DOI:
10.1039/D4RA04499A
(Paper)
RSC Adv., 2024,
14, 33747-33750
Efficient synthesis of β-aminonitriles from arynes and imines in acetonitrile†
Received
19th June 2024
, Accepted 24th September 2024
First published on 23rd October 2024
Abstract
Multicomponent reactions are powerful strategies for synthesizing complex molecules in an efficient manner. In this work, we investigate a novel multicomponent reaction involving arynes, imines, and nitriles, leading to chiral β-aminonitriles. Notably, two new bonds (C–C and C–N) are formed in one step without the use of metal catalysts, showing the great potential of this transformation. We demonstrate that this synthetic methodology is compatible with different arynes and imines, and propose a reasonable reaction mechanism initiated by the nucleophilic addition of the imine to the aryne.
Introduction
(5 + 2) Cycloadditions are useful tools for the synthesis of seven-membered rings,1 which are relevant building blocks in natural products and pharmaceuticals. One of the synthetic protocols to this aim involves the reaction between a vinylcyclopropane derivative and an alkyne.2 Due to our long-standing interest in aryne cycloadditions,3 we wonder if a similar (5 + 2) cycloaddition could be carried out by reacting a cyclopropyl imine I with an aryne II (Scheme 1). Beyond its theoretical interest, this question has practical importance, since the potential (5 + 2) cycloadduct would be a benzazepine III, a very important building block in pharmaceuticals.4 Although most (5 + 2) reactions involving vinylcyclopropanes are catalyzed by transition metals,2 considering the nucleophilicity of imines and the high electrophilicity of benzyne,5 we decided to attempt the cycloaddition without catalysis. However, when this reaction was performed, the expected benzazepine III was not obtained. Instead, a β-aminonitrile IV was isolated as a major product. Apparently, a three-component reaction involving the imine, the aryne and acetonitrile took place. Similar multicomponent reactions involving arynes have been reported previously.6,7 Recent developments in multicomponent reactions have demonstrated various strategies for complex molecular synthesis. For instance, a radical cascade reaction for β-amino acids using Ni/diboron catalysis,8 or an efficient spirocyclization of N-benzylacrylamides with N-halosuccinimides under metal-free conditions.9 Additionally, a photoinduced coupling reaction for C–H heteroarylation of enamines,10 and a radical-polar crossover method for fluoropyrazoles, both utilizing CF2Br2 as a new carbon source, have been introduced.11 Unlike these approaches that often involve metals or specific reagents, our research presents a novel, metal-free multicomponent reaction using arynes, imines, and nitriles to efficiently synthesize chiral β-aminonitriles, offering improved scope and yield.
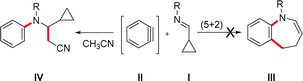 |
| Scheme 1 Reaction of benzyne with a cyclopropyl imine. | |
β-Aminonitriles are privileged skeletons that can be found in many bioactive compounds, such as ruxolitinib,12 nitroquinoline and isoindole derivatives.13,14 Moreover, the nitrile group presented in β-aminonitriles can be reduced to an amine or hydrolyzed to the corresponding amide, adding extra value in the field of the synthesis of natural products and bioactive compounds.15,16 For instance, β-amino acids are part of Taxol®,17 one of the most worldwide studied chemotherapeutic drug and protease inhibitor of different retroviruses, such as HIV.18,19
The novelty of this multicomponent reaction and the interest of β-aminonitriles prompted us to investigate the scope and the mechanism of this transformation.
Results and discussion
Initially, as imine we choose the cyclopropyl derivative 1a while for the generation of benzyne 3a we choose the method based on the fluoride-induced decomposition of o-(trimethylsilyl)phenyl triflate (2a).20,21 When a solution of imine 1a (1 equiv.) and benzyne precursor 2a (1 equiv.) in acetonitrile was treated with CsF (3 equiv.) and stirred at room temperature for 16 h, a mixture of products was obtained. The chromatographic separation led to the isolation of products 4aa as a racemic mixture, 5a and 6a in 41, 12 and 11% yield, which were confirmed by NMR and mass spectra (Fig. S1–S11†), ruling out the formation of a benzazepine by (5 + 2) cycloaddition. Increasing the ratio of reactants 1a
:
2a to 1.5
:
1 and raising the temperature to 60 °C for 16 h improved the yield of isolated racemic mixture 4aa to 88% (Scheme 2).
