 7.29–7.24 (m, 2H), 7.18–7.14 (m, 3H), 7.10 (t, J = 7.0 Hz, 1H), 6.96 (d, J = 7.9 Hz, 1H), 6.69 (d, J = 7.9 Hz, 1H), 6.24 (s, 1H), 6.13 (d, J = 7.9 Hz, 1H), 6.04 (s, 2H), 5.89 (s, 2H), 4.99 (s, 2H) ppm; 13C-NMR (100 MHz, DMSO-D6) δ 148.3, 147.9, 147.3, 146.8, 137.1, 135.1, 131.7, 131.3, 131.2, 130.4, 129.5, 129.4, 128.7, 128.6, 126.7, 126.6, 125.1, 123.2, 119.5, 109.4, 109.0, 108.7, 106.7, 101.9, 101.6, 47.9 ppm.
1-(3,4-Dichlorobenzyl)-2-(3,4-dichlorophenyl)-4,5-diphenyl-1H-imidazole (3j). M.P.: 160–163 °C (Lit.43 158–161 °C); white solid; 1H-NMR (400 MHz, DMSO-D6) δ 7.87 (d, J = 1.2 Hz, 1H), 7.69 (d, J = 8.6 Hz, 1H), 7.59 (dd, J = 7.9, 1.8 Hz, 1H), 7.43–7.41 (m, 6H), 7.28 (d, J = 3.7 Hz, 2H), 7.18 (t, J = 7.3 Hz, 2H), 7.12 (t, J = 7.3 Hz, 1H), 6.98 (s, 1H), 6.70 (d, J = 8.6 Hz, 1H), 5.14 (s, 2H) ppm; 13C-NMR (100 MHz, DMSO-D6) δ 145.1, 138.5, 137.9, 134.6, 132.3, 132.0, 131.7, 131.5, 131.4, 131.3, 130.8, 130.6, 130.5, 129.7, 129.7, 129.0, 128.7, 128.7, 127.1, 126.7, 47.5 ppm.
2-(4,5-Diphenyl-1-(pyridin-2-ylmethyl)-1H-imidazole-2-yl)pyridine (3k). M.P.: 166–168 °C (Lit.43 165–167 °C); light yellow solid; 1H-NMR (400 MHz, DMSO-D6) δ 8.94–8.87 (m, 1H), 8.81 (d, J = 8.6 Hz, 1H), 8.60 (d, J = 7.9 Hz, 1H), 8.45 (d, J = 7.9 Hz, 1H), 8.36 (d, J = 7.3 Hz, 1H), 8.08 (d, J = 7.9 Hz, 1H), 7.81–7.68 (m, 3H), 7.64–7.50 (m, 1H), 7.22–7.17 (m, 2H), 7.05 (d, J = 7.9 Hz, 1H), 6.79–6.72 (m, 1H), 6.46 (d, J = 7.9 Hz, 1H), 6.16–6.11 (m, 1H), 6.09–6.03 (m, 1H), 5.95 (s, 1H), 5.78 (s, 2H) ppm; 13C-NMR (100 MHz, DMSO-D6) δ 152.9, 148.5, 148.1, 147.2, 137.5, 131.4, 130.0, 129.0, 128.3, 127.8, 127.5, 126.8, 126.2, 125.6, 124.3, 124.1, 122.7, 121.8, 121.1, 119.1, 110.0, 109.1, 107.2, 106.6, 102.2, 50.3 ppm.
3-(4,5-Diphenyl-1-(pyridin-3-ylmethyl)-1H-imidazole-2-yl)pyridine (3l). M.P.: 190–192 °C; faint yellow solid; 1H-NMR (400 MHz, DMSO-D6) δ 8.85 (s, 1H), 8.58 (d, J = 3.7 Hz, 1H), 8.30 (d, J = 3.1 Hz, 1H), 8.04 (d, J = 7.9 Hz, 1H), 7.91 (s, 1H), 7.44–7.31 (m, 8H), 7.21–7.11 (m, 5H), 5.14–5.28 (2H) ppm; 13C-NMR (100 MHz, DMSO-D6) δ 150.3, 149.5, 149.1, 147.9, 144.9, 138.0, 136.4, 134.7, 134.2, 133.0, 131.3, 131.3, 130.7, 129.7, 128.7, 127.3, 127.0, 126.7, 124.2, 46.2 ppm; HRMS: m/z [M + H]+ calcd for C26H20N4: 389.1688; found: 389.1774.
