DOI:
10.1039/D4RA05339G
(Paper)
RSC Adv., 2024,
14, 30077-30083
Covalent organic polyrotaxanes based on β-cyclodextrin for iodine capture†
Received
23rd July 2024
, Accepted 28th August 2024
First published on 23rd September 2024
Abstract
Herein, covalent organic polyrotaxanes (COPRs) were integrated with supermolecule self-assembly and dynamic imine bond formation to act as absorbents that captured radioactive iodine from water. The aromatic building blocks were initially complexed with β-cyclodextrin (β-CD) to form pseudorotaxanes, which were then condensed with aromatic tri-aldehyde via mechanical grinding and solvothermal synthesis in sequence. The threading of β-CD throughout the polymer skeleton effectively reduced the usage of expensive building blocks and significantly lowered the cost, while also remarkably enhancing the skeleton polarity, which is closely related to many special applications. Impressively, the threading of CD improved the water dispersibility of COPRs, which displayed an abnormally high iodine adsorption capacity. This novel synthetic strategy allows the incorporation of mechanically interlocked CDs into porous polymeric materials, which provides access to low-cost preparations of COPRs with a brand new structure for specific applications.
Introduction
Similar to the biological polymer, covalent organic polymers (COPs) enable the precise integration of individual small molecules into open networks with two-dimensional (2D) and three-dimensional (3D) topological structure programmatically through the combined action of covalent bonds and noncovalent interactions.1–5 With years of development, there has been substantial achievement in the reaction types and methods for the specific synthesis of COPs. Hitherto, thousands of COPs with sequential connections of various building blocks have been prepared through coded reaction systems.6–8 However, to obtain COPs with brand new skeletons or topological structures, which has always served as the driving force for further advancement of COPs, most research is currently focused on tedious monomer design and low-efficiency post-modification of as-synthesized polymer skeletons, as well as novel reaction screening.9–11 To realize rapid progress, is it highly desired to devise efficient strategies that use existing monomers and methods to produce novel structures and functions.
As ‘chemistry beyond the molecules’, supramolecular chemistry, with its unique structures and properties, has produced intensive research interests due to the innovative architectures and various biomedical applications.12 Interlocked molecules such as the rotaxanes, which are formed via the host–guest assembly technique, have been widely studied.13 There has been frequent investigation of numerous polyrotaxanes formed via the covalent polymerization of rotaxanes with other building blocks to construct new materials with novel structures and functions.14
Most reports describe linear polymers, and to date, only a handful of polyrotaxanes with a spatial multidimensional structure have been synthesized.15,16 The vast majority of pseudorotaxanes employed as building blocks are formed through electrostatic interaction.17 Unfortunately, the vast majority of natural or synthetic molecules are uncharged, which severely hinders the development and application potential of these series of materials.18
Nuclear waste contains radioactive pollutants widely generated during the nuclear fission process. One example is radiological iodine, which poses a great threat to ecological systems due to its ultra-long half-lives, and can lead to severe environmental pollution.19 The development of high-efficiency stable materials and techniques for the capture and storage of radioiodine is vital, but remains challenging.20 It is of primary importance to use eco-friendly materials that can promptly absorb waste iodine, and also avoid secondary pollution of the ecological environment caused by waste materials.21,22
Herein, covalent organic polyrotaxanes (COPRs) named COPR-1 and COPR-2, which integrate the advantages of COPs and supramolecular polymer, were well designed and facilely prepared as solid absorbents to remove iodine pollutants from water. COPR-1 and COPR-2, with the inclusion of β-cyclodextrin (β-CD) as a building block, were able to remarkably accelerate the development of multifunctional materials while avoiding the sophisticated design of building blocks or tedious but low-yield post-modification. At the same product mass, the integration of CD significantly reduced the usage amount of real building blocks, thereby significantly lowering the cost. COPRs combine dynamic imine bonds and supramolecular interactions so that brand new structures can be grown, in which the primary-order skeletal structure is determined by the covalent bonds, and the high-order morphology is precisely shaped by supramolecular forces. The brand new structure endowed great application potential with polyrotaxane-covalent organic frameworks (PR-COF) for the capture and separation of radioactive iodine from the environment.