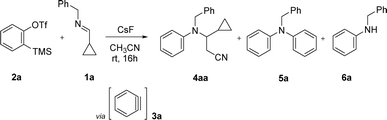 |
| Scheme 2 Reaction of imine 1a with triflate 2a. | |
Encouraged by the high yield of the unexpected product 4aa, we decided to explore the scope of this multicomponent reaction with different arynes. Therefore, we performed the reaction of imine 1a and the arynes 3b–d, generated from the corresponding easily accessible o-(trimethylsilyl)aryl triflates 2b–d (see ESI for details†), obtaining the results shown in Table 1. Symmetrically substituted aryne 3b (Table 1, entry 1) reacted with imine 1a to give the corresponding nitrile 4ab (50%). Asymmetric aryne 3c reacted with imine 1a to give a 1
:
1 mixture of regioisomers 4ac in 58% yield (Table 1, entry 2). When asymmetric aryne 3d was generated in the presence of imine 1a compound 4ad was obtained regioselectively in 48% yield (Table 1, entry 3). It is well known that substitution in position 4 of benzyne (i.e. 3c) has little influence in the regioselectivity of an aryne nucleophilic addition, while a methoxy substituent in position 3 (i.e. 3d) orientates the nucleophilic attack to position 1.5–7 Therefore, the isolation of compounds 4ac and 4ad strongly supports a mechanism initiated by the nucleophilic addition of the imine to the aryne.
Table 1 Reaction of imine 1a and aryne precursors 2b–da
Reaction conditions: imine 1a (1.5 equiv.), aryne precursor 2 (1 equiv.), CsF (3 equiv.) in CH3CN (0.05 M) at 60 °C, 16 h. |
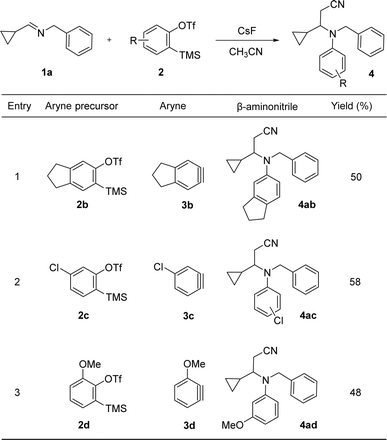 |
The next step was to investigate the scope of the reaction with several imines. In particular, we proposed experiments to test whether the cyclopropyl or the benzyl group are necessary moieties in 1a for the course of the reaction. Thus, the reaction of imine 1b (R2 = C8H17) with benzyne precursor 2a in the presence of acetonitrile led to the formation of compound 4ba in 64% yield (Table 2, entry 1), confirming that the benzyl group in 1a can be replace by an alkyl group in 1b. On the other hand, the multicomponent reaction of imine 1c (R2 = Ph) and aryne precursor 2a in the presence of acetonitrile led to aminonitrile 4ca, isolated in 47% yield (Table 2, entry 2). The substitution of the cyclopropyl group in 1a by a butyl in 1d (R1 = C4H9) led to the corresponding product 4da in 20% yield (Table 2, entry 3). Finally, benzylidene imines 1e,f react with benzyne precursor 2a to afford the aminonitriles 4ea and 4fa, in 40 and 68% yields, respectively (Table 2, entries 4 and 5).