4,5-Diphenyl-2-(thiophen-2-yl)-1-(thiophen-2-ylmethyl)-1H-imidazole (3m). M.P.: 170–173 °C (Lit.43 169–171 °C); white solid; 1H-NMR (400 MHz, DMSO-D6) δ 7.62 (d, J = 6.1 Hz, 1H), 7.44–7.36 (m, 10H), 7.17–6.83 (m, 4H), 6.55 (d, J = 5.5 Hz, 1H), 5.36 (s, 2H) ppm; 13C-NMR (100 MHz, DMSO-D6) δ 141.5, 140.0, 137.6, 134.6, 133.1, 131.5, 130.7, 130.5, 129.7, 129.6, 128.7, 128.5, 128.3, 127.6, 127.0, 126.7, 126.5, 126.3, 125.8, 44.1 ppm.
1-(2-Chlorobenzyl)-2-(2-chlorophenyl)-1H-phenanthro[9,10-d]imidazole (3n). M.P.: 185–187 °C; dark brown solid; 1H-NMR (400 MHz, DMSO-D6) δ 8.96–8.91 (m, 1H), 8.85 (d, J = 6.7 Hz, 2H), 8.58 (d, J = 7.9 Hz, 1H), 8.31 (t, J = 7.9 Hz, 1H), 7.87 (d, J = 8.6 Hz, 1H), 7.66 (t, J = 7.9 Hz, 2H), 7.60 (t, J = 6.4 Hz, 2H), 7.47 (t, J = 6.1 Hz, 2H), 7.36–7.29 (m, 1H), 7.23 (t, J = 7.9 Hz, 1H), 7.08 (d, J = 8.6 Hz, 1H), 6.56 (d, J = 7.9 Hz, 1H), 5.72 (s, 2H) ppm; 13C-NMR (100 MHz, DMSO-D6) δ 141.8, 133.9, 131.4, 130.9, 130.6, 129.6, 128.5, 127.8, 127.8, 127.3, 126.9, 126.9, 126.6, 126.2, 126.1, 125.3, 124.8, 124.5, 124.4, 123.5, 123.2, 42.4; HRMS: m/z [M + H]+ calcd for C28H18Cl2N2: 453.0847; found: 453.0925.
1-(4-Methoxybenzyl)-2-(4-methoxyphenyl)-1H-phenanthro[9,10-d]imidazole (3o). M.P.: 123–125 °C (Lit.42 122–124 °C); white solid; 1H-NMR (400 MHz, DMSO-D6) δ 8.87–8.61 (m, 4H), 8.26–8.08 (m, 1H), 7.71–7.47 (m, 5H), 7.06–6.86 (m, 6H), 5.82 (s, 2H), 3.79 (s, 3H), 3.65 (s, 3H) ppm; 13C-NMR (100 MHz, DMSO-D6) δ 158.2, 157.5, 153.9, 137.6, 132.3, 131.2, 129.4, 129.4, 129.3, 129.3, 127.5, 127.5, 127.4, 127.4, 127.2, 127.1, 126.1, 122.5, 115.1, 114.9, 114.9, 114.8, 114.7, 55.9, 55.6, 45.3 ppm; HRMS: m/z [M + H]+ calcd for C30H24N2O2: 445.1838; found: 445.1919.
2-(Benzo[d][1,3]dioxol-5-yl)-1-(benzo[d][1,3]dioxol-5-ylmethyl)-1H-phenanthro[9,10-d]imidazole (3p). M.P.: 270–272 °C; brown solid; 1H-NMR (400 MHz, DMSO-D6) δ 9.48 (d, J = 1.2 Hz, 1H), 8.93 (t, J = 9.5 Hz, 1H), 8.89 (s, 1H), 8.82 (d, J = 8.6 Hz, 1H), 8.78 (d, J = 3.7 Hz, 1H), 8.71 (d, J = 4.3 Hz, 1H), 8.63 (d, J = 7.3 Hz, 1H), 8.48 (d, J = 7.9 Hz, 1H), 8.43–8.38 (m, 1H), 8.11 (d, J = 7.3 Hz, 1H), 7.84–7.71 (m, 3H), 7.68–7.63 (m, 1H), 7.58–7.55 (m, 1H), 7.50 (t, J = 7.3 Hz, 1H), 7.38 (d, J = 7.9 Hz, 1H), 7.27 (dd, J = 7.3, 4.9 Hz, 1H), 6.00 (s, 2H) ppm; 13C-NMR (100 MHz, DMSO-D6) δ 152.4, 151.1, 150.2, 149.4, 148.3, 147.9, 137.4, 134.8, 134.0, 133.1, 128.5, 128.0, 127.9, 127.7, 127.3, 126.5, 126.0, 124.9, 124.6, 124.3, 124.2, 122.9, 121.7, 121.4, 48.6 ppm; HRMS: m/z [M + H]+ calcd for C30H20N2O4: 473.1423; found: 473.1517.