Results and discussion
Scheme 1 presents the typical design and synthesis strategy for COPRs. Two typical COPRs, denoted as COPR-1 and COPR-2, were quantitatively prepared via a two-step sequential synthesis through the acid-catalyzed Schiff-base condensation reaction. Briefly, the C2-symmetric CD inclusions (bearing two amino groups) were pre-polymerized with C3-symmetric 2,4,6-trihydroxybenzene-1,3,5-tricarbaldehyde via mechanical grinding for 30 min under the catalysis of p-methylbenzene sulfonic acid (TMSA).23 Afterwards, the reaction mixture was transferred to a sealed Pyrex tube containing the mixed solvent of alcohol (EtOH) and orthodichlorobenzene (o-DCB) at 120 °C, which was maintained at that temperature for 3 days to synthesize the final COPRs.24 Because of the prepolymerization, uniform introduction of CD into the porous skeleton occurred. The details for the synthesis are given in the ESI.†
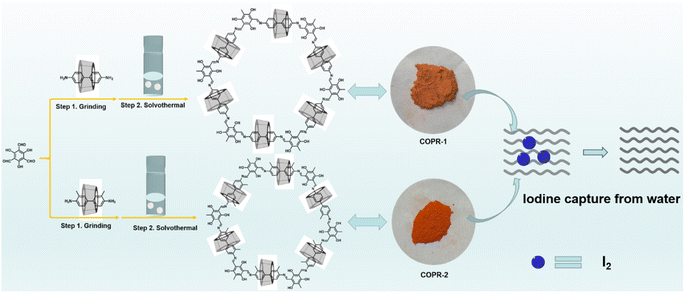 |
| Scheme 1 Typical route and strategy for the synthesis of covalent organic polyrotaxanes (COPRs). | |
The chemical compositions and bond linking of COPR-1 and COPR-2 were characterized by Fourier transform infrared (FT-IR) spectroscopy (Fig. 1a) and solid-state 13C cross-polarization magic angle spinning nuclear magnetic resonance (13C CP/MAS NMR, Fig. 1b) spectroscopy. The FT-IR spectroscopy of COPR-1 and COPR-2 simultaneously shows all the amalgamated features of the CD inclusions and 2,4,6-trihydroxybenzene-1,3,5-tricarbaldehyde. Specifically, both COPRs displayed an evident peak at 1622 cm−1 that corresponded to the newly formed C
N bonds.24 Typical stretching vibrations of the CD rings located at approximately 3368 cm−1 (VOH) and 1031 cm−1 (VC–O–C) were also clearly detected.25 The characteristic vibration bands associated with the CHO groups (1690 cm−1) of 2,4,6-trihydroxybenzene-1,3,5-tricarbaldehyde and NH2 groups (3384, 3322, and 3208 cm−1) of CD inclusion disappeared, further demonstrating the occurrence of aldehyde ammonia Schiff base condensation.26–28 Similarly, apart from the feature signals of aromatic carbon (106 and 141 ppm), the solid-state 13C NMR of COPRs also produced the characteristic resonance of the carbon signal from CD at approximately 72 and 54 ppm.29 These results demonstrated the formation of a CD-threaded porous skeleton.
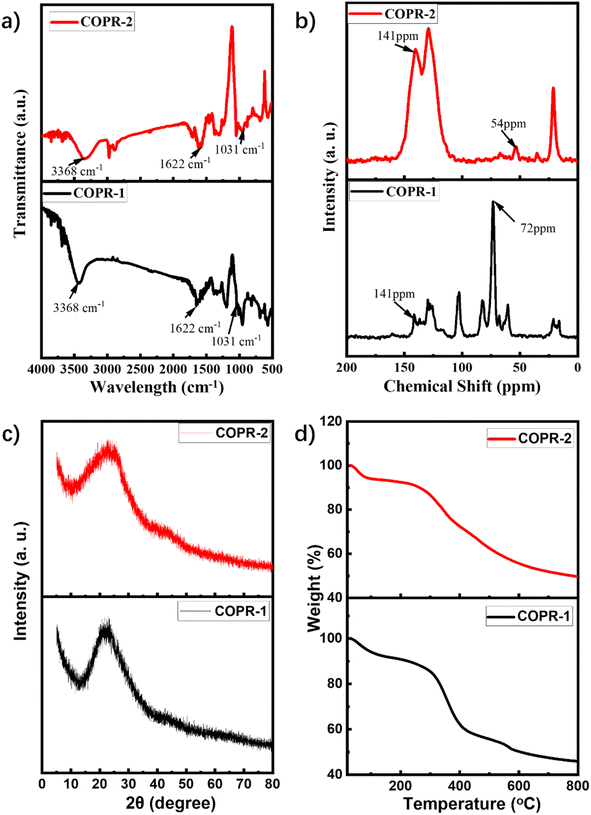 |
| Fig. 1 Physical characterization of COPR-1 and COPR-2: (a) FTIR of COPR-1 and COPR-2. (b) Solid state 13C NMR of COPR-1 and COPR-2. (c) PXRD of COPR-1 and COPR-2. (d) TGA of COPR-1 and COPR-2. | |
The powder X-ray diffraction (PXRD, Fig. 1c) pattern for COPR-1 and COPR-2 only presented strong broad diffraction peaks at 2θ = 25.5°, demonstrating the amorphous structure of the as-synthesized samples.30 The thermogravimetric analysis (TGA) of COPR-1 and COPR-2 displayed a stepwise weight loss. The rapid weight loss occurring below 100 °C was attributed to the evaporation of water strongly absorbed in the O-rich porous networks.31 Afterwards, a relatively flat weight loss at temperatures ranging from 100 to 300 °C was attributed to the decomposition of CD rings threaded in the porous skeleton. Weight loss that occurred at >300 °C was attributed to the thermal decomposition of the conjugated skeleton (Fig. 1d).