Table 2 Reaction of imines 1b–f and aryne precursor 2a
Reaction conditions: imine (1.5 equiv.), aryne precursor 2 (1 equiv.), CsF (3 equiv.) in CH3CN (0.05 M) at 60 °C, 16 h. |
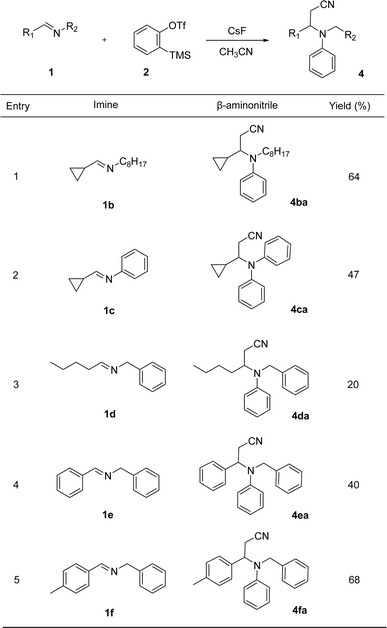 |
Then, considering these findings and the reaction conditions employed in this multicomponent reaction, we proposed the mechanism depicted in Scheme 3. First, fluoride-induced decomposition of triflate 2 afforded aryne 3, which is highly electrophilic species.20,21 Then, the nucleophilic addition of the imine 1 to the aryne 3, should give the zwitterionic structure 7, which would act as a base against acetonitrile, thus generating iminium cation 8 and the conjugated base of acetonitrile. Nucleophilic attack of this base to the electrophilic position of iminium 8, would afford β-aminonitrile 4. Previous works in which the conjugated base of acetonitrile was added to aziridinium,22 isoquinolinium or pyridazinium ions support this mechanistic proposal.23,24
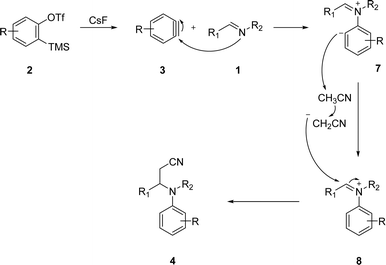 |
| Scheme 3 Proposed mechanism for the formation of the β-aminonitriles. | |
To confirm the mechanistic hypothesis, we designed new reactions using propionitrile and benzonitrile as solvents. According to the proposal, zwitterion 7 should react with propionitrile to form a carbanion in the α position to the CN group, which would attack the iminium ion 8 to give 9 (Scheme 4). In fact, when this reaction was performed under the standard conditions, 9 was obtained in 35% yield. This lower yield can be due to the lower acidity of propionitrile and to its mayor steric hindrance.
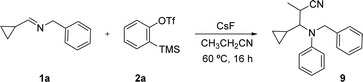 |
| Scheme 4 Reaction of imine 1a with triflate 2a in propionitrile. | |
On the other hand, as expected, when the reaction was carried out with benzonitrile, the formation of the β-aminonitrile was not detected. Lacking hydrogen in α to the CN group, it is not possible to carry out the proton abstraction, preventing the evolution of the reaction towards the corresponding β-aminonitrile. These results are in good accordance with the mechanism proposed above (Scheme 3).
Finally, we focus our attention into minor products 5 and 6. It is known that imines 1a–f react with benzyne to give diarylamines.25 According to this precedent, compound 5 could be formed by the reaction of β-aminonitrile 4 with benzyne. However, we proved that 4 does not react with benzyne under the reaction conditions. Probably 5 was formed in the reaction mixture by arylation of amine 6, which in turn could be formed by hydrolysis of 1.
Conclusions
Overall, we have studied a new multicomponent transformation based on the reaction of imines, nitriles and arynes to obtain chiral β-aminonitriles. It was found that the reaction is compatible with species of different electronic richness. In particular, the reaction with an ortho-substituted aryne, such as 3-methoxybenzyne 3d, is regioselective, while a meta-substituted aryne, such as 4-chlorobenzyne 3c, leads to a mixture of regioisomers. On the other hand, regarding the use of imines, the reaction is compatible with aromatic and aliphatic imines. It is worth to mention that a structural requirement should be maintained, that is, the nitrile group must present α hydrogens to the CN group to obtain the β-aminonitrile.
Data availability
Detailed experimental procedures and characterization data for all novel compounds are included in ESI.†
Author contributions
I. J. G. and C. M. performed the experiments and characterized the novel compounds. I. J. G. wrote the original draft. Di. P. designed and supervised the study. Do. P., E. G., and Di. P. reviewed and edited the original draft and obtained funding.
Conflicts of interest
There are no conflicts to declare.
Acknowledgements
Financial support from the Spanish Agencia Estatal de Investigación (PID2019-107338RB-C62, PID2019-110037GB-I00, PID2022-140845OB-C62 and PCI2019-111933-2), the Xunta de Galicia (Centro de Investigación do Sistema Universitario de Galicia, 2023-2027, ED431G 2023/03 and Oportunius Program) and the European Union (European Regional Development Fund – ERDF), is gratefully acknowledged. I. J. G. acknowledges the Spanish Ministry of Universities for a Beatriz Galindo (BG22/000147).