2-(Pyridin-4-yl)-1-(pyridin-4-ylmethyl)-1H-phenanthro[9,10-d]imidazole (3q). M.P.: 210–212 °C; brown solid; 1H-NMR (400 MHz, DMSO-D6) δ 8.90 (t, J = 9.5 Hz, 2H), 8.36 (d, J = 7.3 Hz, 2H), 8.20 (d, J = 6.7 Hz, 2H), 7.76–7.70 (m, 9H), 7.01 (s, 2H) ppm; 13C-NMR (100 MHz, DMSO-D6) δ 146.9, 144.4, 142.0, 134.1, 133.6, 129.1, 128.9, 128.8, 128.4, 128.3, 127.2, 127.1, 126.8, 125.9, 125.5, 125.0, 124.8, 124.6, 122.7, 120.9, 120.8, 118.1, 45.6 ppm; HRMS: m/z [M + H]+ calcd for C26H18N4: 387.1531; found: 387.1625.
2-(Thiophen-2-yl)-1-(thiophen-2-ylmethyl)-1H-phenanthro[9,10-d]imidazole (3r). M.P.: 258–262 °C; brown solid; 1H-NMR (400 MHz, DMSO-D6) δ 8.95–8.89 (m, 1H), 8.81 (d, J = 8.6 Hz, 1H), 8.57 (d, J = 7.9 Hz, 1H), 8.31–8.26 (m, 1H), 7.80 (d, J = 4.3 Hz, 1H), 7.77–7.68 (m, 1H), 7.65 (d, J = 7.3 Hz, 1H), 7.62–7.55 (m, 3H), 7.45 (t, J = 5.2 Hz, 1H), 7.21 (t, J = 4.3 Hz, 1H), 6.97 (t, J = 4.0 Hz, 2H), 6.21 (s, 2H) ppm; 13C-NMR (100 MHz, DMSO-D6) δ 140.2, 139.1, 129.9, 129.0, 128.8, 128.3, 128.2, 128.1, 128.0, 127.7, 127.2, 126.9, 126.5, 126.5, 125.9, 125.7, 125.0, 124.1, 122.9, 122.8, 122.5, 121.8, 121.1, 47.3 ppm; HRMS: m/z [M + H]+ calcd for C24H16N2S2: 397.0755; found: 397.0839.
Data availability
The data supporting this article have been included as part of the ESI.†
Conflicts of interest
There are no conflicts to declare.
References
- E. Vitaku, D. T. Smith and J. T. Njardarson, J. Med. Chem., 2014, 57, 10257–10274 CrossRef CAS PubMed.
- N. Kerru, L. Gummidi, S. Maddila, K. K. Gangu and S. B. Jonnalagadda, Molecules, 2020, 25, 1909 CrossRef CAS PubMed.
- J. Jampilek, Molecules, 2019, 24, 3839 CrossRef CAS PubMed.
- M. M. Heravi and V. Zadsirjan, RSC Adv., 2020, 10, 44247–44311 RSC.
- A. K. Kabi, S. Sravani, R. Gujjarappa, A. Garg, N. Vodnala, U. Tyagi, D. Kaldhi, R. Velayutham, S. Gupta and C. C. Malakar, in Materials Horizons: From Nature to Nanomaterials, 2022, pp. 79–99 Search PubMed.
- L. De Luca, Curr. Med. Chem., 2006, 13, 1–23 CAS.
- S. Rulhania, S. Kumar, B. Nehra, G. D. Gupta and V. Monga, J. Mol. Struct., 2021, 1232, 129982 CrossRef CAS.