The surface and inner micromorphology of COPR-1 and COPR-2 were investigated via transmission electron microscopy (TEM). The TEM images (Fig. 2) revealed that COPR-1 (Fig. 2a–d) and COPR-2 (Fig. 2f–h) were composed of bulk fused by a layered sheet. Specifically, as presented in Fig. 2a–d, COPR-1 displayed a uniformly distributed fringe structure, assignable to the wide threading of CD units into the porous skeleton. As expected, the elemental mapping and corresponding energy dispersive X-ray spectroscopy (EDS) results for COPR-1 (Fig. 2e) and COPR-2 (Fig. 2j) confirmed the homogeneous distribution of C, N, and O over the CD-threaded polymer skeleton. The oxygen content in COPR-1 and COPR-2 was among the highest level in as-reported polymer-based networks.32 The high heteroatom content enabled facile encapsulation of iodine from water (Fig. 3).
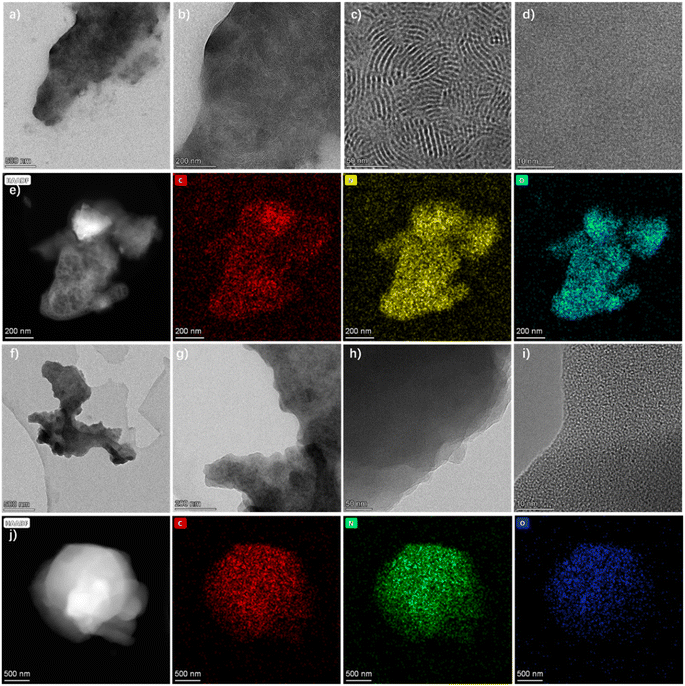 |
| Fig. 2 Electron microscopy characterization of COPR-1 and COPR-2: (a–d) TEM of COPR-1 at different scale bars of 500, 200, 50, and 10 nm, respectively. (e) HAADF elemental mapping of COPR-1. (f–i) TEM of COPR-2 at different scale bars of 500, 200, 50, and 10 nm, respectively. (j) HAADF elemental mapping of COPR-2. | |
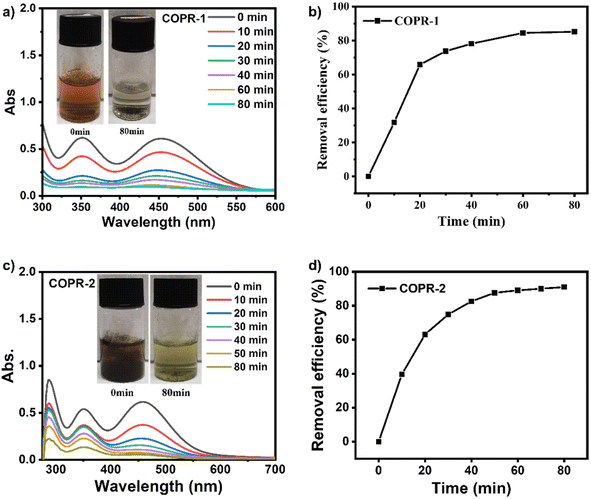 |
| Fig. 3 (a) UV-vis spectra of aqueous iodine solutions before and after the addition of COPR-1. (b) The removal efficiency for COPR-1. (c) UV-vis spectra of aqueous iodine solutions before and after the addition of COPR-2. (d) The removal efficiency for COPR-2. | |
Based on the aforementioned results, the capacity of COPR-1 and COPR-2 to absorb iodine from water was examined using β-CD as a control. Fig. 3 shows that because of the unique O-rich structure, COPR-1 and COPR-2 possessed excellent capacity to absorb saturated I2 solutions, as verified by the obvious color change over the duration of time, which faded from orange to almost colorless within 80 min (insets in Fig. 3a and c). The rate of iodine removal from water significantly increased over time for COPR-1 and COPR-2. Notably, COPR-1 and COPR-2 presented a hierarchical absorption process with a rapid uptake during the initial 20 min, and then a relatively slow increase. Compared with β-CD (Fig. S1†), there was a much faster iodine adsorption rate for COPR-1 and COPR-2. Specifically, the most prominent iodine removal capacity was demonstrated by COPR-2, which rapidly captured over 91% of the iodine from water within 80 min, and was faster than the COPR-1 (85%) and β-CD (74%) samples. All these results revealed that the threading of high-polarity CDs was effective in altering the material properties. Additionally, the efficiency of the COPRs in capturing radioactive iodine was comparable to previously reported efficiencies for various absorbents.33,34