Notes and references
-
(a) K. E. O. Ylijoki and J. M. Stryker, Chem. Rev., 2013, 113, 2244–2266 CrossRef CAS PubMed;
(b) H. Pellissier, Adv. Synth. Catal., 2018, 360, 1551–1583 CrossRef CAS;
(c) K. Gao, Y.-G. Zhang, Z. Wang and H. Ding, Chem. Commun., 2019, 55, 1859–1978 RSC.
-
(a) P. A. Wender, H. Takahashi and B. Witulski, J. Am. Chem. Soc., 1995, 117, 4720–4721 CrossRef CAS;
(b) B. M. Trost, J. Waser and A. Meyer, J. Am. Chem. Soc., 2007, 129, 14556–14557 CrossRef CAS PubMed.
-
(a) I. Pozo, E. Guitián, D. Pérez and D. Peña, Acc. Chem. Res., 2019, 52, 2472–2481 CrossRef CAS PubMed;
(b) F. Schulz, F. García, K. Kaiser, D. Pérez, E. Guitián, L. Gross and D. Peña, Angew. Chem., Int. Ed., 2019, 58, 9038–9042 CrossRef CAS PubMed;
(c) D. Pérez, D. Peña and E. Guitián, Eur. J. Org Chem., 2013, 27, 5981–6013 CrossRef.
-
(a) Y. Aramaki, M. Seto, T. Okawa, T. Oda, N. Kanzaki and M. Shiraishi, Chem. Pharm. Bull., 2004, 52, 254–258 CrossRef CAS PubMed;
(b) C. B. Breitenlechner, T. Wegge, L. Berillon, K. Graul, K. Marzenell, W.-G. Friebe, U. Thomas, R. Schumacher, R. Huber, R. A. Engh and B. Masjost, J. Med. Chem., 2004, 47, 1375–1390 CrossRef CAS PubMed;
(c) M. Seto, K. Aikawa, N. Miyamoto, Y. Aramaki, N. Kanzaki, K. Takashima, Y. Kuze, Y. Iizawa, M. Baba and M. Shiraishi, J. Med. Chem., 2006, 49, 2037–2048 CrossRef CAS PubMed;
(d) S. Gómez-Ayala, J. A. Castrillón, A. Palma, S. M. Leal, P. Escobar and A. Bahsas, Bioorg. Med. Chem., 2010, 18, 4721–4739 CrossRef PubMed.
-
(a) H. Pellisier and M. Santelli, Tetrahedron, 2003, 59, 701–730 CrossRef;
(b) R. Sanz, Org. Prep. Proced. Int., 2008, 40, 215–291 CrossRef CAS;
(c) D. Wu, H. Ge, S. H. Liu and J. Yin, RSC Adv., 2013, 3, 22727–22738 RSC;
(d) S. Yoshida and T. Hosoya, Chem. Lett., 2015, 44, 1450–1460 CrossRef CAS.
-
(a) S. S. Bhojgude and A. T. Biju, Angew. Chem., Int. Ed., 2012, 51, 1520–1522 CrossRef CAS PubMed;
(b) A. Bhunia and A. T. Biju, Synlett, 2014, 25, 608–614 CrossRef CAS;
(c) K. Okuma, Y. Maehata, H. Kinoshita, K. Sosogi and N. Nagahora, ChemistrySelect, 2019, 4, 3625–3629 CrossRef CAS;
(d) J. K. Xu, S. J. Li, H. Y. Wang, W. C. Xu and S. K. Tian, Chem. Commun., 2017, 53, 1708–1711 RSC.
-
(a) M. Feng, B. Tang, H.-X. Xu and X. Jiang, Org. Lett., 2016, 18, 4352–4355 CrossRef CAS PubMed;
(b) A. J. Ansari, G. Joshi, P. Sharma, A. K. Maurya, R. K. Metre, V. K. Agnihotri, C. Gupta Chandaluri, R. Kumar, S. Singh and D. M. Sawant, J. Org. Chem., 2019, 84, 3817–3825 CrossRef CAS PubMed;
(c) T. Zheng, J. Tan, R. Fan, S. Su, B. Liu, C. Tana and K. Xu, Chem. Commun., 2018, 54, 1303–1306 RSC;
(d) S. S. Bhojgude, D. R. Baviskar, R. G. Gonnade and A. T. Biju, Org. Lett., 2015, 17, 6270–6273 CrossRef CAS PubMed;
(e) Y. Fang, S.-Y. Wang and S.-J. Ji, Tetrahedron, 2015, 71, 2768–2771 CrossRef CAS;
(f) E. Yoshioka, S. Kohtani and H. Miyabe, Molecules, 2014, 19, 863–880 CrossRef PubMed.