- S. S. Alghamdi, R. S. Suliman, K. Almutairi, K. Kahtani and D. Aljatli, Drug Des., Dev. Ther., 2021, 15, 3289–3312 CrossRef PubMed.
- P. N. Chopra and J. K. Sahu, Curr. Drug Discovery Technol., 2020, 17, 574–584 CrossRef CAS PubMed.
- L. Zhang, X. M. Peng, G. L. V. Damu, R. X. Geng and C. H. Zhou, Med. Res. Rev., 2014, 34, 340–437 CrossRef CAS PubMed.
- M. Gaba and C. Mohan, Med. Chem. Res., 2016, 25, 173–210 CrossRef CAS.
- L. E. Evans, M. D. Cheeseman, N. Yahya and K. Jones, PLoS One, 2015, 10, e0140006 CrossRef PubMed.
- G. L. Plosker, Drugs, 2009, 69, 2477–2499 CrossRef CAS PubMed.
- A. R. A. Al-Majed, E. Assiri, N. Y. Khalil and H. A. Abdel-Aziz, in Profiles of Drug Substances, Excipients and Related Methodology, Academic Press, 2015, vol. 40, pp. 159–194 Search PubMed.
- G. Kourlaba, F. Gialama, K. Tsioufis and N. Maniadakis, Int. J. Cardiol., 2016, 221, 60–74 CrossRef CAS PubMed.
- N. Rani, A. Sharma, G. K. Gupta and R. Singh, Mini-Rev. Med. Chem., 2013, 13, 1626–1655 CrossRef CAS PubMed.
- L. Wang, K. W. Woods, Q. Li, K. J. Barr, R. W. McCroskey, S. M. Hannick, L. Gherke, R. B. Credo, Y. H. Hui, K. Marsh, R. Warner, J. Y. Lee, N. Zielinski-Mozng, D. Frost, S. H. Rosenberg and H. L. Sham, J. Med. Chem., 2002, 45, 1697–1711 CrossRef CAS PubMed.
- Ü. Uçucu, N. G. Karaburun and I. Işikdag, Farmaco, 2001, 56, 285–290 CrossRef.
- M. Antolini, A. Bozzoli, C. Ghiron, G. Kennedy, T. Rossi and A. Ursini, Bioorg. Med. Chem. Lett., 1999, 9, 1023–1028 CrossRef CAS PubMed.
- J. A. Rocha, I. M. Andrade, L. M. C. Véras, P. V. Quelemes, D. F. Lima, M. J. S. Soares, P. L. S. Pinto, S. J. Mayo, G. Ivanova, M. Rangel, M. Correia, A. C. Mafud, Y. P. Mascarenhas, C. Delerue-Matos, J. de Moraes, P. Eaton and J. R. S. A. Leite, Phytother. Res., 2017, 31, 624–630 CrossRef CAS PubMed.
- P. Gupta, S. Hameed and R. Jain, Eur. J. Med. Chem., 2004, 39, 805–814 CrossRef CAS.
- V. G. Silva, R. O. Silva, S. R. B. Damasceno, N. S. Carvalho, R. S. Prudeîncio, K. S. Aragão, M. A. Guimarães, S. A. Campos, L. M. C. Véras, M. Godejohann, J. R. S. A. Leite, A. L. R. Barbosa and J. V. R. Medeiros, J. Nat. Prod., 2013, 76, 1071–1077 CrossRef CAS PubMed.
- S. Kouser, A. Hezam, M. J. N. Khadri and S. A. Khanum, J. Porous Mater., 2022, 29, 663–681 CrossRef CAS.
- X. W. Zhu, D. Luo, X. P. Zhou and D. Li, Coord. Chem. Rev., 2022, 455, 214354 CrossRef CAS.
- P. Molina, A. Tárraga and F. Otón, Org. Biomol. Chem., 2012, 10, 1711–1724 RSC.
- M. Wang, Z. Zhang and W. Zhang, Acc. Chem. Res., 2022, 55, 2708–2727 CrossRef CAS PubMed.
- O. F. Al Sharif, L. M. Nhari, R. M. El-Shishtawy and A. M. Asiri, Mater. Today Chem., 2023, 29, 101453 CrossRef CAS.
- Y. D. Lin, W. W. Tsai and C. W. Lu, Chem.–Eur. J., 2023, 29, e202203040 CrossRef CAS PubMed.