The capacity of the two as-synthesized adsorbents to capture iodine in hexane was evaluated via recording the change in the UV-visible absorption over time. The iodine solution (500 mg L−1) was prepared via dissolving 3 mg of iodine in 3 mL of hexane solution. As the adsorption time progressed, the color of the hexane solution containing iodine gradually faded (Fig. S2†). Notably, the COPRs exhibited rapid adsorption of iodine within the first 20 h, ultimately reaching adsorption equilibrium after 60 h (Fig. S3†). Both COPRs showcased remarkable capabilities for trapping iodine in n-hexane, which approached 400 mg g−1 for COPR-1 and COPR-2, simultaneously.
Then, the recyclability of COPR-1 and COPR-2 was also investigated in detail. The results, illustrated in Fig. S4,† demonstrated that after undergoing three adsorption–elution cycles, both adsorbents exhibited remarkable regenerability, with only a minimal decrease in their adsorption capacities. This finding suggested that these specially designed adsorbents featured excellent recyclability, and were suitable for repeated use. Additionally, the desorption process of both adsorbents in methanol were evaluated (Fig. S5†). The corresponding results indicated that both adsorbents were capable of effectively eluting the adsorbed iodine into a methanol solution within 60 min.
Subsequently, X-ray photoelectron spectroscopy (XPS) was conducted to estimate the capacity to adsorb iodine by the as-synthesized COPRs. As shown in Fig. 4a and c, characteristic signals belonging to I3d were clearly detected from the XPS survey spectrum of COPR-1 and COPR-2, concurrently, demonstrating the capture of iodine from water. The high resolution I3d spectra of COPR-1 (Fig. 4b) and COPR-2 (Fig. 4d) showed obvious I3d3/2 and I3d5/2 splitting peaks located at approximately 632.4 and 620.9 eV, respectively, which revealed that the iodine dissolved in the aqueous solution was strongly adsorbed on the polyrotaxane skeleton.35 Furthermore, the binding energy belonging to I3− (619.4 and 630.1 eV) and I2 (620.6 and 632.1 eV) was also clearly detected, concurrently.36 All our data revealed that the iodine absorbed in the as-synthesized COPRs was mainly in the form of polyiodide anions and iodine monomers, which demonstrated a hybrid iodine capture process for COPR-1 and COPR-2 dominated by physical and chemical adsorption.