- Y. Hong, Z. C. Liao, J. J. Chen, J. Liu, Y. L. Liu, J. H. Li, Q. Sun, S. L. Chen, S. W. Wang and S. Tang, ACS Catal., 2024, 14, 5491–5502 CrossRef CAS.
- Z. Zhang, W. Zhang, Z. W. Hou and P. L. Wang, J. Org. Chem., 2023, 88, 13610–13621 CrossRef CAS PubMed.
- W. Zuo, L. Zuo, X. Geng, Z. Li and L. Wang, Org. Chem. Front., 2023, 10, 6112–6116 RSC.
- W. Zuo, L. Zuo, X. Geng, Z. Li and L. Wang, Org. Lett., 2023, 25, 6062–6066 CrossRef CAS PubMed.
-
(a) Q. Lin, D. Meloni, Y. Pan, M. Xia, J. Rodgers, S. Shepard, M. Li, L. Galya, B. Metcalf, T. N. Yue, P. Liu and J. Zhou, Org. Lett., 2009, 11, 1999–2002 CrossRef CAS PubMed;
(b) S. Hirasawa, T. Kikuchi and S. Kawazoe, Org. Process Res. Dev., 2019, 23, 2378–2387 CrossRef CAS.
- H. Nakayama, J. Desrivot, C. Bories, X. Franck, B. Figadère, R. Hocquemiller, A. Fournet and P. M. Loiseau, Biomed. Pharmacother., 2007, 61, 186–188 CrossRef CAS PubMed.
- A. A. H. Abdel-Rahman, O. M. Ali and A. A. S. Abdel-Megeed, J. Heterocycl. Chem., 2013, 50, 484–489 CrossRef CAS.
- A. Hager, N. Vrielink, D. Hager, J. Lefranc and D. Trauner, Nat. Prod. Rep., 2016, 33, 491–522 RSC.
- D. C. Blakemore, L. Castro, I. Churcher, D. C. Rees, A. W. Thomas, D. M. Wilson and A. Wood, Nat. Chem., 2018, 10, 383–394 CrossRef CAS PubMed.
-
(a) R. A. Holton, C. Somoza, H. B. Kim, F. Liang, R. J. Biediger, P. D. Boatman, M. Shindo, C. C. Smith, S. Kim, H. Nadizadeh, Y. Suzuki, C. Tao, P. Vu, S. Tang, P. Zhang, K. K. Murthi, L. N. Gentile and J. H. Liu, J. Am. Chem. Soc., 1994, 116, 1597–1598 CrossRef CAS;
(b) Y. J. Hu, C. C. Gu, X. F. Wang, L. Min and C. C. Li, J. Am. Chem. Soc., 2021, 143, 17862–17870 CrossRef CAS PubMed.
- M. I. Aguilar, A. W. Purcell, R. Devi, R. Lew, J. Rossjohn, A. I. Smith and P. Perlmutter, Org. Biomol. Chem., 2007, 5, 2884–2890 RSC.
- D. L. Steer, R. A. Lew, P. Perlmutter, A. I. Smith and M.-I. Aguilar, Curr. Med. Chem., 2002, 9, 811–822 CrossRef CAS.
- Y. Himeshima, T. Sonoda and H. Kobayashi, Chem. Lett., 1983, 12, 1211–1214 CrossRef.
- D. Peña, A. Cobas, D. Pérez and E. Guitián, Synthesis, 2002, 10, 1454–1458 Search PubMed.
- D. Stephens, Y. Zhang, M. Cormier, G. Chavez, H. Arman and O. V. Larionov, Chem. Commun., 2013, 49, 6558–6560 RSC.
- M. Jeganmohan and C.-H. Cheng, Chem. Commun., 2006, 2454–2456 RSC.
- S.-E. Suh and D. M. Chenoweth, Org. Lett., 2016, 18, 4080–4083 CrossRef CAS.
- M. Paz, C. Saá, E. Guitián, L. Castedo and J. M. Saá, Heterocycles, 1993, 36, 1217–1223 CrossRef CAS.
|
This journal is © The Royal Society of Chemistry 2024 |
Click here to see how this site uses Cookies. View our privacy policy here.