- B. Zhang, C. Wan, Q. Wang, S. Zhang, Z. Zha and Z. Wang, Acta Chim. Sin., 2012, 70, 2408–2411 CrossRef CAS.
- H. Huang, X. Ji, W. Wu and H. Jiang, Adv. Synth. Catal., 2013, 355, 170–180 CrossRef CAS.
- J. Cao, X. Zhou, H. Ma, C. Shi and G. Huang, RSC Adv., 2016, 6, 57232–57235 RSC.
- C. T. F. Salfeena, R. Jalaja, R. Davis, E. Suresh and S. B. Somappa, ACS Omega, 2018, 3, 8074–8082 CrossRef CAS PubMed.
- Z. Yang, J. Zhang, L. Hu, A. Li, L. Li, K. Liu, T. Yang and C. Zhou, J. Org. Chem., 2020, 85, 5952–5958 CrossRef CAS PubMed.
- V. D. Kadu, G. A. Mali, S. P. Khadul and G. J. Kothe, RSC Adv., 2021, 11, 21955–21963 RSC.
- M. M. Heravi, F. Derikvand and F. F. Bamoharram, J. Mol. Catal. A: Chem., 2007, 263, 112–114 CrossRef CAS.
- B. Sadeghi, B. B. F. Mirjalili and M. M. Hashemi, Tetrahedron Lett., 2008, 49, 2575–2577 CrossRef CAS.
- T. J. Donohoe, M. A. Kabeshov, A. H. Rathi and I. E. D. Smith, Org. Biomol. Chem., 2012, 10, 1093–1101 RSC.
- C. F. Claiborne, N. J. Liverton and K. T. Nguyen, Tetrahedron Lett., 1998, 39, 8939–8942 CrossRef CAS.
- X. Chen, Z. Wang, H. Huang and G. J. Deng, Adv. Synth. Catal., 2018, 360, 4017–4022 CrossRef CAS.
- M. Wang, L. Li, J. Lu, N. Luo, X. Zhang and F. Wang, Green Chem., 2017, 19, 5172–5177 RSC.
- S. Samanta, D. Roy, S. Khamarui and D. K. Maiti, Chem. Commun., 2014, 50, 2477–2480 RSC.
- R. Sarkar and C. Mukhopadhyay, Eur. J. Org. Chem., 2015, 2015, 1246–1256 CrossRef CAS.
- V. D. Kadu, S. P. Khadul, G. J. Kothe and G. A. Mali, Asian J. Org. Chem., 2022, 11, e202200162 CrossRef CAS.
- V. D. Kadu, N. C. Sankala, M. G. Hublikar, S. I. Bansode and R. B. Bhosale, Synth., 2023, 56, 2277–2283 CrossRef.
- S. Adhikary, L. Majumder, S. Pakrashy, R. Srinath, K. Mukherjee, C. Mandal and B. Banerji, ACS Omega, 2020, 5, 14394–14407 CrossRef CAS PubMed.
- Y. Zhang, T. Zhang and S. Das, Chem, 2022, 8, 3175–3201 CAS.
- V. D. Kadu, ChemistrySelect, 2022, 7, e202104028 CrossRef CAS.
- S. Liu, Z. Zhao and Y. Wang, Chem.–Eur. J., 2019, 25, 2423–2441 CrossRef CAS PubMed.
- Z. Tashrifi, M. M. Khanaposhtani, B. Larijani and M. Mahdavi, Tetrahedron, 2021, 84, 131990 CrossRef CAS.
- V. D. Kadu, A. A. Patil and P. R. Shendage, J. Mol. Struct., 2022, 1267, 133502 CrossRef CAS.
- V. D. Kadu, S. N. Chandrudu, M. G. Hublikar, D. G. Raut and R. B. Bhosale, RSC Adv., 2020, 10, 23254–23262 RSC.
- V. D. Kadu, M. S. Gund and A. S. Godage, ChemistrySelect, 2021, 6, 11954–11960 CrossRef CAS.
- X. Zhang, Z. Wang, K. Xu, Y. Feng, W. Zhao, X. Xu, Y. Yan and W. Yi, Green Chem., 2016, 18, 2313–2316 RSC.
|
This journal is © The Royal Society of Chemistry 2024 |
Click here to see how this site uses Cookies. View our privacy policy here.