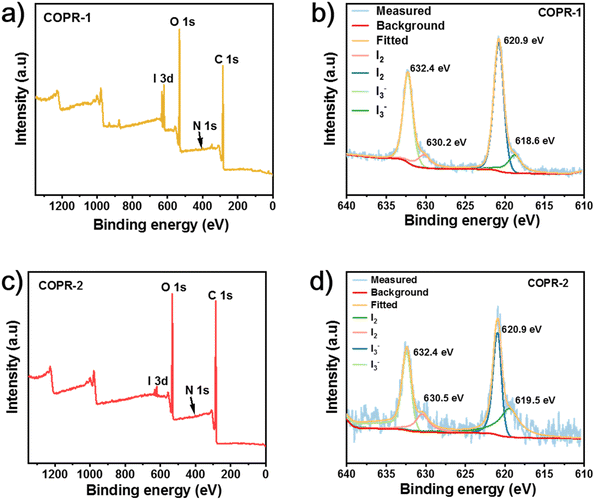 |
| Fig. 4 (a) XPS survey spectra of COPR-1 after iodine adsorption. (b) XPS I3d spectra of COPR-1 after iodine adsorption. (c) XPS survey spectra of COPR-2 after iodine adsorption. (d) XPS I3d spectra of COPR-2 after iodine adsorption. | |
Conclusion
A novel but facile strategy for the concurrent design and synthesis of COPRs with brand new structures and functions was realized by the rational integration of CD-based pseudorotaxane moieties into a COF backbone. COPRs were prepared via a stepwise Schiff base reaction through the integration of mechanical grinding and solvothermal synthesis. Compared with traditional methods, this strategy not only enables the scalable preparation of polyrotaxanes with controllable structure and function, but it also presents a highly effective procedure for controlling the polarity of a porous skeleton for specific applications. This work bridges the field between mechanically interlocked CDs and polymeric materials, and provides a protocol for the low-cost preparation of polyrotaxanes with targeted functions.
Data availability
The authors declare that all the experimental data are available.
Conflicts of interest
There are no conflicts to declare.
Acknowledgements
This work was supported by funding from the Natural Science Foundation of Shandong Province (ZR2020QB067) and the Shandong Second Medical University Public Sent Domestic Study and Visiting Project (20237-21).
References
- Q. Song, D. Xu, W. Bian, J. Wang, G. Peng, M. Sun, N. Wang and B. Zhou, Cationic Cu(I)-covalent organic framework as self-enhanced synergetic Photothermal/Phtodynamic/Cationic/Enzymatic antibacterial agent, React. Funct. Polym., 2023, 192, 105692 CrossRef.
- Y. Zhao, W. Li, Y. Li, T. Qiu, X. Mu, Y. Ma, Y. Zhao, J. Zhang, J. Zhang, Y. Li and H. Tan, 3D Covalent Polyoxovanadate-Organic Framework as Anode for High-Performance Lithium-Ion Batteries, Adv. Funct. Mater., 2023, 2306598 CrossRef.
- J. Sun, L. Shi, N. Wang, C. Miao, X. Xu, J. Yang, J. Wang and B. Zhou, A biodegradable ferrocene-based covalent organic framework as self-gated carrier for controlled drug delivery towards synergetic cancer enzymatic and chemical therapies, J. Drug Delivery Sci. Technol., 2023, 87, 104881 CrossRef.
- X. Xu, Q. Cui, H. Chen and N. Huang, Carborane-Based Three-Dimensional Covalent Organic Frameworks, J. Am. Chem. Soc., 2023, 145(44), 24202–24209 CrossRef.
- F. Haase and B. V. Lotsch, Solving the COF trilemma: towards crystalline, stable and functional covalent organic frameworks, Chem. Soc. Rev., 2020, 49, 8469–8500 RSC.
- H. Lou, L. Chu, W. Zhou, J. Dou, X. Teng, W. Tan and B. Zhou, A diselenium-bridged covalent organic framework with pH/GSH/photo-triple-responsiveness for highly controlled drug release toward joint chemo/photothermal/chemodynamic cancer therapy, J. Mater. Chem. B, 2022, 10, 7955–7966 RSC.
- J. Wang, X. Zhang, N. Wang, J. Wang, H. Kong, J. Li, G. Zhang, H. Du, B. Zhou and B. Wang, Nanoporous BODIPY-Based Cationic Porous Organic Polymer Composites as Photocontrolled NO-Releasing Platforms for Wound Healing and Antimicrobial Applications, ACS Appl. Nano Mater., 2023, 6, 16716–16729 CrossRef.
- C. S. Diercks and O. M. Yaghi, The atom, the molecule, and the covalent organic framework, Science, 2017, 355, eaal1585 CrossRef PubMed.
- Y. Li, W. Chen, G. Xing, D. Jiang and L. Chen, New synthetic strategies toward covalent organic frameworks, Chem. Soc. Rev., 2020, 49, 2852–2868 RSC.
- J. Wang and S. Zhuang, Covalent organic frameworks (COFs) for environmental applications, Coord. Chem. Rev., 2019, 400, 213046 CrossRef.
- J. L. Segura, S. Royuela and M. M. Ramos, Post-synthetic modification of covalent organic frameworks, Chem. Soc. Rev., 2019, 48, 3903–3945 RSC.
- D. B. Amabilino, D. K. Smith and J. W. Steed, Supramolecular materials, Chem. Soc. Rev., 2017, 46, 2404–2420 RSC.
- J. F. Stoddart, Mechanically interlocked molecules (MIMs)—molecular shuttles, switches, and machines, Angew. Chem., Int. Ed., 2017, 56, 11094–11125 CrossRef PubMed.
- J. Sawada, D. Aoki, Y. Sun, K. Nakajima and T. Takata, Effect of coexisting covalent cross-links on the properties of rotaxane-cross-linked polymers, ACS Appl. Polym. Mater., 2019, 2, 1061–1064 CrossRef.
- G. Das, S. Kumar Sharma, T. Prakasam, F. Gándara, R. Mathew, N. Alkhatib, N. Saleh, R. Pasricha, J. C. Olsen, M. Baias, S. Kirmizialtin, R. Jagannathan and A. Trabolsi, A polyrotaxanated covalent organic network based on viologen and cucurbit[7]uril, Commun. Chem., 2019, 2, 106 CrossRef.
- S. An, C. Lu, Q. Xu, C. Lian, C. Peng, J. Hu and H. Liu, Constructing catalytic crown ether-based covalent organic frameworks for electroreduction of CO2, ACS Energy Lett., 2021, 6, 3496–3502 CrossRef CAS.
- P. Martinez-Bulit, C. A. O'Keefe, K. Zhu, R. W. Schurko and S. J. Loeb, Solvent and steric influences on rotational dynamics in porphyrinic metal–organic frameworks with mechanically interlocked pillars, Cryst. Growth Des., 2019, 19, 5679–5685 CrossRef CAS.
- A. Khaligh, Y. Sheidaei and D. Tuncel, Covalent Organic Framework Constructed by Clicking Azido Porphyrin with Perpropargyloxy-Cucurbit[6]uril for Electrocatalytic Hydrogen Generation from Water Splitting, ACS Appl. Energy Mater., 2021, 4, 3535–3543 CrossRef CAS.
- C. Miao, N. Tang, L. Shi, S. Wang, P. Ji, J. Zhang, Z. Wang and B. Zhou, Ionic Functionalized Magnetic Porous Polymers as Advanced Materials for High-Efficiency Water Decontamination: Bactericidal and Iodine Adsorption, ACS Appl. Polym. Mater., 2023, 5, 8679–8692 CrossRef.
- C. Miao, L. Chu, D. Guo, X. Ding, W. Guo, S. Wang, J. Sheng, J. Zhang, Z. Wang and B. Zhou, Ferrocene-based porous polymer as advanced environmental remediation material for self-enhanced synergetic photothermal-enzymatic antibacterial and reversible iodine capture, J. Environ. Chem. Eng., 2023, 11, 110514 CrossRef.
- P. Wang, Q. Xu, Z. Li, W. Jiang, Q. Jiang and D. Jiang, Exceptional iodine capture in 2D covalent organic frameworks, Adv. Mater., 2018, 30, 1801991 CrossRef.
- C. Wang, Y. Wang, R. Ge, X. Song, X. Xing, Q. Jiang and D. Jiang, A 3D covalent organic framework with exceptionally high iodine capture capability, Chem.–Eur. J., 2018, 24, 585–589 CrossRef.
- D. B. Shinde, H. B. Aiyappa, M. Bhadra, B. P. Biswal, P. Wadge, S. Kandambeth, B. G. T. Kundu, S. Kurungot and R. Banerjee, A mechanochemically synthesized covalent organic framework as a proton-conducting solid electrolyte, J. Mater. Chem. A, 2016, 4, 2682 RSC.
- S. Ji, H. Qian, C. Yang, X. Zhao and X. Yan, Thiol−Ene Click Synthesis of Phenylboronic Acid-Functionalized Covalent Organic Framework for Selective Catechol Removal from Aqueous Medium, ACS Appl. Mater. Interfaces, 2019, 11, 46219–46225 CrossRef CAS.
- R. Shi, L. Liu, Y. Lu, C. Wang, Y. Li, L. Li, Z. Yan and J. Chen, Nitrogen-rich covalent organic frameworks with multiple carbonyls for high-performance sodium batteries, Nat. Commun., 2020, 11, 178 CrossRef CAS.
- S. Cui, X. Sun, K. Li, D. Gou, Y. Zhou, J. Hu and Y. Liu, Polylactide nanofibers delivering doxycycline for chronic wound treatment, Mater. Sci. Eng., C, 2019, 104, 109745 CrossRef CAS.
- L. Wu, F. Li, B. R. Morrow, S. Jiang, T. L. Hottel, F. Garcia-Godoy and L. Hong, A novel antimicrobial and remineralizing toothpaste containing CaCl2/chitosan microspheres, Am. J. Dent., 2018, 31, 149 Search PubMed.
- H. Luo, W. Ji, W. Guo, P. Chen, Z. Zhang, X. Xu, B. Yue, W. Tan and B. Zhou, A photoactive Dual-cationic Covalent Organic Framework Encapsulated Sodium Nitroprusside as controllable NO-releasing material for joint cation/photothermal/NO antibacterial therapy, Microporous Mesoporous Mater., 2022, 346, 112281 CrossRef CAS.
- Q. Yue, J. Yu, Q. Zhu, D. Xu, M. Wang, J. Bai, N. Wang, W. Bian and B. Zhou, Polyrotaxanated covalent organic frameworks based on β-cyclodextrin towards high-efficiency synergistic inactivation of bacterial pathogens, Chem. Eng. J., 2024, 486, 150345, DOI:10.1016/j.cej.2024.150345.
- Y. Wang, S. Zhuo, J. Hou, W. Li and Y. Ji, ACS Appl. Mater. Interfaces, 2019, 11, 48363–48369 CrossRef.
- L. Wang, L. Shi, T. Guo, J. Yuan, B. Zhou and J. Zhang, Near-infrared active ferrocenyl porous organic polymer with photothermal enhanced enzymatic activity for combination antibacterial application, RSC Adv., 2023, 13, 26445–26454 RSC.
- S. An, Q. Xu, Z. Ni, J. Hu, C. Peng, L. Zhai, Y. Guo and H. Liu, Construction of Covalent Organic Frameworks with Crown Ether Struts, Angew. Chem., Int. Ed., 2021, 60, 9959–9963 CrossRef.
- G. Li, C. Yan, B. Cao, J. Jiang, W. Zhao, J. Wang and T. Mu, Highly efficient I 2 capture by simple and low-cost deep eutectic solvents, Green Chem., 2016, 18(8), 2522–2527 RSC.
- C. Yan and T. Mu, Investigation of ionic liquids for efficient removal and reliable storage of radioactive iodine: a halogen-bonding case, Phys. Chem. Chem. Phys., 2014, 16(11), 5071–5075 RSC.
- C. Miao, N. Tang, L. Shi, S. Wang, P. Ji, J. Zhang, Z. Wang and B. Zhou, Ionic Functionalized Magnetic Porous Polymers as Advanced Materials for High-Efficiency Water Decontamination: Bactericidal and Iodine Adsorption, ACS Appl. Polym. Mater., 2023, 5, 8679–8692 CrossRef CAS.
- C. Miao, L. Chu, D. Guo, X. Ding, W. Guo, S. Wang, J. Sheng, J. Zhang, Z. Wang and B. Zhou, Ferrocene-based porous polymer as advanced environmental remediation material for self-enhanced synergetic photothermal-enzymatic antibacterial and reversible iodine capture, J. Environ. Chem. Eng., 2023, 11, 110514 CrossRef CAS.
|
This journal is © The Royal Society of Chemistry 2024 |
Click here to see how this site uses Cookies. View our privacy policy here.